全文HTML
--> --> -->迄今为止, 一些材料已经被用作人造突触装置中的活性层. 有机材料, 如聚–3已基噻吩(P3HT)[13], 2, 7-二辛基1苯并噻吩[3, 2-b] 1苯并噻吩(C8-BTBT)[9]等. 无机氧化物, 如氧化锌[14,15], 氧化铟锌 (IZO)[16], 氧化铟镓锌(IGZO)[17], 过渡金属氧化物[18,19]等. 二维材料, 如石墨烯[20-22], 黑磷[23], 钙钛矿材料[24]等. 其中过渡金属氧化物因其独特的层状结构和固有性质使得其在光电水解[25]、锂离子电池[26,27]及场效应晶体管[28]等方面有重要应用. MoO3作为典型的过渡金属氧化物, 已经在化学反应和电子器件中得到了广泛地应用, 例如: 由纳米MoO3和Al粉末制备的含能薄膜可以在短时间内释放大量的能量, 在国防和信息安全领域显示了广泛的前景; 同时作为半导体材料也被广泛地应用于制备人工突触器件中. 在已报道的MoO3人工突触器件中, Li等[29]采用机械剥落法制备; Yang及其团队采用化学气相沉积(CVD)[30]及化学气相传输(CVT)[31]的方式制备MoO3活性层, 构建两端及三端人工突触器件; Wang与其团队[32]以脉冲激光沉积(PLD)的方式制备突触器件中的MoO3薄膜. 本文制备活性层的方法与已报道的方法不同, 以水热法制备MoO3纳米片活性层并且通过TiO2纳米颗粒晶种层来增加MoO3在衬底Si上的附着性[33,34], 制备成本较低, 可以通过调控不同的变量从而来调控产物的尺寸及形貌, 并且高温高压的实验环境有利于生长缺陷少、结晶度较好的晶体. 以此方法所制得的纳米材料具有较大的比表面积, 因此可以提供更大的接触面积, 有较强的界面离子作用. 并且成功模拟了突触的重要功能, 如: 突触后兴奋电流(EPSC)、双脉冲易化(PPF)、脉冲持续时间依赖可塑性(SDDP)、脉冲电压依赖可塑性(SVDP)及脉冲速率依赖可塑性(SRDP), 本工作可能是神经形态研究领域的重要补充.
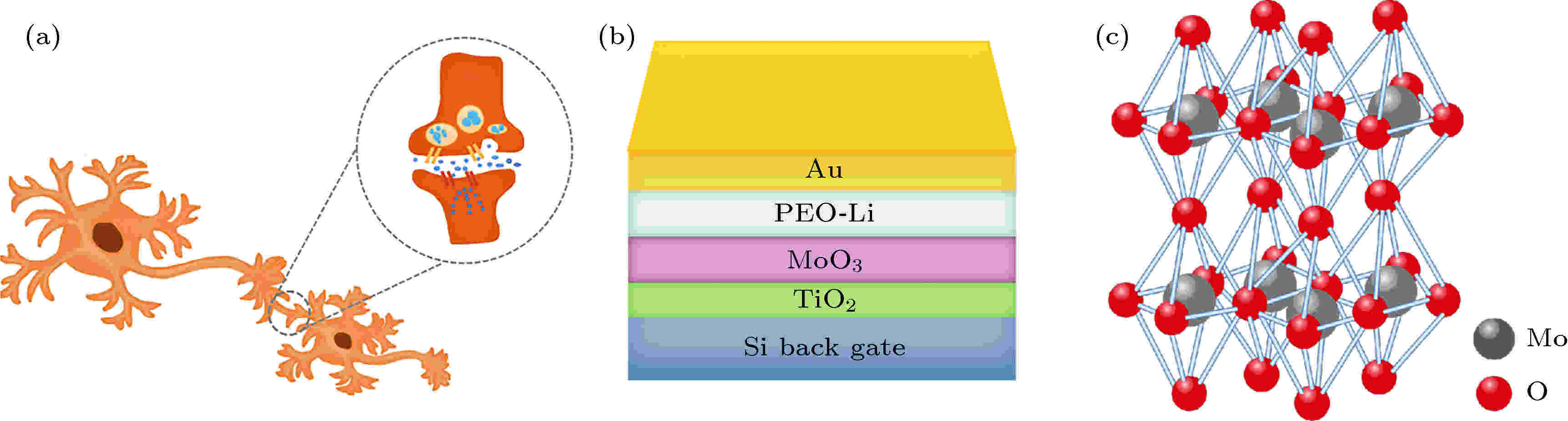
Figure1. (a) Schematic diagram of biological neurons and synapse; (b) schematic diagram of artificial synapse device; (c) schematic diagram of MoO3 structure.
为了模拟生物突触的结构, 设计两端“三明治”结构用于实现人工突触电子器件. 以Au顶电极模拟突触前膜, 高掺杂n型Si衬底模拟突触后膜, MoO3活性层模拟突触间隙, 以溶胶-凝胶法制备的TiO2纳米颗粒晶种层来增加MoO3在衬底Si上的附着性, 通过掺有锂盐的聚环氧乙烷(PEO-Li)固体电解质来修饰活性层较为粗糙的表面, 同时增大了金属电极与活性层的接触面积, 器件结构如图1(b)所示. MoO3晶体是以金属钼原子为中心, 氧原子在角边的八面体为基本结构单元, 各个结构单元之间以链相连, 相似的链边连接成层, 层与层之间以范德瓦耳斯连接. MoO3这种特殊的八面体成链晶体结构使得其具有广延的离子流通和嵌入通道[35], 其结构如图1(c)所示.
4.1.材料晶体结构以及形貌分析
图2(a)给出了XRD测试结果与标准PDF卡片对比之后, 得出MoO3纳米材料的特征衍射峰: 2θ = 12.7°, 23.3°, 25.6°, 27.3°, 33.7°, 49.2°, 51.0°, 55.1°分别与晶面(020), (110), (040), (021), (111), (002), (022), (112), (042)相对应, XRD衍射曲线中显示有较强衍射峰—(040)和(002), 说明在水热过程中MoO3纳米材料的生长具有一定的取向性.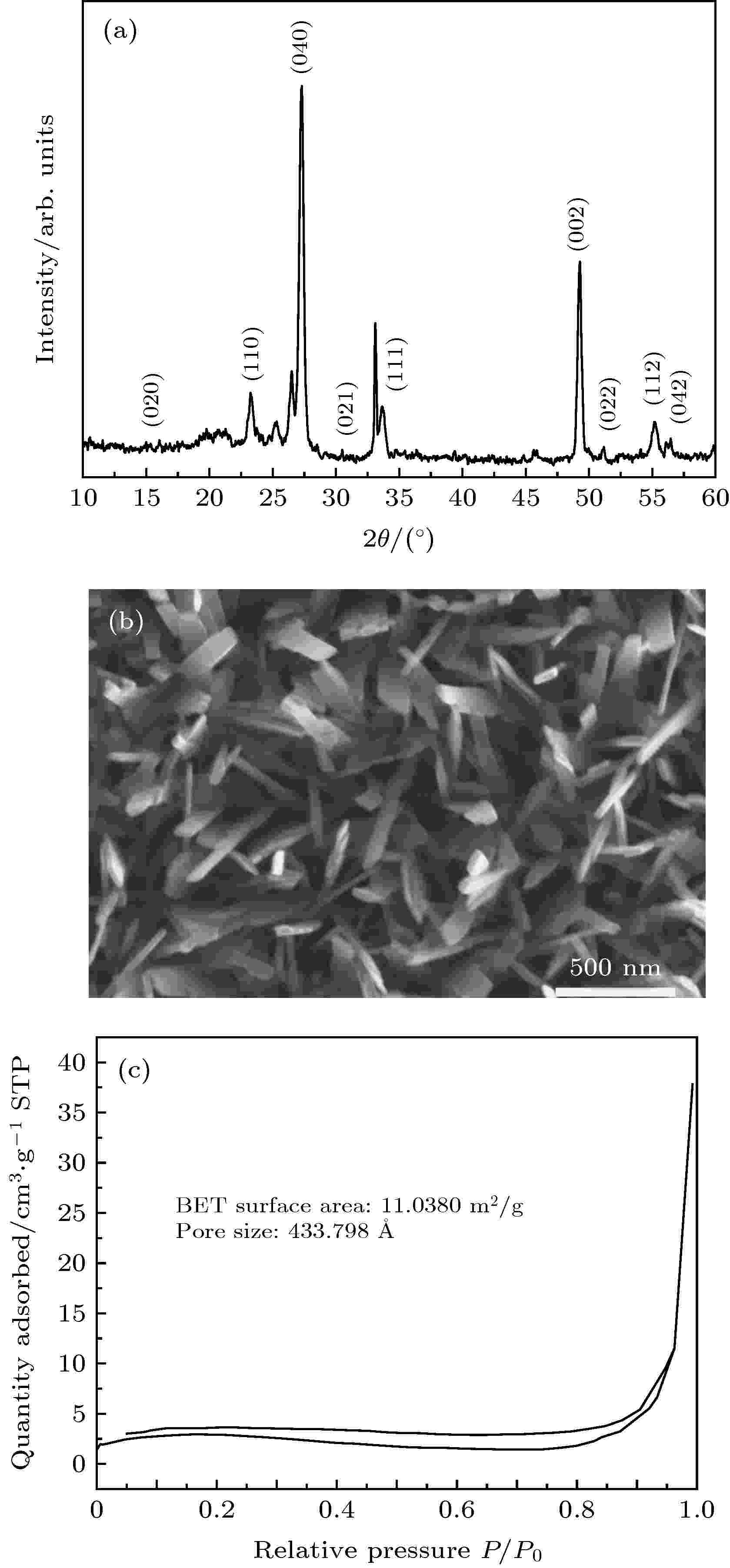
Figure2. (a) XRD of MoO3 nanomaterials; (b) scanning electron microscope image of MoO3 nanomaterials; (c) nitrogen Isothermal absorption and desorption curve of MoO3 nanomaterials.
图2(b)显示了由SEM测量到的MoO3纳米薄膜的表面形貌. 测试结果显示薄膜覆盖较为均匀, 且有呈一定规律排列的趋势. 同时测试结果显示薄膜是由宽约300 nm、长约1 μm的片状物质成. MoO3纳米材料的N2吸附/脱附等温线及相应的Barrett-Joyner-halen(BJH)测试结果如图2(c), 其结果表明该纳米材料的比表面积为11.0380 m2/g, 孔径大小约为433.798 ?, 表明以水热法制备的MoO3纳米材料具有较高比表面积并且为无介孔结构的片状物质.
2
4.2.器件突触性能测试分析
突触可塑性是指突触连接强度可以被持续调节的性质, 会随着自身活动的增强或减弱相应的增强或减弱[37]. 突触可塑性可分为两种: 长程可塑性(LTP)和短程可塑性(STP). STP往往可以实现短时程行为、短时程记忆的重要功能, 可以在大脑接收大量刺激信号时过滤掉不重要的信息, 是提取有价值的信息的关键[38]. EPSC是突触后膜收到刺激后短暂去极化所形成的现象, 是STP的重要表现之一.为了在制备的人工突触电子器件上模拟这种突触兴奋性行为, 以顶电极和底电极作为突触前膜和突触后膜, MoO3活性层模拟突触间隙. 在底电极上施加一个恒定的偏置电压(0.01 V)作为读取电压, 将幅值为1 V的电压施加在顶电极上建立一个临时的外加电场. 外加电压导致了EPSC的产生, 器件受到刺激时电流瞬时增加, 在刺激消失之后突触后电流快速衰退恢复至初始状态如图3(a)所示. 这一现象的产生可能是在器件受到刺激的瞬间, 固体电解质中的Li+在外加电场的作用下有序迁移, 聚集在MoO3表面, 引起MoO3中电子的定向移动从而造成突触后电流的瞬时增加. 消除刺激之后电荷扩散到其平衡位置, 因此电流衰减恢复至初始状态. 这一现象与生物性神经突触中的EPSC现象对应, 一旦适当的刺激到达轴突末端并激活Ca2+通道, 从突触小泡释放的神经递质会迅速扩散穿过突触间隙, 并与突触后细胞膜上的这些受体结合, 最终产生兴奋性突触后电流[39].
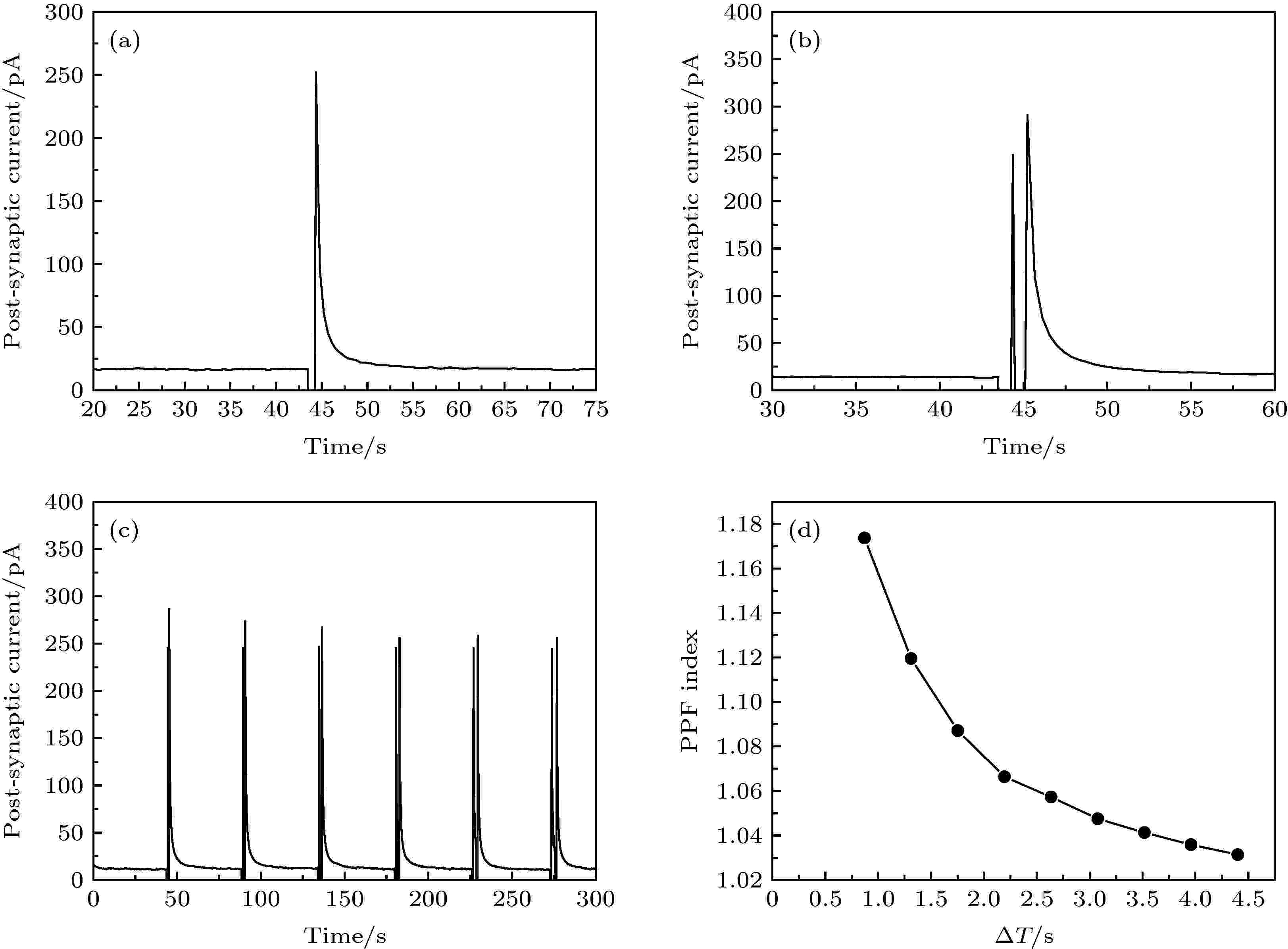
Figure3. (a) EPSC triggered by a single 1 V spike at a MoO3 artificial synapse; (b) PPF triggered by a pair of 1 V spikes at a MoO3 artificial synapse; (c) and (d) PPF and PPF index triggered by spikes with different time intervals and same amplitudes of 1 V.
PPF也是突触短时可塑性的一个重要表现, 在视觉和听觉系统中接收和解读瞬时消息中至关重要[40], PPF体现的是在前突触上施加两次连续的刺激, 第二次刺激造成的突触后兴奋电流高于前一次刺激的现象, 在此器件上模拟的PPF现象如图3(b)所示. 相同幅值电压下的两次刺激, 第二次刺激造成的EPSC与第一次的相比增加17.94%. 在第一次刺激下, Li+的迁移导致MoO3活性层中电子的定向移动, 刺激消失后离子逐渐迁移回到其平衡位置. 但如果第二次刺激在其到达平衡位置之前到达, 会有更多数量的离子在临时电场的作用下发生定向移动从而导致活性层中更多的电子发生定向移动, 所以第二次EPSC高于第一次. 并且高出值的大小由两次之间的时间间隔有关, 越小的时间间隔会造成更大的EPSC[41]. 相同振幅的电压刺激下, 不同时间间隔的PPF如图3(c)所示, 可以很明显的看出, 随着时间间隔的增加第二次EPSC增益逐渐减小, 在这里将增益PPF Index定义为(A2 – A1)/A1, 其中A1是第一次刺激后的EPSC峰值, A2是连续两次刺激后的EPSC峰值.
持续重复的刺激可以使得突触之间的连接性和信息传递性增加, 联系更加紧密[42]. 在神经突触学习规则当中, SRDP描述的是突触前神经元接收不同频率刺激后释放不同数量的神经递质, 从而导致突触后神经元产生的信号电流不同的现象. 在此器件上施加0.01 V的读取电压, 振幅为1 V的刺激电压, 以不同的时间间隔来调制施加刺激的频率, 结果如图4(a)所示. 从实验结果可以看出, 随着施加的刺激频率的降低, 突触后兴奋电流增长的趋势逐渐降低最后趋于平缓. 这一趋势的产生是由于在较高频率的刺激下, 最初产生EPSC的离子在没有回到其平衡位置时下一次刺激就已经到达所以产生了与PPF类似的趋势. 而当施加的刺激频率较低时, 在下一次刺激达到之前, 造成突触后电流的离子大部分已经回到其平衡位置, 所以形成的趋势较为平缓.
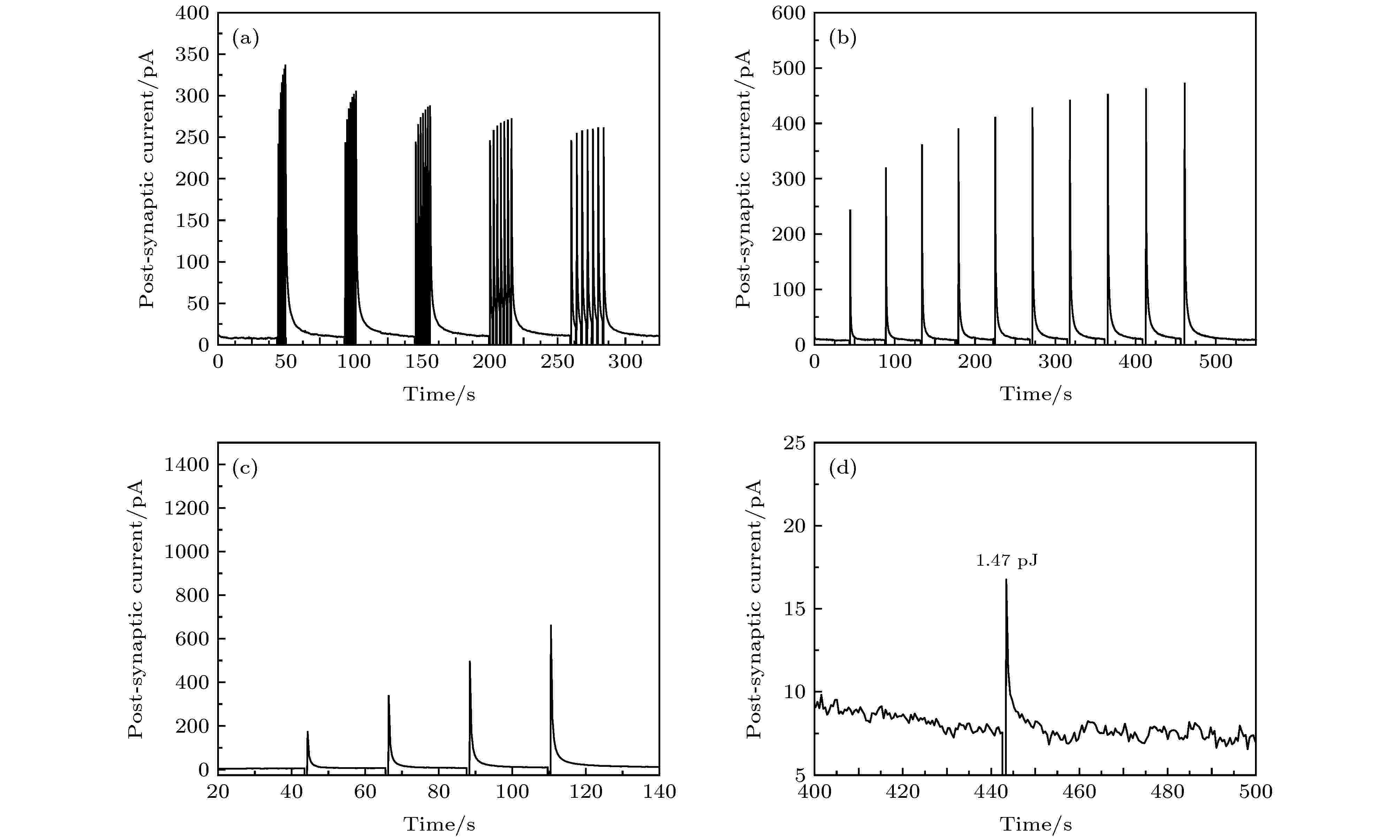
Figure4. (a) SRDP on MoO3 synapses triggered by the number of spikes of 1 V applied at 1, 2, 3, 5, and 8; (b) SDDP triggered by different spike duration time with the same amplitude; (c) SVDP triggered by spikes with amplitudes of 0.5, 1.0, 1.5 V, and 2.0 V on synaptic devices; (d) the excitatory postsynaptic current when the applied spike is 0.2 V and the energy consumed to complete a signal transmission.
同时, 在生物突触中增加刺激的持续时间和刺激强度也会增加突触前膜释放的神经递质的数量, 这两种现象也是人工突触可塑性中重要的表现, 分别为SDDP和SVDP. 在此器件上模拟的SDDP及SVDP的结果分别如图4(b)和图4(c)所示. 从实验结果可以看出传递信息的神经递质的数量是有限的, 随着持续时间的逐渐增加, 引起的EPSC的强度由逐渐增加到趋于平缓, 在实验过程中随着所施加的刺激持续时间的增加, 可移动的离子数目逐渐减少, 最后接近全部的离子都参与了信号的传递. 并且脉冲振幅的增大也会引起EPSC的增加.
能量消耗也是衡量人工突触性能的一个重要准则, 是人工突触完成一次信号传递消耗的能量. 不同金属氧化物材料作为活性层的能量消耗对比如表1所示. 在这里将其定义为 E = Ipeak × V × td, 其中 Ipeak是EPSC的峰值, V是施加的脉冲幅值, td是所施加脉冲的持续时间[43]. 本文制备的器件在施加振幅为0.2 V的刺激时, 完成一次信号传递所消耗的能量为1.47 pJ, 如图4(d)所示. 所制备的MoO3活性层具有较大的比表面积, 能与其上涂覆的PEO-Li有更大的接触面, 在接受刺激时能够引起更多的载流子运动, 因此完成一次信号传递所消耗的能量较小, 而且能量的消耗随着受到刺激振幅的增加而增加.
序号 | 活性层 | 制备方法 | 能量消耗/pJ | 文献 |
1 | MoO3 | 水热法 | 1.47 | 本文 |
2 | MoO3 | 化学气相沉积 | 9.60 | [30] |
3 | HfOx/AlOx | 溶液法 | 6.00 | [44] |
4 | Pt/HfOx/TiOy/HfOx/ TiOy/TiN | 磁控溅射 | 0.30 | [45] |
5 | HfOx/CeOx | 等离子体辅助原子层沉积 (plasma-assisted atomic layer deposition) | ~8.00 | [46] |
6 | ZnSnO | 静电纺丝 | 0.44 | [47] |
7 | IZO/SiO2 | 射频磁控溅射 | 45.00 | [48] |
8 | IZO/GO | 滴涂 | 3.70 | [16] |
表1不同金属氧化物人工突触器件能量消耗
Table1.Energy consumption of different metal oxide artificial synaptic devices.