全文HTML
--> --> -->拓扑理论在有序系统中的应用可以追溯到1979年Mermin[11]的一项重要工作. 他把序参量连续变化的有序体系中序参量不连续的区域(如点、线或面)归结为拓扑缺陷. 如畴壁就是一种常见的拓扑缺陷, 而一些更复杂的奇异畴结构(例如涡旋、反涡漩态、斯格明子等)可以归为准一维拓扑缺陷. 这些拓扑缺陷和畴组成了不同的拓扑结构. 不同的拓扑畴不仅很大程度决定了材料的动力学特征和其他物理性能, 其本身还可以表现出各种新奇的物理现象和功能特性, 为开发新型纳米器件打开了一扇大门. 也因此2016年诺贝尔物理学奖授予了Thouless, Haldane和Kosterlitz三位物理学家, 以表彰他们在凝聚物质中的拓扑相变和拓扑相研究的重要贡献.
过去20余年, 二维拓扑缺陷(畴壁)被广泛研究, 并在其中发现了丰富多彩的新颖物性[12-17]. 然而, 对更为复杂的奇异极化拓扑态的研究仍处于起步阶段. 早在2004年, 就已有理论预测了超小铁电体系中的涡旋态[18], 但是由于缺乏有效的探测技术, 过去多年在实验上的研究依旧扑朔迷离, 难以验证其存在. 近年来, 得益于高分辨压电响应力显微镜(piezoresponse force microscopy, PFM)和球差校正的高分辨透射电子显微镜(high-resolution transmission electron microscopy, HRTEM)的发展, 越来越多的铁电拓扑畴结构被揭示出来, 包括近几年观察到的与磁性拓扑类似的通量闭合畴[19]、极化涡旋阵列[20]、中心型畴[21-24]、斯格明子[25]、麦韧[26]. 图1总结了目前实验上观测到的几种铁电拓扑态, 其中大多数是在尺寸受限的铁电/多铁纳米结构中被观测到的, 例如超薄薄膜[27,28]、多层膜/超晶格[19,20,25]、纳米岛[24,28,29]以及单晶纳米片[30-32]. 在某些情况下, 铁电拓扑结构也可能以亚稳态的形式出现在块体材料或薄膜中, 例如在铁酸铋(BFO)薄膜上通过施加电场所诱导产生的铁电涡旋态[33], 在非常规铁电单晶(如稀土锰酸盐)中出现的多重对称的涡旋结构[34-36], 以及最近在有机杂化结构中观测到的类似涡旋-反涡旋复杂畴结构[37]. 这些新型拓扑态的出现, 为探索极化拓扑结构所蕴含的奇异物理现象及其潜在的新性能和新器件用途创造了有利条件. 例如, 最近发现含有涡旋畴阵列的PbTiO3/SrTiO3 (PTO/STO)超晶格中呈现出宏观负微分电容特征, 为降低场效应晶体管的能耗提供了一种新途径[38]. 此外, 在中心型的拓扑畴中, 也发现了高导电畴壁, 并实现了外场驱动导电态的变化, 为构筑基于极化拓扑畴的存储器件迈出重要一步[21]. 近年来, 这一领域发展迅猛, 呈现出一系列激动人心的发现, 也因此成为铁电物理学的热点.
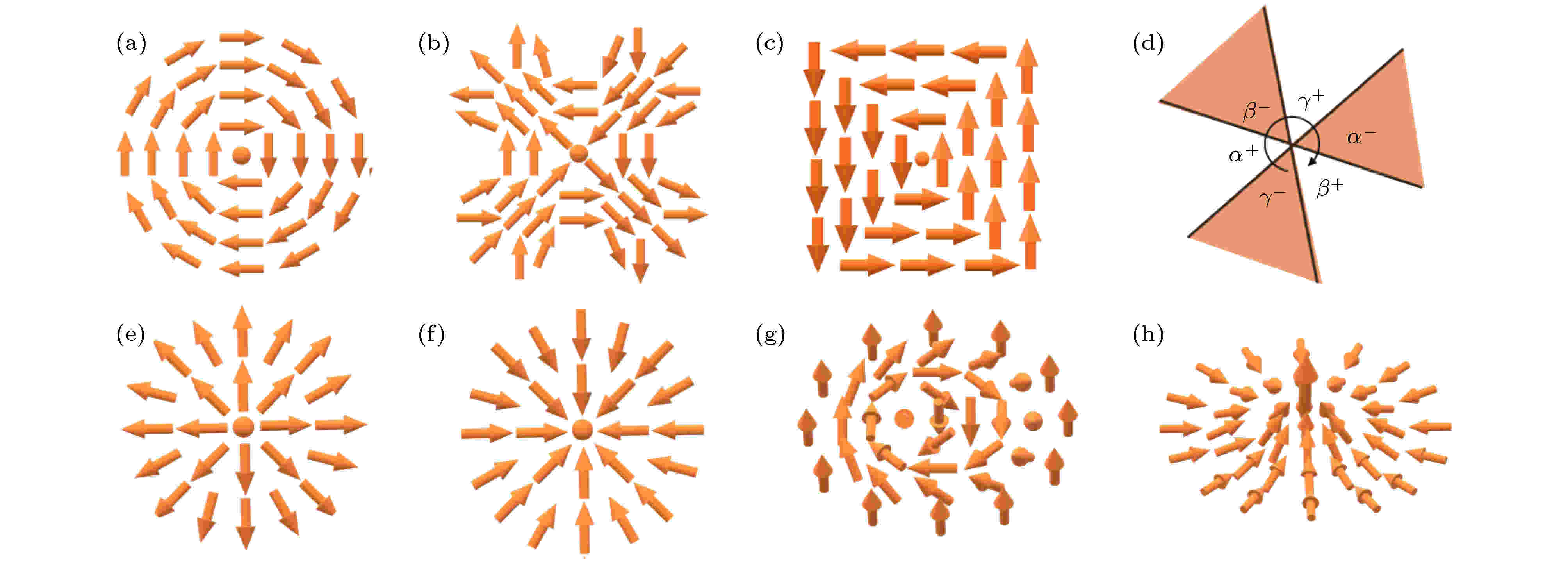
Figure1. Polar topological states in ferroelectric materials: (a) Vortex; (b) anti-vortex; (c) flux-closure; (d) six-fold vortex; (e) center-divergent; (f) convergent states; (g) skyrmion; (h) Meron.
本文将回顾这一新兴领域在近年的进展, 着重介绍在尺寸受限的低维纳米铁电材料中发现的多种奇异拓扑畴态, 并将讨论该领域亟待回答的问题和未来探索的新方向. 对于块体中的拓扑畴[34-36]以及铁电畴壁[13-15]前人已有总结[39,40], 这里就不再赘述.
2
2.1.极化通量闭合畴(flux-closure)与涡旋畴(vortex domain)
超薄膜/超晶格体系中通量闭合畴和涡旋畴. 2004年, Naumov等[42]和Kornev等[18]通过第一性原理模拟, 预测了在小尺度受限体系中存在极化弯曲, 以及通量闭合的涡旋态. 在几纳米尺度(与本征畴壁宽度相当)的铁电纳米岛或薄膜中, 退极化作用较为显著, 可在界面/表面或者畴壁处发生极化弯曲, 为形成复杂性的奇异拓扑畴创造了有利条件. 更有趣的是, 在铁电纳米点中可能存在小至3.2 nm的双稳态涡旋态, 且相邻近涡漩态之间相互干扰很弱, 有望用于开发存储密度超过60 Tbit/in2的超高密度存储器[42]. 这一预测为寻找铁电拓扑畴指出了小尺度体系的方向, 也激起了实验和理论上探求的热情. 然而因拓扑畴稳定的尺度太小, 实验的证据依然长时间萍踪难觅.直到2011年, Jia等[28]用实验观测证实了铁电极化卷曲的存在, 通过原子尺度分辨率的负球差像差校正HRTEM观察到了Pb(Zr,Ti)O3 (PZT)薄膜在畴壁与衬底之间的接合处存在极化卷曲. 同年, Nelson等[27]也在BFO薄膜的畴壁附近发现了一种类似三角状通量闭合的畴结构. 这说明, 在小尺度范围内, 退极化作用有可能导致极化弯曲, 使得形成通量闭合畴成为可能.
2015年, Tang等[19]在GdScO3 (GSO)衬底上沉积的PTO/STO多层膜中取得突破性进展, 发现有规则的方形通量封闭畴阵列(见图2(a)). 通过设计STO/PTO/STO 三明治结构, 有效地降低了空气电荷对退极化的屏蔽, 终于在尺寸受限 (15—40 nm厚)的PTO薄膜中获得了类似磁性材料中的方形通量闭合畴. 值得一提的是, 在尺寸较大的体系中, 极化更容易沿着晶体对称性许可的方向, 并通过铁弹型畴壁产生90°弯曲, 从而形成方形通量闭合畴, 而不是能量更高的连续卷曲型涡旋畴. 不久, Yadav等[20]在DyScO3 (DSO)衬底上制备了具有更薄PTO层 (~4 nm) 的(PTO)n/(STO)n超晶格, 并最终获得了稳定的涡旋排列畴结构(见图2(b)). 这个结果很好地验证了Naumov等[42]的预测, 也说明了超小尺寸约束对形成和稳定极化涡旋畴起到至关重要的作用. 此外, Alexe小组[43]也在Co/PTO/(La,Sr)MnO3/STO铁电隧道结中只有几个纳米厚的PTO层中观察到超小尺度极化弯曲和通量闭合畴(直径约2 nm). 这表明在尺寸足够小的体系中(例如 < 10个晶胞), 即使退极化场被电极部分屏蔽, 仍旧可以产生极化卷曲与通量闭合畴.
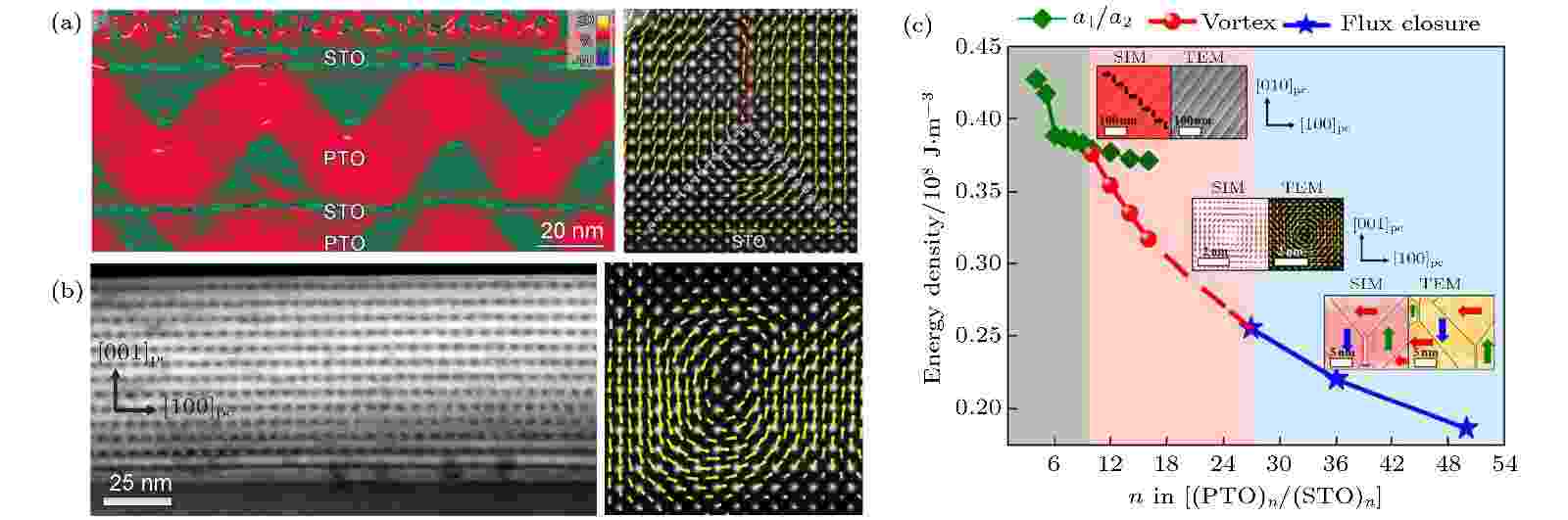
Figure2. Vortex domain states in (SrTiO3)n/(PbTiO3)n multilayers and superlattices: (a) The left panel presents the geometric phase analysis (GPA) image, the right panel is a local polarization distribution map for a single closure domain[19]; (b) the left panel is a cross-section dark-field TEM image of (SrTiO3)n/(PbTiO3)n superlattices, and the right panel is the local magnification of polarization distribution of a single vortex structure[20]; (c) a calculated phase diagram for (SrTiO3)n/(PbTiO3)n illustrating the length scales within which different topological states can be stabilized[44].
为了更清楚地了解这些拓扑态的形成和稳定机制, Hong等对DSO衬底上的(PTO)n/(STO)n超晶格进行了系统的相场模拟, 并与实验观察相比较[44]. 结果表明, 在体重中静电能、弹性能、极化梯度能之间存在微妙的竞争关系, 而这种竞争关系对尺度相当敏感, 从而导致在不同铁电层尺度范围产生不同拓扑畴态结构(见图2(c)). 在PTO厚度为3 nm以下时, 因退极化起主导作用, 极化沿着面内躺倒. 随着厚度增大, 逐渐形成涡旋阵列, 然后转变为方形通量闭合畴, 在厚度很大时变成普通条带畴. 更有趣的是, 基于一个简化Landau-Ginzburg理论的准二维解析模型可很好地预测涡旋畴形成的临界尺寸, 其上限厚度大致比铁电体的本征畴壁宽度大一个数量级(实际上是约8倍). 由于普通铁电体中畴壁的宽度约为几个晶胞, 因此涡旋态的上限尺度应为10 nm左右. 而预测的下限尺寸与本征畴壁宽度处于相同量级(约1.4倍). 对于PTO薄膜, 该临界厚度约为4.0 nm, 低于这个下限时, 过强的退极化场将破坏涡旋畴结构, 并将其转变为面内畴结构. 这一临界厚度主要是由退极化能和畴壁能之间相互竞争所决定的. 这一结果为尺寸受限体系中涡旋类拓扑结构的产生和稳定提供了一个较为普适的尺度规律. 这也为在铁性体系中寻找新拓扑结构, 以及调控拓扑畴提供了一种指导原则.
纳米岛中的通量闭合畴. 另一方面, 纳米点/岛上的极化拓扑畴结构也受到关注. 早期的研究发现了在这类纳米结构中存在通量闭合畴的证据. 例如, 在多孔氧化铝(AAO)模板辅助脉冲激光沉积(PLD)方法制备的高密度PZT纳米岛阵列中观察到类似涡旋的复杂畴结构[37]. 而Gregg小组[31,32]用聚焦离子刻蚀法在BaTiO3 (BTO)和PZT单晶中切出来的单独纳米片中也发现了超细条带畴构成的通量闭合结构. 田国等[45]观察到在BFO纳米岛中存在涡旋和反涡旋对. 然而, 与超薄薄膜或超晶格中含有的规则排列的旋涡/闭合畴不同, 纳米点中只能观察到零星的通量闭合畴且重复性低, 这说明纳米岛中很难自发形成拓扑畴结构, 与理论预测不相符合. 这种不一致性可能是由于退极化场只被部分屏蔽, 故不足以克服涡旋畴形成所带来的应变能增量. 另一方面, 这些纳米点的尺度通常较大(60 nm至微米尺度), 尚未小到涡旋畴形成的尺度. 因此, 在铁电纳米点/岛中制造稳定且可重复的涡旋态仍然是一个挑战.
2
2.2.中心型拓扑畴
与涡旋畴相呼应的是另一种引人注目的中心型拓扑畴, 其极化矢量水平投影都指向中心或从中心向外发散, 形成中心汇聚或中心发散的畴结构[21,24]. 相较于涡旋畴难以在纳米岛上稳定形成, 中心型拓扑畴可以稳定形成且重复性较高. 2017年, Li等[24]报道称, 在高密度BFO纳米岛(直径约60 nm)阵列中观察到自发形成的中心型拓扑畴, 如图3(a)所示. 利用矢量PFM成像进行三维畴结构分析并重构的方法, 在纳米岛阵列中发现中心汇聚型、中心发散型和双中心型等多种中心型拓扑畴. 更令人兴奋的是, 通过对纳米岛施加外电场, 这些拓扑畴可以在中心汇聚和中心发散的两态间可逆切换, 且写入的拓扑畴能够长时间保持稳定. 这使得利用中心型拓扑畴的两种不同构型进行数据的存储和擦写成为可能, 因此引起广泛关注.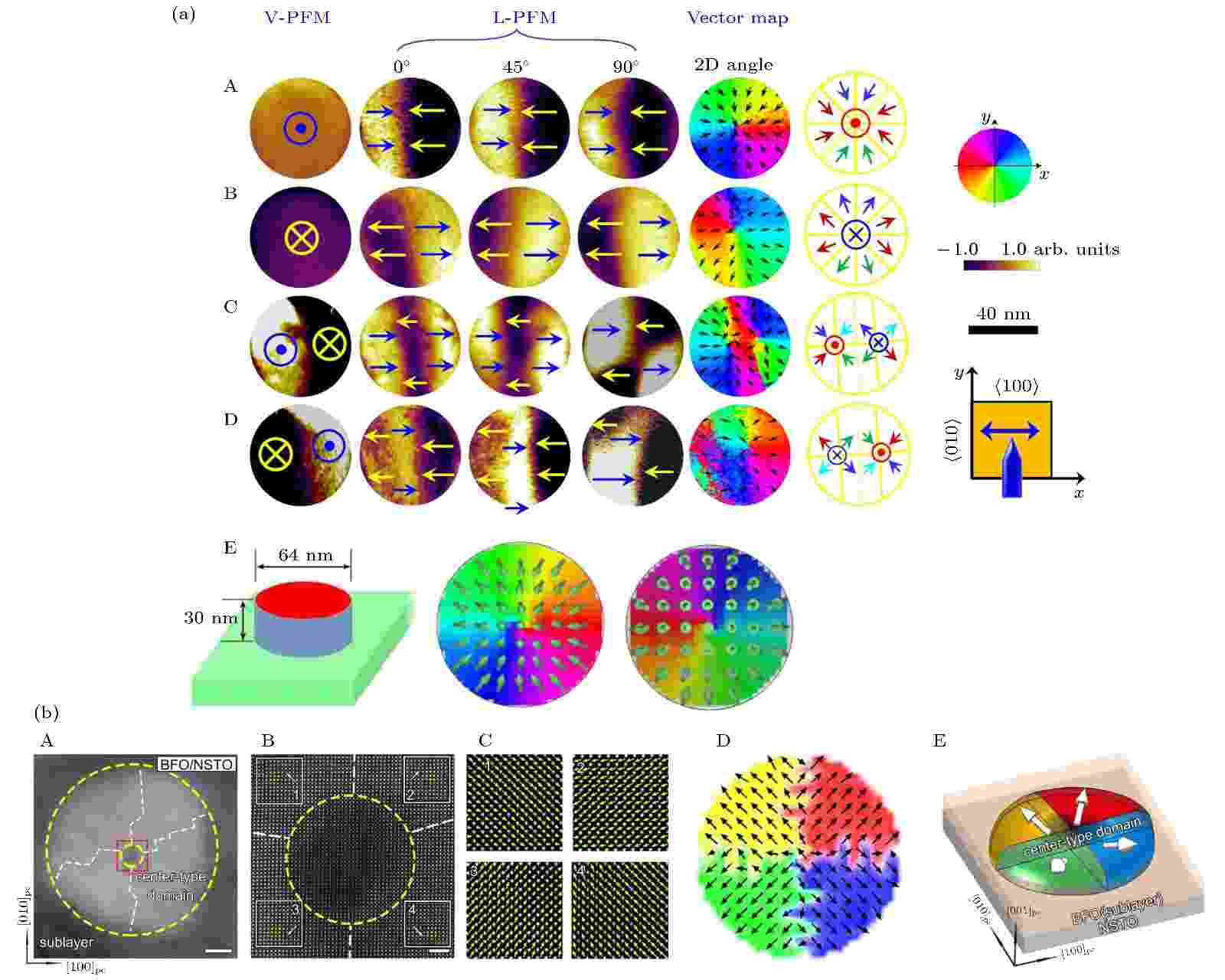
Figure3. Topological center-domain structures in BiFeO3 nanoislands: (a) Four types of center-domain states in BiFeO3 nanodots on SrTiO3 substrate observed by vector PFM analysis[24], where panel A illustrates the center-convergent, panel B illustrates the center-divergent, panel C illustrates the double-center domain with convergent, panel D illustrates the divergent center states, and panel E illustrates the cylinder model for phase-field simulation and two type of polar vector contour maps derived from the simulation; (b) topological center-domain states in BiFeO3 nanodots on Nb-SrTiO3 substrate[23], where panel A and B illustrate the plan-view TEM image for a single nanodot and atomically resolved HAADF-STEM images corresponding to the red square area in panel A, panel C is local magnified view of polarization distribution calculated by TEM corresponding to the white square area in panel B, panel D is the polarization vector distributions of the nanoisland, panel E is the schematic of domain configuration in these BFO nanoislands based on the analysis of both PFM and TEM characterization.
对于这类独特的中心型拓扑畴来说, 极化汇聚(发散)于中心, 通常带来巨大的静电能, 从而难以稳定. 研究人员通过仔细分析实验数据并结合相场模拟, 揭示了静电场可以通过电荷富集得以屏蔽, 从而使得这种拓扑态达到能量稳定态的机制, 并通过模拟重现了实验观察到的不同中心畴构型[24]. 这类带电的拓扑畴的形成机制与带电畴壁有些相似, 通过材料内部的电子、空穴、离子缺陷的富集驱动形成. 这一论断被Han等[23]在自组装的BFO纳米岛中用高分辨扫描透射电子显微镜(high-resolution scanning transmission electron microscope, HRSTEM)观察到类似的中心畴所支持, 见图3(b). 从HRSTEM图像看出, 纳米岛表面存在Bi+空位从而导致电荷富集, 由于纳米岛具有坡度, 富集电荷产生的静电场可以驱动形成中心汇聚型拓扑畴. 值得一提的是, 通过HRSTEM还观察到BFO纳米岛中心存在缺陷区域(中心暗衬度区), 也为该区域存在显著的电荷富集创造了条件. 2018年, 潘晓晴小组[46]在含Bi+缺陷中心的BFO薄膜中, 观测到围绕缺陷区域的刺猬状发散型(hedgehog)的畴结构, 也为缺陷电荷驱动形成带电极化拓扑中心的机制提供了一个有力佐证. 而另一方面, Kim等[22]在嵌入于CoFe2O4 (CFO)基质的自组装方形BFO纳米岛(厚度约300 nm)中也观察到了类似的中心畴结构, 并伴有薄缓冲畴区, 却认为是应变梯度驱动形成的机制.
尽管这类中心畴形成和稳定的机制仍未达成共识, 但是这些观测表明, 除了之前认为应变、退极化、尺度等影响因素外, 电荷、点缺陷和应变梯度也可能对拓扑畴结构带来显著影响. 另外, 由于纳米点/岛结构更便于单独寻址, 以及外场调控单个拓扑畴, 可以作为纳米器件的单元. 在后续研究中也发现了与中心畴紧密相关的十字型高导电畴壁[21], 验证了这类拓扑畴具备一定功能性, 为利用开发电流读出的拓扑畴存储器件迈出重要一步.
2
2.3.斯格明子(skyrmion)、麦韧(Meron)及其他拓扑态
随着对极化拓扑畴研究的深入, 一些新颖的拓扑态也在近两年逐渐被揭示出来. Das等[25]在以STO为衬底的(PTO)n/(STO)n (n~16)超晶格中(STO衬底具有较大压缩应变)发现类似于磁性斯格明子的泡状极化斯格明子(见图4(a)). 尽管铁电超晶格体系中不具备类似磁性斯格明子形成所需要的Dzyaloshinskii-Moriya (DM)交换作用, 但仍可观察到极化斯格明子的长程手性序, 其形成机理还未能很好解释. 不过, 这类极化斯格明子的形成可能与自发形成的柱状畴(环形闭合畴壁)有关. 因为超晶格中局域电场和应力场很强, 一方面可诱导畴壁弯曲形成面内极化, 同时还使得畴壁周边的极化结构发生旋转, 最终形成了极化斯格明子态. 这种独特的极化斯格明子态的发现, 使人们更为确信在铁电体系中也可找到与磁性体系类似的拓扑畴结构.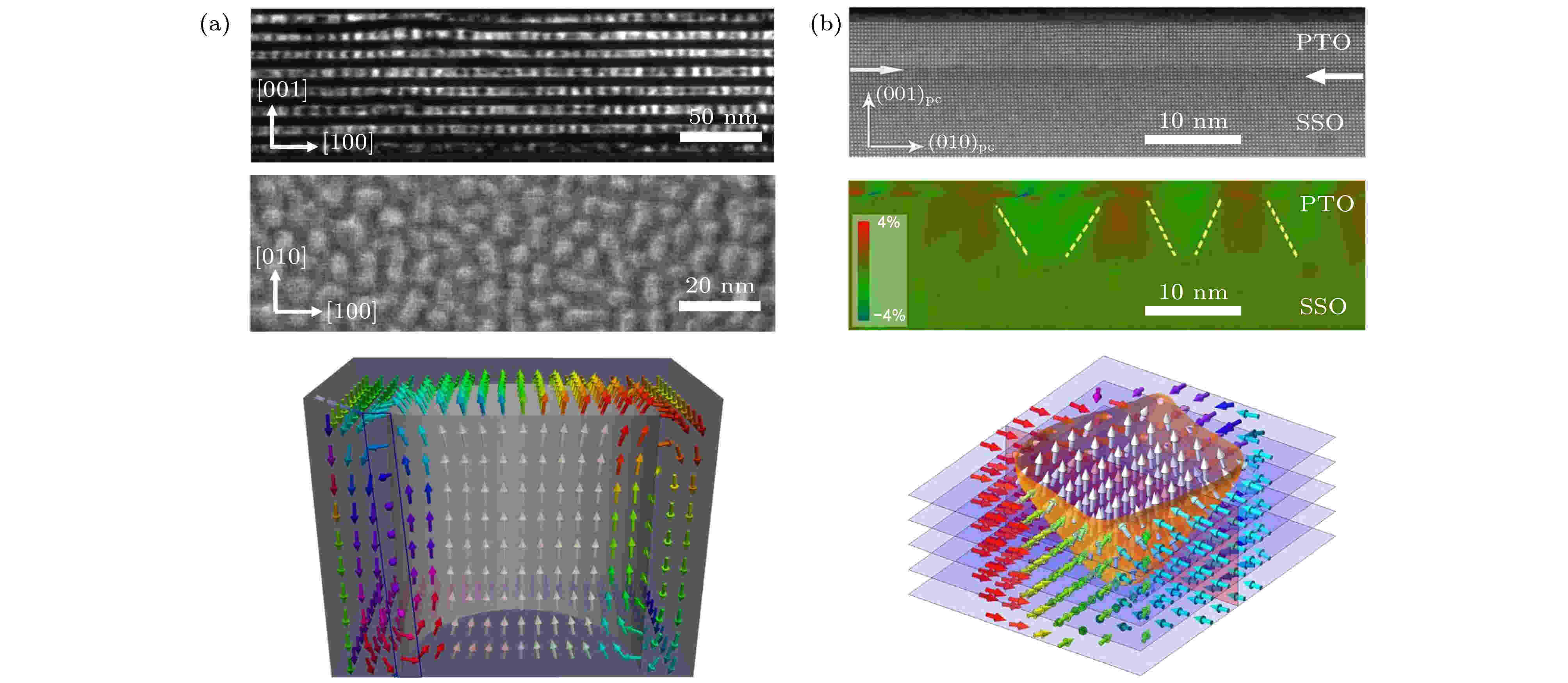
Figure4. The polar skymion bubble and polar meron states: (a) Polar skyrmion bubbles in a (SrTiO3)n/(PbTiO3)n superlattice on SrTiO3 substrate[25], where the upper panel is a cross-section dark-field TEM image, the middle panel is a planar-view dark-field STEM image, and the bottom panel is chematic skyrmion bubble configuration from calculations; (b) polar merons in a untrathin PbTiO3 film on SmScO3 substrate[26], where the upper panel is the cross-section HAADF-STEM image, the middle panel is the corresponding geometric phase analysis (GPA) image, and the bottom panel is a meron configuration from calculations.
最近, Wang等[26]在SmScO3 (SSO)衬底上生长的超薄PTO (5 nm)薄膜中发现了面内汇聚型和面内发散型麦韧和反麦韧结构(anti-Meron). 其中麦韧是拓扑数为1/2的非平面型拓扑畴结构, 其拓扑中心极化指向面外而周边极化指向面内(见图4(b)). 通过对高角环形暗场的扫描透射电子显微镜图像(HAADF-STEM)中离子位移的定量分析, 发现麦韧和反麦韧态可按规律排列形成麦韧晶格, 而相场模拟则表明麦韧形成晶格比随机分布更利于降低体系的总弹性能. 该工作进一步表明了通过外延应力和纳米尺度相结合是形成和调控铁电拓扑畴的一种有效性方法, 并揭示了极化体系在一定条件下可产生类似特殊凝聚体系中的准粒子. 这对开发基于铁电拓扑畴的高密度非易失性信息存储器件具有重要意义.
除外, Zhang等[47,48]在PbZr0.2Ti0.8O3/SrTiO3/PbZr0.2Ti0.8O3多层薄膜体系中也发现了一种约10 nm尺度的球形泡状畴, 其极化方向与周边薄膜的极化相反. 在这种泡状畴中还观察到一些非公度相导致的对称破缺, 并形成类似Bloch-Neel混合型的畴壁.
值得一提的是, 最近东南大学的Zhang等[37]在有机-无机杂化钙钛矿铁电体(4, 4-DFPD)2PbI4(其中4, 4-DFPD 为 4, 4-difluoropiperidinium)二维材料中观测到一种特殊的复合拓扑结构. 这种拓扑畴由交替的头对头和尾对尾的极化排列组成, 可以看作由两套手性相反的通量闭合涡旋畴叠加而成, 类似涡旋-反涡旋结构. 而且, 这类特殊拓扑结构还可形成拓扑网络, 有点类似稀土锰氧化物非常规铁电体中的拓扑畴网络[36]. 这为寻找新型拓扑畴提供了一种新材料体系.
铁电体系中的这些拓扑态与磁性体系相比, 具有更小的尺寸. 因此, 对铁电拓扑态的探索不仅有助于设计更高密度、更低能耗、更快响应速度的存储器件, 并且有望挖掘出丰富的新颖物性. 这类极化拓扑畴可以看作新型基元-序构, 为调控材料性能提供了一个新途径, 有望获得高性能的材料. 例如, 涡旋畴处增强的负电容效应, 可有助于降低铁电晶体管的功耗[38]. 但与此同时, 铁电拓扑畴的研究和实际应用还面临着一些问题和挑战, 包括如何切换、移动、产生和擦除这些拓扑态; 如何探测与表征这些拓扑畴的新物性, 以及如何将这些小尺度拓扑畴应用于存储器件.
为了实现一些可编程器件的功能, 如信息存储和处理器件, 还需要通过外场实施动力学调控(如拓扑态的产生、湮灭与切换等). 如Kalinin课题组的Balke等[33,49]和Vasudevan等[50]使用压电响应力显微镜扫描探针, 在具有铁弹性条带畴的菱方相BFO薄膜中, 通过导电针尖施加电场写入从而形成通量闭合畴、涡旋畴以及中心型拓扑畴, 见图5(a)—(c). 而Li等[51]也在BFO薄膜中, 通过针尖固定在模板上施加点电场, 可有效写入涡旋-反涡漩畴的网络. 其主要机制是在极化翻转过程中, 通过针尖施加电场的面外和面内分量同时起作用, 并与铁弹畴的应变能相互竞争, 从而诱导形成亚稳态的拓扑畴. 此外, McQuaid等 [31]在微米尺度的BTO单晶片中施加面内电场可诱导出复杂性通量闭合畴. 这表明, 通过外加电场可以诱导产生亚稳态的拓扑畴, 并可在电场撤除后保持稳定.
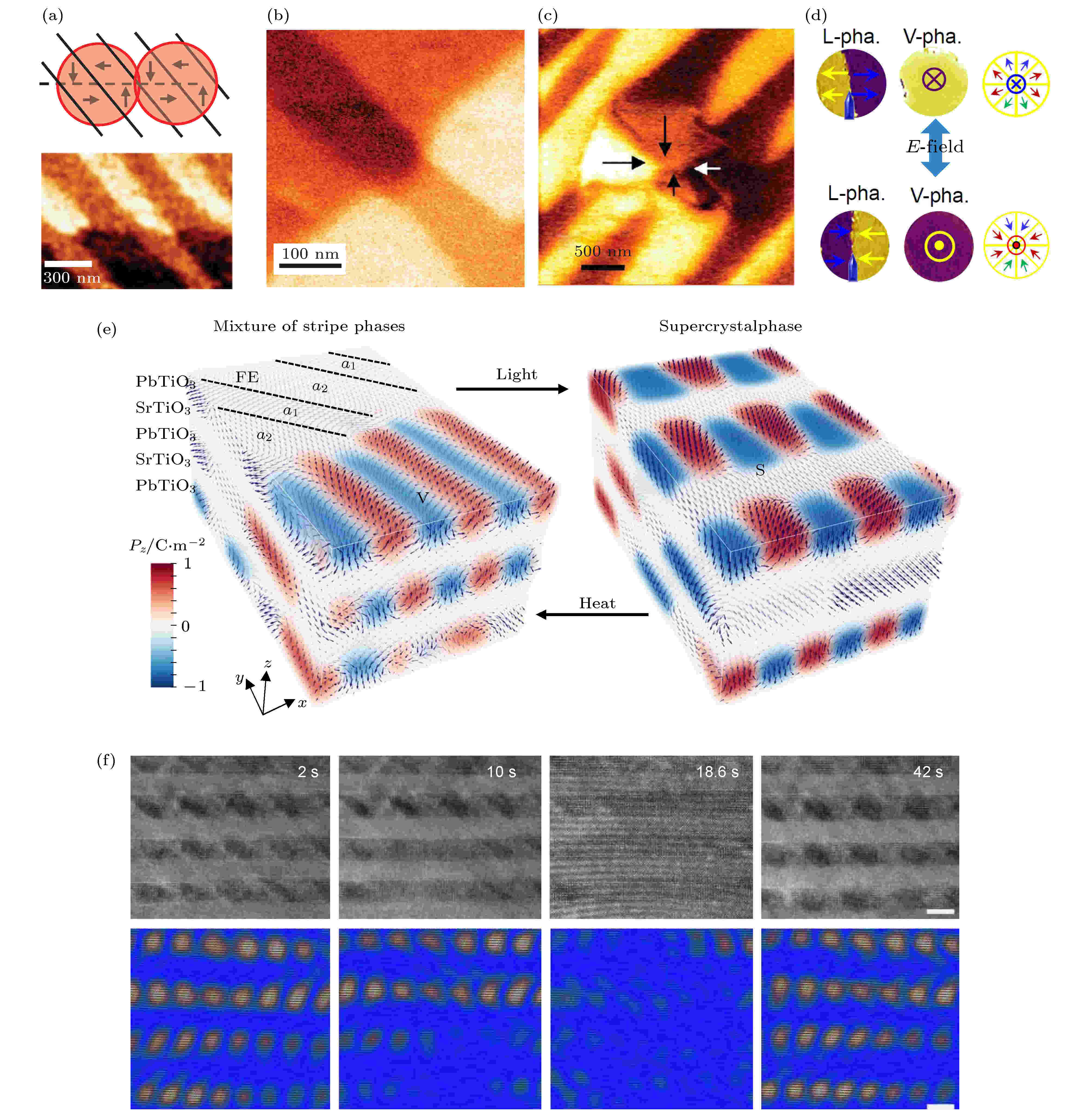
Figure5. External field control of polar topological domains: (a) Creation of closure in-plane domains in BFO film by using scan bias. Domain configuration for several closure domain states arranged along a line (upper panel), in-plane PFM image after switching several closure domains (bottom panel)[33]; (b) in-plane PFM image of a vortex domain structure which was created by using scan bias[49]; (c) in-plane PFM image of a created center-type domain structure[50]; (d) PFM images showing the electric switching of BiFeO3 center domains by scanning electric bias[24]; (e) phase transition from a mixed phase of in-plane a1/a2 domains and polar vortex to a single 3D supercrystal phase triggered by sub-picosecond optical pulses in a (SrTiO3)n/(PbTiO3)n superlattice[54]; (f) Mechanical manipulation of vortices in a (SrTiO3)n/(PbTiO3)n superlattice. A chronological high resolution TEM image series acquired under mechanical loads (upper panel) and the corresponding inverse fast Fourier transform maps (bottom panel)[55].
除外, Ramesh 组[52]利用针尖电场, 把PTO/ STO超晶格中涡旋畴阵列写成面内a1a2畴, 并且可施加反向电压恢复回来. Kim等[53]在BFO/CoFe2O4复合薄膜中的自组构形成的方形BFO纳米岛中, 通过对纳米岛局部施加电场, 实现不同涡旋态的调控(改变其拓扑数). 近年来, Li等[24]也在直径约60 nm的BFO纳米岛阵列中, 实现了单个中心型拓扑的稳定调控, 即在中心-发散与中心-汇聚型两态之间往复切换, 见图5(d). 并且, 切换后的畴可以稳定保持6个月以上. 说明了可以利用这两种拓扑态存储数据“0”和“1”, 并可实现往复擦写的功能. 然而, 对于其他拓扑畴(如涡旋、斯格明子等), 单个稳定调控还很难实现, 因此还需大力探索调控这类拓扑畴的方法.
除了利用电场以外, 用光场、应变场和加热等对畴结构进行多场调控也是一个非常有趣的方向. 例如Stoica 等[54]在PTO/STO超晶格形成的涡旋畴阵列与面内畴的混合畴结构中, 利用光照导致拓扑相变, 产生了一种有序的拓扑态阵列, 见图5(e). 而通过加热可以恢复到初始的拓扑构型, 说明了利用光调控拓扑畴的可行性. 应力调控拓扑畴也有报道, 例如通过应力可实现PTO/STO体系中涡旋阵列的湮灭, 诱导形成面内畴[55], 见图5(f). 通过这种交叉耦合场调控, 为拓扑畴调控提供了一种途径, 并为构筑新概念器件打下基础, 如光(或应力)写-电读的拓扑电子学器件[56].
为了实现超高密度的拓扑态存储器件, 还可利用涡旋畴的两种不同手性来存储数据. 然而, 通常均匀的电场是无法打破这种卷绕对称性的, 因此如何操控单个纳米点的极化环矩, 是其中面临的巨大挑战. 目前, 理论上有几种调控方法, 如Proandeev等[57]提出通过在单个PZT纳米点外安置点电荷, 从而产生横向非均匀电场. 基于第一性原理的仿真表明, 通过非均匀电场可反转涡旋畴的手性. 而这种非均匀电场在实验上可通过压电响应力显微镜的针尖施加. 此外, Lich等[58]利用基于Landau-Ginzburg理论的相模拟, 展示了通过控制纳米点的形状(人为在矩形纳米点中引入缺口或凸起), 可打破纳米点内静电场的对称性(如退极化场在缺口处聚集, 而在凸起处发散), 从而使得均匀电场也可确定性地对涡旋旋畴的手性进行调控.
目前, 大量性能探索主要聚焦在铁电畴壁上, 而随着更为复杂的奇异拓扑畴的发现, 引发了人们对奇异拓扑缺陷中的新颖物理现象和新性能的探索热情. 尤其是对一维拓扑缺陷如涡旋畴中心, 因其与周围薄膜显著不同的极化和应变状态, 导致电子结构和性能产生显著变化[33]. 一方面可导致材料整体性能的变化, 成为调控材料性能的一种新手段. 另一方面, 具备不同性能的拓扑缺陷, 其本身即可作为纳米功能单元, 用于构筑拓扑电子学器件.
其中一个例子是在富含涡旋畴阵列的PTO/ STO超晶格中, 其涡旋畴可导致材料的电荷分布发生变化, 产生了有趣的负电容行为[36]. 这种负微分电容特性可用于提高晶体管的亚阈值摆幅, 降低驱动电压, 有望使现代计算机和其他电子器件更为节能. 这些现象也说明低维拓扑缺陷阵列可作为一种新型基元序构, 用于调控材料宏观性能, 为开发高性能材料提供了一个新途径.
另一个例子是, 在自组装方形BFO纳米岛[21]中发现了中心型拓扑畴具有高电导畴壁. 如图6(a)所示, 而通过导电原子力显微镜(conducting atomic force microscope, CAFM)观察到每个纳米岛内部都有明显的十字交叉状高导电的畴壁通道. 其中中心发散型含有的尾-尾电荷畴壁比中心汇聚型头-头电荷畴壁显示出更高电导性. 在该工作中, BFO主要为p型. 而发散型的中心畴中尾-尾畴壁倾向聚集BFO中的空穴, 从而畴壁电导显著增强. 相反, 头-头畴壁倾向于聚集点缺陷(例如Bi空位), 因此电导较低. 有意思的是, 中心发散和中心收敛两种拓扑态可以往复切换, 导致其所对应的高低两种畴壁导电态也随之切换, 因此可通过畴壁导电态的高低, 读出两种拓扑态. 利用这个发现, 可构筑基于中心型拓扑畴的原型存储器件, 利用对畴壁电导高低的分辨, 实现非破坏性地读出“0”和“1”两种态. 这种器件具有103以上的开/关电阻比, 并在经历100次开关循环后还能保持稳定, 显示出良好的存储性能.
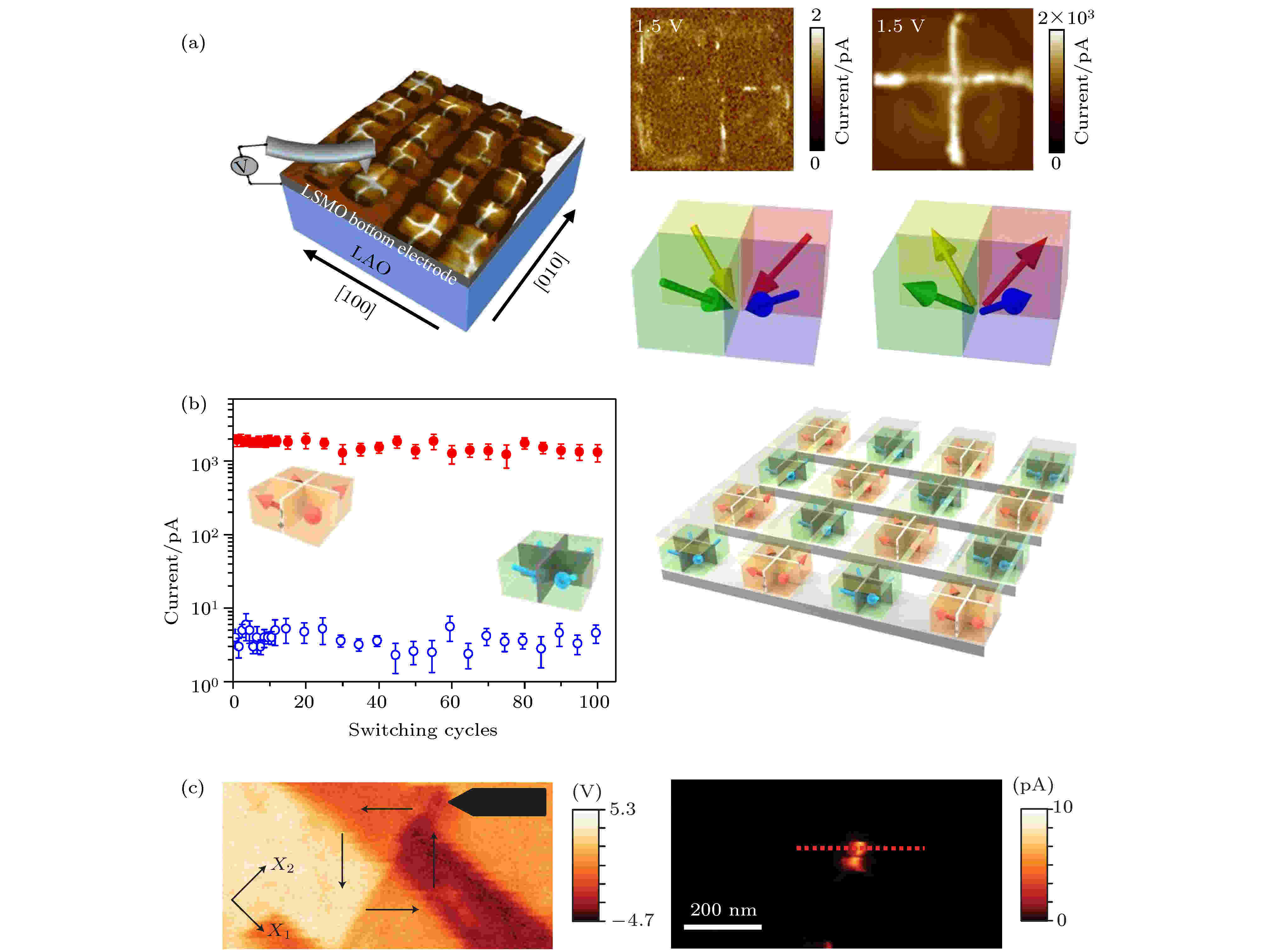
Figure6. Enhanced electric conductivity at domain walls and vortex cores: (a) CAFM images of quadrant center domains in an array of square-shape BiFeO3 nanoislands on LaAlO3 substrate (left panel), different conductive domain wall states for respective convergent (right front panel) and divergent domains (right rear panel) [21]; (b) endurance performance of resistive switching in domain wall conduction (left panel), and a crossbar device architecture of conceptual memory prototype based on domain wall current (right panel)[21]; (c) the PFM image of vortex domains (left panel) and corresponding CAFM image of vortex core (right panel), shows enhanced electric conductivity[33].
这些结果也为通过操控拓扑态来调控其导电性提供了一种思路. 如在BFO纳米岛中写出不同拓扑态, 可产生不同图案的畴壁导电态[62]. 这表明单个拓扑畴具备一定功能性, 可作为某种可编程纳米器件的单元. 需要指出的是, 在这些中心型拓扑畴中观察到的导电态主要还是由导电畴壁产生, 在均匀性、重复性以及器件可微缩性方面还有待提高.
随着奇异拓扑畴的发现, 引起了探索这类拓扑态带来的新颖物性的兴趣. 尤其是一维拓扑缺陷(如涡旋畴、斯格明子等), 可看作准一维功能单元, 有望克服畴壁器件的弱点, 从而构筑更稳定和更高集成度的器件. 早在2012年, Balke等[33]就报道了涡旋畴涡心的导电增强现象, 如图6(c)所示. 主要归因于涡心受外场诱导形成扭曲的亚稳态, 具有带电畴壁片段, 从而导致导电性增强. 这种涡心导电性可认为是超细准一维导电通道, 并可受外场操控, 为开发高密度集成器件提供了一种可能. 然而, 在这种涡心中观察到的导电电流相当小, 大约在pA量级, 且这种亚稳态的导电增强特征的重复性和稳定性较低, 不太适用于制造纳米级电子器件. 因此, 该工作多年来一直没有后续跟进研究. 值得一提的是, 本课题组最近在BFO纳米岛的涡旋畴和中心型拓扑畴中心都发现了显著增强的导电性, 在2 V电压下读到高达3 nA的电流[63]. 更有意思的是, 这种超细准一维导电拓扑缺陷可以被电场可控擦写, 展示出在存储器件应用的优秀潜质.
诚然, 目前对一维拓扑缺陷所呈现出的新颖现象和新功能的研究还处于起步阶段, 可预见在这一肥沃领域进行探索必将带来更多的新发现和新应用机会. 例如新奇拓扑态在磁性、磁电阻、磁电耦合、光电、忆阻和光伏效应等方面的新性能, 可类比于畴壁中的丰富功能. 这些潜在的新功能可用于开发未来可调谐的高性能拓扑电子学器件, 如拓扑存储器、磁场/应变传感器、节能晶体管、致动器、光电子器件等.
近年来, 诸多新颖铁电拓扑畴的发现, 激励人们继续寻找更多新型拓扑畴以及承载拓扑畴的新材料体系(如最近有机无机体系中发现的自发形成的涡旋-反涡旋结构也值得关注[37]). 更重要的是, 拓扑畴蕴含着丰富多彩的新物理和新功能也有待进一步探索, 如磁电耦合、磁电阻、光伏、光电导、电卡效应、非线性光学性能等. 可以预见这是一个有待开发的富矿, 但目前这方面研究还甚少. 而通过多场交叉调控手段, 如利用电、光、磁、热等效应去调控拓扑畴, 有助于探测其交叉耦合性能. 此外, 为了实现拓扑畴器件的用途, 需研究拓扑畴的调控手段, 并理解其调控机制. 实现对拓扑态结构的精准调控, 如拓扑畴形成、产生、湮灭以及从一种态到另一种态切换. 通过对基于拓扑畴的基元序构进行设计, 获得性能优异的材料, 为开发高性能器件打下基础.
虽然目前只有少数的例子可展示这些拓扑态的潜在应用, 但现已揭示的有趣物性足以让我们描绘一些可能的器件用途. 如利用超小拓扑畴作为存储单元, 有望构筑超高密度存储器或逻辑器件, 并可实现低能耗、长寿命、高速读写的功能. 为此, 需要对拓扑畴进行精确操控, 实现其优秀的存储性能. 铁电斯格明子也有可能用于设计新型赛道存储器, 这需要实现外场驱动斯格明子的注入和移动. 而能否准确且有效地读出不同拓扑态也是一个重要课题(如通过电导、光学特性进行读出). 除外, 奇异拓扑态在其他方面的用途也很值得期待, 如基于负电容的低耗能晶体管、应变/磁/光传感器、磁电耦合器件以及基于拓扑畴共振的微波器件等. 总之, 这是一个快速发展的领域, 尚有诸多新结构、新功能、新器件用途、新问题亟待进一步探索. 还需要不同方向的研究人员共同努力, 为这一新兴的拓扑电子学领域添砖加瓦.