全文HTML
--> --> -->最近, 一系列利用飞秒激光脉冲诱导磁性材料产生太赫兹辐射的实验引起了广泛关注[13-15]. 在飞秒激光的激发下, 磁有序材料的超快退磁过程可产生太赫兹辐射[16-18]. 进一步, 2013年, Kamprath等[19]首次利用飞秒激光脉冲激发铁磁/非磁金属异质结Fe/Au, 应用逆自旋霍尔效应(inverse spin-Hall effect, ISHE)得到了太赫兹辐射, 开创了一种结合超快动力学与自旋电子学的新型自旋太赫兹发射器. 该发射方法具有制备简单、破坏阈值高、频带覆盖范围宽等优点. 然而, Au的自旋霍尔系数较小, 降低了ISHE中自旋-电荷转换效率, 限制了辐射太赫兹的强度, 其太赫兹发射强度只有非线性晶体ZnTe的1%. 近年来, 随着对异质结材料与结构的不断优化探索, 目前已能够在W/CoFeB/Pt的三层异质结中实现强度相当于ZnTe晶体的太赫兹发射[20]. 然而, 自旋太赫兹发射器在单一脉冲的激发下仅能实现线偏振太赫兹辐射, 虽然可以通过双抽运[21]或级联激光器[22]激发任意偏振的太赫兹波, 但是这些系统设计较为复杂, 难以集成与小型化, 亟待突破性的进展.
三维拓扑绝缘体材料, 如Bi2Se3, Bi2Te3, Sb2Te3及其合金(BixSb1–x)2Se3, (BixSb1–x)2Te3等最初仅作为热电材料而被广泛研究. 2008年, 斯坦福大学张首晟研究组[23]在理论层面预言了二维拓扑绝缘体的存在, 由于其特殊的拓扑非平庸特性, 拓扑绝缘体再次引起人们的关注并迅速成为凝聚态物理方向的热门研究领域. 拓扑绝缘体还可以进一步地推广到三维体系[24-26], 在三维拓扑绝缘体的体能带隙中存在无能隙的二维拓扑表面态. Fu和Kane[27]通过理论计算提出了甄别三维拓扑绝缘体的简便方法, 并且得益于角分辨光电子能谱(angular resolution photoelectron spectroscopy, ARPES)的发展, 在实验上也已经证实了可以通过观测某种材料的表面态在两个时间反演不变点之间穿越费米能级的次数是否为奇数次来确定其是否具有拓扑性[28]. 这种受时间反演对称保护的表面态赋予拓扑绝缘体很多特殊的性质, 如无质量的狄拉克费米子、自旋-动量锁定[29]和无耗散螺旋性边缘态[30]等. 通过在三维拓扑绝缘体中引入垂直的铁磁性, 会使上下表面态在狄拉克点打开能隙, 进而拥有不同的拓扑性质, 此时薄膜侧面作为两个不同拓扑相的边界, 会呈现手性的边缘态. 于是, 在三维拓扑绝缘体可以实现许多新奇的量子效应, 如量子反常霍尔效应[31]、马约拉纳费米子等[32]. 因此, 拓扑绝缘体被认为是实现未来超低功耗自旋电子器件和量子计算的重要材料[33]. 更重要的是, 由于拓扑绝缘体具有强自旋轨道耦合效应, 其自旋霍尔角超过已知重金属1个数量级以上[34,35], 可以实现高效的自旋电荷转换, 所以, 将拓扑绝缘体与铁磁性材料结合构成异质结构有望实现高效可控自旋太赫兹源; 除了自旋太赫兹辐射外, 异质结中的拓扑绝缘体存在非线性瞬态光电流引起的太赫兹辐射[36,37], 有望通过改变入射光偏振态等手段实现对两种太赫兹辐射的分别调制, 进而实现出射太赫兹波的任意调谐.
本综述从拓扑绝缘体的光电流分析入手, 首先介绍拓扑绝缘体中光电流引入的非线性太赫兹发射机理; 接下来, 通过分析铁磁/非磁异质结表面的自旋注入和自旋-电荷转换机制等超快磁学现象, 深入讨论了自旋太赫兹发射的理论机制; 进一步, 通过介绍拓扑绝缘体/铁磁异质结中太赫兹发射的研究进展, 讨论了拓扑自旋太赫兹的优势和未来的发展方向.
2.1.拓扑绝缘体的基本结构
拓扑绝缘体是一种V-VI族半导体, 常见的辉碲铋矿单晶拓扑绝缘体(Bi2Se3族, 例如Bi2Te3, Sb2Te3)呈六方晶系, 空间点群为
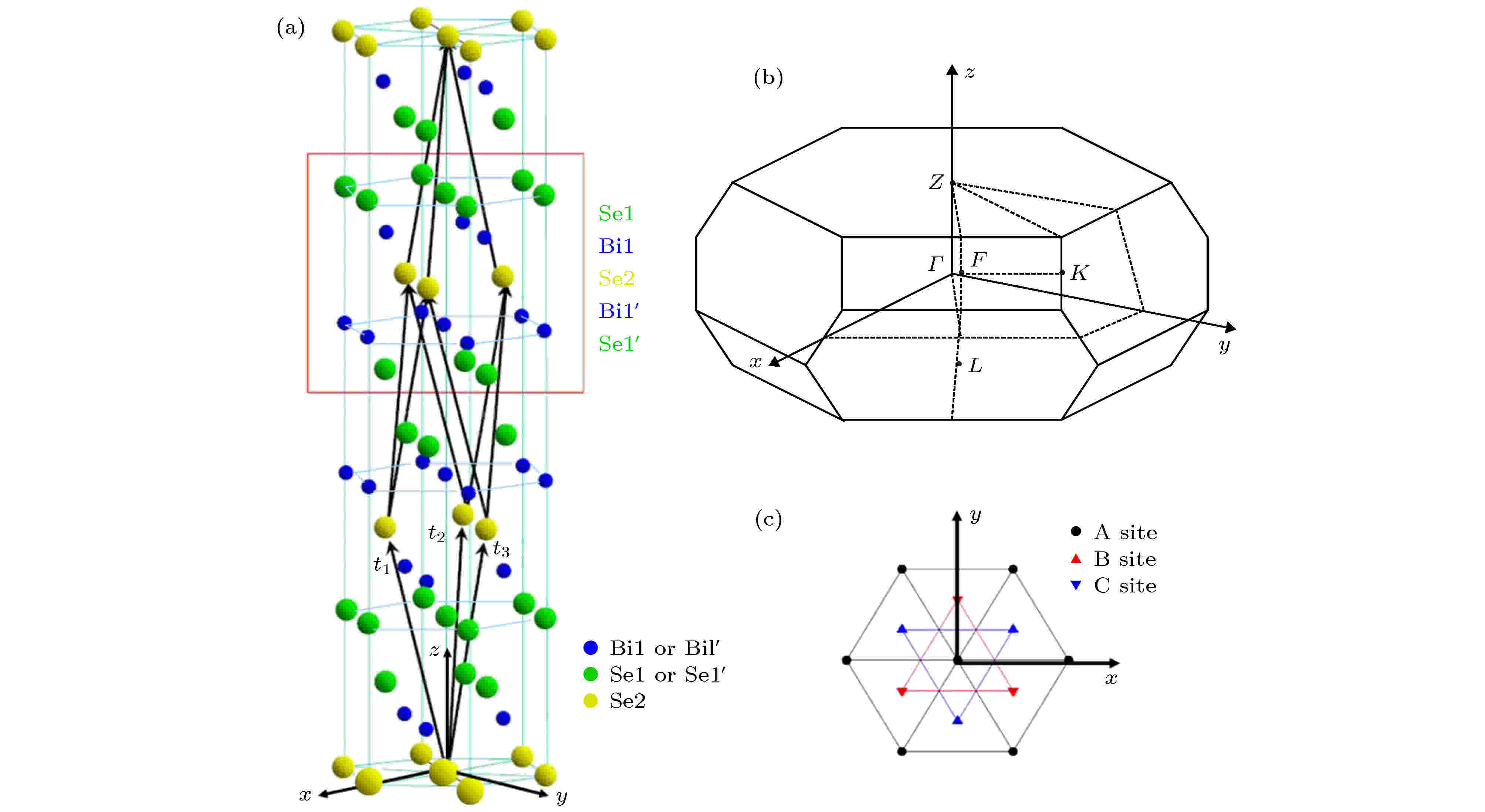

Figure1. The crystal structure of Bi2Se3: (a) 3D schematic of the structure, where

在Bi2Se3的每个QL层内, 相邻原子之间由共价键相连, 原子按照ABCAB的晶体结构堆垛排列(图1(c)). 选择六方晶系的a, b和c作为晶系的x, y和z轴, 由图1(a)可知, Bi2Se3沿z轴呈三重螺旋对称, 而沿x轴呈两重螺旋对称, 并且在原点呈空间反演对称. 图1(c)展示的是Bi2Se3晶格在xy平面的投影(即晶体的0001面), 显然可以看出, 在晶体的表面空间反演对称性被破坏, 晶格的空间群也从


为了确认拓扑绝缘体的表面电子态, 常常使用ARPES来观测生长薄膜的表面和体能带结构. 由于拓扑绝缘体表面电子态具有导体的特性, 而体态绝缘, 所以可以通过ARPES观察到清晰的量子阱态, 如图2所示, 仍以Bi2Se3为例[43]. 由于Bi2Se3主拓扑数为1, 属于强拓扑绝缘体, 所以其表面态只包含一个狄拉克锥[44]. 在E-k能带图中, 自旋轨道耦合导致体能带反转构成拓扑表面态, 表面态贯穿体带隙形成狄拉克锥. 不同于自旋简并的体能带, 这里的两条表面态是高度自旋极化的, 不同表面态上的电子自旋垂直于E-k面且极化方向相反. 由于时间反演对称保护, 在电子动量由k变为–k时, 其自旋方向也会相应改变. 正是强自旋轨道耦合效应赋予了拓扑绝缘体表面的自旋-动量锁定特性, 同时此效应也使拓扑绝缘体能够通过ISHE实现高效自旋-电荷转换. 在实验上通常使用自旋霍尔角(spin Hall angle,

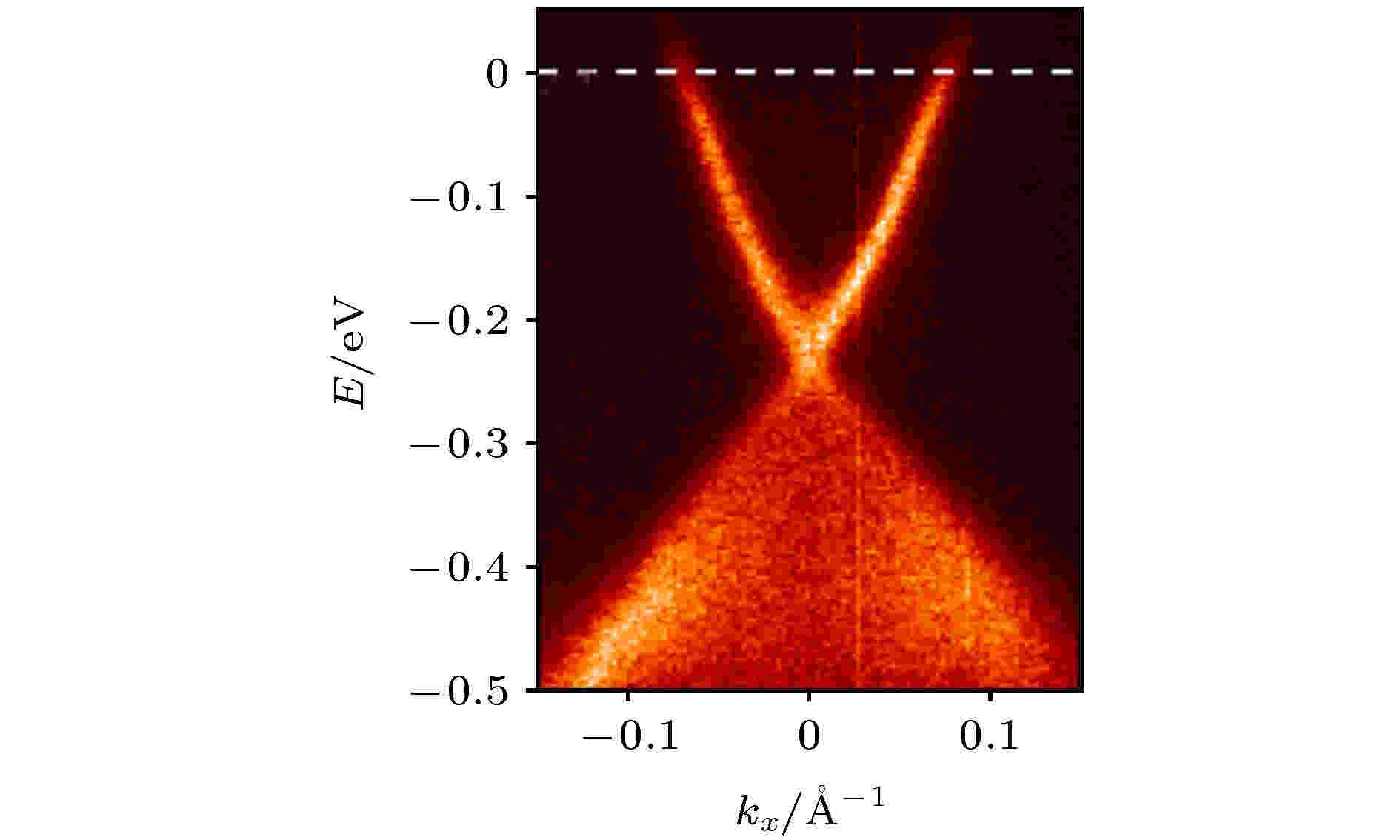
Figure2. ARPES measurements of surface electronic band of Bi2Se3[43].
2
2.2.拓扑绝缘体中的超快光电流
飞秒激光是指时域脉冲宽度在飞秒量级的激光脉冲, 常用的飞秒激光中心波长为800 nm, 激光能量为1.5 eV. 拓扑绝缘体在受到飞秒激光脉冲激发时, 激光能量远大于其体带隙宽度(约为400 meV), 使得低能电子吸收能量向上跃迁[47,4 48], 激发超快光电流J, 产生太赫兹辐射, 其强度正比于
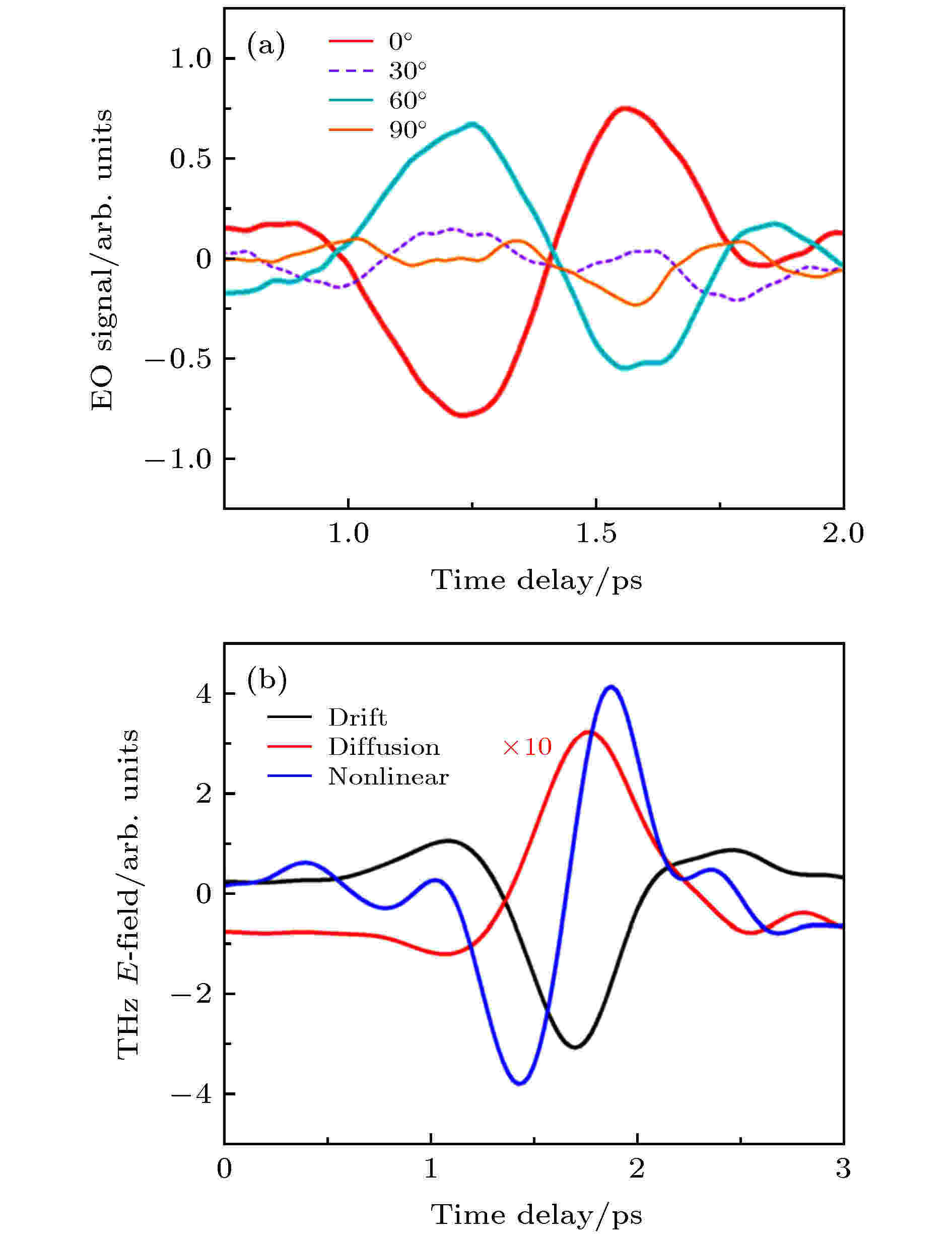
Figure3. Separation of the photo-currents in topological insulator excited by linear femtosecond laser pulse: (a) The derived terahertz signals due to nonlinear currents as a function of azimuthal angle; (b) the extracted terahertz electric field generated by different effects[49].
为了研究圆偏振光对拓扑绝缘体的激发作用, Hamh等[48]在飞秒激光器后面添加一个1/4波片, 并转动波片的角度产生不同偏振态的激光激发 Bi2Se3样品, 通过对出射太赫兹信号的分析得到光电流随激光偏振态的变化关系. 实验结果显示拓扑绝缘体中产生的超快光电流与入射光的手性有关(图4). 随后, Braun等[55]证明了在圆偏振光的激发下, 拓扑绝缘体中存在一个与抽运光手性无关的转移电流和手性依赖的注入电流, 且左旋和右旋圆极化光激发的注入电流极性相反. 这两种电流都来自于拓扑绝缘体表面的PGE效应[56].
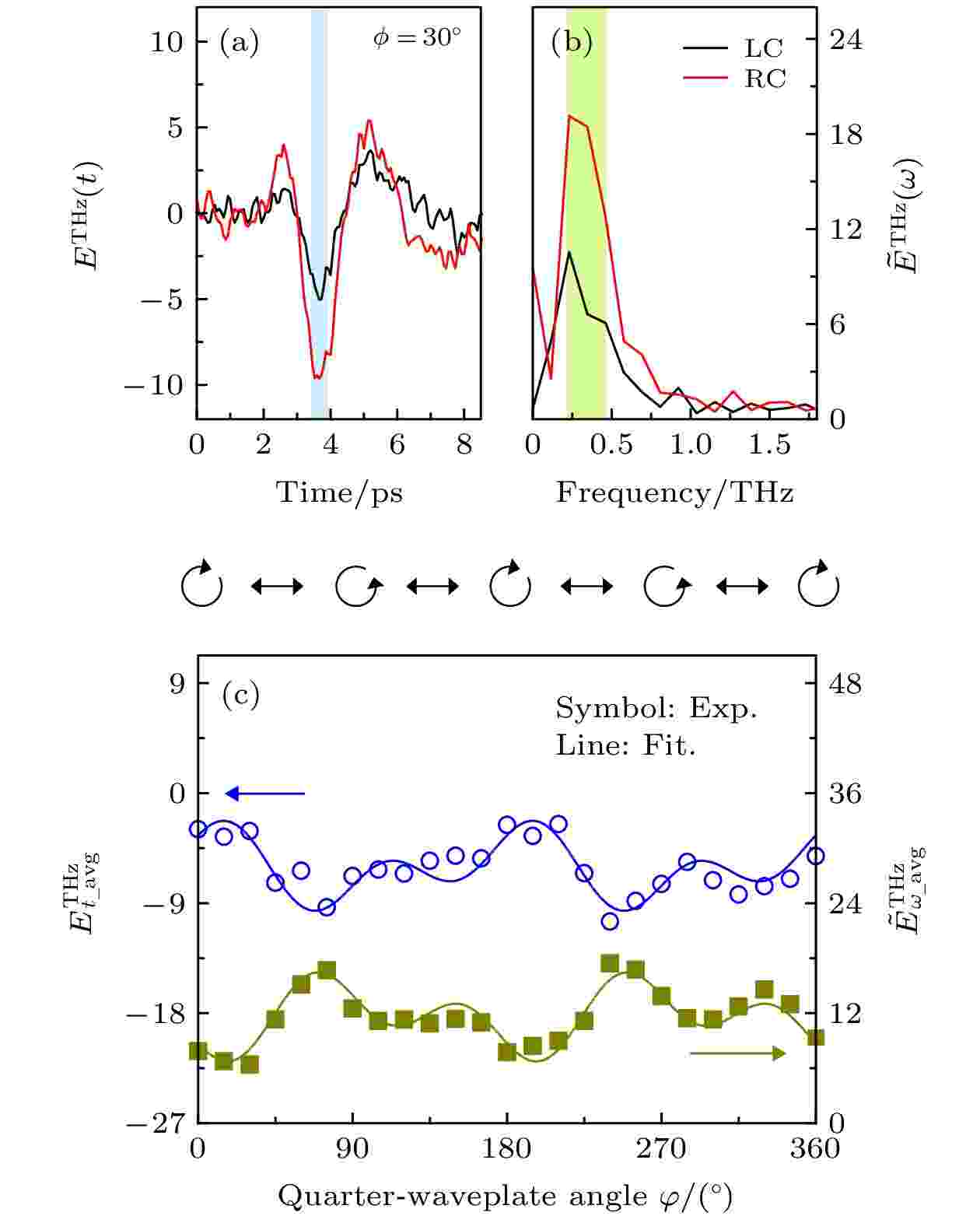

Figure4. (a), (b) THz signals emitted from Bi2Se3 in time and frequency domains under illumination of left- and right- handed circularly polarized light where the azimuth

在1980年, Belinicher和Sturman[57]曾经预言对称破缺晶体中存在PGE效应. 由2.1节可知, 拓扑绝缘体在表面具有中心对称破缺的结构, 由于系统的不对称性激发和弛豫过程, 飞秒激光的照射可以使样品中产生由PGE效应引入的光电流, 并且根据激光的偏振态会产生两种不同的光电效应: 圆光生伏打效应(circular photogalvanic effect, CPGE)和线光生伏打效应(linear photogalvanic effect, LPGE). 为了讨论不同偏振光对这两种光伏打效应的作用, 使用非线性响应理论来唯象地描述拓扑绝缘体材料中PGE效应引起的电磁辐射响应[37,58]:







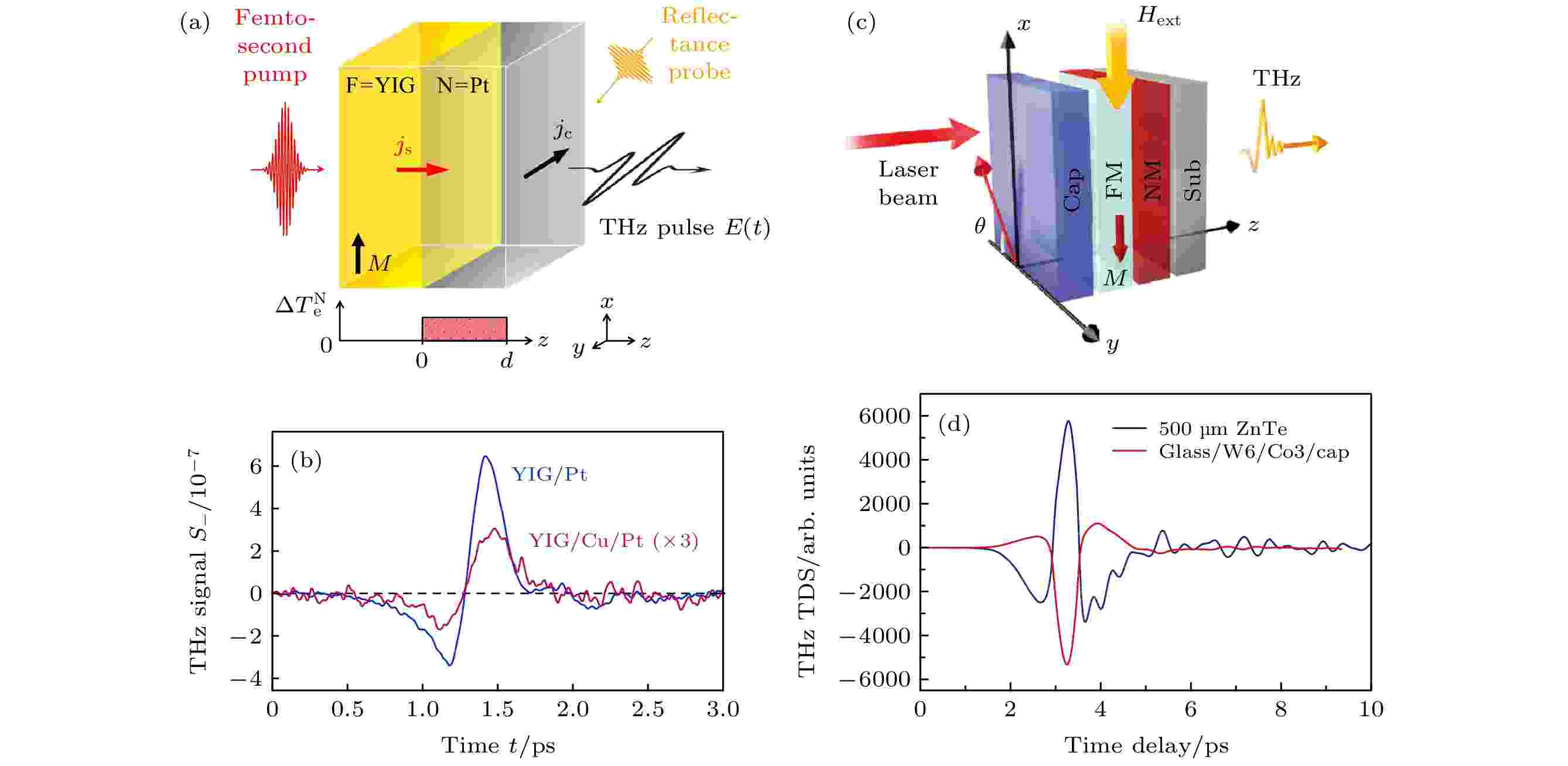
Figure5. (a) The YIG/Pt heterostructure used by Seifert. et al.[74]; (b) after 1.9 nm Cu insertion, the THz field intensity deteriorates because the spin injection is impaired[74]; (c) the Co/W heterostructure used by Wu et al.[82]; (d) the THz waves emitted from Co/W have a peak intensity exceeding that of ZnTe crystals[82].


在拓扑绝缘体的非线性效应中, 除了LPGE与CPGE之外, 还存在着PDE和二阶非线性OR, 即飞秒激光脉冲激发拓扑绝缘体时的非线性光电流响应可以表示为






2
2.3.拓扑绝缘体中的太赫兹辐射调控
由(2)式可知, 左旋和右旋的极化光会在拓扑绝缘体表面激发极性相反的CPGE分量, 即抽运光的极化状态会影响拓扑绝缘体中的太赫兹发射. 事实上, 单层拓扑绝缘体中的太赫兹发射还受到很多因素影响, 如样品方位角(晶体取向)



非线性效应 | 晶体方向$ \phi $ | 入射角($ \theta \to -\theta $) | 1/4波片转角$ \alpha $ |
CPGE | 来源于表面态 | 极性反转 | 2$ \alpha $-周期 |
与$ \phi $无关 | $ \mathrm{s}\mathrm{i}\mathrm{n}\left(2\alpha \right) $ | ||
LPGE | 来源于表面态 | 极性反转 | 4$ \alpha $-周期 |
$ \phi $依赖 | $ \mathrm{s}\mathrm{i}\mathrm{n}\left(4\alpha \right) $ | ||
PDE | $ \phi $依赖 | 极性反转 | 4$ \alpha $-周期 |
$\cos4\alpha$ | |||
OR | $ \phi $依赖 | 极性不反转 | 4$ \alpha $-周期 |
$ \mathrm{c}\mathrm{o}\mathrm{s}\left(4\alpha \right) $ |
表1拓扑绝缘体中的超快光电流与晶体取向?, 入射角θ, 激光偏振态的依赖关系[56]
Table1.The details of the dependences of CPGE, LPGE, PDE, and OR on



值得注意的是, Tu等[56]认为LPGE与方位角无关, 他们将出射太赫兹波中随方位角变化的分量归因于PDE和OR的贡献. 然而, 最近针对外尔半金属的研究证明LPGE来源于不同元素原子之间电荷分离产生的转移电流, 这种转移电流沿原子间的化学键流动[67]. 在Bi2Se3中, 由于Bi—Se键在z轴上的对称性(图5), LPGE产生的光电流一定与样品方位角呈120°周期性变化. 这与Braun等[55]和Fang等[49]的结论是一致的.
2
3.1.异质结中的超快自旋注入
对铁磁/非磁异质结施加一个面内的磁场, 在异质结受到飞秒激光照射时, 可以在非磁层检测到一个超快电流, 它来源于铁磁层中产生的自旋流. Uchida等[73]认为这种自旋流来源于铁磁材料中的自旋塞贝克效应. Seifert等[74]在YIG/Pt异质结中观察到了自旋塞贝克效应导致的超快自旋注入. 当飞秒激光照射时, 金属层的电子被激发撞击磁性层, 使其产生一个随机的转矩, 进而产生净自旋流





然而, 并非所有的自旋注入现象都能单一地用自旋塞贝克效应解释. 当飞秒激光激发异质结时, 会在铁磁层激发超扩散的瞬态自旋流[75,76], 进而导致自旋流注入. Kampfrath等[19]利用飞秒激光脉冲激发Fe/Au异质结, 观察到了太赫兹辐射, 并将异质结中的超快自旋注入归因于超扩散自旋传输(superdiffusive spin transport). 在电子吸收能量跃迁后, 自旋向上的电子表现为sp轨道特性, 而自旋向下的电子表现为类d轨道特性. 由于上下自旋电子寿命与迁移率的不同, 两种电子的自旋取向也处于非平衡状态, 因此在铁磁层界面形成了自旋流的积累, 实现了自旋流向非磁层注入. 这种激光导致的超扩散自旋流, 其动态频率恰好处在亚皮秒量级, 因此也可以实现太赫兹量级的动态自旋注入[77]. 与自旋塞贝克效应一致, 这种自旋流的极化方向与铁磁层磁化方向也是相同的.
除以上两种自旋注入机制之外, 磁性材料中超快退磁现象也会产生自旋流, 这种自旋注入机理被称为自旋抽运效应(spin pumping effect). 由飞秒激光诱导的超快退磁现象在太赫兹领域已经被广泛地报道[18,78], 在超快退磁的过程中, 铁磁层的自旋角动量转移到重金属层, 进而产生自旋注入. 由于自旋抽运的特征时间与自旋轨道相互作用有关, 其时间尺度为皮秒量级[79], 因此同样可以产生太赫兹辐射. 然而, 由于飞秒激光引入的磁性变化很小, 所以自旋抽运效应导致的自旋注入很弱. Kampfrath等[19]计算了Fe/Au异质结中自旋抽运效应注入的自旋流比超扩散导致的超快自旋流小两个数量级以上.
值得注意的是, 目前尚缺乏统一的理论来描述异质结中的超快自旋注入过程, 特别是对于自旋塞贝克效应和超扩散效应这两种自旋注入机制, 现有的光学和电学手段无法将两种效应注入的自旋流区分开来. 因此, 现在无法确切地指出哪种自旋注入机制的贡献对异质结中的太赫兹辐射占主导作用.
2
3.2.异质结中的自旋-电荷转化及太赫兹发射
在自旋流从铁磁层注入相邻非磁层中后, 由于非磁层材料的自旋轨道耦合作用, 不同自旋取向的电子朝不同的方向偏转, 转化为电荷的积累, 这就是ISHE. 在自旋流









经过对铁磁/非磁异质结的材料筛选与结构优化, 现在已经能够实现兼顾辐射强度、带宽与功耗的自旋太赫兹发射源. Wu等[82]研究了不同厚度下的铁磁与非磁层对出射太赫兹强度的影响, 发现W(6 nm)/Co(3 nm)双层异质结所辐射的太赫兹强度接近ZnTe晶体发射源. 并且, 这种结构的自旋太赫兹发射源还具有低功耗的特性, 即使激光能量密度降至0.6 μJ/cm2, 仍能在异质结中实现稳定的太赫兹发射. Seifert等[20]使用W/CoFeB/Pt构成三层异质结构代替传统的双层结构, 由于W和Pt具有相反的自旋霍尔角, 两层界面中产生的太赫兹辐射可以相互叠加, 进而产生1—30 THz的相干增强太赫兹脉冲, 其能量转换效率甚至优于商用光电导天线和GaP(110)太赫兹发射源.
最近, Zhou等[83]首次利用反铁磁外尔半金属Mn3Sn与重金属Pt构成的异质结实现了太赫兹发射. 由于Mn3Sn具有反三角的自旋结构, 不同晶相的Mn3Sn产生的纵向自旋流大小不同, 因而在制备过程中可以通过选择Mn3Sn层的晶相来控制异质结出射太赫兹波的强度. 另外, 由于Mn3Sn同时具有较大的自旋霍尔角, 这种外尔半金属也可以代替重金属层提供ISHE. Zhou等的研究不但证明了太赫兹可以作为研究外尔半金属自旋结构的一种简便方法, 更证明了Mn3Sn具有实现自旋太赫兹发射与调控的重要潜力.
2
3.3.铁磁/非磁异质结中的自旋太赫兹调制
由公式(5)可知, 异质结中发射的自旋太赫兹偏振方向垂直于磁化方向, 然而与拓扑绝缘体中发射的太赫兹不同, 自旋太赫兹不依赖于入射光的偏振状态. 也即, 自旋太赫兹源的抗干扰能力极强, 改变铁磁层磁化方向, 可以任意调控其偏振方向.然而, 在均匀磁性的异质结中, 发射的自旋太赫兹一定是线偏振的, 这大大限制了自旋太赫兹源的应用途径. 为了突破性这一局限, Hibberd等[84]通过改变外磁场的方向, 控制铁磁层磁矩分布, 实现了对出射太赫兹偏振态的调控. 他们使用两个极性相反的永磁铁作为磁场源, NiFe/Pt异质结作为太赫兹发射源, 在调换永磁体极性时, 异质结中出射的太赫兹极性反转. Kong等[85]则从理论上证明了异质结中不均匀的磁性分布可以产生椭圆偏振的自旋太赫兹辐射. 特别地, 在不同磁性区域的有效面积相等且磁矩方向相互垂直时, 还可以产生圆偏振极化的太赫兹辐射. 他们讨论了出射太赫兹椭圆率与磁场极化状态的依赖关系并用实验加以证实. 如图6(a),(b)所示, 将样品置于螺旋的磁场中, 在磁场的手性变化时, 太赫兹波的极化方式由左旋变为右旋. 然而, 由于很难精准控制单级铁磁薄膜中的磁性分布, 所以通过改变外磁场获得偏振可调谐的太赫辐射难度很大. Chen等[22]报道了使用级联发射的方法可以产生可控椭圆偏振太赫兹波. 他们在第一级样品上施加了水平方向的磁场, 使其产生竖直偏振的太赫兹辐射, 在第二级上施加了竖直方向上的磁场产生水平偏振的太赫兹辐射; 通过改变两级薄膜之间的距离控制出射太赫兹波的相位差, 实现了椭圆偏振太赫兹波的合成(图6(c)). 进一步, 当抽运光经过第一级的发射损耗与第二级铁磁薄膜本身对第一级辐射的太赫兹波的损耗相等时, 可以实现圆偏振太赫兹波辐射(图6(d)).
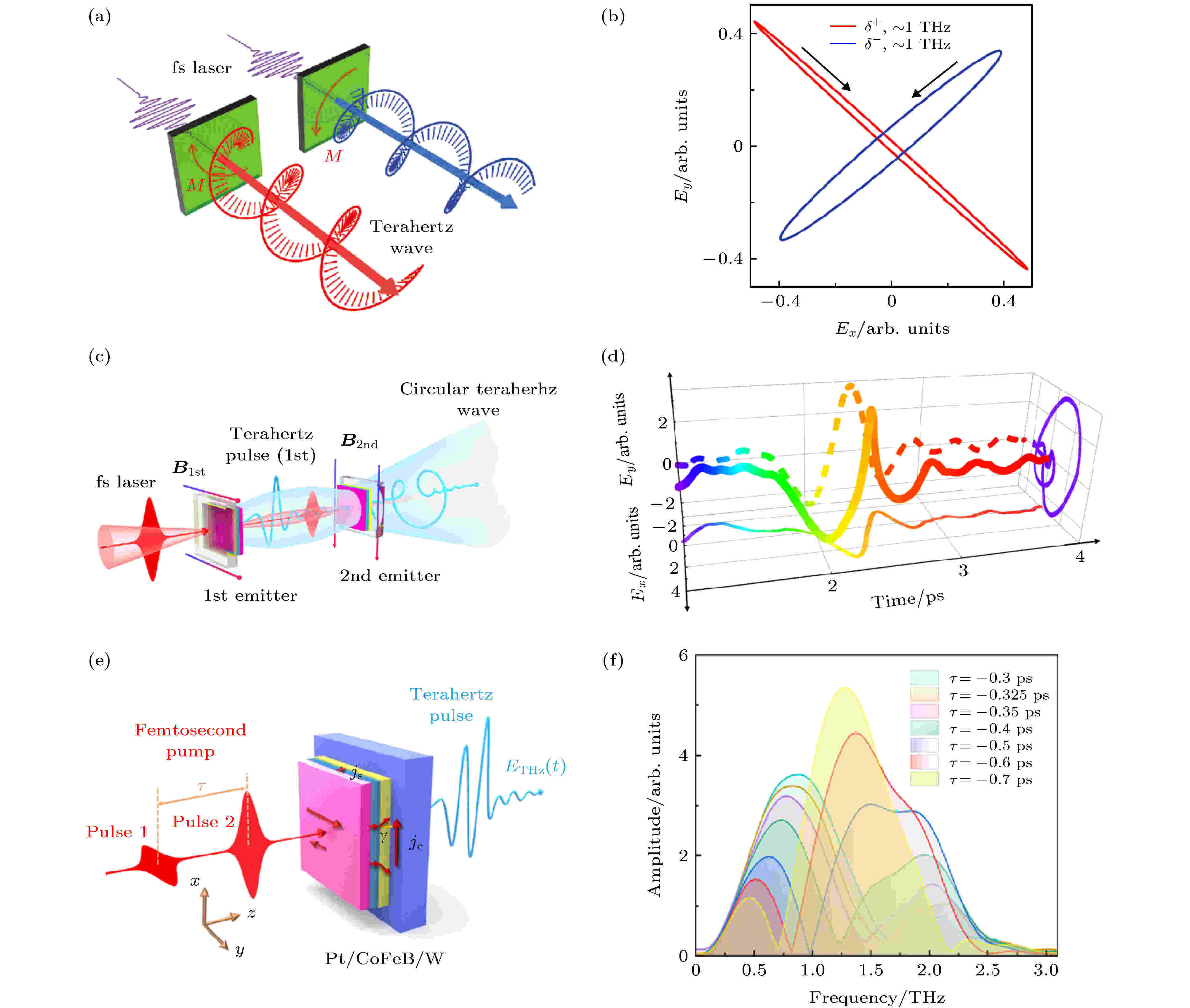


Figure6. (a) Manipulation of the terahertz chirality by changing the twisted magnetic field distribution; (b) the Lissajous curves of the THz signals of (a), where


除了实现时域调制之外, 自旋太赫兹发射源在频域调制方面也具有无可比拟的优势. 2019年, Wang等[21]报道了双抽运效应对自旋太赫兹频谱的调节作用, 如图6(e)—(f)所示, 当使用两束飞秒激光同时抽运铁磁/非磁性金属异质结时, 可以通过操控两束脉冲激光之间的偏振夹角和时延来实现对出射太赫兹频域信号的调制.
4.1.拓扑绝缘体的自旋霍尔角计算
第3节主要介绍了铁磁/非磁异质结中的自旋太赫兹发射, 公式(5)和(6)表明在异质结中自旋太赫兹的发射效率取决于非磁层的自旋霍尔系数, 而自旋霍尔系数正比于自旋霍尔角





自旋-电荷转换效率指的是在自旋注入时, 拓扑绝缘体利用ISHE将其转化成电荷流的能力. 为了能够定量地计算拓扑绝缘体的自旋-电荷转换效率, Wang等[88]使用自旋力矩铁磁共振(spin torque ferromagnetic resonance, ST-FMR)的方法测量了Bi2Se3的自旋霍尔角. 如图7(a)所示, 他们在Bi2Se3/Co40Fe40B20(CFB)两端施加一个射频电流, 通过外部整流电路测得输出的电压信号. 在射频电流流经Bi2Se3层后, 由于自旋霍尔效应在y方向发生极化, 产生纯自旋流并注入到相邻的CFB中, 改变CFB磁矩的阻尼矩引发磁矩进动, 并且射频电流会在系统中产生一个y方向的有效场, 也会使CFB受到力矩的作用. 由于ST-FMR电压信号中对称线形分量来源于自旋流产生的阻尼矩, 而反对称线型分量来源于射频磁场的贡献[89,90], 因此可以通过线形与非线形部分的比值来确定自旋流与电荷流的比值, 即自旋霍尔角


Material | $ {\theta }_{\mathrm{S}\mathrm{H}} $ |
Ta | 0.15 |
W | 0.40 |
Pt | 0.08 |
Bi2Se3 | 2.00—3.50 |
BixSe1–x | 18.80 |
BixSb1–x | 52.00 |
表2拓扑绝缘体与几种重金属材料的自旋霍尔角[35]
Table2.Spin Hall angles of several topological insulators and common heavy metals[35]
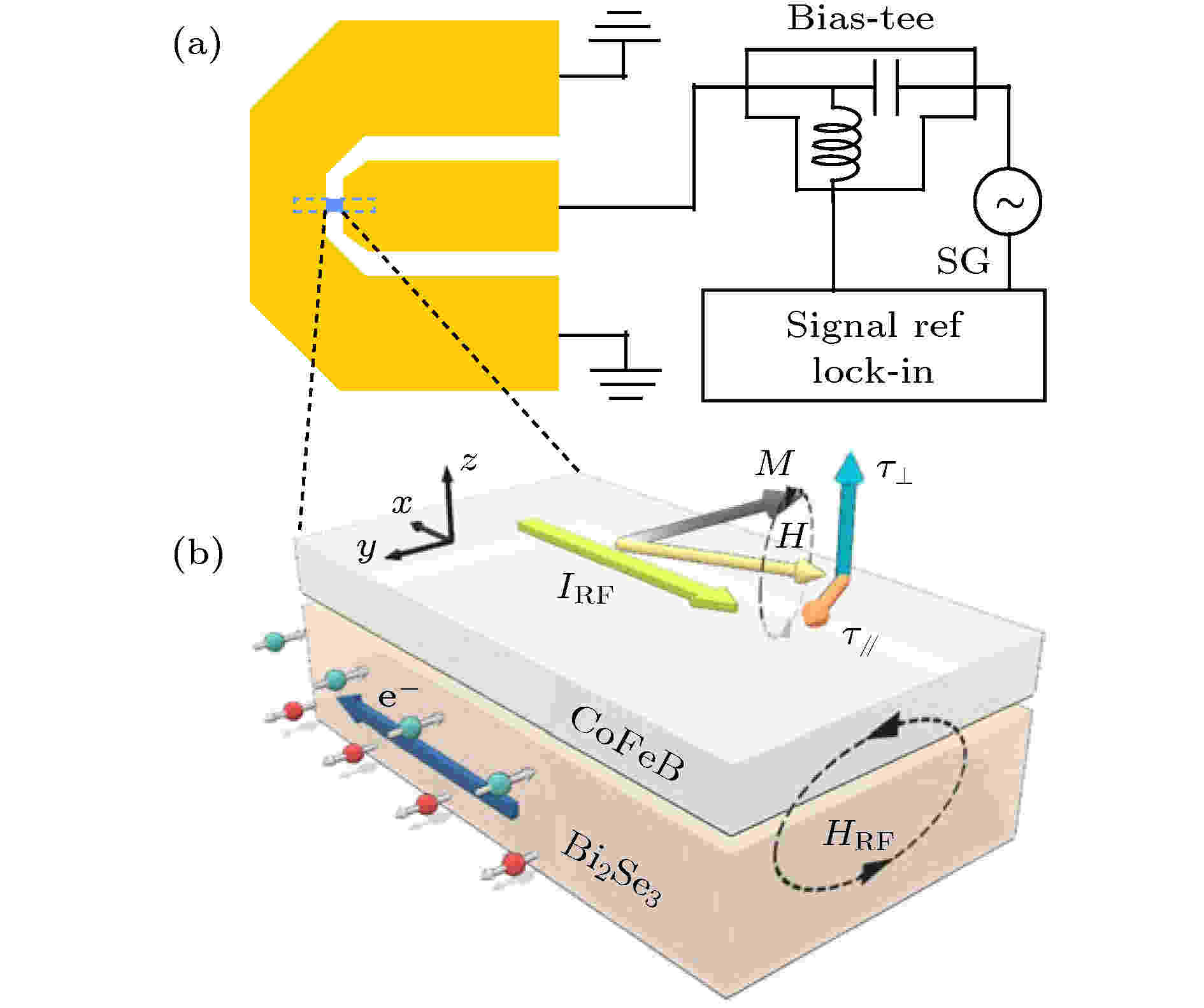
Figure7. (a) The schematic diagram of the ST-FMR measurement setup, an RF current from a signal generator (SG) is injected into the devices; (b) magnetization movements in the ST-FMR measurements[88].
2
4.2.绝缘体/铁磁异质结中的自旋太赫兹发射
由于拓扑绝缘体极大的自旋-电荷转换率, 在铁磁/拓扑绝缘体异质结中可以实现高效的自旋太赫兹发射. 2018年, Wang等[68]首次利用Bi2Se3/Co异质结实现了自旋太赫兹发射. 如图8(a)所示, 他们使用波长为800 nm功率为60 mW的飞秒激光垂直入射异质结, 激光激发使Co层产生超快自旋流并注入相邻的Bi2Se3中, 再由ISHE将飞秒激光诱导的自旋流转换成亚皮秒尺度上的横向电荷流, 进而产生太赫兹辐射. 为了排除单层拓扑绝缘体中非线性电流和铁磁层的超快退磁对出射太赫兹的贡献, 分别测量了飞秒激光激发下Bi2Se3和Co的太赫兹辐射, 如图8(b)所示, 显然异质结中自旋-电荷转换引入的自旋太赫兹发射在总太赫兹辐射中占据主导地位. 当抽运光从前入射变为后入射时, 太赫兹信号的极性发生


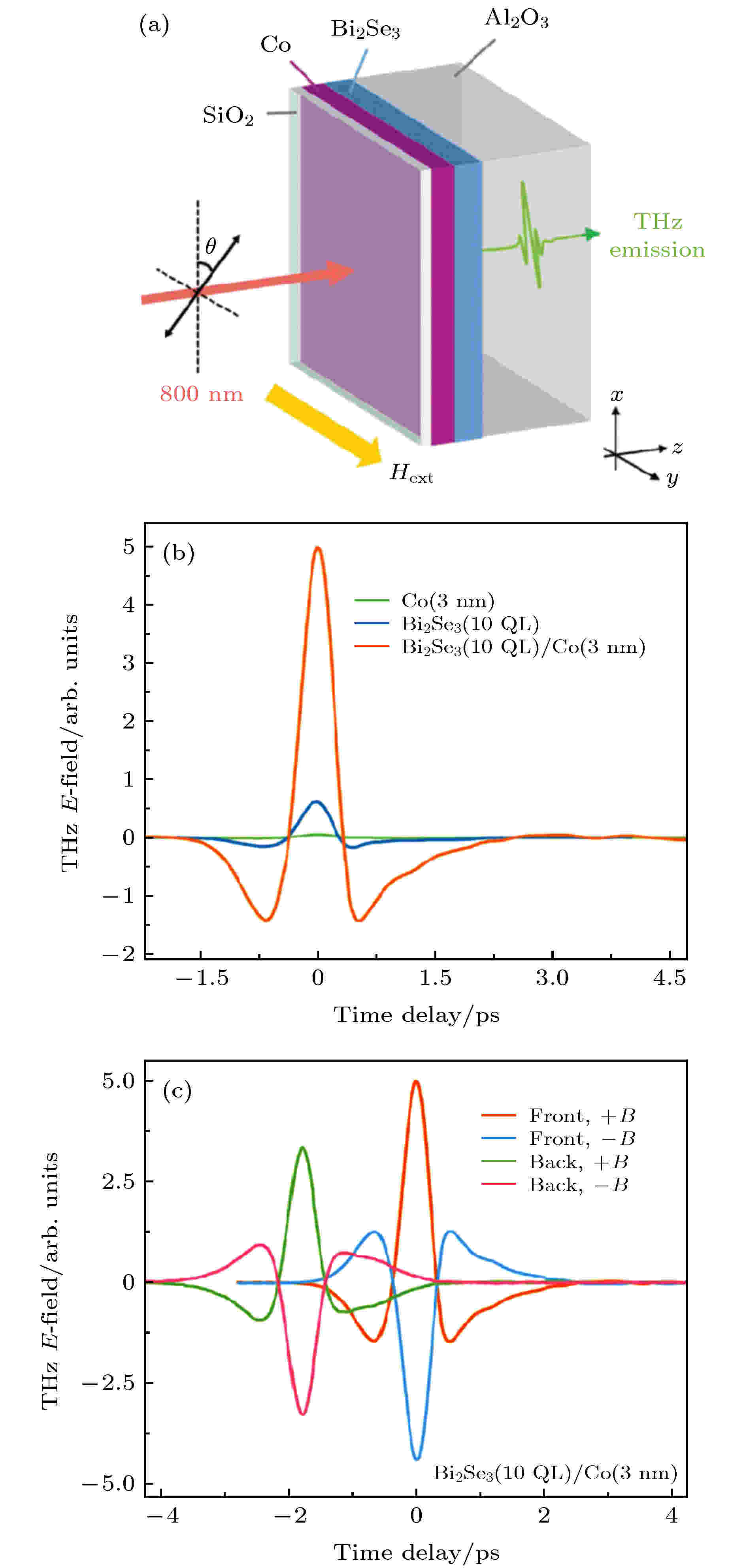
Figure8. (a) The schematic diagram of the Bi2Se3/Co heterostructure; (b) THz waveforms generated from Bi2Se3/Co, Co and Bi2Se3; (c) THz waveforms emitted from the heterostructure measured with front and back sample excitation and reversed magnetic field[68].
为了验证Bi2Se3/Co异质结的发射效率, Wang等[68]使用与Bi2Se3表面相同厚度(2 nm)的Pt作为对照组, 对比他们产生自旋太赫兹幅度的差异. 实验结果显示, 在相同的有效厚度下, Bi2Se3的发射效率是Pt的1.7倍, 其中Bi2Se3 (10 nm)/Co (3 nm)的发射效率更是可以与商用的ZnTe晶体相媲美. 更重要的是, 他们证明了拓扑绝缘体中的自旋-电荷转换对温度的变化不敏感. 这些优点充分说明了拓扑绝缘体/铁磁异质结对实现高效、稳定、廉价的太赫兹发射器的重要意义. 并且, 由于拓扑绝缘体特殊的拓扑表面态, 其本身就可以发射太赫兹信号, 因此对拓扑绝缘体/铁磁异质结的研究有望实现任意调谐的拓扑自旋太赫兹发射器.
2
4.3.拓扑自旋太赫兹发射器的前景
拓扑绝缘体在与铁磁层材料组合构成自旋太赫兹发射器时, 可以产生较大强度的自旋太赫兹辐射, 出射的自旋太赫兹波为线偏振且偏振方向只依赖于铁磁层的磁化方向. 然而, 由前面的讨论可知, 拓扑绝缘体单层薄膜即可辐射太赫兹, 其产生机理为材料内部的非线性效应. 如表1所示, 可以通过改变抽运光入射角、抽运光的极化状态和样品方位角对单层拓扑绝缘体出射太赫兹波进行调控. 因此, 可以通过控制外磁场方向与样品方位角, 调控单层拓扑绝缘体中非线性太赫兹的偏振方向. 由于自旋太赫兹发射不依赖于非线性效应, 即非线性太赫兹和自旋太赫兹的偏振方向会有一个夹角, 且超快电流的响应速度远大于自旋注入, 即两束太赫兹波之间一定存在一个相位差. 因此在飞秒激光激发时, 异质结中出射的两束太赫兹波可以在空间上合成椭圆极化的太赫兹波. 进一步地, 通过控制单层拓扑绝缘体中的超快光电流响应, 可以实现对出射太赫兹手性的调控.在2.2节中讨论了拓扑绝缘体的各种非线性光电流效应, 可以看出拓扑绝缘体薄膜中的太赫兹发射与其狄拉克表面态有关, 然而, 在飞秒激光激发时, 由于体载流子对激光的吸收作用, 拓扑绝缘体产生的超快光电响应可能会被抑制[93]. 因此, 可以改变拓扑绝缘体的费米能级, 使其落在体能隙上, 降低体载流子密度, 进而增强发射的太赫兹强度. 在实验上通常使用施加栅极电压[94,95]或掺杂金属元素[96,97]的方法调控拓扑绝缘体的费米能级. Pan等[98]制备了带有顶电极的(BixSb1–x)2Se3单层薄膜样品(图9(a)), 研究了飞秒激光激发下拓扑绝缘体内部超快光电流与栅极电压的关系. 如图9(b)所示, 当栅极电压为0 V时, 样品的纵向电阻


编号 | 材料 | 载流子浓度/ 1018 cm–3 | 太赫兹峰值/ mV·cm–1 |
1 | Bi2Se3 | –75.5 | 1.24 |
2 | Bi2Se3 | –34.6 | 7.75 |
3 | Bi2Se3 | –31 | 5.27 |
4 | Bi2Se3 | –15.6 | 11.10 |
5 | Cu0.02Bi2Se3 | –3.66 | 54.39 |
6 | Cu0.08Bi2Se3 | –4.23 | 55.77 |
7 | Cu0.1Bi2Se3 | –1.96 | 39.37 |
8 | Cu0.125Bi2Se3 | –1.17 | 52.32 |
表3不同载流子浓度下Bi2Se3辐射的太赫兹峰值强度[99]
Table3.Carrier concentration and THz peak amplitude for Bi2Se3 films[99]
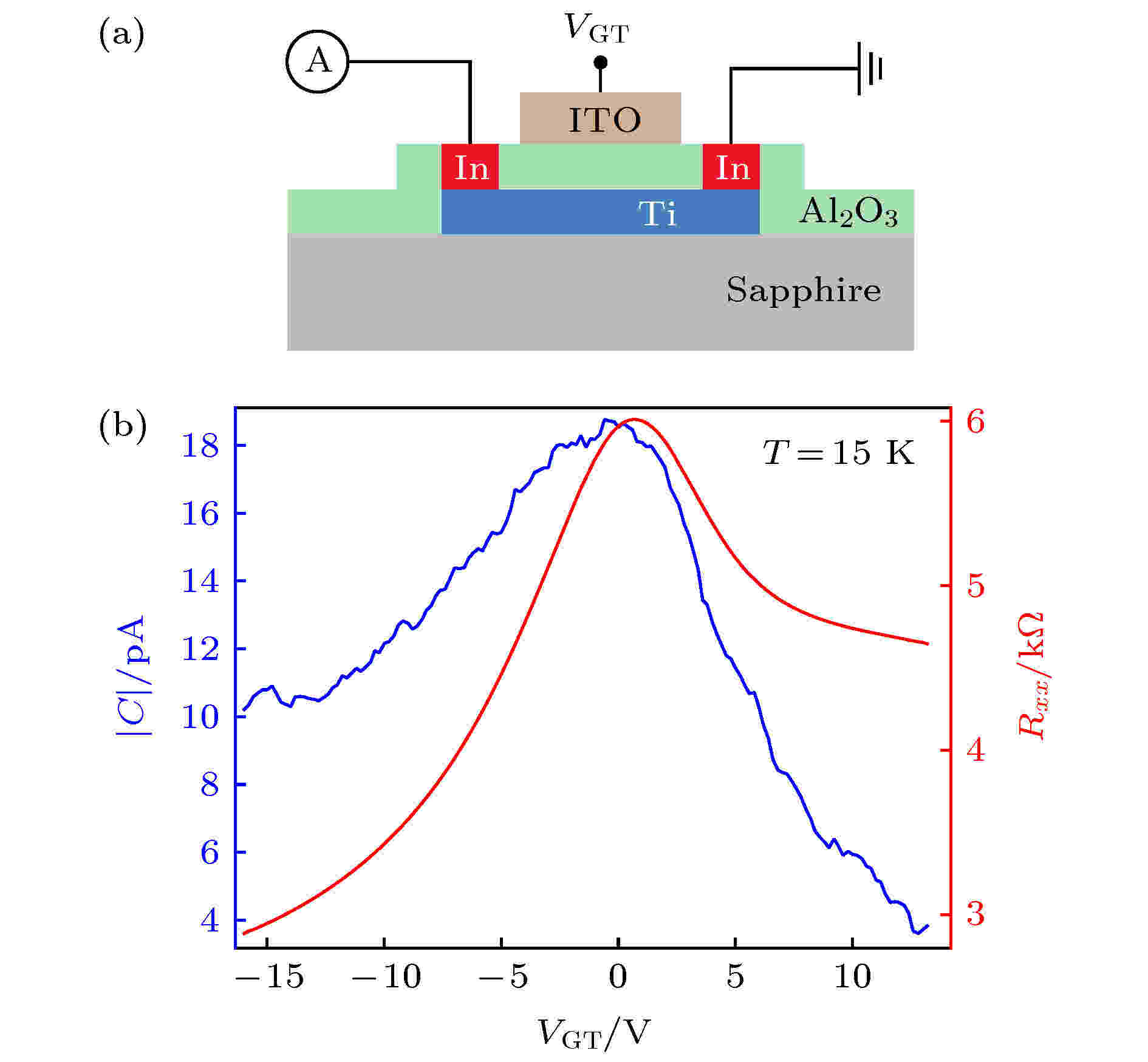
Figure9. (a) The Schematic diagram of the top-gate device prepared by Pan et al, where the Al2O3 is dielectric layer while the ITO serves as top gate material; (b) the gate-dependent longitudinal resistance and nonlinear current in (BixSb1–x)2Se3 film[98].
综上所述, 拓扑绝缘体/铁磁异质结可以发射手性可调的椭圆太赫兹波, 并且可以通过施加栅极电压控制出射非线性太赫兹的幅值, 如果能够结合这两个优势, 即通过改变晶格和磁场方向调控出射太赫兹的偏振态, 施加栅极电压改变非线性太赫兹分量的幅值, 就可以通过拓扑绝缘体/铁磁异质结实现任意调谐的太赫兹波发射.
在这篇综述中, 首先, 从拓扑绝缘体的基本结构入手, 以Bi2Se3为例讨论了拓扑绝缘体的非中心对称表面, 结合ARPES分析了其拓扑保护的非平庸表面态. 这种特殊的能带结构是拓扑绝缘体实现高效自旋太赫兹发射的根本原因. 通过对拓扑绝缘体内部的几种非线性效应的分析, 说明了拓扑绝缘体薄膜出射太赫兹波对晶体方向


接下来, 为了证明拓扑绝缘体/铁磁异质结作为自旋太赫兹发射器的优越性, 首先分析了基于铁磁/非磁异质结中的自旋太赫兹发射. 在飞秒激光的激发下, 由于自旋塞贝克效应等超快动力学效应, 在铁磁层中激发一个纯自旋流并被注入非磁层. 由于ISHE, 注入的自旋流被转换成亚皮秒时间尺度上的横向电荷流, 进而产生太赫兹辐射. 自旋太赫兹具有稳定、廉价、低功耗、易于调控等优点, 比如可以通过级联两组异质结实现圆偏振的太赫兹发射, 或者通过双抽运激发异质结实现对太赫兹频谱的调控. 由于自旋太赫兹的偏振方向只依赖于铁磁层的磁化方向, 因此可以通过改变磁场分布实现手性可控太赫兹发射.
由于拓扑绝缘体有强自旋轨道耦合特性, 可以实现高效的自旋-电荷转化, 因此可以使用拓扑绝缘体材料代替非磁层构成拓扑自旋发射器. 在相同的有效厚度下, 拓扑绝缘体的自旋太赫兹发射效率是重金属的1.7倍, 经厚度优化后, 其发射效率可以与商用的ZnTe晶体相媲美. 拓扑自旋太赫兹发射器在保留自旋太赫兹优势的前提下, 可以通过改变晶体方向单独调控拓扑绝缘体中非线性效应产生的太赫兹辐射, 其出射的非线性太赫兹波与异质结中出射的自旋太赫兹波可以在空间中合成椭圆太赫兹波. 并且, 可以通过施加栅极电压或掺杂调控拓扑绝缘体的费米能级, 进而改变出射非线性太赫兹的幅值. 将两个优点结合起来, 可以实现圆偏振的太赫兹发射. 目前国内外的研究表明, 基于拓扑绝缘体/铁磁异质结构的拓扑自旋太赫兹发射器可以成为实现偏振任意可调谐太赫兹脉冲辐射的一种有效方案.