全文HTML
--> --> -->对于无机填料/聚偏氟乙烯(PVDF)聚复合材料而言, 聚合物和无机的表面性能差异巨大, 导致两相界面的相容性差、结合力较弱, 很难实现良好的界面结合, 特别是高含量的钛酸钡基填料粉体(尤其纳米粉体)填充时, 填料极易团聚, 在复合材料内部形成大量的孔洞, 显著降低复合材料的介电常数和耐击穿强度, 进而影响其在高频、高性能无源器件中的应用. 国内外研究结果表明, 持续增加填充粒子在聚合物基体中的体积分数(> 60%), 室温介电常数的增加并不明显(< ~100). 为了改善此问题, 已有研究表明: 将具有各向异性的材料分散到聚合物基体中, 因其高的长径比(各向异性尺寸), 能在较低的体积分数下建立填充物间的有效连接, 增强颗粒间的相互作用, 从而能有效降低单独颗粒的去极化作用, 可使复合材料在较小的填充量下达到相互作用, 获得高的介电性能, 同时保持聚合物基体优良的机械性能[6]; 南策文课题组[13,14]采用静电纺丝法获得了高长径比的Ba0.6Sr0.4TiO3纳米纤维, 添加到高聚物基体中(PVDF), 显著增加了复合材料的耐击穿特性, 同时具有较好的柔韧性. 如何改善陶瓷粉体的界面性能, 提高其在聚合物基体中的分散性, 增强陶瓷-界面层-聚合物间的键合作用, 已成为陶瓷聚合物复合领域中一个重要的研究方向和研究热点[15-21].
表面处理对复合材料的界面性能、介电性能、储能性能有着重要的影响, 同时顺电材料钛酸锶呈现出较瘦的电滞回线, 具有高的饱和极化强度和较低的剩余极化强度, 顺电材料在相同的电场强度下, 能够放出更多的能量, 具有较高的储能效率. 研究顺电材料储能特性对制备大功率、高储能密度的电容器具有重要的理论价值和实际应用意义, 为了改善钛酸锶粉体填料在PVDF基体中的分散情况、提高钛酸锶填料与PVDF基体间的界面结合情况, 本文采取了表面羟基化处理改性的方法对钛酸锶纳米纤维一维填料进行表面改性, 研究羟基化处理改性钛酸锶纳米纤维一维填料对复合材料的介电性能、储能密度的影响.
ST NF—OH/PVDF复合材料的制备流程: 称取适量的表面羟基化改性后ST NF—OH填料与N, N-二甲基甲酰胺(DMF)加入到烧杯中超声分散, 并向反应体系中加入适量PVDF粉末, 然后用磁力搅拌器在85 ℃搅拌4 h, 将得到的白色黏稠状液体在ITO玻璃上采用流延法铺膜, 然后将涂有浆料的ITO玻璃置于80 ℃的干燥箱中干燥5 h, 得到白色复合薄膜, 再将复合薄膜放入220 ℃的温度下保温10 min, 之后放入冰水混合物中进行淬火, 经80 ℃的条件下干燥4 h, 得到聚合物基复合膜.
采用D8 AdvanceX射线衍射仪(XRD)研究表面羟基化对填料相结构的影响, 采用XL30 FEG场发射扫描电子显微镜(SEM)表征复合薄膜的表面及断面的微观形貌, 利用Kratos Axis Ultra DLD型X射线光电子能谱仪测试样品的X射线光电子能谱(XPS), 采用安捷伦Keysight E4980A精密LCR表测试样品介电常数、介电损耗随频率(20 Hz—2 MHz)的变化, 利用MS2677-I型号的耐压测试仪测试样品的耐击穿性能, 采用TF Analyzer 2000铁电分析仪测试样品的P-E曲线.
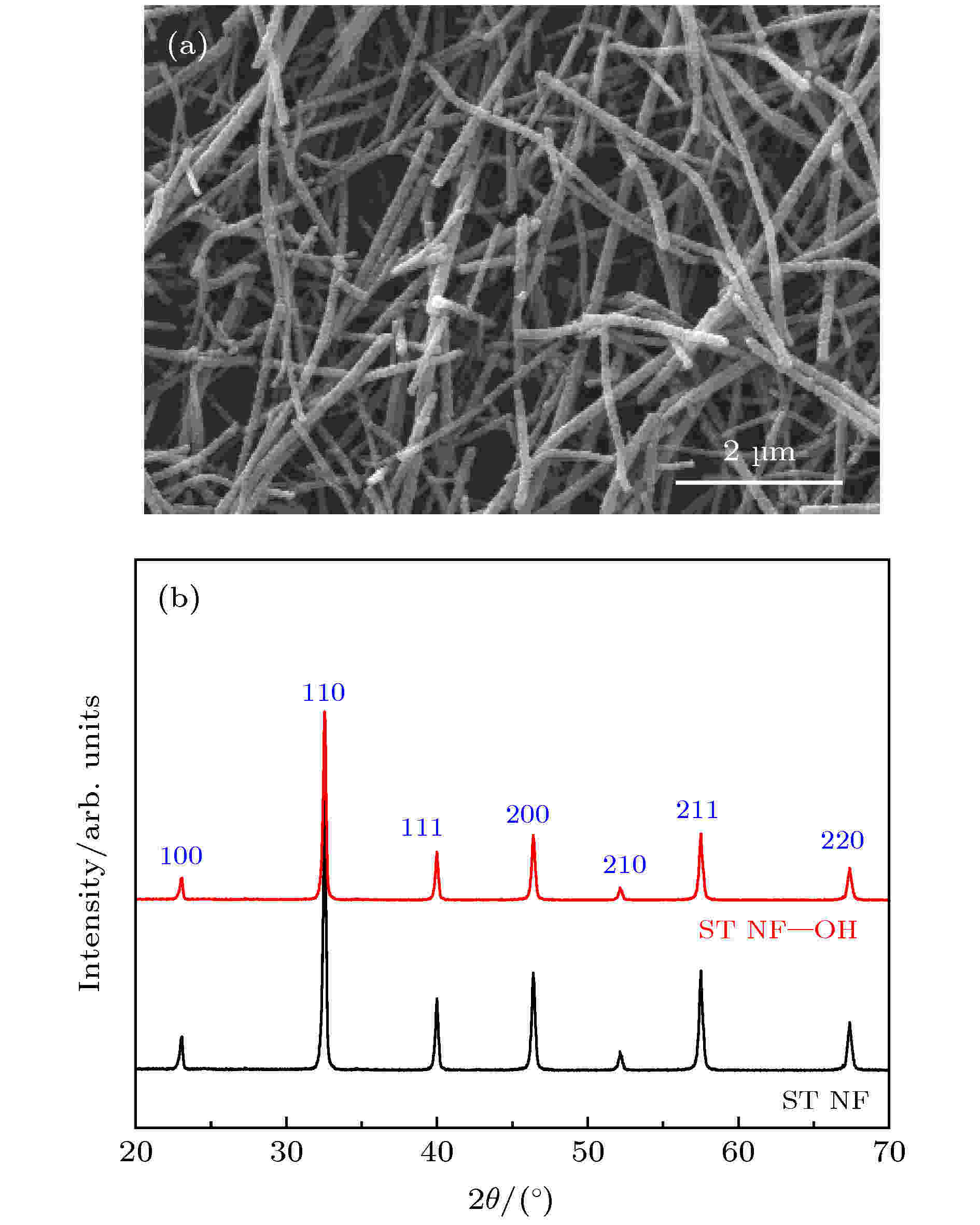
Figure1. (a) SEM image of ST NF; (b) XRD patterns of ST NF and ST NF—OH.
样品 | PDF卡片编号 | 晶相 | 空间群 | 晶格参数/nm |
ST NF | 35-0734 | 立方相 | Pm- | a = b = c = 0.3911 |
ST NF—OH | 35-0734 | 立方相 | Pm-3m | a = b = c = 0.3915 |
表1羟基化处理前后样品的晶体结构
Table1.Crystal structure of the samples before and after hydroxylation.
图2为羟基化表面改性前后的ST NF粉体的FT-IR谱. 从图2可以明显看到: 其中出现在556 cm–1处的峰应的是钛酸锶钡中Ti—O键的振动产生的吸收峰, 1050 cm–1处的峰对应的是钛酸锶钡中C—O键的伸缩振动产生的吸收峰, 1631 cm–1处的峰对应的是

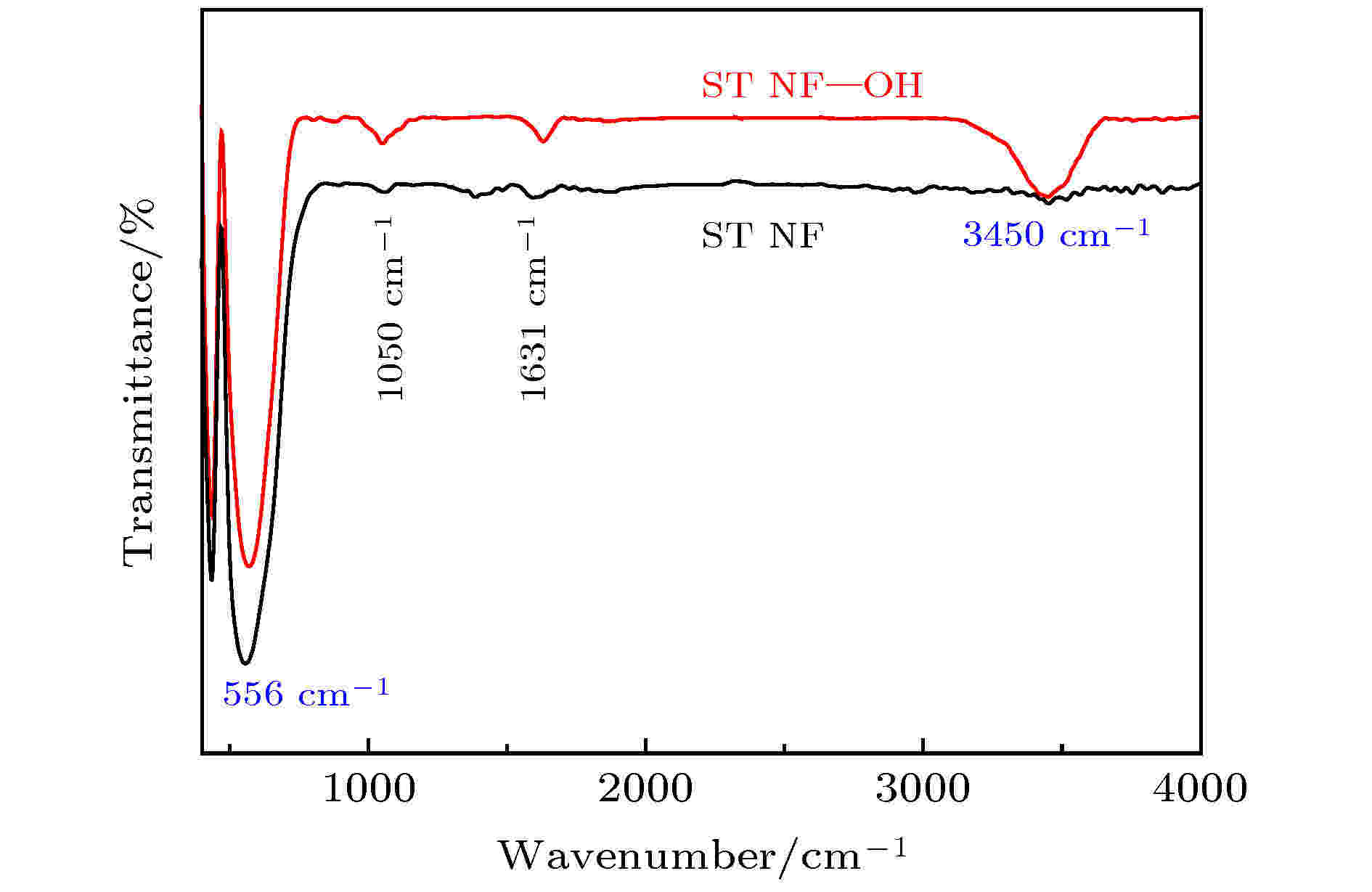
Figure2. FTIR of ST NF and ST NF—OH.
表面羟基化处理前后的ST NF的XPS全谱扫描图见图3, 可以明显看到: 表面羟基化处理前后的ST NF样品中, 均在269 eV (Sr 3p), 458 eV (Ti 2p), 531 eV (O 1s)和285 eV (C 1s)处出现了特征峰, 这些特征峰分别对应于, Sr, Ti, O元素, 此结果对应于文献[4]中的报道, 而位于285 eV附近的C 1s谱峰可能来自用C元素进行仪器矫正时, 少量的C元素残留在仪器表面所致. 插图为表面羟基化处理后的ST NF的O 1s元素的精细扫描谱线, 对O 1s元素进行了分峰拟合. 结果表明, 电子结合能在529.3 eV处附近特征峰对应于SrTiO3中O原子的峰, 电子结合能在531.6 eV的特征峰峰对应于—OH基团中O的峰. 同时通过对拟合得到的SrTiO3中O原子的峰面积和—OH基团中O的峰面积进行换算处理, 可以得到羟基化处理前后ST NF表面羟基化程度相对含量: 羟基化处理之前, ST NF表面的羟基化程度仅为3.7%, 而羟基化处理之后ST NF—OH表面的羟基化程度为14.5%; 羟基化处理之后ST NF—OH表面的羟基化程度远大于处理之前的结果. 以上结果均表明: 羟基化处理后, —OH基团被引入到ST NF的表面[15].
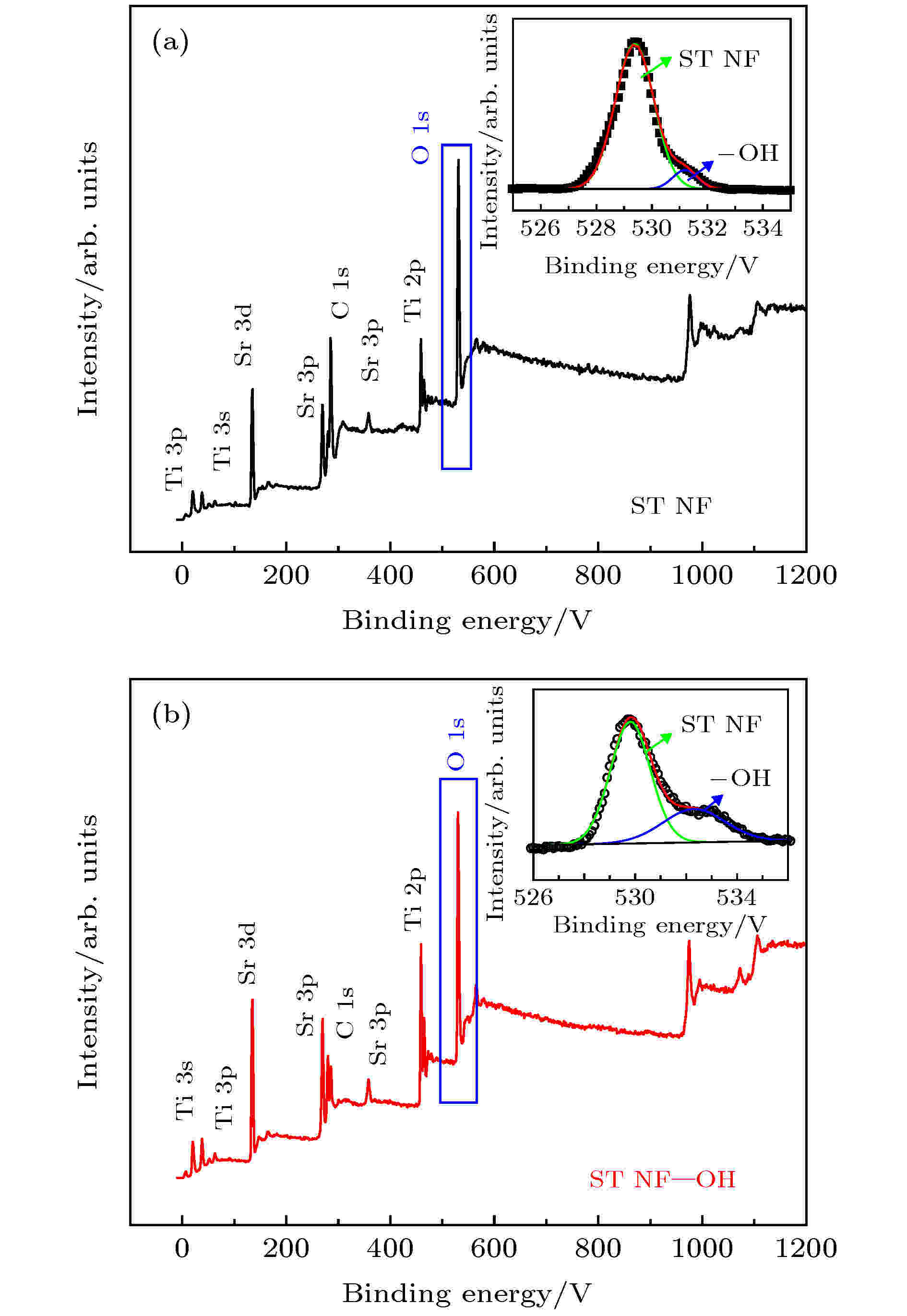
Figure3. (a) XPS spectra of ST NF; (b) XPS spectra of ST NF—OH. High-resolution XPS spectra of O 1 s are shown in the inset.
图4给出了羟基化处理前后ST NF的SEM图, 处理前后ST NF的形貌变化较小, 处理前后ST NF均具有完整的纤维状形貌. 纤维的直径为100—150 nm, 长度为2—10 μm, 均具有较大的长径比.
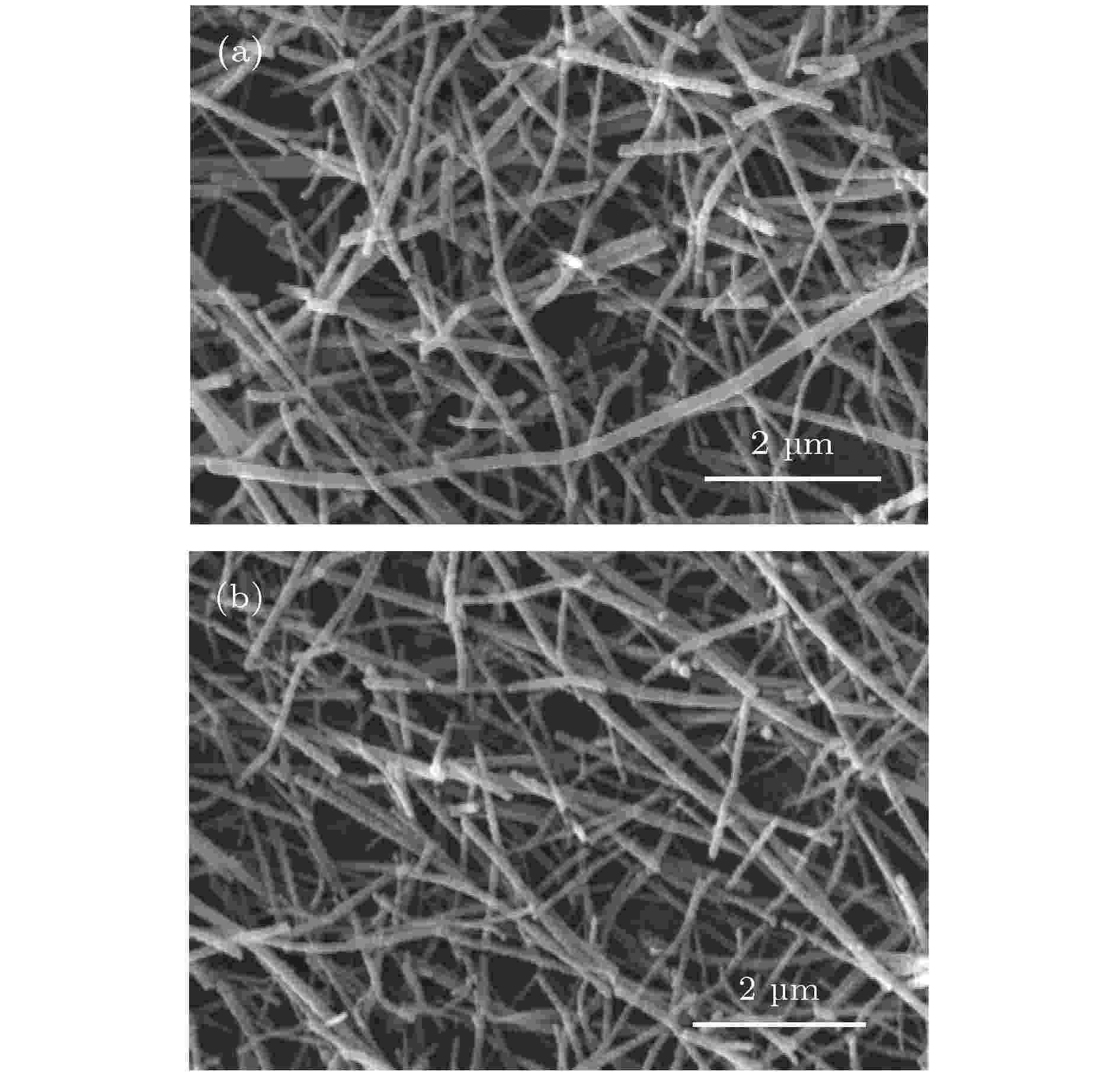
Figure4. (a) SEM image of ST NF before hydroxylation; (b) SEM image of ST NF—OH.
填充量为5% (体积分数)的ST NF—OH/PVDF复合材料表面SEM图和截面的SEM图见图5. 从图5(a)可知, ST NF—OH填料具有良好的一维形貌, ST NF—OH填料均匀分散在PVDF基体中, 没有明显的团聚现象. 另外, 从复合材料的截面图(图5(b))可以看出, ST NF—OH填料沿着平行于复合材料表面的方向均匀分散在PVDF基体中, 基本没有气孔、微裂纹等结构缺陷的出现. 这是因为羟基自身具有较强的电负性, 而PVDF中的F键自身也具有较强的电负性, 羟基与F键两者可以相互作用形成氢键(F—HO), 羟基化处理后ST NF与PVDF形成氢键的示意图见图6, 氢键的形成可以改善ST NF在聚合物基体中的分散、增强ST NF与聚合物基体之间的界面结合性能. 以上结果表明ST NF—OH在PVDF基体中有很好的分散性和兼容性.
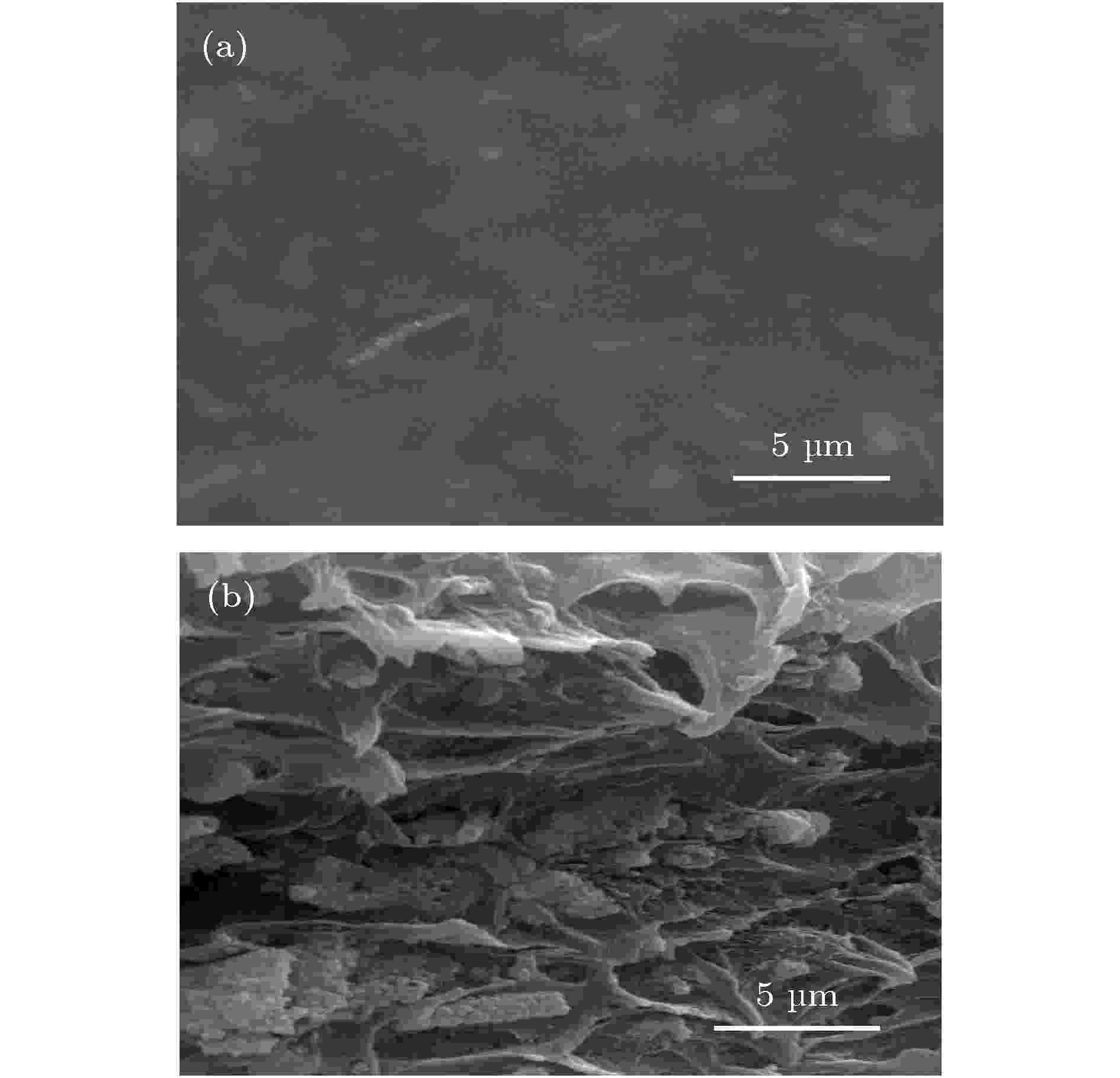
Figure5. (a) Surface SEM and (b) cross-section SEM of 5% (volume fraction) ST NF—OH/PVDF composites
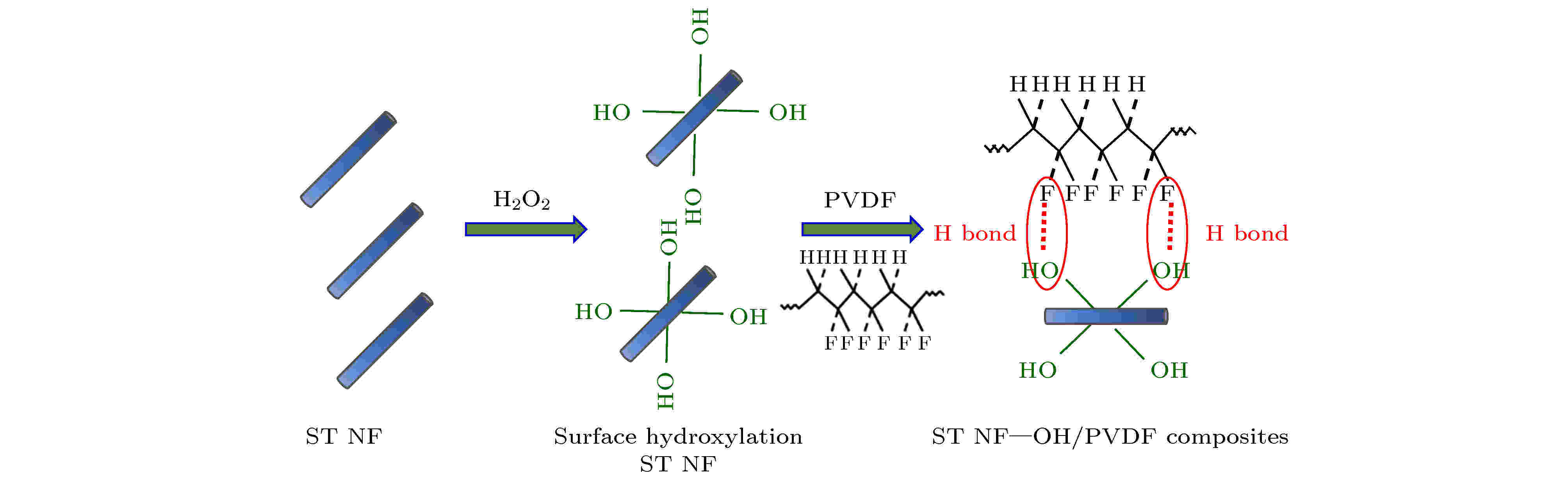
Figure6. Schematic diagrams of formation a bridge between the F atoms on the PVDF and the —OH groups of the hydroxylation of ST NF.
为了研究ST NF—OH填料对复合材料介电性能的影响, 对ST NF—OH/PVDF复合材料室温的介电常数和介电损耗进行了测试, 图7(a)和图7(b)分别为室温条件下不同填充量ST NF—OH/PVDF复合材料的介电常数和介电损耗随频率的变化. 从图7(a)可以看出, 复合材料的介电常数随着ST NF—OH填料的增加而不断增加, 这是因为: 一方面, ST NF—OH填料的介电常数大于PVDF基体, 当填料加入到PVDF基体中时, 介电常数较低的PVDF聚合物基体会承受更大的电场强度, 进而导致PVDF聚合物基体产生更大的极化; 另一方面, 由于填料和基体两相介电常数的差异较大, 造成复合材料两相界面处出现Maxwell-Wager-Sillars (MWS)界面极化, 且随着ST NF—OH填料的增多, 会出现更多的两相界面, 造成了MWS界面极化的增强[22-24], 这两方面的因素导致复合材料介电常数随着填料的增加而增加. 同时从图7(a)可以看出纯PVDF的介电常数低(7.9在1 kHz). 复合材料的介电常数随着ST NF—OH填料含量的增高而不断增加, 这意味着可以通过改变ST NF—OH填料的含量对复合材料的介电常数进行调节. 当ST NF—OH填充量为7.5% (体积分数)时, 复合材料的介电常数达到13.8, 这是纯PVDF介电常数的1.75倍. 而选用钛酸钡纳米粉体填料, 填充量为10% (体积分数)时, 复合材料的介电常数为纯PVDF介电常数的1.60倍[25], 以上结果表明相比零维的纳米粒子, 一维纤维状填料可以在较低填充量下对复合材料的介电常数实现较大的提高. 采用Maxwell-Garnett模型对其进行拟合[26], 图8为不同填充量ST NF—OH/PVDF复合材料的室温1 kHz介电常数测量值和数值模拟.
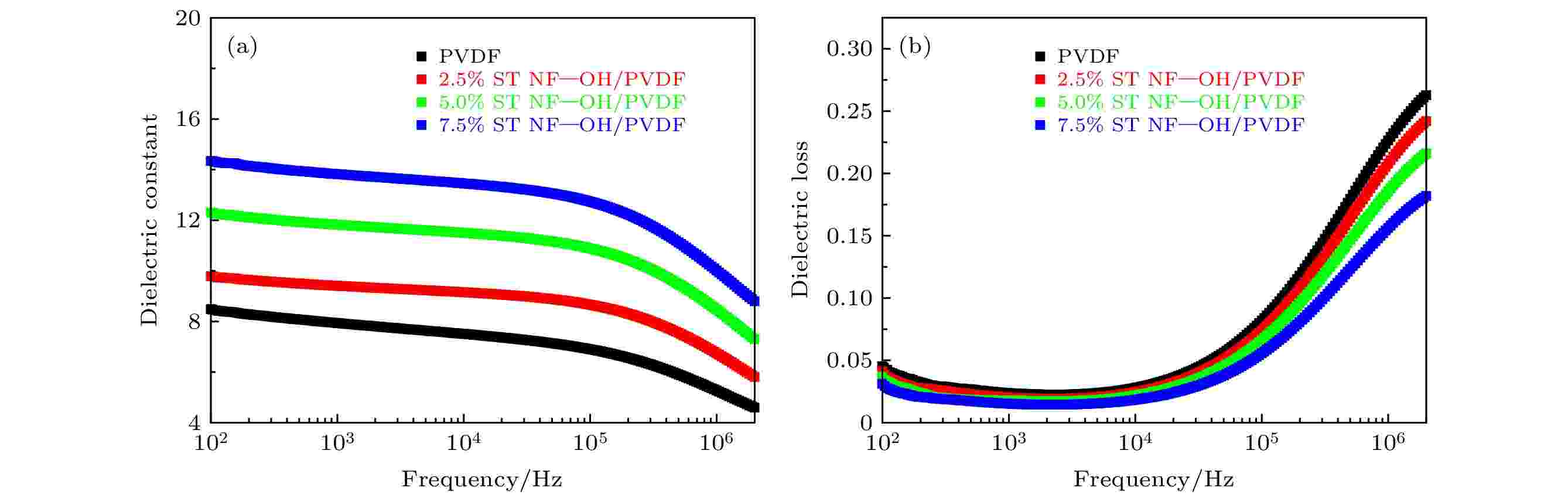
Figure7. Frequency dependence of the dielectric constant (a) and loss tangent (b) of ST NF—OH/PVDF composites with various concentrations (volume fraction) of filler.
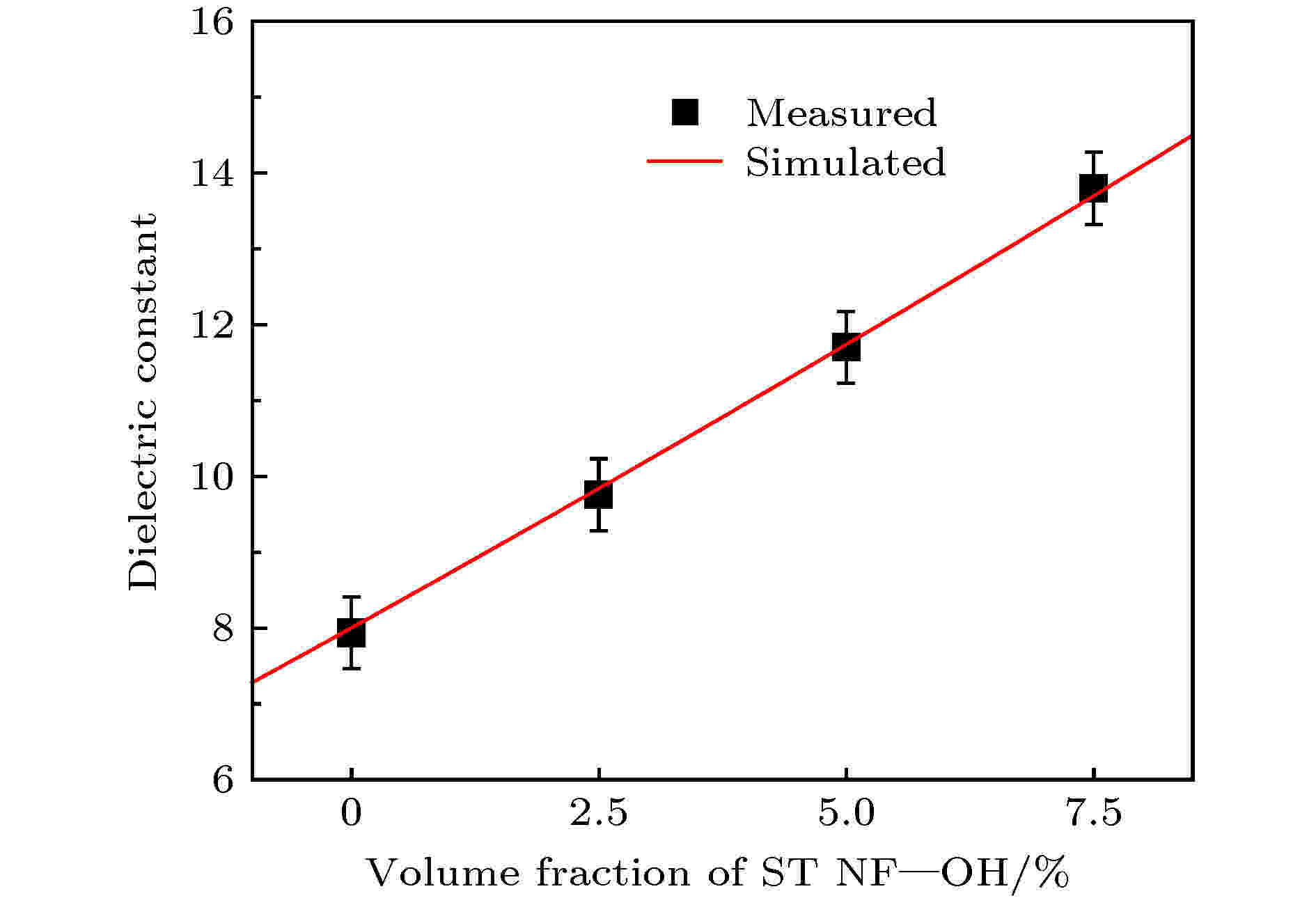
Figure8. Dielectric constant measurement and numerical simulation of ST NF—OH/PVDF composites with different loading.






Weibull分布可用来评价样品的可靠性、失效的分布情况, 被广泛用于耐击穿场强实验数据的处理. 由Weibull分布公式P(E) = 1 – exp[–(E/Eb)β]得到复合材料的耐击穿场强, 公式中P(E)为累计击穿的概率; E为实验的击穿场强; Eb为特征击穿场强, 代表累计击穿概率为63.2%时的击穿场强; β为形状因子, 用来表征介质材料的击穿场强分布情况. 图9(a)为不同填充量ST NF—OH/PVDF复合材料的Weibull分布曲线, 形状因子β值越高代表耐击穿场强测试结果的离散性越小, PVDF和填充量(体积分数)分别为2.5%, 5%和7.5%的ST NF—OH/PVDF复合材料对应的形状因子β分别为27.57, 27.57, 20.81和21.06, PVDF与ST NF—OH/PVDF复合材料的形状因子β值均高于20, 且样品的耐击穿测试结果均匀分布在直线两侧, 表明耐击穿强度测试值的分布较窄, 结果可靠. 图9(b)为填充不同量的ST NF—OH时复合材料的耐击穿场强, 可以看出: 复合材料的耐击穿强度会随着ST NF—OH填料含量的增加而降低, 当ST NF—OH填充量为7.5% (体积分数)时, 复合材料的耐击穿场强为2800 kV/cm, 低于纯PVDF的耐击穿场强(4000 kV/cm). 这主要是因为高介电常数ST NF—OH填料加入至较低介电常数的PVDF基体导致两相的介电常数和电导差异较大, 从而导致复合材料的击穿场强低于纯PVDF薄膜, ST NF—OH填料加入到PVDF基体越多, 复合材料中两相的介电常数和电导差异越大, 导致随着填料的增加复合材料的击穿场强减小.
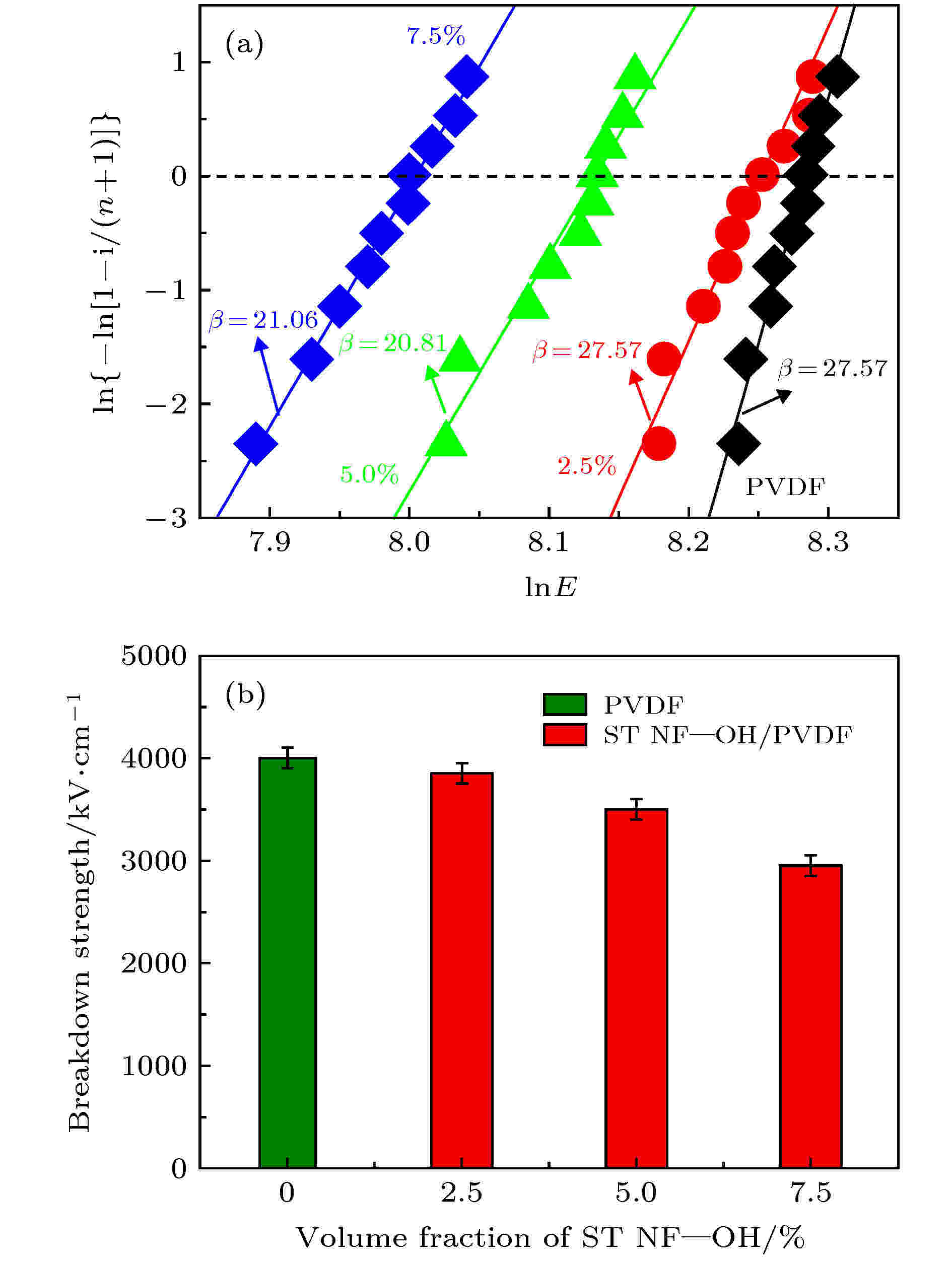
Figure9. (a) Weibull plots and (b) breakdown strength for ST NF—OH/PVDF composites with various concentrations of fillers.
对于铁电体电介质而言, 计算铁电体电介质的储能密度, 对电介质的电滞回线(P-E)进行积分获得. 图10(a)为室温条件下, 频率100 Hz时含不同填充量ST NF—OH的复合材料的P-E曲线. 通过对P-E曲线的积分, 得到了室温条件下不同ST NF—OH填充量ST NF—OH/PVDF复合材料的储能密度随电场变化的曲线, 如图10(b)所示. 可看出: 随着ST NF—OH填料浓度的增加, 复合材料的储能密度呈现出先增加后降低的趋势, 这是因为高储能密度材料需要同时具备较高的介电常数和耐击穿场强, 在较小填充量时, 复合材料具有较高的介电常数, 同时耐击穿场强维持在一个较高的值, 而当填充量较高时, 虽然可以提高复合材料的介电常数, 但复合材料的耐击穿场强却大幅降低. 当ST NF-OH填充量为2.5% (体积分数)时, 在3800 kV/cm的电场下, ST NF—OH/PVDF复合材料的储能密度达到7.96 J/cm3, 这是纯PVDF储能密度的2.79倍. 不同ST NF—OH填充量ST NF—OH/PVDF复合材料的放电效率随电场变化曲线如图10(b)所示. ST NF—OH/PVDF复合材料放电效率随着电场的增加而降低, 这是和复合材料在高电场的损耗有关. 同时随着ST NF—OH填料浓度的增加, 复合材料放电效率呈现出先增加后降低的趋势, 这是因为ST NF—OH和聚合物基体的介电性能差异较大(钛酸锶属于电介质材料具有较高的介电常数, 钛酸锶的室温1 kHz介电常数约为300, 电导率约为5 × 10–4 S/m, 而PVDF属于聚合物, 聚合物介电常数较低, PVDF的室温1 kHz的介电常数为7.9, 电导率约为1 × 10–13 S/m), 导致两相界面处出现空间电荷极化, 在放电过程中这些空间电荷不能完全释放造成的. 然而ST NF—OH填充量为2.5% (体积分数)的复合材料在低于1000 kV/cm时, 放电效率高于85%, 在3800 kV/cm时, 放电效率仍为63%, 均高于纯PVDF的放电效率. 表2列出了前期文献报道PVDF基复合材料的储能密度和本文实验结果的对比, 可以看出, 钛酸锶纳米纤维表面羟基化处理可以提高复合材料的储能性能, 所制备的复合材料具有一定的优势.
材料类型 | 表面改性方式 | 储能 密度/J·cm–3 | 文献 |
BaTiO3/P (VDF-HFP)薄膜 | 氨甲基膦酸 | 3.2 | [27] |
SrTiO3/PVDF 薄膜 | 聚乙烯吡咯烷酮 | 3.54 | [25] |
BaTiO3/ P(VDF-HFP) 复合薄膜 | — | 4.89 | [28] |
Ba0.7Sr0.3TiO3/P (VDF-CTFE)材料 | KH-550偶联剂 | 6.5 | [29] |
SrTiO3/PVDF薄膜 | 表面羟基化 | 7.96 | 本文 |
表2前期文献报道的PVDF基复合材料的储能密度与本文实验结果比较
Table2.Comparison of the energy storage density of PVDF-based composite materials reported in previous literatures and the experimental results in this paper.
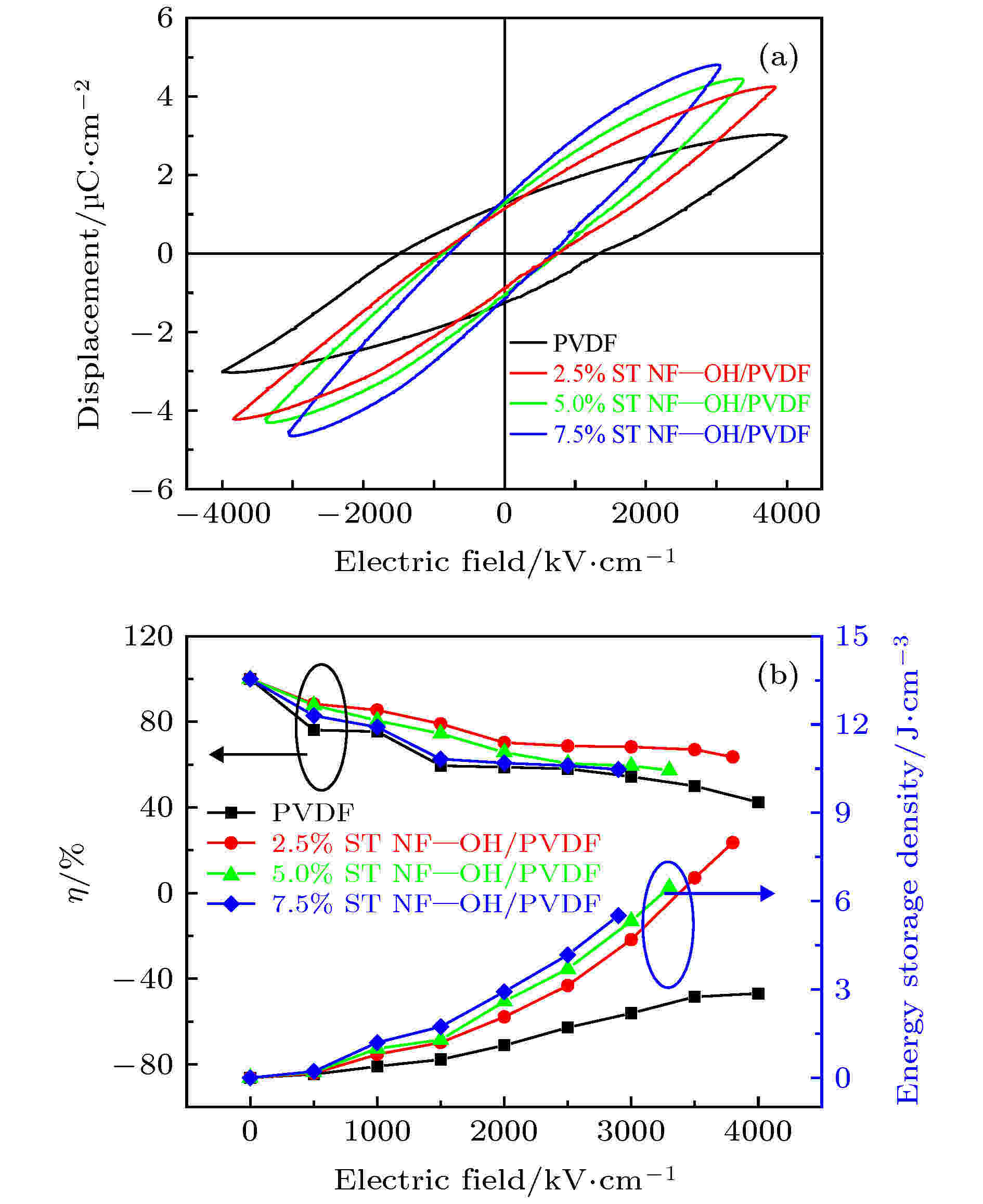
Figure10. (a) P-E curves and (b) the efficiency and energy storage density of ST NF—OH/PVDF composites with various concentration (volume fration) fillers.
为了比较ST NF羟基化表面改性前后对复合材料储能性能的影响, 对羟基化处理前后复合材料的储能进行了测试, 结果发现: ST NF填充量为2.5% (体积分数)复合材料的的耐击穿场强仅为2300 kV/cm, 储能密度为3.73 J/cm3, 而羟基处理后, ST NF—OH填充量为2.5% (体积分数)时, 复合材料的耐击穿场强为3850 kV/cm, 储能密度为7.96 J/cm3, 为ST NF/PVDF复合材料密度的2.13倍. 相比ST NF/PVDF复合材料, ST NF—OH/PVDF复合材料表现出更高的储能密度, 这是因为表面羟基化处理后, 填料表面羟基与F键两者可以相互作用形成氢键, 可以改善填料在聚合中的分散、结合情况, 从而提高复合材料的耐击穿场强和储能性能.