全文HTML
--> --> -->
材料经受辐照后的微结构和力学性能变化已经受到了广泛关注. Shao等[7]和Zhou等[8]应用分子动力学分别模拟了含He泡、孔洞单晶铝材料在方波和衰减波加载下的层裂行为; Lin等[9]和Qiu等[10]研究了空位型缺陷对单晶铜、镍层裂行为的影响, 发现空位的存在会明显降低材料的层裂强度, 且其影响程度与材料晶体取向有关. 辐照产生的SFT对材料的力学性能的影响也进行了大量的实验和理论研究. 大量实验表明, 辐照后产生的SFT会使材料出现硬化、脆化和屈服后软化的现象. Dai和Victoria[11]对辐照后的铜进行了拉伸试验, 观察到材料内部形成了明显的无缺陷通道, 导致材料发生软化. Edwards等[12]对铜及其合金材料进行了一系列辐照实验, 发现随着辐照剂量的增大, 材料屈服应力逐渐增大, 韧性降低, 应变硬化系数逐渐减小. 在理论研究方面, 大量工作主要关注SFT与位错[13-21]、晶界[22-25]等缺陷的相互作用, 研究表明, SFT能够阻碍位错的滑移并导致材料屈服应力升高、韧性降低. 运用分子动力学方法, Osetsky等[15]和Fan等[17]模拟了刃型位错、螺型位错及混合位错与SFT之间的相互作用, 发现SFT可能发生剪切变形、湮灭、旋转或转化为其他缺陷. Wu等[24]模拟了不同尺寸的SFT在含孪晶边界和晶界的Al, Ni, Cu, Ag等金属中的稳定性. Arsenlis和Wirth[19]以及Krishna等[20]采用标量形式描述SFT缺陷, 分别发展了基于连续位错密度和基于微观率无关理论的晶体塑性模型; Xiao等[21]进一步采用张量形式描述SFT缺陷, 对SFT与位错之间的空间作用规律进行了细致刻画. 此外, 还有部分研究关注了SFT对不含初始位错的面心立方晶体材料的力学性能的影响[26-29]. 研究表明, 相比于完美晶体, SFT的存在使得材料的位错成核应力降低了一半[26]. 在不同方向的载荷作用下, SFT的结构会发生不同的转变, 并对材料的压缩、拉伸过程造成不同程度的影响[29].
总体来说, 目前对SFT的研究主要针对的是准静态或常应变率加载条件, 而对于冲击加载条件下SFT对材料动态力学性能的影响, 研究关注较少. 层裂是延性金属材料在冲击加载下的一种典型破坏形式, 深入研究SFT对材料层裂行为的影响具有重要意义. 为了进一步探究SFT在冲击加载条件下对材料层裂行为的影响及其内在机理, 本文采用分子动力学模拟方法对含有一定密度SFT的单晶铜的层裂行为进行模拟研究, 重点关注不同冲击速度下材料中SFT的微结构演化及其对层裂强度、损伤演化的影响.


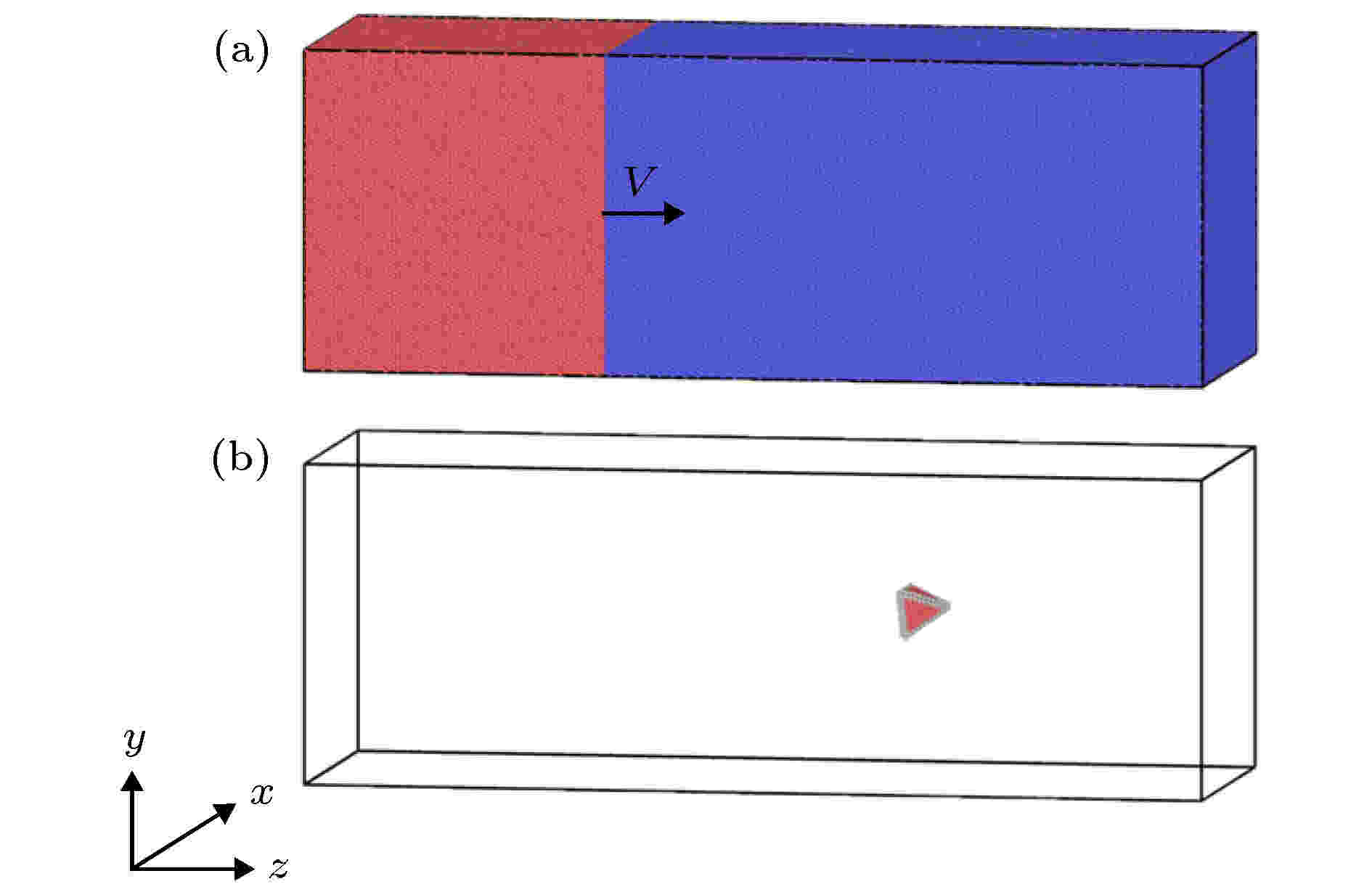
Figure1. Initial configuration of the simulation system: (a) The equilibrated atomic configuration, and the arrow denotes the direction of impact; (b) the internal distribution of SFT.
本文采用Silcox-Hirsch机制[31]产生SFT, 即通过三角形空位层演化形成SFT. 首先在模型中心[1 1 1]面上移除一个包含55个原子的正三角形原子层, 形成边长约为2.5 nm的三角形空位盘, 随后对模型施加三维周期性边界条件, 采用NPT系综将模型在300 K, 0 bar (1 bar = 105 Pa)条件下弛豫100 ps, 使其产生SFT并达到平衡状态. 图2为SFT形成过程微观图像, 历时约1 ps, 最终在模型中部形成棱长约为2.5 nm的SFT结构. SFT在模型中的数密度约为1.3 × 1023 m–3, 这与金属铜在辐照实验中产生的SFT大小和密度[5,6]基本一致.
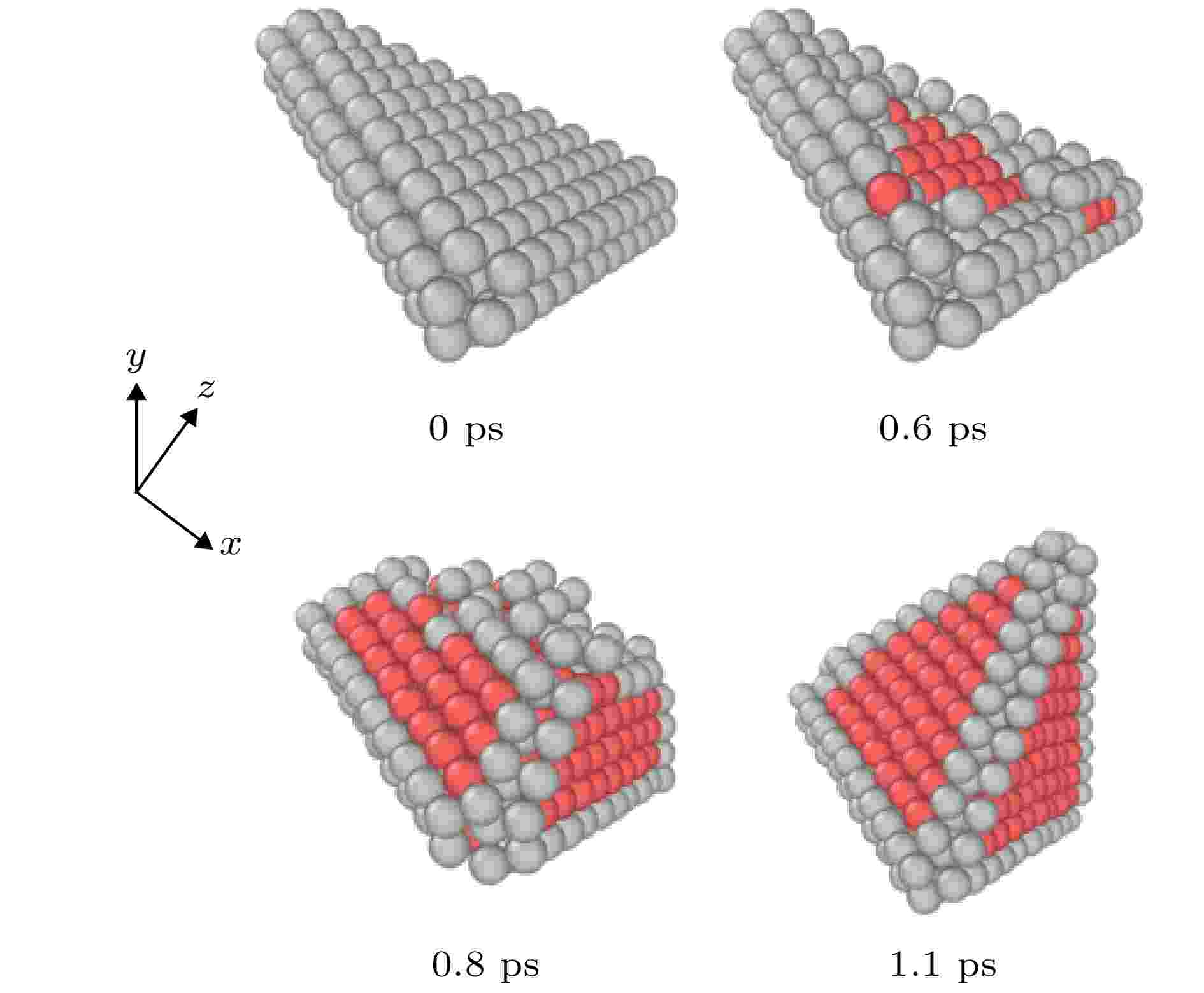
Figure2. Snapshots of SFT formation.
冲击过程采用NVE系综, x, y方向采用周期性边界条件, 冲击方向z采用自由边界条件, 计算时间步长为0.1 fs. 为了模拟层裂过程, 将模型以1∶2的比例分为飞片和靶板两部分. 飞片(图1(a)中红色部分原子)在[1 1 1]方向上以一定的初速度2Up (Up为冲击压缩过程中靶板的粒子速度)撞击右侧靶板, 因此冲击脉冲分别在飞片和靶板内部产生. 冲击波到达飞片和靶板的自由表面后反射回两束相向而行的稀疏波, 两稀疏波在靶板中部相遇形成拉伸脉冲, 如果拉伸应力脉冲足够强, 经过一定的时间后, 靶板就会发生层裂. 为研究不同冲击速度下SFT的演化及其对层裂行为的影响, 本文模拟了Up为0.5—1.25 km/s时材料的层裂行为.
本文统计了应力、温度等物理量沿冲击方向(z方向)的分布, 分析所用应力为维里应力[32]. 使用可视化软件ovito[33]对模拟结果进行分析处理, 其中使用共近邻分析法(common neighbor analysis, CNA)[34]来分析模拟过程中的微结构变化, 使用位错抽取算法(dislocation extraction algorithm, DXA)[35]来分析位错演化情况.
3.1.层裂强度
层裂强度是表示材料抵抗断裂破坏能力的重要参数, 在分子动力学模拟中, 通常用样品层裂过程中的最大拉伸应力(–σ33,max)作为层裂强度[9]. 不同冲击速度下含SFT铜与完美单晶铜的层裂强度如图3所示. 作为对比, 图3还给出了Luo等[36]针对完美单晶铜的分子动力学模拟所得部分结果.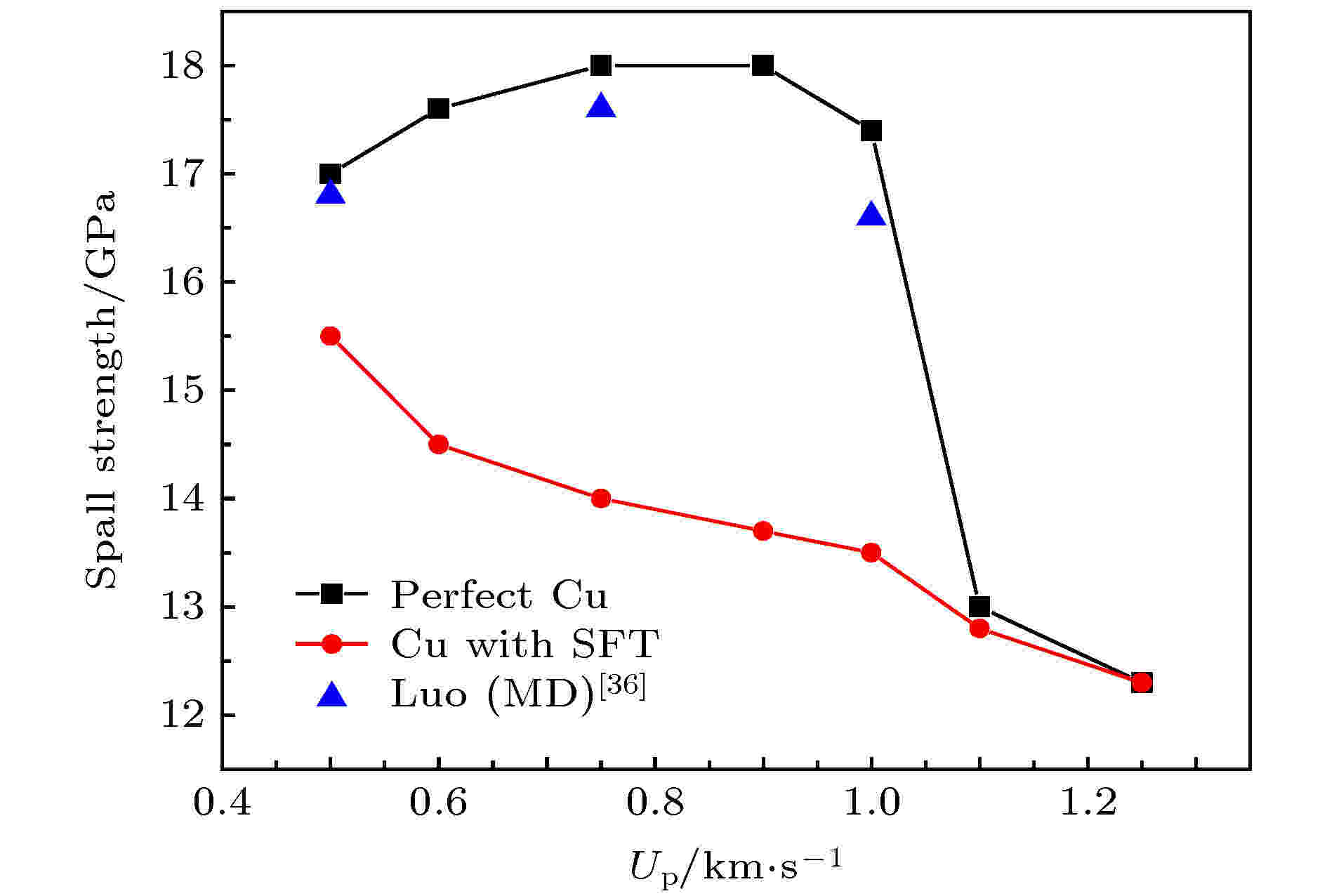
Figure3. Relationship between particle velocity Up and spall strength of perfect Cu and Cu with SFT.
从图3可以看出, 随着Up的增大, 完美单晶铜的层裂强度呈现先增大后减小的趋势, 这与Luo等[36]的分子动力学模拟所得结果是一致的. Up ≤ 1.0 km/s时, 在同等加载条件下, 含有SFT铜的层裂强度比完美单晶铜的要小得多, 且两者之间的差值随着Up的增大先增大后减小. Up为0.5 km/s时, 含SFT铜的层裂强度为15.5 GPa, 比相同加载条件下的完美单晶铜(17 GPa)低1.5 GPa; Up为0.75 km/s时, 含SFT铜的层裂强度为14 GPa, 比相同加载条件下的完美单晶铜(18 GPa)低4 GPa; 而当Up达到1.25 km/s时, 两者的层裂强度均降低为12.5 GPa. 可见, 中低速度(Up ≤ 1.0 km/s)加载下, SFT的存在降低了材料的层裂强度, 且其影响在中等速度冲击时最为显著. 当Up达到1.25 km/s及以上时, 两者的层裂强度基本相同, SFT的存在对材料层裂强度不再有影响. 下面对不同速度冲击下材料内部的微结构演化及应力温度分布情况进行详细分析.
2
3.2.层裂过程
为了进一步深入分析层裂过程中材料内部微结构的演化情况, 采用CNA[34]和DXA[35]来分析微结构的演化. 图4给出了Up分别为0.5和0.75 km/s时, SFT在压缩过程中的演化形态图及相应的位错演化图. SFT在冲击波作用下会发生坍塌, 0.5 km/s时(见图4(a)), 当冲击波扫过SFT时, SFT从顶部开始坍塌(2.67 ps), 形成Shockley不完全位错, 随着Shockley不完全位错在(








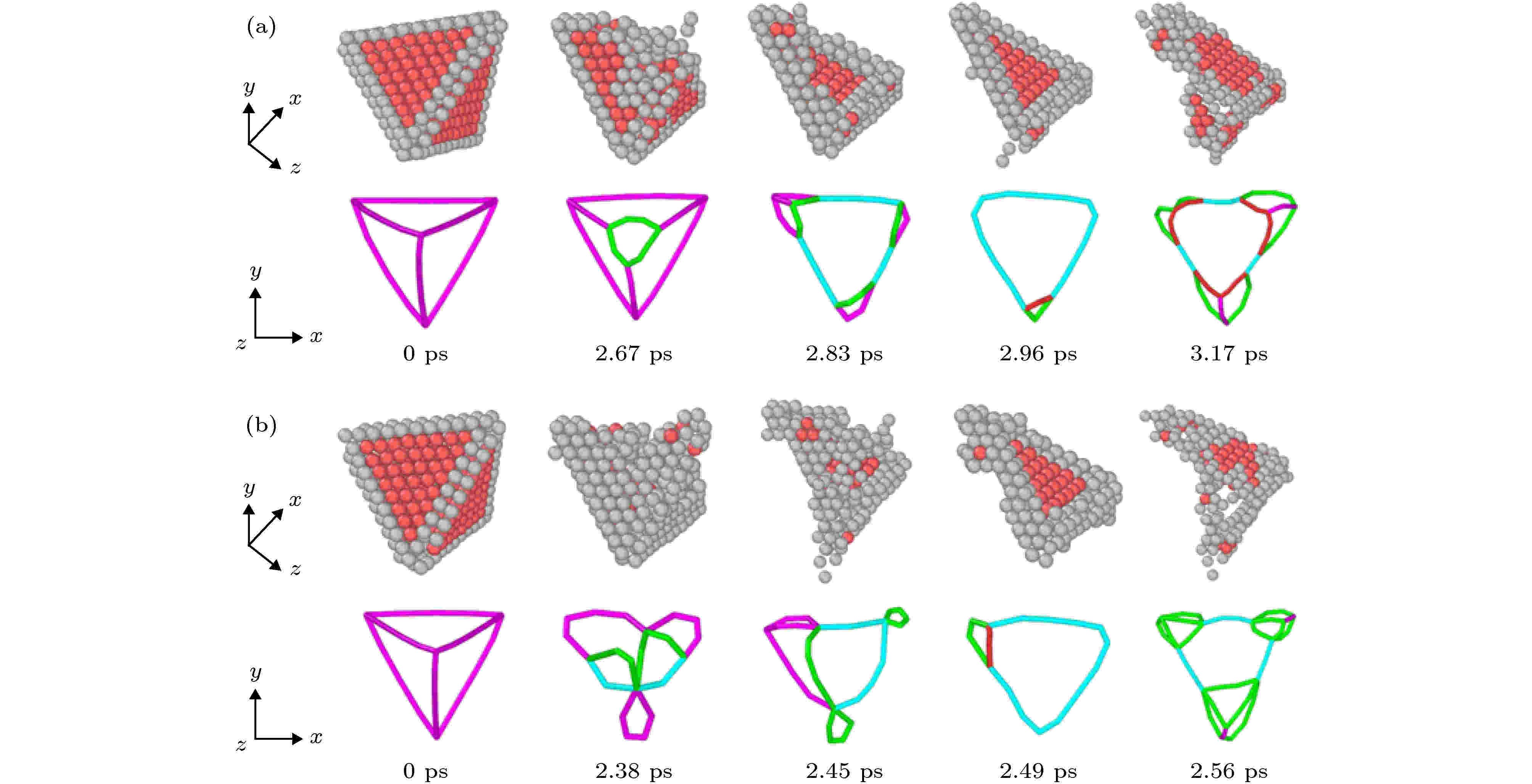
Figure4. Snapshots of SFT configuration and dislocation evolution at different deformation stages during shock compression: (a) Up = 0.5 km/s; (b) Up = 0.75 km/s. The rose red line represents the stair-rod dislocation, the green line represents the Shockley partial dislocation, the light blue line represents the Frank partial dislocation, and the red line is the undefined dislocation.
图5给出了Up分别为0.75和1.25 km/s时材料在压缩和拉伸过程中内部微结构的演化规律, 其中, 绿色、红色和蓝色原子分别表示面心立方、堆垛层错和其他缺陷原子. 速度为0.75 km/s的情况下(图5(a)), 对于完美单晶铜, 冲击波在4.6 ps时到达右侧自由表面, 在冲击压缩过程中材料只发生弹性变形, 材料内部没有形成位错、层错等缺陷. 随后, 从两端自由面反射回的稀疏波在靶板中部相遇, 形成拉伸脉冲, 在拉伸应力的作用下, 靶板中部开始出现孔洞形核(8.7 ps), 随后孔洞成长、合并, 最终导致材料断裂(16 ps). 对于含SFT铜, SFT的存在降低了材料的屈服强度, 材料在压缩过程中便发生塑性变形, SFT坍塌并进一步形成位错、堆垛层错等缺陷(4.6 ps); 这些缺陷结构随后在拉伸应力作用下进一步演化, 形成了较宽的孔洞形核区(8.7 ps). 观察拉伸过程中层裂图像可知, 完美单晶铜在16 ps时已接近完全断裂状态, 断裂面较光滑平整, 而含SFT铜还未完全断裂, 这与含SFT铜的层裂区较宽、孔洞演化较慢有关. 当速度达到1.25 km/s时(图5(b)), 两种样品的压缩拉伸微观图像不再有明显区别, 这与在此冲击速度下两种样品层裂强度相同的结论一致. 较大的冲击压力使得材料在冲击压缩阶段就发生塑性变形, 产生大量缺陷原子(4.2 ps), 与强冲击产生的缺陷相比, 由SFT引起的缺陷不再具有显著影响; 在随后的拉伸过程中, 这些缺陷为孔洞提供了形核点, 能够看出, 此时在两种样品内部都形成了较宽的孔洞形核区(11 ps), SFT的存在对材料层裂损伤演化的影响几乎可以忽略不计.
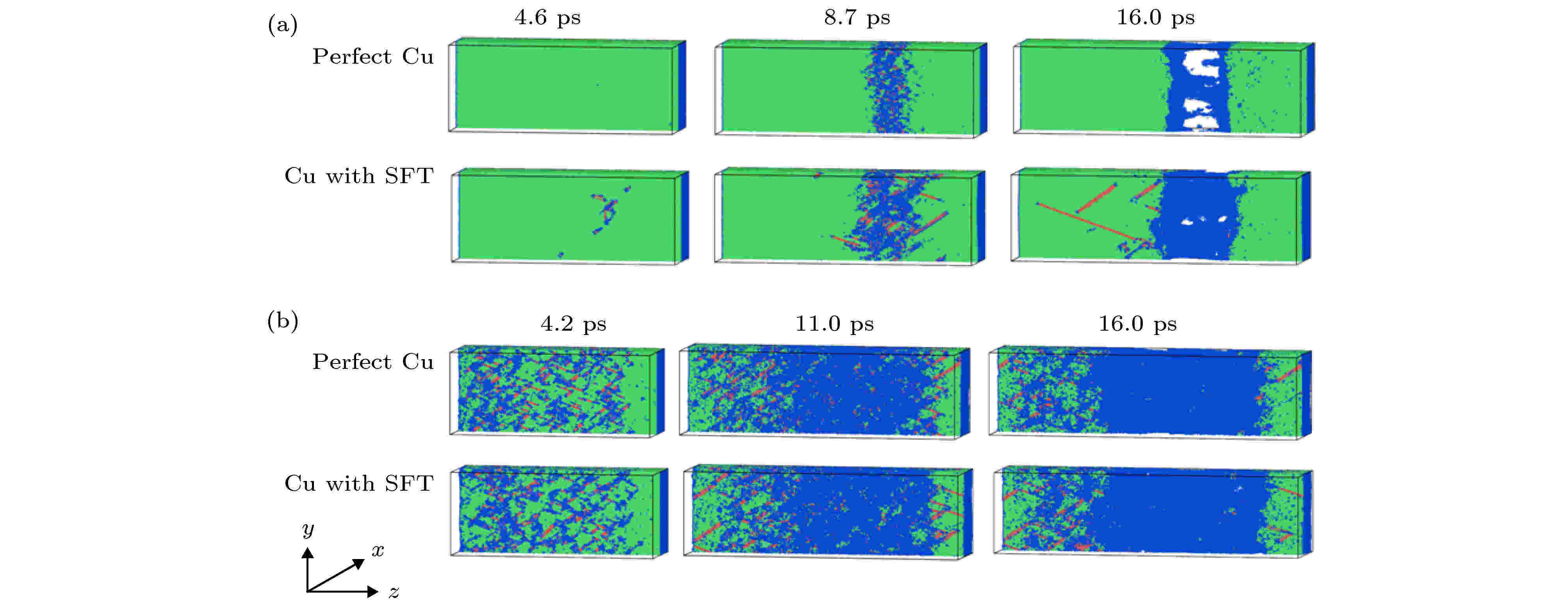
Figure5. Atomic configuration in Cu crystal during shock compression and tension at different impact velocity: (a) Up = 0.75 km/s; (b) Up = 1.25 km/s.
2
3.3.孔洞成核与演化
关于层裂初期样品内部的孔洞演化过程, 图6给出了Up为0.75 km/s时不同时刻材料内部的微结构切片图像及相关位错演化图, 其中绿色、红色和蓝色原子分别表示面心立方、堆垛层错和其他缺陷原子. 在8.0 ps时, 完美单晶铜在拉伸应力作用下开始形成位错, 并伴有缺陷原子出现; 随后位错进一步增殖, 在8.7 ps时, 孔洞开始形核, 可以看出, 初期孔洞均匀集中分布在样品中部一个较窄的区域内(9.5 ps). 随后, 位错继续向两侧扩展并伴随堆垛层错产生, 孔洞在局部区域内快速成长合并(10.5 ps), 造成材料的快速断裂失效(11 ps). 而对于含SFT铜, 在8.0 ps时样品中已分布有大量位错、层错等缺陷, 这是前期压缩过程中的缺陷演化导致的, 这些缺陷在拉伸应力作用下进一步向周围扩展, 形成了更宽的孔洞成核区. 孔洞成核开始于9.5 ps, 较相同速度冲击下的完美单晶铜(8.7 ps)要晚0.8 ps. 此外, 可以观察到, 含SFT铜的孔洞形核区域较完美单晶铜要宽得多, 孔洞的分布更为分散, 大小不均匀, 孔洞演化速度也要比完美单晶铜慢得多. 在11 ps时, 完美单晶铜的孔洞成长合并导致材料接近完全断裂状态, 而含SFT铜内的孔洞仍呈现离散的状态.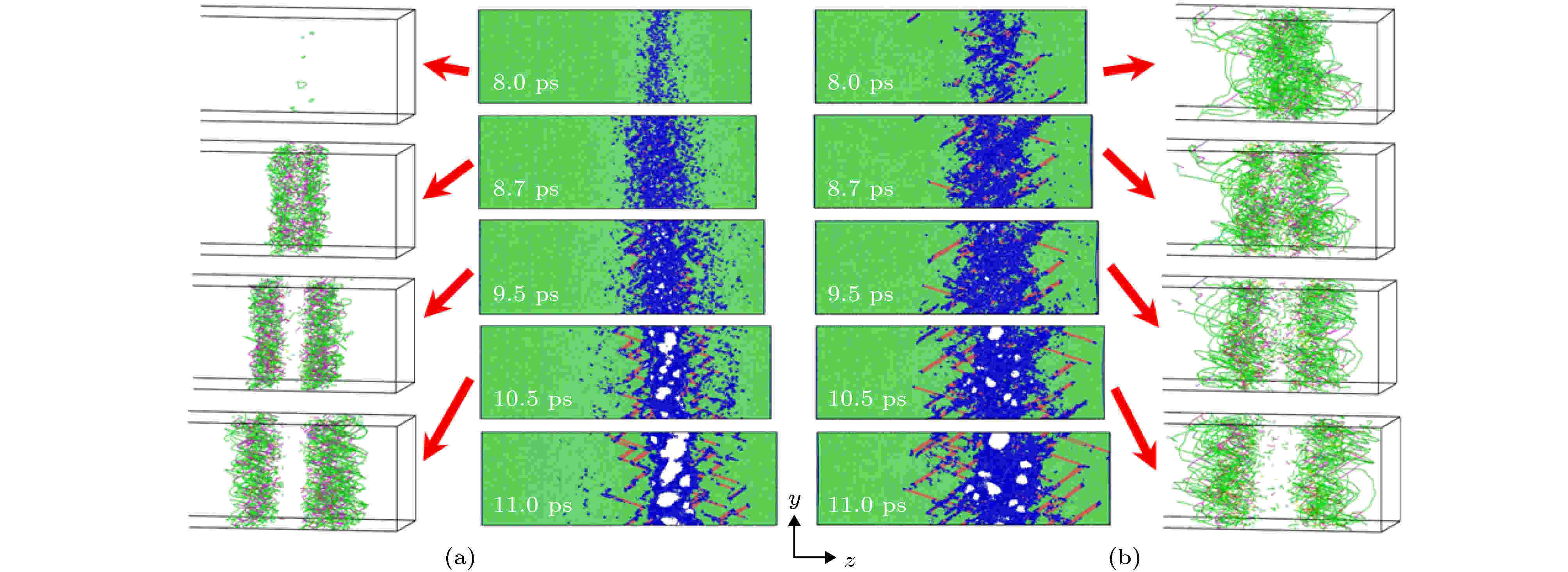
Figure6. Void and dislocation evolution during spallation at Up = 0.75 km/s: (a) Perfect crystal Cu; (b) Cu with SFT.
图7给出了Up为0.75 km/s时孔洞演化过程中对应的应力及温度变化图. 8.0 ps时, 含SFT铜中的拉伸应力较完美单晶铜要小得多, 但温度整体较完美单晶铜要高. 这与含SFT铜在压缩过程发生了塑性变形有关, SFT坍塌导致位错的形核增殖和层错的产生, 一部分冲击能量因此而耗散, 引起温度升高, 拉伸过程中的应力水平也随着缺陷的生成而大大降低. 8.7 ps时, 完美单晶铜的层裂区域开始出现孔洞形核, 此区域应力降低, 温度开始上升; 9.5 ps时, 层裂区域的孔洞进一步增长, 应力大幅度降低, 温度大幅度升高并迅速超过含SFT铜该区域的温度, 这说明完美单晶铜在此过程中的塑性变形较含SFT铜剧烈, 这和完美单晶铜中孔洞演化速度更快是一致的. 在9.5 ps时, 含SFT铜的层裂区也开始出现孔洞的形核和增长, 该区域应力降低, 温度升高. 可以发现, 一旦发生孔洞成核, 含SFT铜的局部峰值温度要远低于完美单晶铜的峰值温度, 而整体温升区域却较完美单晶铜的更宽, 说明含SFT铜形成孔洞的区域更宽, 这与在微观图中观察到的孔洞形核现象是一致的.
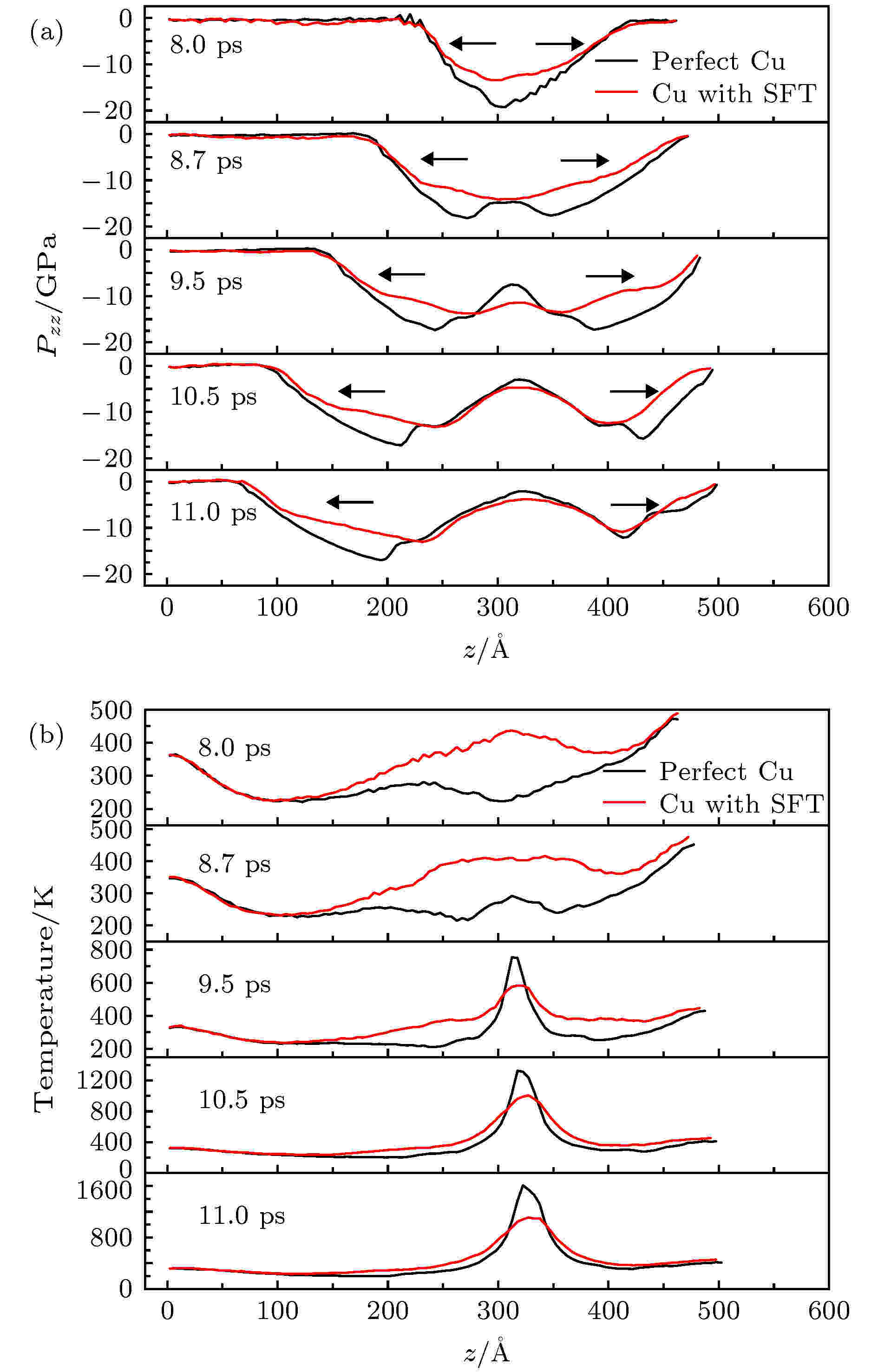
Figure7. Stress and temperature profiles for single crystal copper at Up = 0.75 km/s: (a) Stress; (b) temperature.
3.1节的结果表明: 在中低冲击速度下, SFT的存在显著降低了样品的层裂强度, 3.2节的层裂过程和本节的微结构演化规律为此提供了微观尺度上的解释. 一方面, SFT缺陷的存在降低了材料的屈服强度[29], 使材料在较低的压缩应力下便发生塑性变形, SFT坍塌诱发位错、层错等缺陷的增殖演化, 为孔洞提供了更宽的形核点, 同时塑性变形导致材料区域温度升高, 材料层裂强度下降; 另一方面SFT坍塌及缺陷演化耗散了部分冲击能, 材料在拉伸阶段所受拉应力下降, 孔洞生长动力不足, 使得含SFT材料的损伤演化速率较低, 孔洞生长合并较慢.
2
3.4.自由表面速度曲线
样品自由表面速度曲线能在一定程度上反映材料的层裂损伤过程, 是宏观层裂实验的重要分析内容[37]. 通常若材料发生层裂, 自由表面速度下降到第一个极小值后会重新回跳, 产生Pullback信号. 一般来说, Pullback信号的临界点对应于微孔洞的成核; 速度曲线峰值与第一个极小值之间的幅值(Pullback幅值)可作为预估层裂强度的依据, 幅值越大则层裂强度越大; Pullback信号后的回跳斜率代表了损伤演化速率, 斜率越大则损伤演化速率越大; Pullback信号后的速度回跳幅值在一定程度上反映了层裂区域的损伤程度, 幅值越大则损伤程度越大.图8给出了Up分别为0.75和1.25 km/s时含SFT铜与完美单晶铜的自由表面速度曲线对比图. 在速度为0.75 km/s时(图8(a)), 含有SFT的铜的最大速度已无法维持在平台峰值速度, 说明在冲击压缩过程中由于SFT的坍塌及缺陷演化已经耗散了部分冲击能. 观察二者的Pullback幅值, 含SFT铜的Pullback幅值(0.65 km/s)明显要比完美单晶铜的Pullback幅值(0.85 km/s)小, 即SFT的存在降低了铜材料的层裂强度; 同时含SFT铜产生Pullback信号的时间(t2)明显晚于完美单晶铜(t1), 说明含SFT铜的孔洞成核时间较晚; 比较二者Pullback信号后的回跳斜率和回跳幅值, 发现含SFT铜的回跳斜率和回跳幅值均比完美单晶铜的要小, 说明含SFT铜的损伤演化速率降低, 局部损伤程度也有一定程度的减小, 这与冲击过程中的能量耗散造成的拉伸应力降低有关. 而当速度达到1.25 km/s时(图8(b)), 二者的自由表面速度曲线几乎重合, 此时两种材料的层裂强度、损伤情况等基本一致, SFT的存在对材料的层裂行为不再有明显影响. 以上对自由表面速度的分析与前面在微观结构中所得分析结论是一致的.
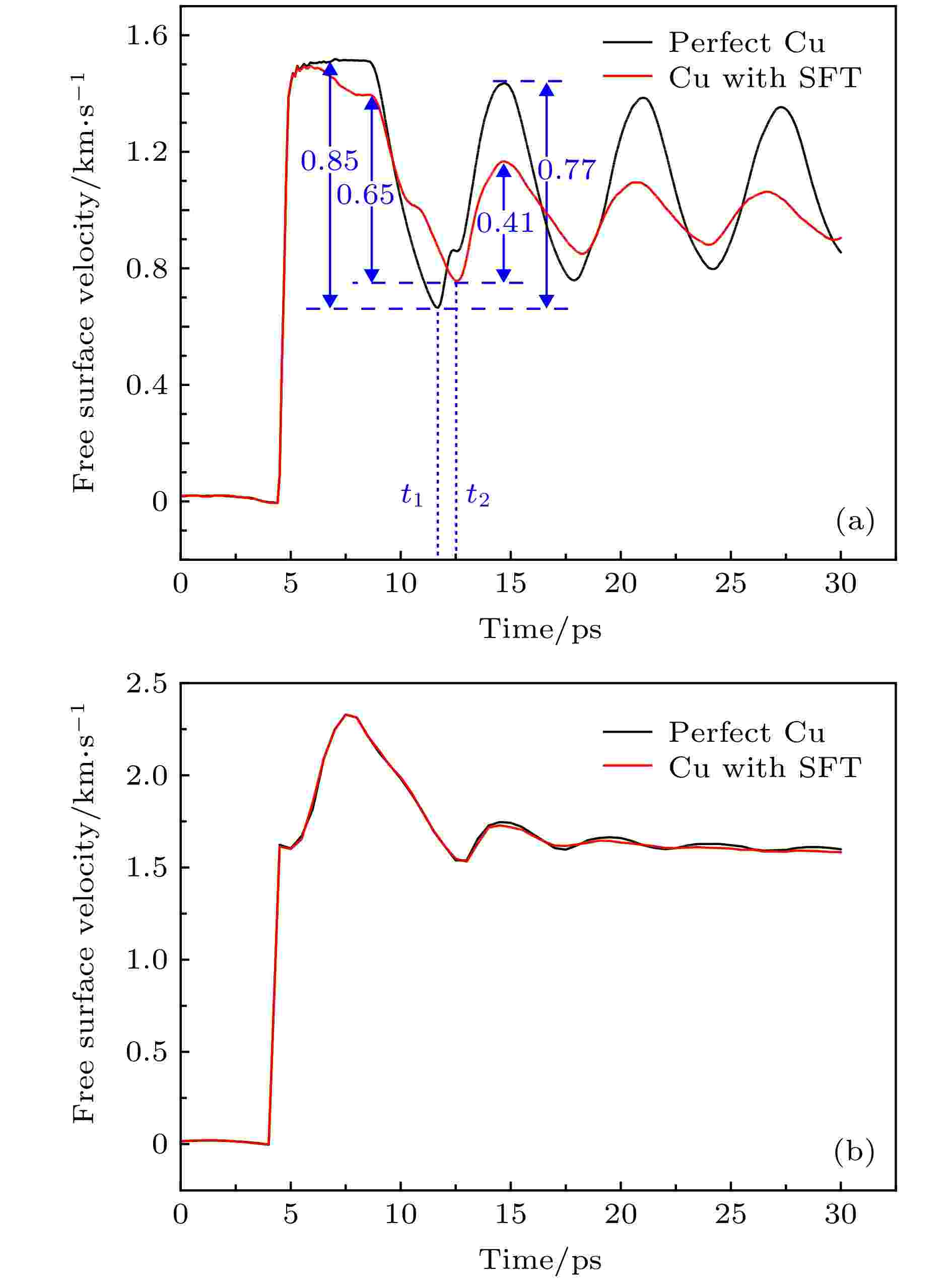
Figure8. Free surface velocity evolution history for single crystal copper at different velocities: (a) Up = 0.75 km/s; (b) Up = 1.25 km/s.