

A method for constructing GLP-1 overexpression intestinal organoids
Zhiyang Zeng

通讯作者: 李大力,博士,研究员,研究方向:基因编辑。E-mail:dlli@bio.ecnu.edu.cn
编委: 林鑫华
收稿日期:2021-03-1修回日期:2021-04-14网络出版日期:2021-07-20
基金资助: |
Received:2021-03-1Revised:2021-04-14Online:2021-07-20
Fund supported: |
作者简介 About authors
曾之扬,博士,博后,研究方向:发育生物学。E-mail:

摘要
胰高血糖素样肽1 (glucagon-like peptide 1, GLP-1)作为一种肠促胰岛素,主要由肠道L细胞分泌,由于其能够有效促进胰岛素的释放从而降低血糖,因此GLP-1及其类似物在2型糖尿病的治疗上具有良好的应用前景。本研究优化了慢病毒感染类器官的方法,利用该方法成功构建了GLP-1过表达的小鼠小肠类器官(organoids)。结果显示该类器官分泌的GLP-1能够有效地提高野生型及糖尿病小鼠的葡萄糖耐受能力。因此,本研究构建的GLP-1过表达类器官可以为2型糖尿病的治疗提供一种新的策略。
关键词:
Abstract
As a potent insulinotrophic hormone, glucagon-like peptide 1 (GLP-1) is mainly secreted by intestinal L cells, which can effectively promote the release of insulin and thus reduce blood glucose. Therefore, GLP-1 and its analogs have a good prospect in the treatment of type 2 diabetes. In this study, we constructed mouse intestinal organoids that overexpress GLP-1 by optimizing the GLP-1 lentivirus infection method. We found that supernatants secreted by the GLP-1 overexpression organoids effectively enhanced glucose tolerance in wild-type and diabetic mouse. Thus, the GLP-1 overexpression organoids built in this study may provide a novel strategy for the treatment of type 2 diabetes.
Keywords:
PDF (808KB)元数据多维度评价相关文章导出EndNote|Ris|Bibtex收藏本文
本文引用格式
曾之扬, 陆佳微, 曹希雅, 王芯悦, 李大力. 一种GLP-1过表达肠类器官构建的方法. 遗传[J], 2021, 43(7): 694-703 doi:10.16288/j.yczz.20-423
Zhiyang Zeng.
胰岛素分泌障碍是2型糖尿病的标志。2型糖尿病治疗策略之一是基于肠道内分泌型L细胞产生的胰高血糖素样肽1 (glucagon-like peptide 1, GLP-1)的降血糖作用,GLP-1具有促进胰岛素的释放,提高胰岛β细胞增殖及存活,促进饱腹感等功能[1,2,3]。GLP-1在机体摄取营养物质时被释放,随后被二肽基肽酶4(dipeptidyl peptidase 4, DPP4)迅速降解,因此其半衰期大约只有1~2 min[4,5]。已有研究表明将人GLP-1与小鼠免疫球蛋白G重链恒定区(IgG1 heavy chain constant regions)结合形成融合蛋白(GLP-1/Fc)可以有效延长其半衰期,提高生物利用率[6,7]。目前GLP-1/Fc融合蛋白已在糖尿病小鼠模型的治疗中被广泛应用。例如通过肌肉注射GLP-1/Fc过表达质粒能够促进胰岛素产生并提高小鼠的糖耐受能力[7]。此外,通过光控诱导HEK293T细胞表达GLP-1/Fc可以有效减轻2型糖尿病小鼠的血糖损伤[8,9]。然而以上的研究均未能实现GLP-1/Fc长期及原位表达,因此通过构建GLP-1/Fc过表达的小肠类器官并将其原位移植到小鼠小肠中可能是一种有效治疗2型糖尿病的方法。
小肠类器官是指将小肠隐窝或干细胞在体外培养形成具有肠干细胞及各种终末分化细胞的类似于花环状的囊状细胞团,因其能在体外模拟体内小肠的自我更新,因此又可以被称为迷你器官[10]。这种迷你器官的培养体系最早是由Sato等[11,12]在2009年构建,除了含有Lgr5+肠干细胞,类器官还包含有Lysozyme+潘氏细胞,Muc2+杯状细胞,Chromogranin A+肠内分泌型细胞等终末分化细胞。最新的研究表明体外培养的人肠及小鼠肠类器官中均含有能表达GLP-1的L细胞[13,14,15,16],这说明肠类器官几乎包含着所有肠上皮的分化细胞类型,因而具有重建器官的潜能。研究人员进一步将体外培养的类器官原位移植到小鼠的肠内后,发现能重新形成基本的隐窝——绒毛结构,并长期稳定存在[17,18,19]。因此,类器官还可以为再生医学提供供体细胞或组织来源。
基于以上背景,本研究建立了一种GLP-1/Fc过表达小鼠小肠类器官构建的方法。利用该方法构建的GLP-1/Fc过表达类器官能够高效表达活性GLP-1/Fc,通过腹腔注射该活性GLP-1/Fc能有效提高野生型及糖尿病小鼠的糖耐受能力。随后本研究初步验证了小肠类器官原位移植的可能性,为2型糖尿病提供一种潜在的治疗方案。
1 材料与方法
1.1 实验材料
实验所用野生型C57BL/6J小鼠均来源于华东师范大学实验动物中心,糖尿病(db/db)小鼠(C57BL/6J背景)购自上海实验动物中心,所有的小鼠均饲养于华东师范大学SPF级实验动物中心。所有涉及小鼠和实验方案的程序均符合上海市实验动物护理评估与认证协会起草的规定,并经华东师范大学实验动物伦理委员会批准。HEK293T购买于美国ATCC公司。1.2 过表达载体的构建
实验所用过表达载体为pLenti-CMV-IRES2- ZsGreen,购于美国Addgene公司。GLP-1/Fc片段(约900 bp)由华东师范大学叶海峰研究员惠赠。设计扩增GLP-1/Fc的PCR引物,并分别在正向引物及反向引物中添加Age I和XbaI酶切位点和相应保护碱基序列。PCR扩增GLP-1/Fc片段后胶回收约900 bp的DNA片段,然后同时用AgeI和XbaI酶分别酶切pIRES2-ZsGreen载体及GLP-1/Fc回收产物,随后再次胶回收酶切过后的载体及PCR片段。接着用T4 DNA连接酶连接酶切过后的载体和片段,在16℃中连接过夜,连接产物转化到感受态细胞中,随后涂板,挑取单克隆,培养过夜后提取质粒,通过一代测序验证得到pLenti-CMV-GLP-1/Fc-IRES2- ZsGreen质粒。慢病毒包装所需pMD2.G及psPAX2质粒均由北京大学魏文胜教授惠赠。1.3 慢病毒的包装、浓缩及滴度测定
培养20皿HEK293T细胞,待细胞长至70%~ 80%密度时进行转染。将pLenti-CMV-GLP-1/Fc- IRES2-ZsGreen、pMD2.G和psPAX2质粒各10 μg与90 μL的PEI充分混匀,DMEM补足到1 mL,涡旋震荡后室温放置20 min,随后均匀的加入到细胞中。转染8 h后更换培养基,次日补充适量培养基并在荧光显微镜下观察细胞状态及转染效率。转染48 h后再次补充适量培养基,72 h后收集上清,3500 r/min离心10 min,上清用0.45 µm细胞滤器过滤,滤液置于4℃冰箱暂时保存。病毒滤液转移至贝克曼离心管中,4℃、25,000 r/min离心3 h。离心过后看到离心管底部出现半透明的病毒颗粒沉淀,弃上清,用1 mL的类器官培养基重悬病毒沉淀得到病毒原液,病毒收集后分装置于-80℃冰箱保存,避免反复冻融。在24孔板中接种10 5个HEK293T细胞,待细胞长至 70%密度时用病毒进行感染。取10 μL病毒原液制备10倍连续梯度稀释的病毒液,然后往每个孔中加入稀释后的病毒液10 μL,即从高往低分别加入1 μL~10 -6μL的病毒,加完稍微混匀,感染48~72 h后,流式细胞仪分析GFP阳性的细胞,选取感染率为1%~10%之间的数据及其对应所加的病毒量,然后根据公式:病毒滴度=103×N×P/V IU/mL (N代表感染时候的细胞量,P代表阳性细胞的百分比,V代表加到每个孔中的病毒量)计算病毒滴度。
1.4 小肠类器官的培养、传代、冻存与复苏
取6~8周大的小鼠小肠约10 cm,纵向剪开,预冷的PBS洗干净,载玻片轻轻地将小肠表面的绒毛刮去,将绒毛去除的小肠置于50 mL离心管中,放在冰盒中短暂保存。将冰盒转移至安全柜中,用含抗生素的PBS清洗小肠5~8次,将小肠转移至25 mL含 2.5 mmol/L的EDTA溶液中,4℃摇床中消化20 min。弃EDTA溶液,将小肠转移至新的50 mL离心管中,加25 mL含抗生素的PBS,轻晃20~30次,悬液用70 μm的细胞过滤器过滤,重复上述步骤,将两次过滤后的悬液转移至同一个50 mL离心管中,4℃、800 r/min离心5 min。弃上清,用2 mL的基础培养基重悬沉淀,计数。取一定体积的悬液(2000~2500个隐窝)加入到1.5 mL的离心管中,室温900 r/min离心5 min。用事先在冰上预冷的200 μL基质胶(matrigel, BD)重悬沉淀,隐窝的密度控制在10~15个/μL。接板之前,将96孔板事先在培养箱中预热30 min,随后每个孔接种5 μL的隐窝-基质胶混合液,若为24孔板则每孔接种50 μL。将接种好的板于37℃培养箱中放置25~30 min让基质胶凝固。最后,每孔加100 μL培养基(24孔板加500 μL),每2~3 d更换一次培养基,5~7 d传代一次(注:所有的试剂均需添加抗生素以避免染菌,隐窝需尽可能置于冰上以保持活性)。将培养5~7 d的类器官在冰上放置5~10 min。弃上清,加入预冷的1 mL的DMEM,用大枪头将基质胶打碎,反复吹打30~50次,操作过程中尽量避免产生气泡。随后取适量的悬液观察,若在显微镜下能观察到较多完整的类器官团,则继续用大枪吹打,直至将类器官吹散为止。将悬液转移至1.5 mL离心管中,室温800 r/min离心5 min,弃上清,沉淀用适量的matrigel重悬。接板过程同上述步骤 (注:理论上类器官传一次可以扩增3~5倍,但由于操作过程中的损失,因此一般只能扩增2~3倍)。将需要冻存的类器官于冰上放置5~10 min。弃上清,加入预冷的1 mL的DMEM,用大枪头将基质胶打碎,随后均匀吹打30~50次左右,此过程应该尽量避免产生气泡。悬液转移至15 mL离心管中,用预冷的PBS补足到10 mL,4℃、800 r/min 离心5 min。去上清,用1 mL的冻存培养基(Recovery™ Cell Culture Freezing Medium,美国Gibco公司)重悬类器官,将重悬液转移至冻存管中,标记好日期、品系,放入冻存盒中于-80℃冰箱中过夜,随后转移至液氮罐中保存。若需要复苏,将冻存的隐窝取出,置于37℃水浴锅,快速解冻,随后将悬液转移至15 mL离心管中,加入14 mL预冷的基础培养基,4℃、800 r/min离心5 min。去上清,用适量的预冷基质胶重悬沉淀,然后接板 (注:复苏的过程中加入ROCK抑制剂Y-27632 (Selleck公司,美国)能够显著提高类器官的存活率) 。
1.5 类器官培养基的配制
基础培养基(basal medium):45 mL的ADMEM (Gibco公司,美国)中加入500 μL的双抗,终浓度为5 mmol/L的HEPES及谷氨酰胺(Sigma公司,德国),终浓度为1 mmol/L的N-乙酰半胱氨酸(Sigma公司,德国),500 μL N2(Invitrogen公司,美国),1000 μL B27 (Invitrogen公司,美国),用ADMEM定容至50 mL,配好后用0.22 μm的细胞滤器过滤后分装置于4℃冰箱备用。完全培养基(complete medium):在基础培养基的基础上加入终浓度为500 ng/mL的Wnt激动剂R-spondin1 (PeproTech公司,美国),100 ng/mL的BMP抑制剂Noggin (PeproTech公司,美国),以及50 ng/mL的表皮生长因子EGF (PeproTech公司,美国),配制好后用0.22 μm的细胞滤器过滤后置于-20℃冰箱备用。若需要将类器官中的大部分细胞变为干细胞,可以在完全培养基的基础上加入40 ng/mL WNT配体Wnt3a (PeproTech公司,美国)和5 μmmol/L GSK3β的抑制剂CHIR-99021 (Selleck公司,美国)。
1.6 慢病毒感染小肠类器官
在完全培养基中加入Wnt3a (40 ng/mL)和CHIR-99021 (5 μmmol/L)培养类器官,2~3 d后,类器官大部分变为由干细胞构成的圆形结构。弃上清,加1 mL的DMEM,用大枪头搅碎基质胶,悬液转移至1.5 mL离心管,用大枪吹打悬浮液30~50次,直到所有类器官被吹散(可取少许悬液在显微镜下观察)。室温900 r/min离心5 min,弃上清,加入500 μL的TrypLE™ Express (Thermo Fisher公司,美国)重悬沉淀,37℃孵育5 min,镜检观察类器官消化情况,如消化不充分可每次增加2 min的消化时间,直到类器官大部分消化为单细胞为止,随后加入500 μL的完全培养基终止消化(消化过程中可以加入适量的DNA酶来去除死亡细胞的DNA)。消化完成后,室温900 r/min离心5 min,弃上清,加入适量浓缩病毒液重悬,然后加入到48孔板中,充分混匀,用封口膜封好,在离心机中以32℃,600× g离心1 h,移除封口膜,将类器官在37℃培养箱中孵育6 h (在病毒感染的过程中可以加入8 μg/mL的polybrene (Sigma公司,德国)来提高慢病毒的感染能力,此外也可以添加Y-27632来提高类器官的生存率)。将感染好的类器官转移至1.5 mL离心管,室温900 r/min离心5 min,弃上清,冰上放置5 min,加入适量的基质胶,混匀,随后接板,最后用含有Wnt3a、CHIR-99021和Y-27632的完全培养基培养感染后的类器官,2~3天后将培养基更换为完全培养基。在荧光显微镜下观察类器官的感染效率。1.7 酶联免疫吸附实验(enzyme linked immunosorbent assay, ELISA)
接种类器官,待类器官出芽较多时,弃上清,用PBS清洗3次,每次5 min,加入500 μL的PBS,在37℃培养箱中放置6 h,取上清保存于-20℃冰箱用于后续实验。本研究购买了美国Millipore公司的Glucagon Like Peptide-1 (Active) ELISA Kit并按照其中的说明书来检测类器官中活性GLP-1的含量。1.8 腹腔内糖耐受实验(intraperitoneal glucose tolerance test, IPGTT)
为了进行腹腔内糖耐受实验,小鼠需经过16 h的饥饿处理。称量每只小鼠体重,计算2.5 g/kg体重的葡萄糖剂量,提前配置浓度为250 mg /mL的葡萄糖溶液。通过尾静脉取血来测定0 min时的血糖浓度,在断尾之前,将尾巴末端浸入0.25%布比卡因进行局部麻醉,以减轻疼痛。随后腹腔注射小鼠体重×10 μL的250 mg /mL的葡萄糖溶液和400 μL收集的类器官上清液或者PBS,在葡萄糖注射后的15、30、45、60、75、90、120 min后测量血糖浓度。1.9 小肠类器官的原位移植
小肠类器官收集到1.5 mL离心管中,基质胶重悬,置于冰上待用。腹腔注射适量的阿佛丁麻醉小鼠,随后用灭菌胶带将小鼠固定在塑料板上,剃除小鼠腹部的毛发。用剪刀在小鼠腹部剪开约0.5 cm的口子,用镊子撑开约1 cm,随后将小鼠的小肠缓慢拉出摆放在生理盐水润湿的纱布上,接着用1 mL注射器吸取约50 μL的类器官悬液,缓慢地将其注射到小肠的肠壁中。将小肠放回到小鼠腹腔中,清理并缝合伤口。最后将小鼠放在37℃的加热器上直至苏醒。移植一周后,解剖小鼠,取小肠在荧光显微镜下观测类器官的整合情况。2 结果与分析
2.1 GLP-1/Fc载体的构建,慢病毒的包装及验证
GLP-1/Fc过表达的慢病毒载体构建如图1A所示,将GLP-1/Fc置于CMV启动子后,通过IRES2与ZsGreen连接,ZsGreen的荧光强度能够体现GLP-1/Fc的表达情况。经Sanger测序验证,pLenti- CMV-GLP-1/Fc-IRES2-ZsGreen质粒构建成功,随后将该质粒转染到293T细胞中,48 h后,在荧光显微镜下观察到绿色荧光的表达(图1B)。接着将pLenti- CMV-GLP-1/Fc-IRES2-ZsGreen,pMD2.G和psPAX2质粒共转到293T细胞中,72 h后收集病毒上清。经检测,上清中病毒滴度为2.62×106/mL。用该病毒感染293T,发现感染复数(multiplicity of infection, MOI)为100,48 h后也检测到了绿色荧光的表达(图1B)。以上结果说明本研究成功构建了具有感染能力的GLP-1/Fc过表达慢病毒,可用于后续类器官的感染实验。图1
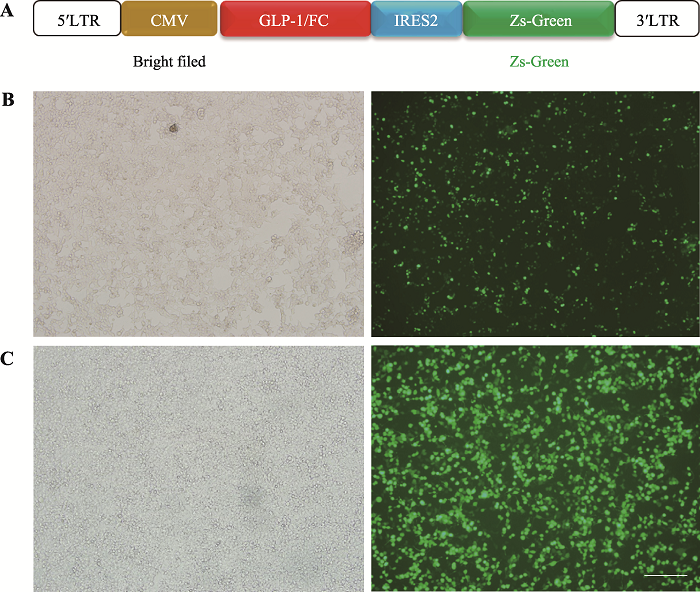
图1GLP-1/Fc慢病毒的包装及验证
A:GLP-1/Fc过表达载体构建示意图;B:GLP-1/Fc过表达质粒感染293T 48 h后Zs-Green表达情况;C:GLP-1/Fc慢病毒感染293T 48 h后Zs-Green表达情况,标尺=200 μm。
Fig. 1Packaging and validation of GLP-1/Fc lentivirus
2.2 慢病毒感染类器官条件的优化
利用上述GLP-1/Fc慢病毒感染类器官,当MOI为100,48 h后未能检测到绿色荧光的表达(图2A)。这提示类器官可能较难被慢病毒感染,因此需要对类器官的感染条件进行优化。通过查阅文献发现,在培养基中添加polybrene(8 μg/mL)以及在600× g 离心力的作用下,慢病毒的感染率能显著提高[20,21]。在上述条件下,MOI为100,再次感染类器官,48 h后依然未能检测到大量绿色荧光的表达(图2B)。为此本研究将病毒上清进行浓缩,得到3.28×108/mL的高滴度病毒,随后用该浓缩病毒感染类器官,MOI分别为200、500、1000、2000,结果显示MOI为200和500时类器官也不能被有效感染(图2,C和D)。当MOI提升到1000时,感染48 h后大部分类器官都表达绿色荧光(图2E),当将感染复数提高到2000时,类器官全部凋亡(图2F)。上述结果表明MOI为1000时,慢病毒对类器官的感染效果最佳。图2
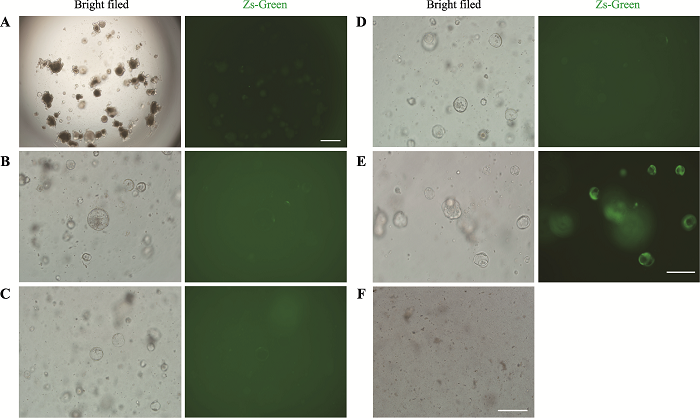
图2慢病毒感染类器官条件的优化
A:MOI=100时,病毒上清感染类器官48 h后ZsGreen的表达情况。标尺=200 μm。B~E:优化条件下,MOI=100、200、500、1000时,类器官感染病毒48 h后ZsGreen的表达情况。标尺=100 μm。F:MOI=2000时,类器官基本全部死亡。标尺=100 μm。
Fig. 2Optimization of conditions for organoids’lentivirus infection
2.3 GLP-1/FC过表达类器官的构建及验证
利用前文中优化的慢病毒感染体系,本研究成功构建了表达Zs-Green的类器官,在荧光显微镜下挑选出绿色荧光表达较强的类器官传代,经过两次传代之后的类器官依然能表达较强的绿色荧光(图3,A和B),这一结果证明能够持续稳定表达GLP-1/Fc- IRES2-ZsGreen的类器官构建成功。为了验证类器官分泌的GLP-1/Fc是否具有活性,取类器官上清进行ELISA检测,结果发现GLP-1/Fc过表达类器官产生的上清中活性GLP-1浓度可达180 pmmol/L (图3C),相比WT提高了约20倍。图3
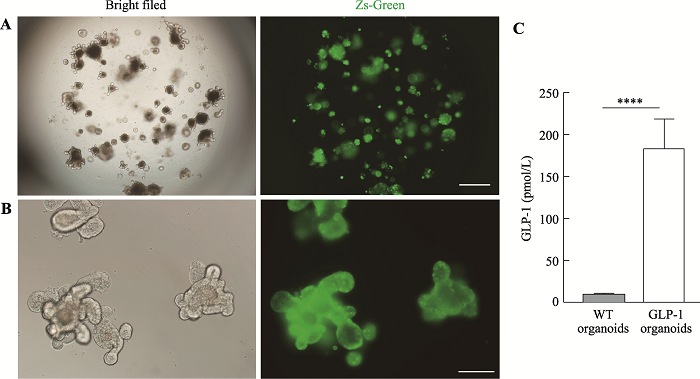
图3GLP-1/Fc过表达类器官的构建
A:GLP-1/Fc过表达类器官第一次传代3 d后Zs-Green的表达情况。标尺=200 μm。B:GLP-1/Fc过表达类器官第二次传代3 d后Zs-Green的表达情况。标尺=100 μm。C:GLP-1/Fc过表达类器官及野生型类器官上清中活性GLP-1浓度。****: P<0.0001。
Fig. 3Construction of GLP-1/Fc overexpression organoids
2.4 GLP-1/Fc显著提升WT和糖尿病小鼠的糖耐受能力
为了进一步验证过表达类器官分泌的GLP-1/Fc活性,本研究分别用WT和糖尿病小鼠进行了糖耐受实验。结果发现注射GLP-1/Fc过表达类器官上清的WT小鼠在葡萄糖注射30 min后血糖就能回复到正常水平,而注射PBS的WT小鼠在葡萄糖注射30 min后血糖依然保持在较高水平(图4A)。与此同时,在糖尿病小鼠的糖耐受实验当中,过表达类器官分泌的GLP-1/Fc上清也能够快速地降低血糖(图4B)。这些结果表明,过表达类器官分泌的GLP-1/Fc具有很强的活性,能够显著提高WT和糖尿病小鼠的糖耐受能力。图4
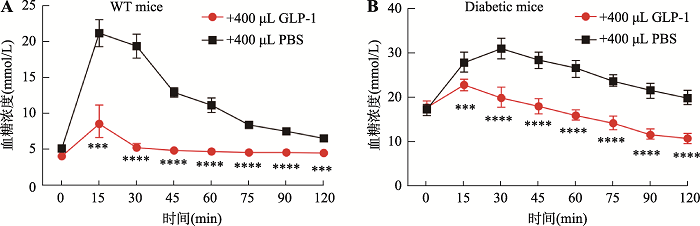
图4GLP-1/Fc在糖耐受实验中能快速回复WT和糖尿病小鼠血糖平衡
A:WT小鼠的腹腔内糖耐受实验。n=6,其中曲线下面积(area under curve, AUC)GLP-1组为38.57,PBS组为87.17。B:糖尿病小鼠的腹腔内糖耐受实验。n=6,AUC:GLP-1组为116.6,PBS组为177.6。***:P<0.001,****:P<0.0001。
Fig. 4GLP-1/Fc can quickly restore blood glucose balance in WT and diabetic mice in glucose tolerance test
2.5 类器官原位移植到小肠
为了探索GLP-1/Fc类器官原位移植到小肠从而治疗糖尿病的可能性,本研究取GFP小鼠的小肠隐窝,将其在体外培养形成能自发绿色荧光的类器官(图5A),随后通过原位移植的方式将GFP类器官注射到小鼠肠壁中(图5B)。移植一周后,取移植小鼠的小肠在荧光显微镜下观察,结果显示GFP类器官成功整合到了受体小鼠的小肠中(图5C)。以上结果说明通过原位移植GLP-1/Fc类器官治疗糖尿病具有一定的可行性。图5
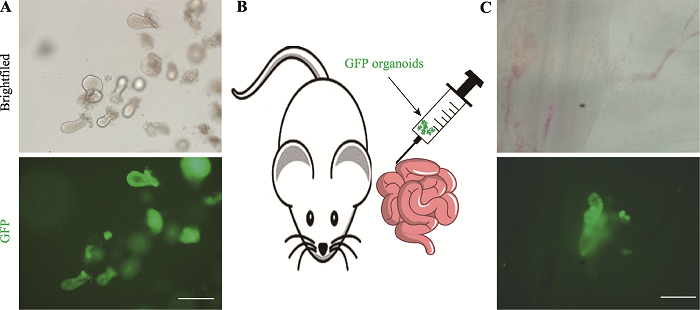
图5GFP类器官原位移植到小鼠小肠
A:GFP小鼠的隐窝在体外培养3 d后形成类器官及绿色荧光表达情况。标尺=100 μm。B:GFP类器官原位移植到小肠示意图。C:移植7 d后检测GFP类器官的整合情况。标尺=200 μm。
Fig. 5GFP-organoids were orthotopically transplanted into the small intestine of mice
3 讨论
GLP-1主要是由肠道的L细胞分泌,而葡萄糖则是促进L细胞分泌GLP-1的高效刺激物。已经有研究表明肠道L细胞内存在多种可能的葡萄糖感应机制,包括甜味受体的激活[22],钠偶联葡萄糖转运体(sodium coupled glucose transporters, SGLTs)的电活性改变[23]以及代谢依赖的钾通道(KATP)的关闭[24,25]等。其中研究的最为清楚的是葡萄糖通过改变L细胞中SGLTs的电活性从而促进GLP-1的释放。其中,SGLT1是负责小肠刷状缘顶端葡萄糖吸收的转运体,通过在每个葡萄糖分子耦合两个钠离子后内流实现逆浓度梯度的葡萄糖运输。在富含碳水化合物或游离糖的膳食或者注射葡萄糖之后,L 细胞内通过SGLT1转运的葡萄糖增多,而与之偶联的钠离子内流则足以驱动细胞膜去极化从而导致GLP-1的释放[23]。因此,在葡萄糖摄入几分钟之内就可以检测到血浆中GLP-1的升高[26]。GLP-1通过GLP-1受体发挥作用,而GLP-1受体在许多组织中表达,包括胰岛、肝脏、平滑肌,以及中枢神经系统等,所以GLP-1可以通过多种途径降低血糖。在胰岛中,GLP-1与胰岛β细胞中的GLP-1受体结合后导致腺苷酸环化酶的激活和cAMP的产生。随后通过以下几种机制刺激胰岛素分泌:(1)抑制KATP通道,导致细胞膜去极化;(2)提高细胞内钙离子浓度;(3)促进线粒体ATP合成增加,导致细胞膜的进一步去极化;(4)关闭电压依赖性钾离子通道防止胰岛β细胞的再次极化;(5)促进胰岛β细胞中胰岛素储存颗粒的胞吐[27]。此外,GLP-1还与葡萄糖协同作用,促进胰岛素基因转录、mRNA稳定和生物合成,从而补充胰岛β细胞中胰岛素储备,防止胰岛β细胞储备的耗尽[28]。还有研究报道GLP-1能抑制胰高血糖素的分泌[29]。
除了影响胰岛,GLP-1对其他组织也有显著作用。GLP-1是回肠制动的重要介质,回肠制动是一种反馈回路,当营养物质到达远端肠道时,抑制胃排空,确保食物离开胃的速度不超过近端小肠的消化吸收能力,从而维持血糖的正常[30]。在大脑中,GLP-1通过激活下丘脑和脑干中的GLP-1受体促进饱腹感,从而减少食物摄入。在肝脏与肌肉中,GLP-1能促进葡萄糖转变为肝糖原与肌糖原并增强葡萄糖的代谢[27]。
2型糖尿病患者GLP-1分泌不足,这提示GLP-1在这一高发疾病的发病机制中发挥作用,因此GLP-1及其类似物在2型糖尿病的治疗中被广泛应用[31]。但是目前注射或者口服GLP-1相关药物的治疗方式只能暂时将血糖维持在正常水平。通过293T过表达GLP-1的细胞治疗方式也存在一定局限性,比如细胞存活周期短,不能原位表达等[7,9]。因此,构建一种GLP-1过表达小鼠小肠类器官可以有效弥补以上不足之处。
本研究首先构建了GLP-1/Fc过表达的慢病毒载体,并包装了具有感染能力的慢病毒。随后,优化了慢病毒感染类器官的方法,发现当感染复数为1000时,病毒可以高效地感染类器官。通过WT和糖尿病小鼠的糖耐受实验发现GLP-1/Fc过表达类器官分泌的活性GLP-1可以显著提高小鼠的糖耐受能力。最后,本研究还验证了原位移植类器官到小肠的可行性。总的来说,本研究为2型糖尿病提供了一种潜在的治疗方法。
然而,本研究依然存在部分有待完善的地方。首先,虽然本研究初步验证了类器官原位移植的可能,但是原位移植多少GLP-1过表达类器官才能起到治疗糖尿病的效果还需要进一步的实验探究。其次,虽然有研究表明GLP-1的降血糖作用是血糖依赖型的[32],但是GLP-1在体内的持续表达是否会产生副作用也需要进一步的探究,因此可能的解决方案是设计一套血糖响应的GLP-1过表达体系,只有当血糖升高时才启动GLP-1的表达,反之则GLP-1表达关闭。最后,考虑到肠类器官中就有L细胞及GLP-1的表达[13,14],此外也已经有研究表明在培养基中加入胆酸或者G蛋白偶联胆汁酸受体1 (G- protein-coupled bile acid receptor 1)激动剂可以导致类器官中L细胞增多[15],那么是否能够通过类似的方法增加糖尿病小鼠体内的L细胞,从而治疗糖尿病,这些有意思的想法都值得进一步去探究、求证。
(责任编委: 林鑫华)
参考文献 原文顺序
文献年度倒序
文中引用次数倒序
被引期刊影响因子
PMID:10605628 [本文引用: 1]
DOI:10.1210/jcem-63-2-492URL [本文引用: 1]
PMID:6074000 [本文引用: 1]

The plasma insulin responses of normal weight and obese, diabetic, and nondiabetic subjects to intravenous glucose was only 30-40% of that seen after oral glucose, indicating that alimentary mechanism(s) in addition to the arterial blood sugar concentration regulate insulin secretion. Observations made in subjects with diverted portal circulation indicate that the alimentary insulinogenic mechanism is located in the intestinal tract. The insulinogenic potency of the alimentary and glycemic stimuli expressed in terms of insulin secretion per gram of glucose were remarkably similar within each group of individuals. Between these groups, however, there were considerable differences. Obesity, with or without associated diabetes, was associated with a true hypersecretory responsiveness, whereas diabetes was characterized, with or without obesity, by a marked impairment in insulin secretion. The experimental design used in these studies permitted quantitation of the magnitude of the glycemic component of an oral glucose load. As a consequence of impaired insulin secretion, a greater than normal proportion of the oral glucose load escapes initial hepatic extraction in the maturity-onset diabetic and enters the peripheral circulation. Therefore, in the noninsulin-requiring maturity-onset diabetic, the glycemic insulinogenic stimulus for a given oral glucose load is significantly greater than in normal subjects and accounts for the excessive plasma insulin responses observed late in the course of an oral glucose tolerance test.
DOI:10.2337/diabetes.53.3.654URL [本文引用: 1]
[本文引用: 1]
PMID:16943856 [本文引用: 1]

Glucagon-like peptide (GLP-1), a major physiological incretin, plays numerous important roles in modulating blood glucose homeostasis and has been proposed for the treatment of type 2 diabetes. The major obstacles for using native GLP-1 as a therapeutic agent are that it must be delivered by a parenteral route and has a short half-life. In an attempt to develop a strategy to prolong the physiological t(1/2) and enhance the potency of GLP-1, a fusion protein consisting of active human GLP-1 and mouse IgG(1) heavy chain constant regions (GLP-1/Fc) was generated. A plasmid encoding an IgK leader peptide-driven secretable fusion protein of the active GLP-1 and IgG(1)-Fc was constructed for mammalian expression. This plasmid allows for expression of bivalent GLP-1 peptide ligands as a result of IgG-Fc homodimerization. In vitro studies employing purified GLP-1/Fc indicate that the fusion protein is functional and elevates cAMP levels in insulin-secreting INS-1 cells. In addition, it stimulates insulin secretion in a glucose concentration-dependent manner. Intramuscular gene transfer of the plasmid in db/db mice demonstrated that expression of the GLP-1/Fc peptide normalizes glucose tolerance by enhancing insulin secretion and suppressing glucagon release. This strategy of using a bivalent GLP-1/Fc fusion protein as a therapeutic agent is a novel approach for the treatment of diabetes.
PMID:17410180 [本文引用: 3]

Glucagon-like peptide 1 (GLP-1) and its analogue exendin-4 (Ex4) have displayed potent glucose homeostasis-modulating characteristics in type 2 diabetes (T2D). However, there are few reports of effectiveness in type 1 diabetes (T1D) therapy, where there is massive loss of beta cells. We previously described a novel GLP-1 analogue consisting of the fusion of active GLP-1 and IgG heavy chain constant regions (GLP-1/IgG-Fc), and showed that in vivo expression of the protein, via electroporation-enhanced intramuscular plasmid-based gene transfer, normalized blood glucose levels in T2D-prone db/db mice. In the present study, GLP-1/IgG-Fc and Ex4/IgG-Fc were independently tested in multiple low-dose streptozotocin-induced T1D. Both GLP-1/IgG-Fc and Ex4/IgG-Fc effectively reduced fed blood glucose levels in treated mice and ameliorated diabetes symptoms, where as control IgG-Fc had no effect. Treatment with GLP-1/IgG-Fc or Ex4/IgG-Fc improved glucose tolerance and increased circulating insulin and GLP-1 levels. It also significantly enhanced islet beta-cell mass, which is likely a major factor in the amelioration of diabetes. This suggests that GLP-1/IgG-Fc gene therapy may be applicable to diseases where there is either acute or chronic beta-cell injury.
DOI:10.1126/scitranslmed.aal2298URL [本文引用: 1]
DOI:10.1126/science.1203535URL [本文引用: 2]
DOI:S0092-8674(16)30729-2PMID:27315476 [本文引用: 1]

Recent advances in 3D culture technology allow embryonic and adult mammalian stem cells to exhibit their remarkable self-organizing properties, and the resulting organoids reflect key structural and functional properties of organs such as kidney, lung, gut, brain and retina. Organoid technology can therefore be used to model human organ development and various human pathologies 'in a dish." Additionally, patient-derived organoids hold promise to predict drug response in a personalized fashion. Organoids open up new avenues for regenerative medicine and, in combination with editing technology, for gene therapy. The many potential applications of this technology are only beginning to be explored. Copyright © 2016 Elsevier Inc. All rights reserved.
DOI:10.1053/j.gastro.2011.07.050URL [本文引用: 1]
DOI:10.1038/nature07935URL [本文引用: 1]
DOI:10.2337/db13-0991PMID:24130334 [本文引用: 2]

Upon a nutrient challenge, L cells produce glucagon-like peptide 1 (GLP-1), a powerful stimulant of insulin release. Strategies to augment endogenous GLP-1 production include promoting L-cell differentiation and increasing L-cell number. Here we present a novel in vitro platform to generate functional L cells from three-dimensional cultures of mouse and human intestinal crypts. We show that short-chain fatty acids selectively increase the number of L cells, resulting in an elevation of GLP-1 release. This is accompanied by the upregulation of transcription factors associated with the endocrine lineage of intestinal stem cell development. Thus, our platform allows us to study and modulate the development of L cells in mouse and human crypts as a potential basis for novel therapeutic strategies in patients with type 2 diabetes.
DOI:S2211-1247(20)30814-7PMID:32610134 [本文引用: 2]

Glucagon-like peptide-1 (GLP-1) from intestinal L-cells stimulates insulin secretion and reduces appetite after food ingestion, and it is the basis for drugs against type-2 diabetes and obesity. Drugs targeting L- and other enteroendocrine cells are under development, with the aim to mimic endocrine effects of gastric bypass surgery, but they are difficult to develop without human L-cell models. Human ileal organoids, engineered by CRISPR-Cas9, express the fluorescent protein Venus in the proglucagon locus, enabling maintenance of live, identifiable human L-cells in culture. Fluorescence-activated cell sorting (FACS)-purified organoid-derived L-cells, analyzed by RNA sequencing (RNA-seq), express hormones, receptors, and ion channels, largely typical of their murine counterparts. L-cells are electrically active and exhibit membrane depolarization and calcium elevations in response to G-protein-coupled receptor ligands. Organoids secrete hormones in response to glucose and other stimuli. The ability to label and maintain human L-cells in organoid culture opens avenues to explore L-cell function and develop drugs targeting the human enteroendocrine system. Copyright © 2020 The Author(s). Published by Elsevier Inc. All rights reserved.
DOI:10.2337/db19-0764URL [本文引用: 2]
DOI:S2212-8778(17)30750-0PMID:29167062 [本文引用: 1]

The aim of this study was to investigate the electrical properties of ileal Glucagon-like peptide 1 (GLP-1) secreting L-cells using murine organoid cultures and the electrophysiological and intracellular signaling pathways recruited following activation of the G-coupled free fatty acid receptors FFA1 and G-coupled bile acid receptors GPBAR1. Experiments were performed using ileal organoids generated from mice transgenically expressing fluorescent reporters (Epac2-camps and GCaMP3) under control of the proglucagon promoter. Electrophysiology and single cell imaging were performed on identified L-cells in organoids, and GLP-1 secretion from cultured organoids was measured by immunoassay. The FFA1 ligand TAK-875 triggered L-cell electrical activity, increased intracellular calcium, and activated a depolarizing current that was blocked by the TRPC3 inhibitor Pyr3. TAK-875 triggered GLP-1 secretion was Pyr3 sensitive, suggesting that the TRPC3 channel links FFA1 activation to calcium elevation and GLP-1 release in L-cells. GPBAR1 agonist triggered PKA-dependent L-type Ca current activation and action potential firing in L-cells. The combination of TAK-875 and a GPBAR1 agonist triggered synergistic calcium elevation and GLP-1 secretory responses. FFA1 and GPBAR1 activation individually increased electrical activity in L-cells by recruiting pathways that include activation of TRPC3 and L-type voltage-gated Ca channels. Synergy between the pathways activated downstream of these receptors was observed both at the level of Ca elevation and GLP-1 secretion. Copyright © 2017 The Authors. Published by Elsevier GmbH.. All rights reserved.
DOI:10.1038/nm.2695URL [本文引用: 1]
DOI:10.1101/gad.245233.114URL [本文引用: 1]
DOI:10.1038/nbt.3837PMID:28459450 [本文引用: 1]

Colorectal cancer (CRC) is a leading cause of death in the developed world, yet facile preclinical models that mimic the natural stages of CRC progression are lacking. Through the orthotopic engraftment of colon organoids we describe a broadly usable immunocompetent CRC model that recapitulates the entire adenoma-adenocarcinoma-metastasis axis in vivo. The engraftment procedure takes less than 5 minutes, shows efficient tumor engraftment in two-thirds of mice, and can be achieved using organoids derived from genetically engineered mouse models (GEMMs), wild-type organoids engineered ex vivo, or from patient-derived human CRC organoids. In this model, we describe the genotype and time-dependent progression of CRCs from adenocarcinoma (6 weeks), to local disseminated disease (11-12 weeks), and spontaneous metastasis (>20 weeks). Further, we use the system to show that loss of dysregulated Wnt signaling is critical for the progression of disseminated CRCs. Thus, our approach provides a fast and flexible means to produce tailored CRC mouse models for genetic studies and pre-clinical investigation.
[本文引用: 1]
[本文引用: 1]
DOI:10.1073/pnas.0706890104URL [本文引用: 1]
DOI:10.2337/db11-1029PMID:22124465 [本文引用: 2]

To clarify the physiological role of Na(+)-D-glucose cotransporter SGLT1 in small intestine and kidney, Sglt1(-/-) mice were generated and characterized phenotypically. After gavage of d-glucose, small intestinal glucose absorption across the brush-border membrane (BBM) via SGLT1 and GLUT2 were analyzed. Glucose-induced secretion of insulinotropic hormone (GIP) and glucagon-like peptide 1 (GLP-1) in wild-type and Sglt1(-/-) mice were compared. The impact of SGLT1 on renal glucose handling was investigated by micropuncture studies. It was observed that Sglt1(-/-) mice developed a glucose-galactose malabsorption syndrome but thrive normally when fed a glucose-galactose-free diet. In wild-type mice, passage of D-glucose across the intestinal BBM was predominantly mediated by SGLT1, independent the glucose load. High glucose concentrations increased the amounts of SGLT1 and GLUT2 in the BBM, and SGLT1 was required for upregulation of GLUT2. SGLT1 was located in luminal membranes of cells immunopositive for GIP and GLP-1, and Sglt1(-/-) mice exhibited reduced glucose-triggered GIP and GLP-1 levels. In the kidney, SGLT1 reabsorbed ~3% of the filtered glucose under normoglycemic conditions. The data indicate that SGLT1 is 1) pivotal for intestinal mass absorption of d-glucose, 2) triggers the glucose-induced secretion of GIP and GLP-1, and 3) triggers the upregulation of GLUT2.
PMID:12196469 [本文引用: 1]

Glucagon-like peptide-1 (GLP-1) is released from intestinal L-cells in response to carbohydrate and fat in the diet. Despite the interest in GLP-1 as an antidiabetic agent, very little is known about the mechanism of stimulus-secretion coupling in L-cells. We investigated the electrophysiological events underlying glucose-induced GLP-1 release in the GLP-1-secreting cell line, GLUTag. Cells were studied using perforated-patch and standard whole-cell patch clamp recordings. GLUTag cells were largely quiescent and hyperpolarized in the absence of glucose. Increasing the glucose concentration between 0 and 20 mmol/l decreased the membrane conductance, caused membrane depolarization, and triggered the generation of action potentials. Action potentials were also triggered by tolbutamide (500 micro mol/l) and were suppressed by diazoxide (340 micro mol/l) or the metabolic inhibitor azide (3 mmol/l), suggesting an involvement of K(ATP) channels. Large tolbutamide-sensitive washout currents developed in standard whole-cell recordings, confirming the presence of K(ATP) channels. RT-PCR detected the K(ATP) channel subunits Kir6.2 and SUR1 and glucokinase. GLP-1 secretion was also stimulated by glucose over the concentration range 0-25 mmol/l and by tolbutamide. Our results suggest that glucose triggers GLP-1 release through closure of K(ATP) channels and action potential generation.
DOI:10.1007/s00125-012-2585-2PMID:22638549 [本文引用: 1]

Several glucose-sensing pathways have been implicated in glucose-triggered secretion of glucagon-like peptide-1 (GLP-1) from intestinal L cells. One involves glucose metabolism and closure of ATP-sensitive K(+) channels, and another exploits the electrogenic nature of Na(+)-coupled glucose transporters (SGLTs). This study aimed to elucidate the role of these distinct mechanisms in glucose-stimulated GLP-1 secretion. Glucose uptake into L cells (either GLUTag cells or cells in primary cultures, using a new transgenic mouse model combining proglucagon promoter-driven Cre recombinase with a ROSA26tdRFP reporter) was monitored with the FLII(12)Pglu-700 μδ6 glucose sensor. Effects of pharmacological and genetic interference with SGLT1 or facilitative glucose transport (GLUT) on intracellular glucose accumulation and metabolism (measured by NAD(P)H autofluorescence), cytosolic Ca(2+) (monitored with Fura2) and GLP-1 secretion (assayed by ELISA) were assessed. L cell glucose uptake was dominated by GLUT-mediated transport, being abolished by phloretin but not phloridzin. NAD(P)H autofluorescence was glucose dependent and enhanced by a glucokinase activator. In GLUTag cells, but not primary L cells, phloretin partially impaired glucose-dependent secretion, and suppressed an amplifying effect of glucose under depolarising high K(+) conditions. The key importance of SGLT1 in GLUTag and primary cells was evident from the impairment of secretion by phloridzin or Sglt1 knockdown and failure of glucose to trigger cytosolic Ca(2+) elevation in primary L cells from Sglt1 knockout mice. SGLT1 acts as the luminal glucose sensor in L cells, but intracellular glucose concentrations are largely determined by GLUT activity. Although L cell glucose metabolism depends partially on glucokinase activity, this plays only a minor role in glucose-stimulated GLP-1 secretion.
DOI:10.1530/EJE-14-0309URL [本文引用: 1]
DOI:10.1053/j.gastro.2007.03.054URL [本文引用: 2]
DOI:10.1056/NEJMoa1612917URL [本文引用: 1]
DOI:10.1038/ijo.2013.120URL [本文引用: 1]
[本文引用: 1]
DOI:S2212-8778(19)30913-5PMID:31767182 [本文引用: 1]

The glucagon-like peptide-1 (GLP-1) is a multifaceted hormone with broad pharmacological potential. Among the numerous metabolic effects of GLP-1 are the glucose-dependent stimulation of insulin secretion, decrease of gastric emptying, inhibition of food intake, increase of natriuresis and diuresis, and modulation of rodent β-cell proliferation. GLP-1 also has cardio- and neuroprotective effects, decreases inflammation and apoptosis, and has implications for learning and memory, reward behavior, and palatability. Biochemically modified for enhanced potency and sustained action, GLP-1 receptor agonists are successfully in clinical use for the treatment of type-2 diabetes, and several GLP-1-based pharmacotherapies are in clinical evaluation for the treatment of obesity. In this review, we provide a detailed overview on the multifaceted nature of GLP-1 and its pharmacology and discuss its therapeutic implications on various diseases. Since its discovery, GLP-1 has emerged as a pleiotropic hormone with a myriad of metabolic functions that go well beyond its classical identification as an incretin hormone. The numerous beneficial effects of GLP-1 render this hormone an interesting candidate for the development of pharmacotherapies to treat obesity, diabetes, and neurodegenerative disorders. Copyright © 2019 The Authors. Published by Elsevier GmbH.. All rights reserved.
PMID:16929351 [本文引用: 1]

Glucagon-like peptide 1 (GLP-1) is a promising candidate for the treatment of type II diabetes. However, the short in vivo half-life of GLP-1 has made peptide-based treatments challenging. Gene therapy aimed at achieving continuous GLP-1 expression presents one way to circumvent the rapid turnover of GLP-1. We have created a GLP-1 minigene that can direct the secretion of active GLP-1 (amino acids 7-37). Plasmid and adenoviral expression vectors encoding the 31-amino-acid peptide linked to leader sequences required for secretion of GLP-1 yielded sustained levels of active GLP-1 that were significantly greater than endogenous levels. Systemic administration of expression vectors to animals using two diabetic rodent models, db/db mice and Zucker Diabetic Fatty (ZDF) rats, yielded elevated GLP-1 levels that lowered both the fasting and random-fed hyperglycemia present in these animals. Because the insulinotropic actions of GLP-1 are glucose dependent, no evidence of hypoglycemia was observed. Improved glucose homeostasis was demonstrated by improvements in %HbA1c (glycated hemoglobin) and in glucose tolerance tests. GLP-1-treated animals had higher circulating insulin levels and increased insulin immunostaining of pancreatic sections. GLP-1-treated ZDF rats showed diminished food intake and, in the first few weeks following vector administration, a diminished weight gain. These results demonstrate the feasibility of gene therapy for type II diabetes using GLP-1 expression vectors.