

Uptake kinetics of nitrogen and phosphorus by Zostera marina
Shi-Qi LI

通讯作者: *zhangpdsg@ouc.edu.cn
编委: 陈小勇
责任编辑: 李敏
收稿日期:2019-12-3接受日期:2020-05-1网络出版日期:2020-07-20
基金资助: |
Corresponding authors: *zhangpdsg@ouc.edu.cn
Received:2019-12-3Accepted:2020-05-1Online:2020-07-20
Fund supported: |
作者简介 About authors
李诗奇:ORCID:0000-0002-6100-457X。

摘要
营养盐是影响海草生长的关键因子, 目前有关海草不同组织对不同形式氮和磷的吸收特征尚不明确。该研究通过利用海草地上和地下组织分隔培养装置, 设置不同的氨态氮、硝态氮和磷酸盐浓度, 探究了大叶藻(Zostera marina)植株及其地上和地下组织对氮磷营养盐的吸收动力学特征。结果显示: (1)大叶藻对氮磷的吸收符合饱和吸收动力学特征, 吸收速率和水体氮磷浓度可用米式方程描述; (2)大叶藻植株对NH4+-N的最大吸收速率(Vmax, 52 μmol·g-1·h-1)显著高于其对NO3--N的Vmax (39 μmol·g-1·h-1); (3)大叶藻地上组织和地下组织均可吸收氮磷, 但地上组织对氨态氮、硝态氮、磷酸盐的Vmax分别为43.1、30.5和15.6 μmol·g-1·h-1, 为地下组织的2.6、1.2和6倍。结果表明, 大叶藻对氨态氮的吸收能力高于硝态氮, 且对氮磷的吸收可能主要依赖地上组织(叶片)。结果为查明大叶藻对氮磷的吸收利用机制及评估大叶藻的海洋生态效应提供了理论依据。
关键词:
Abstract
Aims Nutrient availability is an external factor that affect the growth of seagrasses. However, the demand for and absorption of different forms of nitrogen and phosphorus by different tissues of seagrasses are unclear. In this study, the uptake of nitrogen and phosphorus by Zostera marina was studied to determine the nutrient uptake kinetics. The objectives of this research are to: 1) investigate the absorption characteristics of ammonia nitrogen, nitrate nitrogen and phosphorus in Z. marina; 2) evaluate the differences in absorption between the different forms of nitrogen; and 3) analyse the differences in absorption between the different tissues of Z. marina.
Methods Equipment was used to separate the aboveground and belowground tissues of Z. marina. Six concentration levels of ammonia nitrogen, nitrate nitrogen and phosphorus were established to experimentally test the uptake kinetics of nutrients by Z. marina. The nutrient concentrations in different parts of seawater column were measured to determine the nutrient changes and calculate the kinetic characteristics of nutrient uptake.
Important findings 1) The absorption of ammonia nitrogen, nitrate nitrogen and phosphorus by Z. marina was consistent with the characteristics of saturated absorption kinetics. The relationship between the absorption rate and the nutrient concentrations in water could be described by the Michaelis-Menten equation. 2) The maximum absorption rate (Vmax) of ammonia nitrogen by Z. marina (52 μmol·g-1·h-1) was significantly higher than that of nitrate nitrogen (39 μmol·g-1·h-1). 3) Both aboveground and belowground tissues of Z. marina could absorb nutrient, but the Vmax of leaves (aboveground tissues) for ammonia nitrogen, nitrate nitrogen and phosphorus absorption were 43.1, 30.5 and 15.6 μmol·g-1·h-1, respectively, which were 2.6-fold, 1.2-fold and 6-fold higher than the corresponding Vmax of belowground tissues. The results show that the absorption capacity of Z. marina for ammonia nitrogen is higher than that for nitrate nitrogen, and the absorption of nitrogen and phosphorus may depend primarily on the aboveground tissues (leaves). The results provide a theoretical basis for the study of the mechanisms of nitrogen and phosphorus uptake and utilization by Z. marina and the evaluation of marine ecological impacts.
Keywords:
PDF (1317KB)元数据多维度评价相关文章导出EndNote|Ris|Bibtex收藏本文
引用本文
李诗奇, 张彦浩, 李政, 张沛东. 大叶藻对氮磷营养盐的吸收动力学特征. 植物生态学报, 2020, 44(7): 772-781. DOI: 10.17521/cjpe.2019.0335
LI Shi-Qi, ZHANG Yan-Hao, LI Zheng, ZHANG Pei-Dong.
海草是一类海洋高等单子叶开花植物, 具有极高的初级生产力, 在我国主要分布于温带的山东、辽宁、河北, 亚热带、热带的广东、广西和海南等沿岸海域(李文涛和张秀梅, 2009; Short et al., 2011; 郑凤英等, 2013)。海草床具有重要的生态功能和极高的生态服务价值(Unsworth et al., 2019)。Short等(2011)估算发现, 海草床的生态服务价值高达34 000 $·hm-2·a-1, 超过大多数陆地生境及海洋 生境。
海草床生态系统较脆弱, 受全球变化和人类活动影响, 目前已是地球生物圈退化速率最快的生态系统, 且退化速率仍在逐年增加(Waycott et al., 2009; 郑凤英等, 2013; 郭美玉等, 2017; Perry et al., 2020)。然而在海草床修复过程中, 对促进海草快速生长的关键因子及其生态幅的科学认知还远远不够, 限制了高效修复方法的建立。光照、温度和营养盐是影响海草生长的三大外界因素(Lee et al., 2007)。Dennison等(1993)在对澳大利亚、古巴、美国等17个国家或地区的15种海草种类进行研究后分别得出了不同地区不同海草种类的最小需光量(%); Lee等(2007)综合分析已有文献报道, 提出大叶藻(Zostera marina)生长的适宜温度范围为15-20 ℃; 尽管20世纪70年代就有****尝试探究海草的营养生理生态学理论, 但至今仍未得出海草的适宜营养盐浓度范围(Orth, 1973; van Katwijk et al., 1999; Hemminga & Duarte, 2000; Touchette & Burkholder, 2000; Romero et al., 2006; Kaldy, 2014; Pereda-Briones et al., 2018)。
氮磷是水生植物最主要的限制性营养盐, ****普遍使用米氏方程来表示稳态时水生植物对营养盐的吸收特征(Duarte, 1990; Geider et al., 1997; Thormann & Bayley, 1997; 刘静雯等, 2001; Güsewell et al., 2003; Pietro et al., 2006; Rubio et al., 2018)。研究表明, 大多数海草种类都优先吸收氨态氮(Vonk et al., 2008; van Engeland et al., 2011), 但其地上组织和地下组织对营养盐的吸收能力存在差异(Gras et al., 2003; Nielsen et al., 2006)。因此, 查明海草对营养盐的吸收动力学特征, 得出不同组织对不同形式氮和磷的吸收参数, 对明确海草最适营养盐生态幅具有重要科学意义。
本研究以我国北方海域优势海草种类——大叶藻为对象, 针对大叶藻营养生理生态学存在的理论欠缺, 通过利用海草地上组织和地下组织分隔培养装置, 设置不同的氨态氮、硝态氮、磷酸盐浓度, 探讨了大叶藻对不同形式氮和磷的吸收动力学特征, 评价了大叶藻植株、地上组织和地下组织对氨态氮、硝态氮和磷酸盐的吸收动力学差异, 为探明大叶藻对氮磷的需求和吸收理论提供参考, 并为建立我国海草床退化生境恢复技术提供理论依据。
1 材料和方法
1.1 植株采集
实验用大叶藻植株于2019年7月采自山东省荣成市天鹅湖海域(37.34°-37.36° N, 122.56°-122.58° E), 采样时海水温度26.2 ℃。采集时, 选择叶片颜色鲜绿的植株, 将植株从底部连根挖出, 确保大叶藻植株完整。进一步筛选植株, 所选植株具备相对一致的形态学特征: 株高24-26 cm, 叶片4-5片, 茎节长5 cm。植株经海水浸洗, 去除底质、贝类及其他附生生物, 移入盛有海水的泡沫箱中, 于室外水槽中暂养3天, 暂养期间采用自然海水, 遮阳网遮阳, 平均水温24.4 ℃。1.2 实验处理与分隔装置
van Katwijk等(1997)研究发现, 水体氨态氮浓度≥125 μmol·L-1时对大叶藻产生毒性; Udy和Dennison (1997)的研究表明, 水体磷酸盐浓度≥ 30 μmol·L-1时对海草生长具有显著促进作用。根据这些已有报道以及预实验结果, 设置氨态氮(NH4Cl)和硝态氮(KNO3)浓度分别为10、20、40、60、80和100 μmol·L-1, 磷酸盐(KH2PO4)浓度为2、4、8、16、24和32 μmol·L-1。各营养盐水平分别设置3个实验组: 未分隔组(UG)、分隔且地上组织添加组(SGA)和分隔且地下组织添加组(SGB), 分别用于评价大叶藻植株、地上组织和地下组织对氨态氮、硝态氮和磷酸盐的吸收动力学特征。不同实验组水体营养盐浓度设置方案见表1。其中天然海水氨态氮浓度为(3.04 ±0.14) μmol·L-1, 硝态氮浓度为(2.41 ± 0.18) μmol·L-1, 磷酸盐浓度为(0.25 ± 0.02) μmol·L-1。各实验组设置12个重复。Table 1
表1
表1大叶藻分隔培养不同实验装置水体营养盐浓度设置方案
Table 1
分隔装置 Separation apparatus | 未分隔组 No-separating group | 分隔且地上组织添加组 Separation group with N, P addition in the leaf compartment | 分隔且地下组织添加组 Separation group with N, P addition in the root- rhizome compartment |
---|---|---|---|
玻璃水槽 Glass aquarium | 设置的营养盐浓度 Designed nutrient concentration | 设置的营养盐浓度 Designed nutrient concentration | 天然海水营养盐浓度 Nutrient concentration of natural seawater |
聚乙烯瓶 Polyethylene bottle | 设置的营养盐浓度 Designed nutrient concentration | 天然海水营养盐浓度 Nutrient concentration of natural seawater | 设置的营养盐浓度 Designed nutrient concentration |
新窗口打开|下载CSV
实验选取40 cm × 30 cm × 60 cm的玻璃水槽, 用玻璃将其均匀分为12个10 cm × 10 cm × 55 cm的封闭独立小格, 用于模拟大叶藻地上组织的生活环境; 选取250 mL聚乙烯瓶, 在其瓶盖上均匀制造3个直径为3 cm的小孔, 并用黑色胶布将聚乙烯瓶瓶身遮住, 以模拟大叶藻地下组织的暗光环境, 如图1所示。
图1
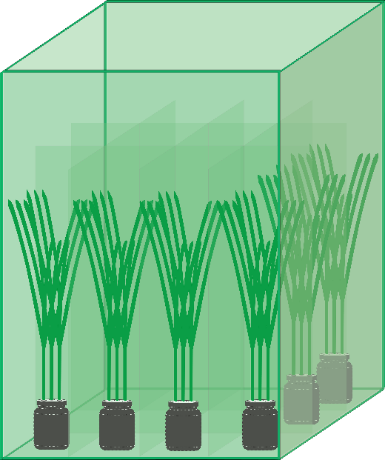
图1大叶藻分隔培养实验装置示意图。
Fig. 1Schematic diagram of experimental apparatus for separate cultivation of Zostera marina.
1.3 实验过程
实验时, 首先在聚乙烯瓶内注满设定营养盐浓度的海水; 然后随机选择3株大叶藻植株, 将其地下组织缓慢穿过聚乙烯瓶盖上的小孔至植株维管束顶端即分生组织处为止, 记为1个重复; UG实验组不对小孔密封, 随机选择玻璃水槽的独立小格, 将植有3株植株的聚乙烯瓶放入后添加5 L设定营养盐浓度海水; 两个分隔实验组则用硅脂密封瓶盖小孔, 其他过程同UG实验组。实验开始后, 各实验组分别于1、2、3、4 h随机选取3个重复, 利用50 mL离心管分别采集UG实验组和SGA实验组独立小格的水体水样以及SGB实验组聚乙烯瓶的水体水样, 置于-20 ℃冰箱冷冻保存, 待测氮磷浓度, 并取出3个重复的植株用于干质量的测定。实验期间, 遮阳网遮阳, 平均水温(24.4 ± 1.2) ℃, 平均光量子通量密度为(15.0 ± 0.3) mol·m-2·d-1。1.4 样品采集与测定
1.4.1 水体营养盐指标的测定水体营养盐浓度测定参照海洋监测规范第4部分: 海水分析(GB 17378.4—2007)。氨态氮浓度采用次溴酸盐氧化法测定; 硝态氮浓度采用锌-镉还原法测定; 磷酸盐浓度采用磷钼蓝分光光度法测定。
1.4.2 植株干质量的测定
大叶藻植株样品用去离子水冲洗干净, UG实验组取整株植株, SGA实验组取植株的地上组织, SGB实验组取植株的地下组织, 分别置于60 ℃烘干箱48 h至恒质量, 取出后称质量。
1.4.3 米氏方程
大叶藻植株及其地上组织、地下组织对营养盐的吸收速率随水体营养盐浓度的升高而逐渐升高, 最后趋于平缓, 符合饱和吸收动力学特征, 因此可用米式方程来表征大叶藻不同部位对不同营养盐的吸收动力曲线。米氏方程(v)使用如下公式计算:
式中, v为吸收速率(μmol·g-1·h-1); Vmax为最大吸收速率(μmol·g-1·h-1); [S]为介质中营养盐浓度(μmol·L-1); Km为半饱和常数(μmol·L-1)。
根据得到的水体营养盐浓度随时间的变化趋势, 首先选择合适的时间程用于计算Vmax和Km。时间程是准确计算v值时所需的具体时间, 该时间段内营养盐的变化与时间呈线性关系, 即植株对营养盐的吸收速率只与营养盐浓度有关, 而与时间无关, 从而能够准确绘制吸收动力学曲线, 求算v值。根据实验期间水体氨态氮、硝态氮、磷酸盐浓度随时间的变化趋势, 确定UG实验组氨态氮、硝态氮和磷酸盐的时间程均为1 h, SGA实验组分别为2、2和1 h, SGB实验组分别为1、2和1 h。
根据确定的时间程、营养盐浓度的变化和植株/组织干质量, 计算时间程内植株在不同营养盐浓度下的吸收速率, 再利用双倒数作图法计算Vmax和Km。
1.5 数据处理与分析
数据以平均值±标准偏差(SD)表示, 利用Excel 2007、SPSS 19.0及ORIGIN 8.5软件对数据进行统计分析并绘图。采用单因素方差分析和Duncan’s多重比较分析相同营养盐形式下植株不同组织之间吸收动力学参数的差异显著性以及相同营养盐浓度下植株不同组织之间吸收速率的差异显著性, 若差异显著则根据多重比较结果进行差异显著性标注。以p < 0.05作为差异显著水平, p < 0.01作为差异极显著 水平。2 结果
实验用大叶藻植株平均干质量为1.05 g, 其中地上组织的平均干质量为0.88 g, 地下组织的平均干质量为0.20 g。根据米式方程, 得出各营养盐形式的Vmax和Km, 如表2所示。大叶藻不同组织的Vmax呈现植株>地上组织>地下组织的趋势, 其中地上组织对NH4+-N和PO43--P吸收的Vmax显著高于地下组织, 分别为地下组织的2.6和6.0倍; 大叶藻植株对NH4+-N吸收的Vmax显著高于对NO3--N吸收的Vmax, 是其1.3倍; 地上组织和地下组织之间的Km值均无显著性差异(p > 0.05)。Table 2
表2
表2大叶藻对NH4+-N、NO3--N和PO43--P的吸收动力学参数
Table 2
营养盐形式 Form of nutrient | 植株部位 Tissue of plant | Vmax (μmol·g-1·h-1) | Km (μmol·L-1) | R2 |
---|---|---|---|---|
NH4+-N | 植株 Shoot | 51.8 ± 7.1b | 68.1 ± 15.9 | 0.98 |
地上组织 Aboveground tissue | 43.1 ± 7.2b | 58.9 ± 19.4 | 0.92 | |
地下组织 Belowground tissue | 16.3 ± 8.5a | 48.6 ± 17.9 | 0.95 | |
NO3--N | 植株 Shoot | 39.1 ± 7.0 | 68.6 ± 16.8 | 0.99 |
地上组织 Aboveground tissue | 30.5 ± 10.9 | 42.8 ± 13.5 | 0.97 | |
地下组织 Belowground tissue | 26.5 ± 3.8 | 53.3 ± 9.1 | 0.99 | |
PO43--P | 植株 Shoot | 27.9 ± 8.4b | 24.8 ± 4.4b | 0.97 |
地上组织 Aboveground tissue | 15.6 ± 0.9b | 7.6 ± 0.2a | 0.90 | |
地下组织 Belowground tissue | 2.6 ± 0.9a | 6.0 ± 1.9a | 0.97 |
新窗口打开|下载CSV
大叶藻对NH4+-N的吸收动力学曲线见图2。大叶藻植株及其地上和地下组织对NH4+-N的吸收速率均随水体NH4+-N浓度的增加而增大, 至80 μmol·L-1后趋于平缓。单因素方差分析显示, NH4+-N浓度为10 μmol·L-1时, 各组织之间的吸收速率无显著差异(p > 0.05); 当NH4+-N浓度≥20 μmol·L-1时, 大叶藻地上组织的吸收速率与植株的吸收速率无明显不同(p > 0.05), 但显著高于地下组织(p < 0.05), 是地下组织的1.4-3.4倍。
图2
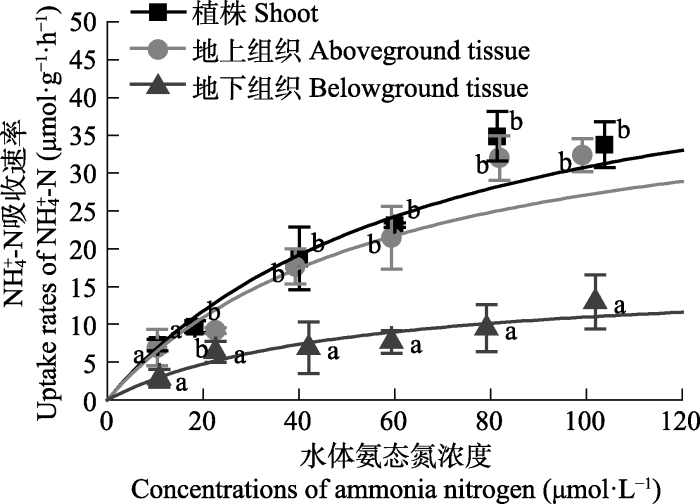
图2不同氨态氮浓度下大叶藻对NH4+-N的吸收动力学特征(平均值±标准偏差)。
误差线上不同小写字母表示同一浓度下植株不同组织之间存在显著差异(p < 0.05)。
Fig. 2Kinetics characteristics of NH4+-N uptake of Zostera marina in the different ammonia nitrogen concentrations (mean ± SD).
Different lowercase letters above bars indicate significant differences between different tissues under the same nutrient concentration (p < 0.05).
大叶藻对NO3--N的吸收动力学曲线见图3。大叶藻植株及其地上和地下组织对NO3--N的吸收速率均随水体NO3--N浓度的增加而增大, 至80 μmol·L-1后趋于平缓。不同组织的吸收速率呈现植株>地上组织>地下组织的趋势, 但各组织之间无显著性差异(p > 0.05)。
图3
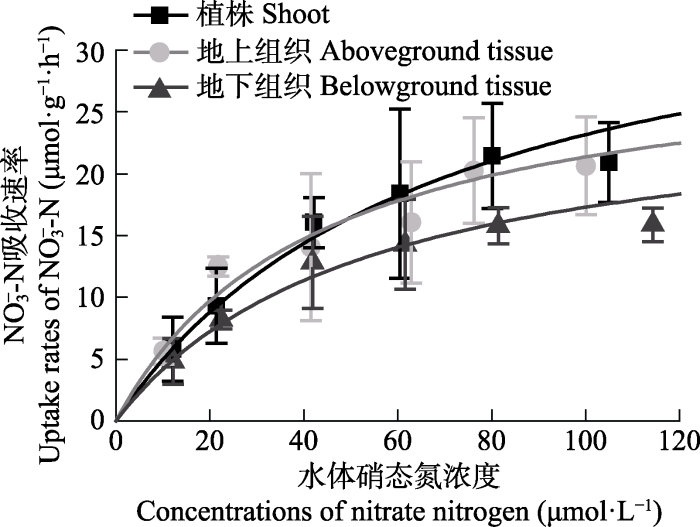
图3不同硝态氮浓度下大叶藻对NO3--N的吸收动力学特征(平均值±标准偏差)。
Fig. 3Kinetics characteristics of NO3--N uptake of Zostera marina in the different nitrate nitrogen concentrations (mean ± SD).
大叶藻对PO43--P的吸收动力学曲线见图4。大叶藻植株及其地上和地下组织对PO43--P的吸收速率均随水体PO43--P浓度的增加而增大, 至24 μmol·L-1后趋于平缓。单因素方差分析显示, PO43--P浓度为2-4 μmol·L-1时, 各组织之间的吸收速率无显著差异(p > 0.05); 当PO43--P浓度≥8 μmol·L-1时, 地上组织的吸收速率与植株的吸收速率无明显不同(p > 0.05), 但显著高于地下组织(p < 0.05), 平均是地下组织的5.1倍。
图4
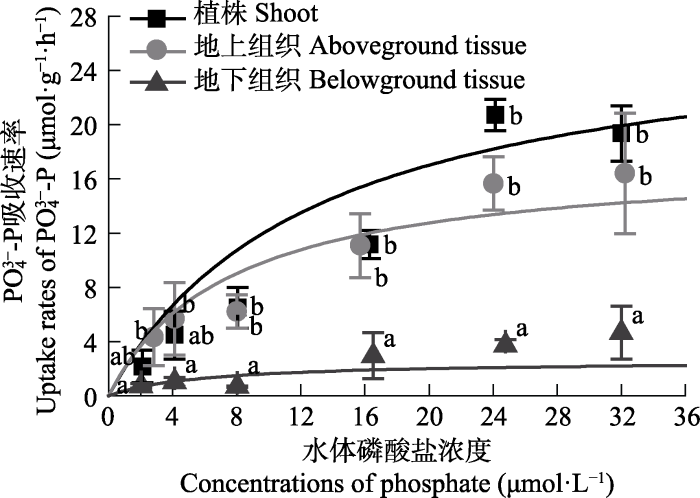
图4不同磷酸盐浓度下大叶藻对PO43--P的吸收动力学特征(平均值±标准偏差)。
误差线上不同小写字母表示同一浓度下植株不同组织之间存在显著差异(p < 0.05)。
Fig. 4Kinetics characteristics of PO43--P uptake of Zostera marina in the different phosphate concentrations (mean ± SD).
Different lowercase letters above bars indicate significant differences between different tissues under the same nutrient concentration (p < 0.05).
3 讨论
3.1 大叶藻对氮磷元素的吸收动力学特征
在米氏方程中, Vmax表示底物饱和时的吸收速率, 越大则吸收的内在潜力越大, Km表示当吸收速率为最大吸收速率一半时的底物浓度, Km值越小, 对底物亲和力越大(刘静雯和董双林, 2001; 田霄鸿等, 2001)。研究表明, 大叶藻植株对NH4+-N、NO3--N和PO43--P表现出饱和吸收动力学的特征, 其对营养盐的吸收速率和水体氮磷浓度可用米式方程描述, 这与之前对有些藻类和海草吸收动力学特征的研究结果(Lavery & McComb, 1991; Hanisak & Harlin, 2010)相符合。Rubio等(2018)研究发现, 大洋波喜荡草(Posidonia oceanica)对NO3--N和PO43--P的吸收亦符合饱和吸收动力学, 但其Km值显著低于大叶藻。这可能反映了不同海草种类栖息环境氮磷营养盐条件的差异以及对NO3--N和PO43--P的转运系统不同, 大洋波喜荡草在低氮、低磷条件下, 主要通过Na+依赖式转运系统吸收NO3--N和PO43--P, 而大叶藻则主要采用H+依赖式转运系统(Lara et al., 1993; Bethoux et al., 2005; Rubio et al., 2018)。大叶藻在寡营养盐条件下具有明显的竞争优势, 减少氮营养盐的输入则可以明显促进退化海草床的自我恢复(Greening & Janicki, 2006; Sandoval-Gil et al., 2019)。富营养化会对大叶藻产生显著的负效应(Campbell et al., 2018; Li et al., 2019), 如van Katwijk等(1997)研究发现, 北半球富营养化水域大叶藻海草床的退化主要归咎于水体氨态氮的毒性。高氮环境下, 大叶藻植株的存活、生长和碳贮存均受到显著抑制(Villazán et al., 2015; Darnell & Dunton, 2017)。与藻类相比, 大叶藻对NH4+-N和NO3--N吸收的Vmax值较低, 而对PO43--P的Vmax较高(表3)。这种低氮高磷的吸收特征表明, 大型海藻的引入可能会导致大叶藻海草床的退化, 大型藻类能够抢先利用氮进行自身的快速繁殖, 进而造成大叶藻的氮营养盐限制, 因此在大叶藻海草床的修复过程中, 应警惕藻类的爆发, 一旦藻类大量繁殖, 不仅会减弱水下光照, 影响海草的光合作用, 还会掠夺海水中的营养盐, 限制大叶藻对氮营养盐的利用(Phillips et al., 1978; Orth & van Montfrans, 1984; Haglund & Pedersén, 1993)。
Table 3
表3
表3海草和藻类对NH4+-N、NO3--N和PO43--P的吸收动力学参数
Table 3
种类 Species | 研究地点 Study area | Vmax (μmol·g-1·h-1) | Km (μmol·L-1) | 文献 Reference | ||||
---|---|---|---|---|---|---|---|---|
NH4+-N | NO3--N | PO43--P | NH4+-N | NO3--N | PO43--P | |||
大叶藻 Zostera marina | 山东荣成天鹅湖 Tian’e Lake, Rongcheng, Shandong China (37.35° N, 122.57° E) | 51.8 | 39.1 | 27.9 | 68.1 | 68.6 | 24.8 | 本研究 This study |
美国罗德岛 Rhode Island, USA (41.30° N, 71.30° W) | 48 | Thursby & Harlin, 1982 | ||||||
Phyllospadix torreyi | 美国拉由拉市 La Jolla, USA (32.48° N, 117.16° W) | 96-204 | 25-75 | 9-34 | 4-17 | Terrados & Williams, 1997 | ||
泰来藻 Thalassia hemprichii | 印度尼西亚斯佩蒙德群岛 Spermonde Archipelago, Indonesia (5.03° S, 119.20° E) | 32-37 | 21-60 | Stapel et al., 1996 | ||||
Thalassia testudinum | 美国圣体湾和马德雷湖 Corpus Christi Bay (27.49° N, 97.07° W) & Laguna Madre, USA (26.09° N, 97.12° W) | 8-16 | 4-7 | 8-15 | 2-39 | Lee & Dunton, 1999 | ||
Posidonia oceanica | 西班牙马拉加 Málaga, Spain (36.40° N, 4.21° W) | 8.7 | 5.8 | Rubio et al., 2018 | ||||
真江蓠 Gracilari asiatica | 浙江奉化象山港 Xiangshan Harbor, Fenghua, China (29.11° N, 122.01° E) | 159.4 | Wen et al., 2008 | |||||
龙须菜 Asparagus schoberioides | 福建东山岛 Dongshan Island, Fujian China (23.36° N, 117.14° E) | 3.1 | Xu et al., 2007 | |||||
浒苔 Ulva prolifera | 山东青岛汇泉湾 Huiquan Bay, Qingdao, Shandong China (36.03° N, 123.20° E) | 250.4 | 5.8 | Wang et al., 2011 |
新窗口打开|下载CSV
此外, 植株的吸收动力学特征受到环境光照、温度和盐度等环境条件的影响。光照的影响主要体现在植株的光合作用和酶活性等方面, 如有研究表明, 水生植物在光照条件下对营养盐的吸收速率远大于其在黑暗条件下的吸收速率, 光照还能激活水生植物体内的碱性磷酸酶活性, 为植物吸收利用磷酸盐提供条件(Weich & Granéli, 1989; 黄邦钦等, 1999; 刘长发等, 2001)。温度则主要通过影响植株的呼吸作用及光合作用暗反应的酶活性来影响植株对氮的吸收(Lomas & Glibert, 1999)。研究发现, 温度升高时, 龟裂泰来草(Thalassia testudinum)对NH4+-N和NO3--N的Vmax值也随之提高(Lee & Dunton, 1999)。同时, 光照和温度的交互作用也会对水生植物的营养盐吸收过程产生影响, 如程丽巍等(2010)研究表明, 在适温范围时, 水生植物对NO3--N的吸收速率随光强的增加而增加, 但在高温条件下, 光强的升高并不能促进营养盐的吸收。盐度也是调节水生植物营养盐吸收过程的关键因子, 低盐度条件下植物对NH4+-N的吸收速率较高(刘静雯和董双林, 2001)。
3.2 大叶藻对不同形式氮的吸收利用
陆生高等植物主要以硝态氮和氨态氮作为无机氮素的来源, 但更多的是吸收硝态氮, 只有在某些还原性较强的土壤或者水淹地, 其主要的氮源才可能是氨态氮(McCarthy et al., 2004)。本研究结果发现, 大叶藻优先吸收氨态氮作为氮源, 与大多数海草种类相同(Brun et al., 2002; Guo et al., 2007; Alexandre et al., 2015), 这是因为海草营沉水生活, 海草体内空间不足以积聚硝态氮, 因此当硝态氮被海草吸收后, 其将转化为氨态氮的形式进行储存或者存于液泡中, 该过程需要消耗大量的能量和磷, 从而增加磷限制风险(Turpin, 1991; Burkholder et al., 1992; Alexandre et al., 2004; Burkholder et al., 2007)。刘静雯和董双林(2001)总结了影响藻类对两种氮吸收差异的原因, 氮缺乏时植株优先吸收NH4+-N, 氮充足时NH4+-N还会抑制NO3--N的吸收, 这与植株的生长速率和碳代谢以及环境因子密切相关。同时, 由于两种离子各自传递和同化过程的特点不同, NO3--N的吸收过程比NH4+-N的吸收过程需要更多的能量支出, 从而可能进一步影响NO3--N的吸收利用(Flynn, 1991)。
3.3 大叶藻不同部位对氮磷的吸收利用
尽管已有研究表明, 海草既可以通过根系从沉积物中吸收氮磷, 也可以通过叶片从海水中获取, 但多数海草种类的叶片是吸收氮磷的主要部位(Lee & Dunton, 1999; Gras et al., 2003; Nielsen et al., 2006)。如Pedersen等(1997)研究表明, 南极根枝草(Amphibolis antarctrica)的叶片对NH4+的吸收速率是其根茎的5-38倍; Hemminga等(1994)研究表明, 大叶藻叶片在氮吸收过程中发挥主要作用, 68%-92%的氮吸收量来源于叶片; 龟裂泰来草叶片对PO43-P的最大吸收速率是地下组织的3.3倍(Gras et al., 2003); 川蔓草(Ruppia maritima)叶片对NH4+-N的最大吸收速率是地下组织的5倍(Thursby & Harlin, 1984)。本研究也发现, 尽管大叶藻的地上组织和地下组织均可吸收氮磷营养盐, 但地上组织的Vmax值更高, 因此大叶藻可能更依赖叶片吸收氮磷, 其对氮磷的吸收模式如图5所示。海草叶片对氮磷营养盐的吸收率较高, 与其谷氨酰胺合成酶和硝酸还原酶活力显著高于地下组织密切相关, 叶片在酶的催化下可以高效吸收并充分利用氮磷营养盐(Kraemer et al., 1997; Alexandre et al., 2004)。图5
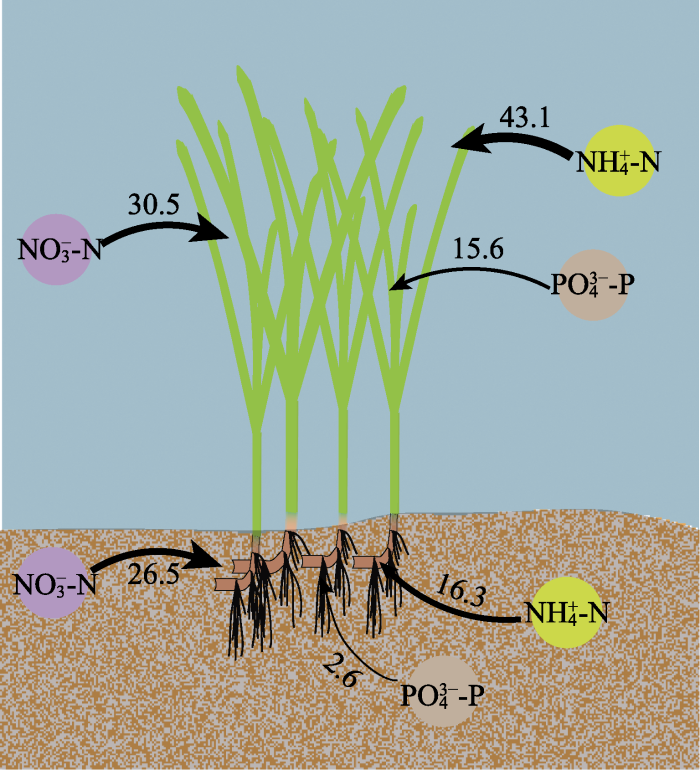
图5大叶藻对氮磷元素的吸收模式示意图。
图中数字表示最大吸收速率(Vmax, μmol·g-1·h-1)。
Numbers in the figure mean maximum absorption rate (Vmax, μmol·g-1·h-1).
也有部分海草并非依赖地上组织吸收氮磷营养盐。如Erftemeijer和Middelburg (1995)认为, 热带海草种类地上组织和地下组织对营养盐的摄取比例相差甚小; Lee和Dunton (1999)的研究表明, 龟裂泰来草叶片的氮吸收量约占植株的50%; Stapel等(1996)则发现, 泰来草(Thalassia hemprichii)主要依赖地下组织吸收营养盐, 这主要是因为其栖息海域间隙水含有丰富的营养盐, 从而为海草提供了主要的营养来源。
海草对营养盐的吸收还受到环境营养盐浓度的影响。当环境处于低营养盐状态时, 海草则增加从 底质吸收营养盐的比例, 以适应低营养盐环境(刘伟妍等, 2017)。当水体营养盐处于富营养化条件时,海草植株通常更多地利用地下组织吸收营养盐, 如富营养化条件下大叶藻植株从间隙水吸收利用的氨态氮占植株生长所需氮的55% (Mukai et al., 1979)。
同时, 在海草体内存在营养转移的现象, 氮磷在海草不同叶龄结构的叶片间转移可以提高叶片对氮磷的利用效率, 进而促进海草的生长(Terrados & Williams, 1997; Lee & Dunton, 2000)。如Alcoverro等(1995)研究表明, 大洋波喜荡草每年从其衰老组织中再吸收的氮磷可以占到年需总量的37%-44%; Stapel和Hemminga (1997)研究表明, 营养转移可以为海草叶片生长提供15%的磷; 在氨态氮浓度较低的水环境中, 当海草处于生长旺盛期时, 海草叶片30%的NH4+来源于植株内部的重新分配(Pedersen et al., 1997)。这可能与大多数海草营完全沉水生活有关。
4 结论
大叶藻植株及其不同组织对氨态氮、硝态氮和磷酸盐的吸收符合饱和吸收动力学特征, 其对氮磷的吸收速率随营养盐浓度的增加而逐渐上升。大叶藻对氨态氮的最大吸收速率显著高于其对硝态氮的最大吸收速率, 是硝态氮的1.3倍; 大叶藻的地上组织和地下组织均可吸收氨态氮、硝态氮和磷酸盐, 但地上组织(叶片)的最大吸收速率更高, 分别为地下组织最大吸收速率的2.6、1.2和6倍, 推测大叶藻主要利用地上组织(叶片)进行氮磷营养盐的吸收。本研究明确了大叶藻对氮磷的吸收理论, 为建立我国海草床退化生境恢复技术提供了理论依据。致谢
马山集团有限公司为本研究的开展提供了大量帮助, 中国海洋大学的吴晓晓、许军阁、李洪辰、王仙宁、谢坤秀、张宏瑜和李超同学对本研究的样品采集与测定工作付出了辛勤劳动, 在此一并致谢。参考文献 原文顺序
文献年度倒序
文中引用次数倒序
被引期刊影响因子
[本文引用: 1]
[本文引用: 1]
[本文引用: 2]
[本文引用: 1]
[本文引用: 1]
DOI:10.3354/meps081163URL [本文引用: 1]
DOI:10.1016/j.jembe.2007.06.024URL [本文引用: 1]
DOI:10.1111/jec.2018.106.issue-1URL [本文引用: 1]
URL [本文引用: 1]

Ulva lactuca. Under nitrogen repletion, the maximum nitrate uptake rate of U. lactuca increased with increasing light intensity. At 30 ℃, the maximum uptake rate increased with increasing light intensity, but the Vmax/Km value reached the highest at medium light intensity. The optimal temperature for the nitrate uptake by U. lactuca was 20 ℃, and the nitrate uptake rate was much higher under nitrogen starved condition than under nitrogen replete condition. The nitrate uptake rate of U. lactuca was similar at 10 ℃ and 20 ℃. At 30 ℃, U. lactuca had the highest maximum uptake rate at medium light intensity. The increase of light intensity enhanced the nitrate uptake at 10 ℃ and 20 ℃, but hadless effect at 30 ℃. Nitrogen starved U. lactuca had a higher nitrate uptake rate than nitrogen replete U. lactuca. Even under nitrogen replete condition, U. lactuca could still take nitrate from the ambient environment.]]>
[本文引用: 1]
DOI:10.1016/j.aquabot.2017.01.007URL [本文引用: 1]
DOI:10.2307/1311969URL [本文引用: 1]
[本文引用: 1]
DOI:10.1016/0304-3770(94)00440-WURL [本文引用: 1]
DOI:10.1093/plankt/13.2.373URL [本文引用: 1]
DOI:10.3354/meps148187URL [本文引用: 1]
DOI:10.1016/S0304-3770(03)00069-XURL [本文引用: 3]
URLPMID:16788855 [本文引用: 1]
URLPMID:29745185 [本文引用: 1]
PMID:29745185 [本文引用: 1]
DOI:10.1007/s11104-007-9302-9URL [本文引用: 1]

An hydroponic experiment with a simulated water stress induced by PEG (6000) was conducted in a greenhouse to study the effects of nitrate (NO3 −), ammonium (NH4 +) and the mixture of NO3 − and NH4 +, on water stress tolerance of rice seedlings. Rice (Shanyou 63) was grown under non- or simulated water stress condition (10% (w/v) PEG, MW6000) with the 3 different N forms during 4weeks. Under non-stressed condition no difference was observed among the N treatments. Under simulated water stress, seedlings grown on N-NO3 − were stunted. Addition of PEG did not affect rice seedling growth in the treatment of only NH4 + supply but slightly inhibited the rice seedling growth in the treatment of mixed supply of NO3 − and NH4 +. Simulated water stress, when only N-NH4 + was present, did not affect leaf area and photosynthesis rate, however, both parameters decreased significantly in the NO3 − containing solutions. Under water stress, Rubisco content in newly expanded leaves significantly increased in the sole NH4 + supplied plants as compared to that in plants of the other two N treatments. Under water stress, the ratio of carboxylation efficiency to Rubisco content was, respectively, decreased by 13 and 23% in NH4 + and NO3 − treatments, respectively. It is concluded that, water stress influenced the Rubisco activity than stomatal limitation, and this effects could be regulated by N forms.]]>
DOI:10.1111/fec.2003.17.issue-6URL [本文引用: 1]
DOI:10.1007/BF02186230URL [本文引用: 1]
DOI:10.1111/j.1529-8817.1978.tb02467.xURL [本文引用: 1]
[本文引用: 1]
DOI:10.1007/BF00349788URL [本文引用: 1]
URL [本文引用: 1]

Fault structures of basement in the study area develop well. The large basement faults in EW, NEE and NE direcdons construct and control the principle frame of the regional geological structure on a grand scale. The faults in NS, NNW and NW directions are mostly tensile translational faults, which strongly cut apart, destroy and break the principle structure, form and control secondary differential regional tectonic movement and magmatism characteristics. The burial depth of magnetic basement in the edge rise zone of the East China Sea shelf is ordinarily 3-4 km. The basement comprises Pre-Miocene metamorphic rock and series of igneous rocks formed in different epoches. The crust belongs to continental crust, whose thickness is 24-28 km. The burial depth of magnetic basement in the southern Okinawa Trough basin is ordinarily 5-7 km. The basin can be divided into 4 secondary basins. The basement may mostly comprise basalt or part-metamorphic rock formed by trough tensile movement since Pre-Miocene, which may include old Pre-Tertiary stratigraphy close to continental slope foot. The crust belongs to suboceanic:rust, whose thickness is 15-20 km.
[本文引用: 1]
DOI:10.1016/j.jembe.2013.12.020URL [本文引用: 1]

Global climate change will impact the three dominate drivers of estuarine seagrass productivity, temperature, light and nutrients. The response of Pacific Northwest (PNW) Z. marina to interactive effects of temperature and nitrogen conditions was experimentally evaluated. Experimental manipulations were conducted hydroponically in acrylic chambers. Preliminary single factor experiments were conducted to evaluate physiological tolerances to temperature and nitrogen concentrations. Eelgrass exhibited a statistically significant linear increase in relative growth with increasing NH4 concentration (range from 5 to 1000 mu M); in contrast, there was no relationship between relative growth rate and increasing NO3 concentration. After 14 d there were no indications of plant mortality associated with NH4 or NO3. Leaf growth metrics exhibited strong statistically significant linear relationships with increasing water temperature (temperature range 4-25 degrees C), indicating broad thermal tolerance. In the factorial experiment, triplicate plants were exposed to 3 temperatures (10, 18 and 25 C) and 3 nitrate concentrations (10,30 and 100 mu M). Most metrics (leaf elongation, growth, relative growth, wasting index) exhibited a statistically significant temperature effect but no nutrient concentration effect, indicating the importance of temperature on metabolic rates. Plants at 18 and 25 C had significantly more wasting disease than plants at 10 C. Tissue delta N-15 and C:N values exhibited significant nutrient concentration and temperature effects, indicating that both factors influenced how much NO3 was incorporated into the tissues. Whole plant non structural carbohydrate content exhibited no significant response to either temperature or nitrate concentration. Temperature played a dominant role controlling Z. marina response to nutrients; nitrogen toxicity was not observed, and in general the effects of temperature and nutrients were not synergistic. This work highlights the complex nature of Z marina response to environmental conditions; additional multifactor experiments will be required to tease apart these interactions. Published by Elsevier B.V.
DOI:10.1001/archpsyc.1997.01830160065009URLPMID:9107150 [本文引用: 1]

Terms such as risk, risk factors, and especially the term cause are inconsistently and imprecisely used, fostering scientific miscommunication and misleading research and policy. Clarifying such terms is the essential first step. We define risk and a risk factor (protective factor) and their potency, set out the conceptual basis of the methods by which risk factors are identified and potency demonstrated, and propose criteria for establishing the status of a risk factor as a fixed or variable marker or a causal risk factor. All definitions are based on the state of scientific knowledge (empirical documentation), rather than on hypotheses, speculations, or beliefs. We discuss common approaches and pitfalls and give a psychiatric research example. Imprecise reports can impede the search for understanding the cause and course of any disease and also may be a basis of inadequate clinical or policy decision-making. The issues in risk research are much too important to tolerate less than precise terminology or the less than rigorous research reporting that results from imprecise and inconsistent terminology.
DOI:10.1111/jpy.1993.29.issue-4URL [本文引用: 1]
DOI:10.1016/0272-7714(91)90021-3URL [本文引用: 1]
DOI:10.1016/j.jembe.2007.06.016URL [本文引用: 2]
DOI:10.4319/lo.1999.44.5.1204URL [本文引用: 4]
DOI:10.3354/meps196039URL [本文引用: 1]
DOI:10.1002/aqc.v29.9URL [本文引用: 1]
[本文引用: 1]
[本文引用: 1]
[本文引用: 1]
[本文引用: 1]
[本文引用: 2]
[本文引用: 2]
URL [本文引用: 2]

A total of 862 biobenthic species are found in the intertidal zone Fujian islands,which belong to 734 species of animals,128 species of algae.Jaccard's similarity index of species is measured,and the distribution of the benthic community is studied using hierarchical clustering(WPGMA) and polarordination.The result indicates that the benthic communities are divided into three classifications:the estuary type,harbour type and wide sea type of the community.The distribution of the benthic community is related to the island location and its environment.The salinity and motive power of the water are major factors which influence the community.
[本文引用: 2]
[本文引用: 1]
[本文引用: 1]
DOI:10.1007/s002270050494URL [本文引用: 1]
DOI:10.1016/j.marchem.2004.06.021URL [本文引用: 1]
DOI:10.1016/0304-3770(79)90006-8URL [本文引用: 1]
DOI:10.4319/lo.2006.51.1.0208URL [本文引用: 2]
DOI:10.2307/1350754URL [本文引用: 1]
DOI:10.1016/0304-3770(84)90080-9URL [本文引用: 1]
DOI:10.1016/S0304-3770(96)01100-XURL [本文引用: 2]
DOI:10.1016/j.marpolbul.2017.09.034URLPMID:28964500 [本文引用: 1]

Seedlings are a key life stage in seagrasses, providing genetic diversity and being a useful tool for restoration. We examined the influence of increased sediment nutrients and the presence of the invasive macroalga Caulerpa cylindracea on the success of in situ transplanting Posidonia oceanica seedlings in a six-month experiment. Our results indicate that one-year old seedlings successfully survive in the field and their survival and growth are positively affected by the presence of C. cylindracea. Furthermore, nutrient addition in the sediment had positive effects on both C. cylindracea (increasing its cover) and seedlings (increasing leaf development), and the increased C. cylindracea cover did not result in detrimental effects on seedlings. Therefore, biological invasions and nutrient addition do not reinforce each other in the short term to negatively impact transplanted seedlings, which highlights facilitative interactions between invasive algae and native seagrass and provides useful information for successful strategies of seagrass restoration.
URLPMID:31978099 [本文引用: 1]
DOI:10.1016/0304-3770(78)90012-8URL [本文引用: 1]
DOI:10.1016/j.ecoleng.2006.05.014URL [本文引用: 1]

AbstractRemoval of phosphorus (P) by Ceratophyllum demersum L. and associated epiphytic periphyton was quantified by measuring the disappearance of soluble reactive P (SRP) from microcosms during 1-h in situ incubations conducted over a 1-year period. Initial P concentrations in these incubations ranged from 30 to >10,000 μg P L−1. Phosphorus removal was proportional to initial P concentrations and was weakly correlated with solar irradiance and water temperature. Removal rates (0.6–32.8 mg P m−2 d−1) and kv coefficients (0.68–1.93 h−1) from experiments run at low initial P concentrations (up to 200 μg P L−1) were comparable to results reported for other macrophytes. Removal rates from experiments run at the highest (>10,000 μg P L−1) initial P concentrations (5300 and 11,100 mg P m−2 d−1) most likely represented luxury nutrient consumption and were not thought to be sustainable long term. We were unable to determine a Vmax for P removal, suggesting that the nutrient-storage capability of the C. demersum/periphyton complex was not saturated during our short-term incubations. Based on N:P molar ratios, the marsh was P limited, while the C. demersum/periphyton complex was either N limited or in balance for N and P throughout this study. However, despite its tissue stoichiometry, the C. demersum/periphyton complex always exhibited an affinity for P. It appeared that the biochemical mechanisms, which mediate P removal, at least on a short-term basis, were more influenced by increases in ambient P levels than by tissue nutrient stoichiometry.]]>
[本文引用: 1]
DOI:10.3390/ijms19061570URL [本文引用: 4]
DOI:10.1007/s12237-019-00523-3URL [本文引用: 1]
DOI:10.1016/j.biocon.2011.04.010URL [本文引用: 2]

Seagrasses, a functional group of marine flowering plants rooted in the world's coastal oceans, support marine food webs and provide essential habitat for many coastal species, playing a critical role in the equilibrium of coastal ecosystems and human livelihoods. For the first time, the probability of extinction is determined for the world's seagrass species under the Categories and Criteria of the International Union for the Conservation of Nature (IUCN) Red List of Threatened Species. Several studies have indicated that seagrass habitat is declining worldwide. Our focus is to determine the risk of extinction for individual seagrass species, a 4-year process involving seagrass experts internationally, compilation of data on species' status, populations, and distribution, and review of the biology and ecology of each of the world's seagrass species. Ten seagrass species are at elevated risk of extinction (14% of all seagrass species), with three species qualifying as Endangered. Seagrass species loss and degradation of seagrass biodiversity will have serious repercussions for marine biodiversity and the human populations that depend upon the resources and ecosystem services that seagrasses provide. (C) 2011 Elsevier Ltd.
DOI:10.3354/meps134195URL [本文引用: 2]
DOI:10.1007/s002270050083URL [本文引用: 1]
DOI:10.3354/meps149267URL [本文引用: 2]
DOI:10.1007/BF03161516URL [本文引用: 1]
DOI:10.1007/BF00396910URL [本文引用: 1]
DOI:10.1007/BF00393086URL [本文引用: 1]
[本文引用: 1]
[本文引用: 1]
DOI:10.1016/s0022-0981(00)00195-7URLPMID:10969167 [本文引用: 1]

Within the past few decades, major losses of seagrass habitats in coastal waters impacted by cultural eutrophication have been documented worldwide. In confronting a pressing need to improve the management and protection of seagrass meadows, surprisingly little is known about the basic nutritional physiology of these critical habitat species, or the physiological mechanisms that control their responses to N and P gradients. The limited available evidence to date already has revealed, for some seagrass species such as the north temperate dominant Zostera marina, unusual responses to nutrient enrichment in comparison to other vascular plants. Seagrasses derive N and P from sediment pore water (especially ammonium) and the water column (most nitrate). The importance of leaves versus roots in nutrient acquisition depends, in part, on the enrichment conditions. For example, a shift from reliance on sediment pore water to increased reliance on the overlying water for N and P supplies has been observed under progressive water-column nutrient enrichment. Seagrasses may be N-limited in nutrient-poor waters with sandy or (less so) organic sediments, and P-limited in carbonate sediments. On the basis of data from few species, seagrasses appear to have active uptake systems for NO(3)(-) and PO(4)(-3), but NH(4)(+) uptake may involve both low- and high-affinity systems. P(i) uptake affinities reported thus far are much lower than values for active ammonium uptake, but comparable to values for nitrate uptake by leaf tissues. Beyond such basic information, seagrass species have shown considerable variation in nutritional response. Dominance of acropetal versus basipetal nutrient translocation appears to vary among species as an innate trait. While some species follow classic Michaelis-Menten kinetics for N(i) uptake, others have exhibited sustained linear uptake with limited or negligible product feedback inhibition, perhaps in adaptation to oligotrophic environments. Zostera marina also is able to maintain nitrate reductase (NR) activity during dark periods if adequate carbohydrate reserves and substrate are available. Thus, this species can respond to nitrate pulses throughout a diel cycle, rather than being limited as most plants to nitrate uptake during the light period. Further adaptations may have occurred for seagrasses in extremely nitrate-depauperate conditions. For example, Halophila decipiens and H. stipulacea lack inducible NR and apparently have lost the ability to reduce nitrate; and a biphasic rather than hyperbolic P(i) uptake curve, with 'surge' uptake, has been described for Zostera noltii. Many seagrasses respond favorably to low or moderate N and/or P enrichment. However, excessive N(i) loading to the water column can inhibit seagrass growth and survival, not only as an indirect effect by stimulating algal overgrowth and associated light reduction, but-for some species-as a direct physiological effect. The latter direct impact has been most pronounced for plants growing in sandy (nutrient-poor) sediments, and is exacerbated by elevated temperatures and/or light reduction. Ammonia toxicity, known for many vascular plants, has been reported in seagrasses Ruppia drepanensis and Z. marina (125 microM water-column NH(4)(+), 5 weeks). Z. marina has shown to be inhibited, as well, by pulsed water-column nitrate enrichment (as low as 3.5-7 microM NO(3)(-), 3-5 weeks), which is actively taken up without apparent product feedback inhibition. Inhibition by elevated nitrate has also been reported, with description of the underlying physiological mechanisms, in certain macroalgae and microalgae. In Z. marina, this effect has been related to the high, sustained energy demands of nitrate uptake, and to inducement of internal carbon limitation by the concomitant 'carbon drain' into amino acid assimilation. In contrast, nitrate enrichment can stimulate growth of Z. marina when the sediment, rather than the water column, is the source. Because seagrass species have shown considerable variation in nutritional response, inferences about one well-studied species, from one geographic location, should not be applied a priori to that species in other regions or to seagrasses in general. Most of the available information has been obtained from study of a few species, and the basic nutritional physiology of many seagrasses remains to be examined and compared across geographic regions. Nonetheless, the relatively recent gains in general understanding about the physiological responses of some seagrass species to nutrient gradients already have proven valuable in both basic and applied research. For example, physiological variables such as tissue C:N:P content have begun to be developed as integrative indicators of nutrient conditions and anthropogenic nutrient enrichment. To strengthen insights for management strategies to optimize seagrass survival in coastal waters adjacent to exponential human population growth and associated nutrient inputs, additional emphasis is critically needed to assess the role of variable interactions-among inorganic as well as organic N, P and C, environmental factors such as temperature, light, and other community components-in controlling the physiology, growth and survival of these ecologically important marine angiosperms.
DOI:10.1111/j.0022-3646.1991.00014.xURL [本文引用: 1]
DOI:10.1016/S0022-0981(97)00060-9URL [本文引用: 1]
DOI:10.1111/conl.2019.12.issue-1URL [本文引用: 1]
DOI:10.3354/meps09054URL [本文引用: 1]

Dissolved organic nitrogen (DON) acts as a large reservoir of fixed nitrogen. Whereas DON utilization is common in the microbial community, little is known about utilization by macrophytes. We investigated the ability of the coexisting temperate marine macrophytes Zostera noltii, Cymodocea nodosa, and Caulerpa prolifera to take up nitrogen and carbon from small organic substrates of different molecular complexities (urea, glycine, L-leucine, and L-phenylalanine) and from dissolved organic matter (DOM) derived from algal and bacterial cultures (substrates with a complex composition). In addition to inorganic nitrogen, nitrogen from small organic substrates could be taken up in significant amounts by all macrophytes. Substrate uptake by the aboveground tissue differed from that of the belowground tissue. No relationships between carbon and nitrogen uptake of small organics were found. The preference for individual organic substrates was related to their structural complexity and C:N ratio. Uptake of algae-derived organic nitrogen was of similar magnitude as inorganic nitrogen, and was preferred over bacteria-derived nitrogen. These results add to the growing evidence that direct or quick indirect DON utilization may be more widespread among aquatic macrophytes than traditionally thought.
DOI:10.3354/meps190155URL [本文引用: 1]
DOI:10.3354/meps157159URL [本文引用: 2]
DOI:10.3354/meps11435URL [本文引用: 1]
DOI:10.4319/lo.2008.53.2.0542URL [本文引用: 1]
[本文引用: 1]
[本文引用: 1]
DOI:10.1073/pnas.0905620106URLPMID:19587236 [本文引用: 1]

Coastal ecosystems and the services they provide are adversely affected by a wide variety of human activities. In particular, seagrass meadows are negatively affected by impacts accruing from the billion or more people who live within 50 km of them. Seagrass meadows provide important ecosystem services, including an estimated $1.9 trillion per year in the form of nutrient cycling; an order of magnitude enhancement of coral reef fish productivity; a habitat for thousands of fish, bird, and invertebrate species; and a major food source for endangered dugong, manatee, and green turtle. Although individual impacts from coastal development, degraded water quality, and climate change have been documented, there has been no quantitative global assessment of seagrass loss until now. Our comprehensive global assessment of 215 studies found that seagrasses have been disappearing at a rate of 110 km(2) yr(-1) since 1980 and that 29% of the known areal extent has disappeared since seagrass areas were initially recorded in 1879. Furthermore, rates of decline have accelerated from a median of 0.9% yr(-1) before 1940 to 7% yr(-1) since 1990. Seagrass loss rates are comparable to those reported for mangroves, coral reefs, and tropical rainforests and place seagrass meadows among the most threatened ecosystems on earth.
DOI:10.1016/0022-0981(89)90061-0URL [本文引用: 1]
DOI:10.3724/SP.J.00001URL [本文引用: 1]

Gracilaria asiatica with different culture densities were determined, and dynamics character of ammonium nitrogen uptaking at N-limited and the N-replete situation and the effect of different initial concentration of ammonium nitrogen on absorption rate were studied. Gracilaria asiatica was collected at Xiangshan harbor, Zhejiang province, southern China, and cultivated in the laboratory. Samples of the alga were maintained in a flask with 250 mL seawater of 21 salinity. The alga were cultured in culture boxes at (20±1) ℃, under 60 μmol·m-2·s-1 illumination, and on 12 h:12 h light/dark cycle.The result showed that the ability of ammonium nitrogen removing was enhanced by seaweed culture density (in 2-24 g·L-1) and experiment time (in 5 hours) increasing. The highest efficiency of ammonium nitrogen removing with highest density (24 g·L-1) of G. asiatica was up to 99.77% after 5 hours. Ammonium nitrogen concentration declined from 300 μmol·L-1 to zero. The ammonium nitrogen removing efficiency with lowest density (2 g·L-1) was only 20%. Higher ammonium nitrogen absorption rate (30-41 μmol·g-1·h-1) was kept in different density groups during initial culture period, especially in 16 g·L-1 density group, then decreased with density increase. The maximum uptake rates reached as high as 28.33 μmol·g-1h-1and 18.85 μmol·g-1·h-1 at 3 h and 5 h, respectively, in 2 g·L-1 minimum density group. The lowest ammonium nitrogen uptake rate of 11.70 μmol·g-1·h-1 and 1.90 μmol·g-1·h-1 were obtained at 3 h and 5 h with the highest density group (24g/L). In initial concentration experiment, maximum uptake rates (Vmax) and half-saturation constant(Km) of G. asiatica at N-limited and the N-replete situation reached the highest value, 116.47, 159.40 μmol·g-1·h-1 and 439.70, 913.61 μmol·L-1 in the first hour, then they declined from 24.29, 23.22 μmol·g-1h-1 and 166.87, 255.30 μmol·L-1 with culture prolonging from 1 h to 5 h. In initial concentration experiment, ammonium nitrogen removing efficiency were increased with initial concentration reduced in the range of 100-300 μmol·L-1, especially with lowest ammonium nitrogen concentration (100 μmol·L-1). Ammonium nitrogen removing efficiency kept in 56.7%-67.4% with 300-500 μmol·L-1 of ammonium nitrogen concentration. There was no difference for ammonium nitrogen absorption of G. asiatica at between N-limited and the N-replete situation. Within 300-500 μmol·L-1 of ammonium nitrogen concentration, a fast absorption rate of G. asiatica at N-half hungry situation presented in the first stage(40.7-102.1 μmol·g-1·h-1), and almost had a positive relation with concentration, which was not fit the Micheal dynamics saturation equations. But, within 100-200 μmol·L-1 of ammonium nitrogen concentration, the uptake rates of G. asiatica were as low as 17.8-40.8 μmol·g-1·h-1. When the concentration decreased in some limited range, the absorption rate reached the maximum and fit the Micheal dynamics saturation equations. It would provide important theory data for cleaning water and bioremediation by cultivating G. asiatica, and large-scale cultivation of G. asiatica could be a good solution to the problem of eutrophication due to their capability of removing nutrients.]]>
[本文引用: 1]
[本文引用: 1]
[本文引用: 1]
DOI:10.3724/SP.J.1003.2013.10038URL [本文引用: 2]

Posidonia australis in Hainan is in factEnhalus acoroides. From our analyses, two Chinese seagrass biotas are proposed. These include the South China Sea Bioregion (SCSBR) and China’s Yellow Sea and Bohai Sea Bioregion (CYSBSBR). The SCSBR includes Hainan, Guangxi, Guangdong, Hongkong, Taiwan and Fujian provinces, and contains 15 seagrass species representing nine genera with Halophila ovalis being most widely distributed. The CYSBSBR includes Shandong, Hebei, Tianjin and Liaonin provinces and contains nine seagrass species belonging to three genera with Zostera marina being most widely distributed. The total distribution area for China’s seagrass meadows is estimated to be 8,765.1 ha, with Hainan, Guangdong and Guangxi provinces accounting for 64%, 11% and 10% of the area, respectively. Both the number and area of seagrass meadows are much higher in the SCSBR than in the CYSBSBR. In the SCSBR, seagrass meadows are mainly located in the eastern Hainan coast, Zhanjiang in Guangdong, Beihai in Guangxi and Dongsha Island in Taiwan, whereas in the CYSBSBR they predominate in Rongcheng in Shandong and Changhai in Liaoning. Halophila ovalis, Thalassia hemprichii and Z. marina are the dominated species in seagrass meadows in Guangdong and Guangxi, Hainan and Taiwan, Shandong and Liaoning respectively. Seagrass degradation in China is mainly attributed to human disturbances caused by fishing, aquaculture and sea reclamation. For conservation purposes we advise the following: (1) initiate an extensive national survey of spatial patterns of seagrass species diversity; (2) conduct long-term monitoring of typical seagrass meadows and establish a national seagrass monitoring network; (3) accelerate legislation for seagrass conservation and include some ecologically-significant seagrass meadows as reserves; (4) invest more finance in research on the restoration of seagrass beds and conservation of seagrass germplasm resources; (5) standardize the Chinese names of seagrassesin China.]]>
[本文引用: 2]
Annual growth dynamics of Posidonia oceanica: contribution of large- scale versus local factors to seasonality
1
1995
... 同时, 在海草体内存在营养转移的现象, 氮磷在海草不同叶龄结构的叶片间转移可以提高叶片对氮磷的利用效率, 进而促进海草的生长(
Dissolved organic nitrogen: a relevant, complementary source of nitrogen for the seagrass Zostera marina
1
2015
... 陆生高等植物主要以硝态氮和氨态氮作为无机氮素的来源, 但更多的是吸收硝态氮, 只有在某些还原性较强的土壤或者水淹地, 其主要的氮源才可能是氨态氮(
The maximum nitrate reductase activity of the seagrass Zostera noltii(Hornem.) varies along its vertical distribution
2
2004
... 陆生高等植物主要以硝态氮和氨态氮作为无机氮素的来源, 但更多的是吸收硝态氮, 只有在某些还原性较强的土壤或者水淹地, 其主要的氮源才可能是氨态氮(
... 尽管已有研究表明, 海草既可以通过根系从沉积物中吸收氮磷, 也可以通过叶片从海水中获取, 但多数海草种类的叶片是吸收氮磷的主要部位(
Nutrient, oxygen and carbon ratios, CO2 sequestration and anthropogenic forcing in the Mediterranean Sea//Saliot A
1
2005
... 在米氏方程中, Vmax表示底物饱和时的吸收速率, 越大则吸收的内在潜力越大, Km表示当吸收速率为最大吸收速率一半时的底物浓度, Km值越小, 对底物亲和力越大(
Assessing the toxicity of ammonium pulses to the survival and growth of Zostera noltii
1
2002
... 陆生高等植物主要以硝态氮和氨态氮作为无机氮素的来源, 但更多的是吸收硝态氮, 只有在某些还原性较强的土壤或者水淹地, 其主要的氮源才可能是氨态氮(
Water- column nitrate enrichment promotes decline of eelgrass Zostera marina: evidence from seasonal mesocosm experiments
1
1992
... 陆生高等植物主要以硝态氮和氨态氮作为无机氮素的来源, 但更多的是吸收硝态氮, 只有在某些还原性较强的土壤或者水淹地, 其主要的氮源才可能是氨态氮(
Seagrasses and eutrophication
1
2007
... 陆生高等植物主要以硝态氮和氨态氮作为无机氮素的来源, 但更多的是吸收硝态氮, 只有在某些还原性较强的土壤或者水淹地, 其主要的氮源才可能是氨态氮(
Herbivore community determines the magnitude and mechanism of nutrient effects on subtropical and tropical seagrasses
1
2018
... 大叶藻在寡营养盐条件下具有明显的竞争优势, 减少氮营养盐的输入则可以明显促进退化海草床的自我恢复(
光照和温度对氮饥饿及饱和营养条件下石莼(Ulva lactuca)的硝态氮吸收动力学影响
1
2010
... 此外, 植株的吸收动力学特征受到环境光照、温度和盐度等环境条件的影响.光照的影响主要体现在植株的光合作用和酶活性等方面, 如有研究表明, 水生植物在光照条件下对营养盐的吸收速率远大于其在黑暗条件下的吸收速率, 光照还能激活水生植物体内的碱性磷酸酶活性, 为植物吸收利用磷酸盐提供条件(
光照和温度对氮饥饿及饱和营养条件下石莼(Ulva lactuca)的硝态氮吸收动力学影响
1
2010
... 此外, 植株的吸收动力学特征受到环境光照、温度和盐度等环境条件的影响.光照的影响主要体现在植株的光合作用和酶活性等方面, 如有研究表明, 水生植物在光照条件下对营养盐的吸收速率远大于其在黑暗条件下的吸收速率, 光照还能激活水生植物体内的碱性磷酸酶活性, 为植物吸收利用磷酸盐提供条件(
Plasticity in turtle grass (Thalassia testudinum) flower production as a response to porewater nitrogen availability
1
2017
... 大叶藻在寡营养盐条件下具有明显的竞争优势, 减少氮营养盐的输入则可以明显促进退化海草床的自我恢复(
Assessing water quality with submersed aquatic vegetation: habitat requirements as barometers of Chesapeake Bay health
1
1993
... 海草床生态系统较脆弱, 受全球变化和人类活动影响, 目前已是地球生物圈退化速率最快的生态系统, 且退化速率仍在逐年增加(
Seagrass nutrient content
1
1990
... 氮磷是水生植物最主要的限制性营养盐, ****普遍使用米氏方程来表示稳态时水生植物对营养盐的吸收特征(
Mass balance constraints on nutrient cycling in tropical seagrass beds
1
1995
... 也有部分海草并非依赖地上组织吸收氮磷营养盐.如
Algal carbon-nitrogen metabolism: a biochemical basis for modelling the interactions between nitrate and ammonium uptake
1
1991
...
Dynamic model of phytoplankton growth and acclimation: responses of the balanced growth rate and the chlorophyll a:carbon ratio to light, nutrient-limitation and temperature
1
1997
... 氮磷是水生植物最主要的限制性营养盐, ****普遍使用米氏方程来表示稳态时水生植物对营养盐的吸收特征(
Phosphorus uptake kinetics of a dominant tropical seagrass Thalassia testudinum
3
2003
... 氮磷是水生植物最主要的限制性营养盐, ****普遍使用米氏方程来表示稳态时水生植物对营养盐的吸收特征(
... 尽管已有研究表明, 海草既可以通过根系从沉积物中吸收氮磷, 也可以通过叶片从海水中获取, 但多数海草种类的叶片是吸收氮磷的主要部位(
... -P的最大吸收速率是地下组织的3.3倍(
Toward reversal of eutrophic conditions in a subtropical estuary: water quality and seagrass response to nitrogen loading reductions in Tampa bay, Florida, USA
1
2006
... 大叶藻在寡营养盐条件下具有明显的竞争优势, 减少氮营养盐的输入则可以明显促进退化海草床的自我恢复(
鳗草在荣成天鹅湖不同生境中生长的适应性
1
2017
... 海草床生态系统较脆弱, 受全球变化和人类活动影响, 目前已是地球生物圈退化速率最快的生态系统, 且退化速率仍在逐年增加(
鳗草在荣成天鹅湖不同生境中生长的适应性
1
2017
... 海草床生态系统较脆弱, 受全球变化和人类活动影响, 目前已是地球生物圈退化速率最快的生态系统, 且退化速率仍在逐年增加(
Ammonium nutrition increases photosynthesis rate under water stress at early development stage of rice (Oryza sativa L.)
1
2007
... 陆生高等植物主要以硝态氮和氨态氮作为无机氮素的来源, 但更多的是吸收硝态氮, 只有在某些还原性较强的土壤或者水淹地, 其主要的氮源才可能是氨态氮(
Contrasting effects of nitrogen, phosphorus and water regime on first- and second-year growth of 16 wetland plant species
1
2003
... 氮磷是水生植物最主要的限制性营养盐, ****普遍使用米氏方程来表示稳态时水生植物对营养盐的吸收特征(
Outdoor pond cultivation of the subtropical marine red algaGracilaria tenuistipitata in brackish water in Sweden. Growth, nutrient uptake, co-cultivation with rainbow trout and epiphyte control
1
1993
... 大叶藻在寡营养盐条件下具有明显的竞争优势, 减少氮营养盐的输入则可以明显促进退化海草床的自我恢复(
Uptake of inorganic nitrogen by Codium fragile subsp. tomentosoides(chlorophyta)
1
2010
... 在米氏方程中, Vmax表示底物饱和时的吸收速率, 越大则吸收的内在潜力越大, Km表示当吸收速率为最大吸收速率一半时的底物浓度, Km值越小, 对底物亲和力越大(
1
2000
... 海草床生态系统较脆弱, 受全球变化和人类活动影响, 目前已是地球生物圈退化速率最快的生态系统, 且退化速率仍在逐年增加(
The nitrogen supply to intertidal eelgrass (Zostera marina)
1
1994
... 尽管已有研究表明, 海草既可以通过根系从沉积物中吸收氮磷, 也可以通过叶片从海水中获取, 但多数海草种类的叶片是吸收氮磷的主要部位(
溶解态磷在海洋微藻碱性磷酸酶活力变化中的调控作用
1
1999
... 此外, 植株的吸收动力学特征受到环境光照、温度和盐度等环境条件的影响.光照的影响主要体现在植株的光合作用和酶活性等方面, 如有研究表明, 水生植物在光照条件下对营养盐的吸收速率远大于其在黑暗条件下的吸收速率, 光照还能激活水生植物体内的碱性磷酸酶活性, 为植物吸收利用磷酸盐提供条件(
溶解态磷在海洋微藻碱性磷酸酶活力变化中的调控作用
1
1999
... 此外, 植株的吸收动力学特征受到环境光照、温度和盐度等环境条件的影响.光照的影响主要体现在植株的光合作用和酶活性等方面, 如有研究表明, 水生植物在光照条件下对营养盐的吸收速率远大于其在黑暗条件下的吸收速率, 光照还能激活水生植物体内的碱性磷酸酶活性, 为植物吸收利用磷酸盐提供条件(
Effect of temperature and nutrient manipulations on eelgrass Zostera marina L. from the Pacific Northwest, USA
1
2014
... 海草床生态系统较脆弱, 受全球变化和人类活动影响, 目前已是地球生物圈退化速率最快的生态系统, 且退化速率仍在逐年增加(
Coming to terms with the terms of risk
1
1997
... 尽管已有研究表明, 海草既可以通过根系从沉积物中吸收氮磷, 也可以通过叶片从海水中获取, 但多数海草种类的叶片是吸收氮磷的主要部位(
Sodium-dependent nitrate transport and energetics of cyanobacteria
1
1993
... 在米氏方程中, Vmax表示底物饱和时的吸收速率, 越大则吸收的内在潜力越大, Km表示当吸收速率为最大吸收速率一半时的底物浓度, Km值越小, 对底物亲和力越大(
Macroalgal-sediment nutrient interactions and their importance to macroalgal nutrition in a eutrophic estuary
1
1991
... 在米氏方程中, Vmax表示底物饱和时的吸收速率, 越大则吸收的内在潜力越大, Km表示当吸收速率为最大吸收速率一半时的底物浓度, Km值越小, 对底物亲和力越大(
Effects of irradiance, temperature, and nutrients on growth dynamics of seagrasses: a review
2
2007
... 海草床生态系统较脆弱, 受全球变化和人类活动影响, 目前已是地球生物圈退化速率最快的生态系统, 且退化速率仍在逐年增加(
... 在对澳大利亚、古巴、美国等17个国家或地区的15种海草种类进行研究后分别得出了不同地区不同海草种类的最小需光量(%);
Inorganic nitrogen acquisition in the seagrass Thalassia testudinum: development of a whole-plant nitrogen budget
4
1999
... Uptake kinetics of NH4+-N, NO3--N and PO43--P by seagrasses and seaweeds
种类 Species | 研究地点 Study area | Vmax (μmol·g-1·h-1) | Km (μmol·L-1) | 文献 Reference | ||||
---|---|---|---|---|---|---|---|---|
NH4+-N | NO3--N | PO43--P | NH4+-N | NO3--N | PO43--P | |||
大叶藻 Zostera marina | 山东荣成天鹅湖 Tian’e Lake, Rongcheng, Shandong China (37.35° N, 122.57° E) | 51.8 | 39.1 | 27.9 | 68.1 | 68.6 | 24.8 | 本研究 This study |
美国罗德岛 Rhode Island, USA (41.30° N, 71.30° W) | 48 | |||||||
Phyllospadix torreyi | 美国拉由拉市 La Jolla, USA (32.48° N, 117.16° W) | 96-204 | 25-75 | 9-34 | 4-17 | |||
泰来藻 Thalassia hemprichii | 印度尼西亚斯佩蒙德群岛 Spermonde Archipelago, Indonesia (5.03° S, 119.20° E) | 32-37 | 21-60 | |||||
Thalassia testudinum | 美国圣体湾和马德雷湖 Corpus Christi Bay (27.49° N, 97.07° W) & Laguna Madre, USA (26.09° N, 97.12° W) | 8-16 | 4-7 | 8-15 | 2-39 | |||
Posidonia oceanica | 西班牙马拉加 Málaga, Spain (36.40° N, 4.21° W) | 8.7 | 5.8 | |||||
真江蓠 Gracilari asiatica | 浙江奉化象山港 Xiangshan Harbor, Fenghua, China (29.11° N, 122.01° E) | 159.4 | ||||||
龙须菜 Asparagus schoberioides | 福建东山岛 Dongshan Island, Fujian China (23.36° N, 117.14° E) | 3.1 | ||||||
浒苔 Ulva prolifera | 山东青岛汇泉湾 Huiquan Bay, Qingdao, Shandong China (36.03° N, 123.20° E) | 250.4 | 5.8 |
... 此外, 植株的吸收动力学特征受到环境光照、温度和盐度等环境条件的影响.光照的影响主要体现在植株的光合作用和酶活性等方面, 如有研究表明, 水生植物在光照条件下对营养盐的吸收速率远大于其在黑暗条件下的吸收速率, 光照还能激活水生植物体内的碱性磷酸酶活性, 为植物吸收利用磷酸盐提供条件(
... 尽管已有研究表明, 海草既可以通过根系从沉积物中吸收氮磷, 也可以通过叶片从海水中获取, 但多数海草种类的叶片是吸收氮磷的主要部位(
... 也有部分海草并非依赖地上组织吸收氮磷营养盐.如
Effects of nitrogen enrichment on biomass allocation, growth, and leaf morphology of the seagrass Thalassia testudinum
1
2000
... 同时, 在海草体内存在营养转移的现象, 氮磷在海草不同叶龄结构的叶片间转移可以提高叶片对氮磷的利用效率, 进而促进海草的生长(
Implications of nutrient enrichment for the conservation and management of seagrass Zostera muelleri meadows
1
2019
... 大叶藻在寡营养盐条件下具有明显的竞争优势, 减少氮营养盐的输入则可以明显促进退化海草床的自我恢复(
海草场的生态功能
1
2009
... 海草是一类海洋高等单子叶开花植物, 具有极高的初级生产力, 在我国主要分布于温带的山东、辽宁、河北, 亚热带、热带的广东、广西和海南等沿岸海域(
海草场的生态功能
1
2009
... 海草是一类海洋高等单子叶开花植物, 具有极高的初级生产力, 在我国主要分布于温带的山东、辽宁、河北, 亚热带、热带的广东、广西和海南等沿岸海域(
盐度、光照和营养盐对孔石莼(Ulva pertusa)光合作用的影响
1
2001
... 此外, 植株的吸收动力学特征受到环境光照、温度和盐度等环境条件的影响.光照的影响主要体现在植株的光合作用和酶活性等方面, 如有研究表明, 水生植物在光照条件下对营养盐的吸收速率远大于其在黑暗条件下的吸收速率, 光照还能激活水生植物体内的碱性磷酸酶活性, 为植物吸收利用磷酸盐提供条件(
盐度、光照和营养盐对孔石莼(Ulva pertusa)光合作用的影响
1
2001
... 此外, 植株的吸收动力学特征受到环境光照、温度和盐度等环境条件的影响.光照的影响主要体现在植株的光合作用和酶活性等方面, 如有研究表明, 水生植物在光照条件下对营养盐的吸收速率远大于其在黑暗条件下的吸收速率, 光照还能激活水生植物体内的碱性磷酸酶活性, 为植物吸收利用磷酸盐提供条件(
海藻的营养代谢及其对主要营养盐的吸收动力学
2
2001
... 氮磷是水生植物最主要的限制性营养盐, ****普遍使用米氏方程来表示稳态时水生植物对营养盐的吸收特征(
...
海藻的营养代谢及其对主要营养盐的吸收动力学
2
2001
... 氮磷是水生植物最主要的限制性营养盐, ****普遍使用米氏方程来表示稳态时水生植物对营养盐的吸收特征(
...
温度和盐度对几种大型海藻生长率和NH4-N吸收的影响
2
2001
... 在米氏方程中, Vmax表示底物饱和时的吸收速率, 越大则吸收的内在潜力越大, Km表示当吸收速率为最大吸收速率一半时的底物浓度, Km值越小, 对底物亲和力越大(
... 此外, 植株的吸收动力学特征受到环境光照、温度和盐度等环境条件的影响.光照的影响主要体现在植株的光合作用和酶活性等方面, 如有研究表明, 水生植物在光照条件下对营养盐的吸收速率远大于其在黑暗条件下的吸收速率, 光照还能激活水生植物体内的碱性磷酸酶活性, 为植物吸收利用磷酸盐提供条件(
温度和盐度对几种大型海藻生长率和NH4-N吸收的影响
2
2001
... 在米氏方程中, Vmax表示底物饱和时的吸收速率, 越大则吸收的内在潜力越大, Km表示当吸收速率为最大吸收速率一半时的底物浓度, Km值越小, 对底物亲和力越大(
... 此外, 植株的吸收动力学特征受到环境光照、温度和盐度等环境条件的影响.光照的影响主要体现在植株的光合作用和酶活性等方面, 如有研究表明, 水生植物在光照条件下对营养盐的吸收速率远大于其在黑暗条件下的吸收速率, 光照还能激活水生植物体内的碱性磷酸酶活性, 为植物吸收利用磷酸盐提供条件(
营养盐富集和全球温度升高对海草的影响
1
2017
... 海草对营养盐的吸收还受到环境营养盐浓度的影响.当环境处于低营养盐状态时, 海草则增加从 底质吸收营养盐的比例, 以适应低营养盐环境(
营养盐富集和全球温度升高对海草的影响
1
2017
... 海草对营养盐的吸收还受到环境营养盐浓度的影响.当环境处于低营养盐状态时, 海草则增加从 底质吸收营养盐的比例, 以适应低营养盐环境(
Interactions between NH4+ and NO3- uptake and assimilation: comparison of diatoms and dinoflagellates at several growth temperatures
1
1999
... 此外, 植株的吸收动力学特征受到环境光照、温度和盐度等环境条件的影响.光照的影响主要体现在植株的光合作用和酶活性等方面, 如有研究表明, 水生植物在光照条件下对营养盐的吸收速率远大于其在黑暗条件下的吸收速率, 光照还能激活水生植物体内的碱性磷酸酶活性, 为植物吸收利用磷酸盐提供条件(
Amino acid carbon isotopic fractionation patterns in oceanic dissolved organic matter: an unaltered photoautotrophic source for dissolved organic nitrogen in the ocean?
1
2004
... 陆生高等植物主要以硝态氮和氨态氮作为无机氮素的来源, 但更多的是吸收硝态氮, 只有在某些还原性较强的土壤或者水淹地, 其主要的氮源才可能是氨态氮(
Growth and organic production of eelgrass (Zostera marina L.) in temperate waters of the pacific coast of Japan. I. Growth analysis in spring-summer
1
1979
... 海草对营养盐的吸收还受到环境营养盐浓度的影响.当环境处于低营养盐状态时, 海草则增加从 底质吸收营养盐的比例, 以适应低营养盐环境(
Thalassia testudinum phosphate uptake kinetics at low in situ concentrations using a 33P radioisotope technique
2
2006
... 氮磷是水生植物最主要的限制性营养盐, ****普遍使用米氏方程来表示稳态时水生植物对营养盐的吸收特征(
... 尽管已有研究表明, 海草既可以通过根系从沉积物中吸收氮磷, 也可以通过叶片从海水中获取, 但多数海草种类的叶片是吸收氮磷的主要部位(
Benthic infauna of eelgrass,Zostera marina, beds
1
1973
... 海草床生态系统较脆弱, 受全球变化和人类活动影响, 目前已是地球生物圈退化速率最快的生态系统, 且退化速率仍在逐年增加(
Epiphyte-seagrass relationships with an emphasis on the role of micrograzing: a review
1
1984
... 大叶藻在寡营养盐条件下具有明显的竞争优势, 减少氮营养盐的输入则可以明显促进退化海草床的自我恢复(
Nitrogen uptake and allocation in the seagrass Amphibolis antarctica
2
1997
... 尽管已有研究表明, 海草既可以通过根系从沉积物中吸收氮磷, 也可以通过叶片从海水中获取, 但多数海草种类的叶片是吸收氮磷的主要部位(
... 同时, 在海草体内存在营养转移的现象, 氮磷在海草不同叶龄结构的叶片间转移可以提高叶片对氮磷的利用效率, 进而促进海草的生长(
Field transplantation of seagrass (Posidonia oceanica) seedlings: effects of invasive algae and nutrients
1
2018
... 海草床生态系统较脆弱, 受全球变化和人类活动影响, 目前已是地球生物圈退化速率最快的生态系统, 且退化速率仍在逐年增加(
Spatial risk assessment of global change impacts on Swedish seagrass ecosystems
1
2020
... 海草床生态系统较脆弱, 受全球变化和人类活动影响, 目前已是地球生物圈退化速率最快的生态系统, 且退化速率仍在逐年增加(
A mechanism to account for macrophyte decline in progressively eutrophicated freshwaters
1
1978
... 大叶藻在寡营养盐条件下具有明显的竞争优势, 减少氮营养盐的输入则可以明显促进退化海草床的自我恢复(
Phosphorus removal by the Ceratophyllum/periphyton complex in a south Florida (USA) freshwater marsh
1
2006
... 氮磷是水生植物最主要的限制性营养盐, ****普遍使用米氏方程来表示稳态时水生植物对营养盐的吸收特征(
Nutrient dynamics in seagrass ecosystems //Larkum AWD, Orth RJ, Duarte CM. Seagrasses: Biology, Ecology and Conservation
1
2006
... 海草床生态系统较脆弱, 受全球变化和人类活动影响, 目前已是地球生物圈退化速率最快的生态系统, 且退化速率仍在逐年增加(
Na+-dependent high-affinity nitrate, phosphate and amino acids transport in leaf cells of the seagrass Posidonia oceanica (L.) delile
4
2018
... 氮磷是水生植物最主要的限制性营养盐, ****普遍使用米氏方程来表示稳态时水生植物对营养盐的吸收特征(
... 在米氏方程中, Vmax表示底物饱和时的吸收速率, 越大则吸收的内在潜力越大, Km表示当吸收速率为最大吸收速率一半时的底物浓度, Km值越小, 对底物亲和力越大(
... ;
... Uptake kinetics of NH4+-N, NO3--N and PO43--P by seagrasses and seaweeds
种类 Species | 研究地点 Study area | Vmax (μmol·g-1·h-1) | Km (μmol·L-1) | 文献 Reference | ||||
---|---|---|---|---|---|---|---|---|
NH4+-N | NO3--N | PO43--P | NH4+-N | NO3--N | PO43--P | |||
大叶藻 Zostera marina | 山东荣成天鹅湖 Tian’e Lake, Rongcheng, Shandong China (37.35° N, 122.57° E) | 51.8 | 39.1 | 27.9 | 68.1 | 68.6 | 24.8 | 本研究 This study |
美国罗德岛 Rhode Island, USA (41.30° N, 71.30° W) | 48 | |||||||
Phyllospadix torreyi | 美国拉由拉市 La Jolla, USA (32.48° N, 117.16° W) | 96-204 | 25-75 | 9-34 | 4-17 | |||
泰来藻 Thalassia hemprichii | 印度尼西亚斯佩蒙德群岛 Spermonde Archipelago, Indonesia (5.03° S, 119.20° E) | 32-37 | 21-60 | |||||
Thalassia testudinum | 美国圣体湾和马德雷湖 Corpus Christi Bay (27.49° N, 97.07° W) & Laguna Madre, USA (26.09° N, 97.12° W) | 8-16 | 4-7 | 8-15 | 2-39 | |||
Posidonia oceanica | 西班牙马拉加 Málaga, Spain (36.40° N, 4.21° W) | 8.7 | 5.8 | |||||
真江蓠 Gracilari asiatica | 浙江奉化象山港 Xiangshan Harbor, Fenghua, China (29.11° N, 122.01° E) | 159.4 | ||||||
龙须菜 Asparagus schoberioides | 福建东山岛 Dongshan Island, Fujian China (23.36° N, 117.14° E) | 3.1 | ||||||
浒苔 Ulva prolifera | 山东青岛汇泉湾 Huiquan Bay, Qingdao, Shandong China (36.03° N, 123.20° E) | 250.4 | 5.8 |
Regulation of nitrate uptake by the seagrassZostera marina during upwelling.
1
2019
... 大叶藻在寡营养盐条件下具有明显的竞争优势, 减少氮营养盐的输入则可以明显促进退化海草床的自我恢复(
Extinction risk assessment of the world’s seagrass species
2
2011
... 海草是一类海洋高等单子叶开花植物, 具有极高的初级生产力, 在我国主要分布于温带的山东、辽宁、河北, 亚热带、热带的广东、广西和海南等沿岸海域(
... ).
Nutrient uptake by leaves and roots of the seagrass Thalassia hemprichii in the Spermonde Archipelago, Indonesia
2
1996
... Uptake kinetics of NH4+-N, NO3--N and PO43--P by seagrasses and seaweeds
种类 Species | 研究地点 Study area | Vmax (μmol·g-1·h-1) | Km (μmol·L-1) | 文献 Reference | ||||
---|---|---|---|---|---|---|---|---|
NH4+-N | NO3--N | PO43--P | NH4+-N | NO3--N | PO43--P | |||
大叶藻 Zostera marina | 山东荣成天鹅湖 Tian’e Lake, Rongcheng, Shandong China (37.35° N, 122.57° E) | 51.8 | 39.1 | 27.9 | 68.1 | 68.6 | 24.8 | 本研究 This study |
美国罗德岛 Rhode Island, USA (41.30° N, 71.30° W) | 48 | |||||||
Phyllospadix torreyi | 美国拉由拉市 La Jolla, USA (32.48° N, 117.16° W) | 96-204 | 25-75 | 9-34 | 4-17 | |||
泰来藻 Thalassia hemprichii | 印度尼西亚斯佩蒙德群岛 Spermonde Archipelago, Indonesia (5.03° S, 119.20° E) | 32-37 | 21-60 | |||||
Thalassia testudinum | 美国圣体湾和马德雷湖 Corpus Christi Bay (27.49° N, 97.07° W) & Laguna Madre, USA (26.09° N, 97.12° W) | 8-16 | 4-7 | 8-15 | 2-39 | |||
Posidonia oceanica | 西班牙马拉加 Málaga, Spain (36.40° N, 4.21° W) | 8.7 | 5.8 | |||||
真江蓠 Gracilari asiatica | 浙江奉化象山港 Xiangshan Harbor, Fenghua, China (29.11° N, 122.01° E) | 159.4 | ||||||
龙须菜 Asparagus schoberioides | 福建东山岛 Dongshan Island, Fujian China (23.36° N, 117.14° E) | 3.1 | ||||||
浒苔 Ulva prolifera | 山东青岛汇泉湾 Huiquan Bay, Qingdao, Shandong China (36.03° N, 123.20° E) | 250.4 | 5.8 |
... 也有部分海草并非依赖地上组织吸收氮磷营养盐.如
Nutrient resorption from seagrass leaves
1
1997
... 同时, 在海草体内存在营养转移的现象, 氮磷在海草不同叶龄结构的叶片间转移可以提高叶片对氮磷的利用效率, 进而促进海草的生长(
Leaf versus root nitrogen uptake by the surfgrass Phyllospadix torreyi
2
1997
... Uptake kinetics of NH4+-N, NO3--N and PO43--P by seagrasses and seaweeds
种类 Species | 研究地点 Study area | Vmax (μmol·g-1·h-1) | Km (μmol·L-1) | 文献 Reference | ||||
---|---|---|---|---|---|---|---|---|
NH4+-N | NO3--N | PO43--P | NH4+-N | NO3--N | PO43--P | |||
大叶藻 Zostera marina | 山东荣成天鹅湖 Tian’e Lake, Rongcheng, Shandong China (37.35° N, 122.57° E) | 51.8 | 39.1 | 27.9 | 68.1 | 68.6 | 24.8 | 本研究 This study |
美国罗德岛 Rhode Island, USA (41.30° N, 71.30° W) | 48 | |||||||
Phyllospadix torreyi | 美国拉由拉市 La Jolla, USA (32.48° N, 117.16° W) | 96-204 | 25-75 | 9-34 | 4-17 | |||
泰来藻 Thalassia hemprichii | 印度尼西亚斯佩蒙德群岛 Spermonde Archipelago, Indonesia (5.03° S, 119.20° E) | 32-37 | 21-60 | |||||
Thalassia testudinum | 美国圣体湾和马德雷湖 Corpus Christi Bay (27.49° N, 97.07° W) & Laguna Madre, USA (26.09° N, 97.12° W) | 8-16 | 4-7 | 8-15 | 2-39 | |||
Posidonia oceanica | 西班牙马拉加 Málaga, Spain (36.40° N, 4.21° W) | 8.7 | 5.8 | |||||
真江蓠 Gracilari asiatica | 浙江奉化象山港 Xiangshan Harbor, Fenghua, China (29.11° N, 122.01° E) | 159.4 | ||||||
龙须菜 Asparagus schoberioides | 福建东山岛 Dongshan Island, Fujian China (23.36° N, 117.14° E) | 3.1 | ||||||
浒苔 Ulva prolifera | 山东青岛汇泉湾 Huiquan Bay, Qingdao, Shandong China (36.03° N, 123.20° E) | 250.4 | 5.8 |
... 同时, 在海草体内存在营养转移的现象, 氮磷在海草不同叶龄结构的叶片间转移可以提高叶片对氮磷的利用效率, 进而促进海草的生长(
Response of aboveground net primary plant production to nitrogen and phosphorus fertilization in peatlands in southern boreal Alberta, Canada
1
1997
... 氮磷是水生植物最主要的限制性营养盐, ****普遍使用米氏方程来表示稳态时水生植物对营养盐的吸收特征(
Leaf-root interaction in the uptake of ammonia by Zostera marina
1
1982
... Uptake kinetics of NH4+-N, NO3--N and PO43--P by seagrasses and seaweeds
种类 Species | 研究地点 Study area | Vmax (μmol·g-1·h-1) | Km (μmol·L-1) | 文献 Reference | ||||
---|---|---|---|---|---|---|---|---|
NH4+-N | NO3--N | PO43--P | NH4+-N | NO3--N | PO43--P | |||
大叶藻 Zostera marina | 山东荣成天鹅湖 Tian’e Lake, Rongcheng, Shandong China (37.35° N, 122.57° E) | 51.8 | 39.1 | 27.9 | 68.1 | 68.6 | 24.8 | 本研究 This study |
美国罗德岛 Rhode Island, USA (41.30° N, 71.30° W) | 48 | |||||||
Phyllospadix torreyi | 美国拉由拉市 La Jolla, USA (32.48° N, 117.16° W) | 96-204 | 25-75 | 9-34 | 4-17 | |||
泰来藻 Thalassia hemprichii | 印度尼西亚斯佩蒙德群岛 Spermonde Archipelago, Indonesia (5.03° S, 119.20° E) | 32-37 | 21-60 | |||||
Thalassia testudinum | 美国圣体湾和马德雷湖 Corpus Christi Bay (27.49° N, 97.07° W) & Laguna Madre, USA (26.09° N, 97.12° W) | 8-16 | 4-7 | 8-15 | 2-39 | |||
Posidonia oceanica | 西班牙马拉加 Málaga, Spain (36.40° N, 4.21° W) | 8.7 | 5.8 | |||||
真江蓠 Gracilari asiatica | 浙江奉化象山港 Xiangshan Harbor, Fenghua, China (29.11° N, 122.01° E) | 159.4 | ||||||
龙须菜 Asparagus schoberioides | 福建东山岛 Dongshan Island, Fujian China (23.36° N, 117.14° E) | 3.1 | ||||||
浒苔 Ulva prolifera | 山东青岛汇泉湾 Huiquan Bay, Qingdao, Shandong China (36.03° N, 123.20° E) | 250.4 | 5.8 |
Interaction of leaves and roots of Ruppia maritima in the uptake of phosphate, ammonia and nitrate
1
1984
... 尽管已有研究表明, 海草既可以通过根系从沉积物中吸收氮磷, 也可以通过叶片从海水中获取, 但多数海草种类的叶片是吸收氮磷的主要部位(
几种作物NO3 吸收动力学参数测定方法初探
1
2001
... 在米氏方程中, Vmax表示底物饱和时的吸收速率, 越大则吸收的内在潜力越大, Km表示当吸收速率为最大吸收速率一半时的底物浓度, Km值越小, 对底物亲和力越大(
几种作物NO3 吸收动力学参数测定方法初探
1
2001
... 在米氏方程中, Vmax表示底物饱和时的吸收速率, 越大则吸收的内在潜力越大, Km表示当吸收速率为最大吸收速率一半时的底物浓度, Km值越小, 对底物亲和力越大(
Review of nitrogen and phosphorus metabolism in seagrasses
1
2000
... 海草床生态系统较脆弱, 受全球变化和人类活动影响, 目前已是地球生物圈退化速率最快的生态系统, 且退化速率仍在逐年增加(
Effects of inorganic n availability on algal photosynthesis and carbon metabolism
1
1991
... 陆生高等植物主要以硝态氮和氨态氮作为无机氮素的来源, 但更多的是吸收硝态氮, 只有在某些还原性较强的土壤或者水淹地, 其主要的氮源才可能是氨态氮(
Growth and physiological responses of three seagrass species to elevated sediment nutrients in Moreton Bay, Australia
1
1997
...
Seagrass meadows support global fisheries production
1
2019
... 海草是一类海洋高等单子叶开花植物, 具有极高的初级生产力, 在我国主要分布于温带的山东、辽宁、河北, 亚热带、热带的广东、广西和海南等沿岸海域(
Potential uptake of dissolved organic matter by seagrasses and macroalgae
1
2011
... 氮磷是水生植物最主要的限制性营养盐, ****普遍使用米氏方程来表示稳态时水生植物对营养盐的吸收特征(
Effects of salinity and nutrient load and their interaction on Zostera marina
1
1999
... 海草床生态系统较脆弱, 受全球变化和人类活动影响, 目前已是地球生物圈退化速率最快的生态系统, 且退化速率仍在逐年增加(
Ammonium toxicity in eelgrass Zostera marina
2
1997
...
... 大叶藻在寡营养盐条件下具有明显的竞争优势, 减少氮营养盐的输入则可以明显促进退化海草床的自我恢复(
High ammonium availability amplifies the adverse effect of low salinity on eelgrass Zostera marina
1
2015
... 大叶藻在寡营养盐条件下具有明显的竞争优势, 减少氮营养盐的输入则可以明显促进退化海草床的自我恢复(
Dissolved organic nitrogen uptake by seagrasses
1
2008
... 氮磷是水生植物最主要的限制性营养盐, ****普遍使用米氏方程来表示稳态时水生植物对营养盐的吸收特征(
浒苔对NO3-N和PO4-P吸收动力学特征
1
2011
... Uptake kinetics of NH4+-N, NO3--N and PO43--P by seagrasses and seaweeds
种类 Species | 研究地点 Study area | Vmax (μmol·g-1·h-1) | Km (μmol·L-1) | 文献 Reference | ||||
---|---|---|---|---|---|---|---|---|
NH4+-N | NO3--N | PO43--P | NH4+-N | NO3--N | PO43--P | |||
大叶藻 Zostera marina | 山东荣成天鹅湖 Tian’e Lake, Rongcheng, Shandong China (37.35° N, 122.57° E) | 51.8 | 39.1 | 27.9 | 68.1 | 68.6 | 24.8 | 本研究 This study |
美国罗德岛 Rhode Island, USA (41.30° N, 71.30° W) | 48 | |||||||
Phyllospadix torreyi | 美国拉由拉市 La Jolla, USA (32.48° N, 117.16° W) | 96-204 | 25-75 | 9-34 | 4-17 | |||
泰来藻 Thalassia hemprichii | 印度尼西亚斯佩蒙德群岛 Spermonde Archipelago, Indonesia (5.03° S, 119.20° E) | 32-37 | 21-60 | |||||
Thalassia testudinum | 美国圣体湾和马德雷湖 Corpus Christi Bay (27.49° N, 97.07° W) & Laguna Madre, USA (26.09° N, 97.12° W) | 8-16 | 4-7 | 8-15 | 2-39 | |||
Posidonia oceanica | 西班牙马拉加 Málaga, Spain (36.40° N, 4.21° W) | 8.7 | 5.8 | |||||
真江蓠 Gracilari asiatica | 浙江奉化象山港 Xiangshan Harbor, Fenghua, China (29.11° N, 122.01° E) | 159.4 | ||||||
龙须菜 Asparagus schoberioides | 福建东山岛 Dongshan Island, Fujian China (23.36° N, 117.14° E) | 3.1 | ||||||
浒苔 Ulva prolifera | 山东青岛汇泉湾 Huiquan Bay, Qingdao, Shandong China (36.03° N, 123.20° E) | 250.4 | 5.8 |
浒苔对NO3-N和PO4-P吸收动力学特征
1
2011
... Uptake kinetics of NH4+-N, NO3--N and PO43--P by seagrasses and seaweeds
种类 Species | 研究地点 Study area | Vmax (μmol·g-1·h-1) | Km (μmol·L-1) | 文献 Reference | ||||
---|---|---|---|---|---|---|---|---|
NH4+-N | NO3--N | PO43--P | NH4+-N | NO3--N | PO43--P | |||
大叶藻 Zostera marina | 山东荣成天鹅湖 Tian’e Lake, Rongcheng, Shandong China (37.35° N, 122.57° E) | 51.8 | 39.1 | 27.9 | 68.1 | 68.6 | 24.8 | 本研究 This study |
美国罗德岛 Rhode Island, USA (41.30° N, 71.30° W) | 48 | |||||||
Phyllospadix torreyi | 美国拉由拉市 La Jolla, USA (32.48° N, 117.16° W) | 96-204 | 25-75 | 9-34 | 4-17 | |||
泰来藻 Thalassia hemprichii | 印度尼西亚斯佩蒙德群岛 Spermonde Archipelago, Indonesia (5.03° S, 119.20° E) | 32-37 | 21-60 | |||||
Thalassia testudinum | 美国圣体湾和马德雷湖 Corpus Christi Bay (27.49° N, 97.07° W) & Laguna Madre, USA (26.09° N, 97.12° W) | 8-16 | 4-7 | 8-15 | 2-39 | |||
Posidonia oceanica | 西班牙马拉加 Málaga, Spain (36.40° N, 4.21° W) | 8.7 | 5.8 | |||||
真江蓠 Gracilari asiatica | 浙江奉化象山港 Xiangshan Harbor, Fenghua, China (29.11° N, 122.01° E) | 159.4 | ||||||
龙须菜 Asparagus schoberioides | 福建东山岛 Dongshan Island, Fujian China (23.36° N, 117.14° E) | 3.1 | ||||||
浒苔 Ulva prolifera | 山东青岛汇泉湾 Huiquan Bay, Qingdao, Shandong China (36.03° N, 123.20° E) | 250.4 | 5.8 |
Accelerating loss of seagrasses across the globe threatens coastal ecosystems
1
2009
... 海草床生态系统较脆弱, 受全球变化和人类活动影响, 目前已是地球生物圈退化速率最快的生态系统, 且退化速率仍在逐年增加(
Extracellular alkaline phosphatase activity in Ulva lactuca L
1
1989
... 此外, 植株的吸收动力学特征受到环境光照、温度和盐度等环境条件的影响.光照的影响主要体现在植株的光合作用和酶活性等方面, 如有研究表明, 水生植物在光照条件下对营养盐的吸收速率远大于其在黑暗条件下的吸收速率, 光照还能激活水生植物体内的碱性磷酸酶活性, 为植物吸收利用磷酸盐提供条件(
真江蓠对氨氮去除效率与吸收动力学研究
1
2008
... Uptake kinetics of NH4+-N, NO3--N and PO43--P by seagrasses and seaweeds
种类 Species | 研究地点 Study area | Vmax (μmol·g-1·h-1) | Km (μmol·L-1) | 文献 Reference | ||||
---|---|---|---|---|---|---|---|---|
NH4+-N | NO3--N | PO43--P | NH4+-N | NO3--N | PO43--P | |||
大叶藻 Zostera marina | 山东荣成天鹅湖 Tian’e Lake, Rongcheng, Shandong China (37.35° N, 122.57° E) | 51.8 | 39.1 | 27.9 | 68.1 | 68.6 | 24.8 | 本研究 This study |
美国罗德岛 Rhode Island, USA (41.30° N, 71.30° W) | 48 | |||||||
Phyllospadix torreyi | 美国拉由拉市 La Jolla, USA (32.48° N, 117.16° W) | 96-204 | 25-75 | 9-34 | 4-17 | |||
泰来藻 Thalassia hemprichii | 印度尼西亚斯佩蒙德群岛 Spermonde Archipelago, Indonesia (5.03° S, 119.20° E) | 32-37 | 21-60 | |||||
Thalassia testudinum | 美国圣体湾和马德雷湖 Corpus Christi Bay (27.49° N, 97.07° W) & Laguna Madre, USA (26.09° N, 97.12° W) | 8-16 | 4-7 | 8-15 | 2-39 | |||
Posidonia oceanica | 西班牙马拉加 Málaga, Spain (36.40° N, 4.21° W) | 8.7 | 5.8 | |||||
真江蓠 Gracilari asiatica | 浙江奉化象山港 Xiangshan Harbor, Fenghua, China (29.11° N, 122.01° E) | 159.4 | ||||||
龙须菜 Asparagus schoberioides | 福建东山岛 Dongshan Island, Fujian China (23.36° N, 117.14° E) | 3.1 | ||||||
浒苔 Ulva prolifera | 山东青岛汇泉湾 Huiquan Bay, Qingdao, Shandong China (36.03° N, 123.20° E) | 250.4 | 5.8 |
真江蓠对氨氮去除效率与吸收动力学研究
1
2008
... Uptake kinetics of NH4+-N, NO3--N and PO43--P by seagrasses and seaweeds
种类 Species | 研究地点 Study area | Vmax (μmol·g-1·h-1) | Km (μmol·L-1) | 文献 Reference | ||||
---|---|---|---|---|---|---|---|---|
NH4+-N | NO3--N | PO43--P | NH4+-N | NO3--N | PO43--P | |||
大叶藻 Zostera marina | 山东荣成天鹅湖 Tian’e Lake, Rongcheng, Shandong China (37.35° N, 122.57° E) | 51.8 | 39.1 | 27.9 | 68.1 | 68.6 | 24.8 | 本研究 This study |
美国罗德岛 Rhode Island, USA (41.30° N, 71.30° W) | 48 | |||||||
Phyllospadix torreyi | 美国拉由拉市 La Jolla, USA (32.48° N, 117.16° W) | 96-204 | 25-75 | 9-34 | 4-17 | |||
泰来藻 Thalassia hemprichii | 印度尼西亚斯佩蒙德群岛 Spermonde Archipelago, Indonesia (5.03° S, 119.20° E) | 32-37 | 21-60 | |||||
Thalassia testudinum | 美国圣体湾和马德雷湖 Corpus Christi Bay (27.49° N, 97.07° W) & Laguna Madre, USA (26.09° N, 97.12° W) | 8-16 | 4-7 | 8-15 | 2-39 | |||
Posidonia oceanica | 西班牙马拉加 Málaga, Spain (36.40° N, 4.21° W) | 8.7 | 5.8 | |||||
真江蓠 Gracilari asiatica | 浙江奉化象山港 Xiangshan Harbor, Fenghua, China (29.11° N, 122.01° E) | 159.4 | ||||||
龙须菜 Asparagus schoberioides | 福建东山岛 Dongshan Island, Fujian China (23.36° N, 117.14° E) | 3.1 | ||||||
浒苔 Ulva prolifera | 山东青岛汇泉湾 Huiquan Bay, Qingdao, Shandong China (36.03° N, 123.20° E) | 250.4 | 5.8 |
两种大型海藻自然环境下的营养动力学研究
1
2007
... Uptake kinetics of NH4+-N, NO3--N and PO43--P by seagrasses and seaweeds
种类 Species | 研究地点 Study area | Vmax (μmol·g-1·h-1) | Km (μmol·L-1) | 文献 Reference | ||||
---|---|---|---|---|---|---|---|---|
NH4+-N | NO3--N | PO43--P | NH4+-N | NO3--N | PO43--P | |||
大叶藻 Zostera marina | 山东荣成天鹅湖 Tian’e Lake, Rongcheng, Shandong China (37.35° N, 122.57° E) | 51.8 | 39.1 | 27.9 | 68.1 | 68.6 | 24.8 | 本研究 This study |
美国罗德岛 Rhode Island, USA (41.30° N, 71.30° W) | 48 | |||||||
Phyllospadix torreyi | 美国拉由拉市 La Jolla, USA (32.48° N, 117.16° W) | 96-204 | 25-75 | 9-34 | 4-17 | |||
泰来藻 Thalassia hemprichii | 印度尼西亚斯佩蒙德群岛 Spermonde Archipelago, Indonesia (5.03° S, 119.20° E) | 32-37 | 21-60 | |||||
Thalassia testudinum | 美国圣体湾和马德雷湖 Corpus Christi Bay (27.49° N, 97.07° W) & Laguna Madre, USA (26.09° N, 97.12° W) | 8-16 | 4-7 | 8-15 | 2-39 | |||
Posidonia oceanica | 西班牙马拉加 Málaga, Spain (36.40° N, 4.21° W) | 8.7 | 5.8 | |||||
真江蓠 Gracilari asiatica | 浙江奉化象山港 Xiangshan Harbor, Fenghua, China (29.11° N, 122.01° E) | 159.4 | ||||||
龙须菜 Asparagus schoberioides | 福建东山岛 Dongshan Island, Fujian China (23.36° N, 117.14° E) | 3.1 | ||||||
浒苔 Ulva prolifera | 山东青岛汇泉湾 Huiquan Bay, Qingdao, Shandong China (36.03° N, 123.20° E) | 250.4 | 5.8 |
两种大型海藻自然环境下的营养动力学研究
1
2007
... Uptake kinetics of NH4+-N, NO3--N and PO43--P by seagrasses and seaweeds
种类 Species | 研究地点 Study area | Vmax (μmol·g-1·h-1) | Km (μmol·L-1) | 文献 Reference | ||||
---|---|---|---|---|---|---|---|---|
NH4+-N | NO3--N | PO43--P | NH4+-N | NO3--N | PO43--P | |||
大叶藻 Zostera marina | 山东荣成天鹅湖 Tian’e Lake, Rongcheng, Shandong China (37.35° N, 122.57° E) | 51.8 | 39.1 | 27.9 | 68.1 | 68.6 | 24.8 | 本研究 This study |
美国罗德岛 Rhode Island, USA (41.30° N, 71.30° W) | 48 | |||||||
Phyllospadix torreyi | 美国拉由拉市 La Jolla, USA (32.48° N, 117.16° W) | 96-204 | 25-75 | 9-34 | 4-17 | |||
泰来藻 Thalassia hemprichii | 印度尼西亚斯佩蒙德群岛 Spermonde Archipelago, Indonesia (5.03° S, 119.20° E) | 32-37 | 21-60 | |||||
Thalassia testudinum | 美国圣体湾和马德雷湖 Corpus Christi Bay (27.49° N, 97.07° W) & Laguna Madre, USA (26.09° N, 97.12° W) | 8-16 | 4-7 | 8-15 | 2-39 | |||
Posidonia oceanica | 西班牙马拉加 Málaga, Spain (36.40° N, 4.21° W) | 8.7 | 5.8 | |||||
真江蓠 Gracilari asiatica | 浙江奉化象山港 Xiangshan Harbor, Fenghua, China (29.11° N, 122.01° E) | 159.4 | ||||||
龙须菜 Asparagus schoberioides | 福建东山岛 Dongshan Island, Fujian China (23.36° N, 117.14° E) | 3.1 | ||||||
浒苔 Ulva prolifera | 山东青岛汇泉湾 Huiquan Bay, Qingdao, Shandong China (36.03° N, 123.20° E) | 250.4 | 5.8 |
中国海草的多样性, 分布及保护
2
2013
... 海草是一类海洋高等单子叶开花植物, 具有极高的初级生产力, 在我国主要分布于温带的山东、辽宁、河北, 亚热带、热带的广东、广西和海南等沿岸海域(
... 海草床生态系统较脆弱, 受全球变化和人类活动影响, 目前已是地球生物圈退化速率最快的生态系统, 且退化速率仍在逐年增加(
中国海草的多样性, 分布及保护
2
2013
... 海草是一类海洋高等单子叶开花植物, 具有极高的初级生产力, 在我国主要分布于温带的山东、辽宁、河北, 亚热带、热带的广东、广西和海南等沿岸海域(
... 海草床生态系统较脆弱, 受全球变化和人类活动影响, 目前已是地球生物圈退化速率最快的生态系统, 且退化速率仍在逐年增加(