

Impacts of elevated carbon dioxide concentration on terrestrial ecosystems: problems and prospective
Zhao-Zhong FENG

通讯作者: *ORCID: 冯兆忠: 0000-0002-9775-5113, E-mail:zhaozhong.feng@nuist.edu.cn
编委: 刘菊秀
责任编辑: 李 敏
收稿日期:2019-05-24接受日期:2019-08-25网络出版日期:2020-05-20
基金资助: |
Received:2019-05-24Accepted:2019-08-25Online:2020-05-20
Fund supported: |

摘要
理解生态系统对过去、现在和未来CO2浓度变化的响应,对于在生态进化的时间尺度上认识和预测全球变化的后果至关重要。过去三十多年来CO2浓度升高相关的科学问题主要集中在对植物生长和生产力的影响, 碳氮周转, 生态系统渐进式氮限制(PNL)形成, 与其他胁迫因子(O3污染、氮沉降、升温、干旱)之间的交互作用等方面。尽管生态学家在数据累积、基础理论上取得了一定进展, 但是仍然存在较大不确定性和大量未知有待解决。该文探究了近30年来CO2浓度升高对陆地生态系统影响研究的国际研究进展、重点领域及热点, 回顾了CO2浓度升高对植物影响的模拟实验研究发展, 重点论述了CO2浓度升高对粮食产量及品质、碳固定、水分利用效率、生态系统氮利用和土壤微生物响应等国际前沿动态研究中存在的主要问题与不足, 在此基础上展望了未来研究中值得关注的前沿研究方向。
关键词:
Abstract
Characterizing ecosystem responses to past, present and future changes in atmopsheric carbon dioxide (CO2) concentration is critical for understanding and predicting the consequences of global change over evolutionary and ecological timescales. Over the past two decades, CO2 studies have provided great insights into the effects of rising CO2 concentration on plant growth and productivity, carbon-nitrogen turnover, the formation of progressive nitrogen limitation (PNL) in ecosystems, and the interaction between elevated CO2 concentration and other envrionmental factors (O3 pollution, N deposition, warming and drought). However, scaling CO2 effects across wide spatial and temporal scales, especially at belowground part, has many uncertainties. Here we explore major research areas and hotspots of CO2 studies on plants and ecosystems from 1990 to 2018, and review the development of manipulated experiments in the field of elevated CO2 impacts. In detail, we discussed the states of art in five international frontiers research directions: crop yield and quality, carbon fixation, water use efficiency, ecosystem nitrogen use and soil microorganism. Finally, we identify several topics and research outlooks to facilitate further developments in the field of CO2 effects on ecosystems.
Keywords:
PDF (1184KB)元数据多维度评价相关文章导出EndNote|Ris|Bibtex收藏本文
引用本文
冯兆忠, 李品, 张国友, 李征珍, 平琴, 彭金龙, 刘硕. 二氧化碳浓度升高对陆地生态系统的影响: 问题与展望. 植物生态学报, 2020, 44(5): 461-474. DOI: 10.17521/cjpe.2019.0125
FENG Zhao-Zhong, LI Pin, ZHANG Guo-You, LI Zheng-Zhen, PING Qin, PENG Jin-Long, LIU Shuo.
工业革命以来, 人类活动导致化石燃料过量使用和土地利用方式改变等, 引发了大气中CO2等温室气体浓度的急剧上升。目前大气中CO2浓度已由1860年的280 μL·L-1升高至目前的410 μL·L-1左右, 并以每年0.45%的速率升高, 预计到2030年将增加到550 μL·L-1 (IPCC, 2013)。CO2浓度升高加速全球变暖并引发冰川融化和海平面上升, 继而引发洪水、台风、热浪等极端天气频发的连锁反应, 严重影响生态系统结构和功能的可持续性。目前已认识到大气CO2浓度升高对植物光合作用、产量及生产力具有促进作用, 这种现象被称为CO2的“施肥效应”。由于在最近几十年内CO2浓度升高的趋势很难改变, 因此, CO2 “施肥效应”对生态系统“碳汇”功能的促进作用不失为一种积极地应对气候变化的策略, 但其影响程度还存在较大不确定性。CO2浓度升高显著增加植被“碳汇”功能, 但对土壤“碳汇”功能的影响却不一致。大气CO2浓度升高对陆地碳汇的长期影响在很大程度上取决于土壤中的氮含量(Zak et al., 2000; Luo et al., 2004; Reich et al., 2006; Feng et al., 2015; Terrer et al., 2018)。以往的研究主要聚焦在叶片尺度的气体交换、碳动态以及植物生长等方面, 取得了一定的系统性认识(Becklin et al., 2017)。然而, 跨尺度的植物-土壤-微生物互作过程的响应、生态系统碳-氮-水循环关键过程的响应以及对植物的生态反馈机制的影响等关键科学问题仍未解决, 从而增加了对于理解和预测CO2浓度升高对生态系统影响的不确定性。本文总结了当前CO2浓度升高对粮食产量及品质、碳固定、水分利用效率、生态系统氮利用和土壤微生物响应等研究领域的国际前沿动态, 重点综述了目前研究存在的主要问题与不足, 在此基础上展望了未来研究中值得关注的重点方向和研究内容。
1 基于文献计量的研究发展态势分析(1990- 2018)
1.1 中国与世界SCI文章发表数量对比分析
本文基于CO2浓度升高与生态系统研究领域的基本状况, 应用文献计量学的方法, 利用Web of Science核心数据库, 选取检索式TS = ([“vegetation” OR “plant” OR “soil” OR “ecosystem”] AND [“CO2” OR “carbon dioxide”]), 对1990-2018年29年间世界CO2浓度升高对生态系统影响研究的SCI文章的发表数量年际间的变化趋势进行了统计分析(图1), 最终共检索到文献6 181篇。检索数据显示, 1997年以后国际上该领域的发文量有明显的上升趋势, 对CO2浓度升高的生态效应研究领域的重视程度增强。2013年以后发文量较1998-2012年再次呈现上升趋势。与世界相比, 我国发文量的发展趋势基本一致。其中, 2011-2014年增长较快。从整体发文数量上来讲, 1998-2018年中国发文总量(824篇)占全世界(6 181篇)的13%。表明在CO2浓度升高的生态效应研究领域, 仅从发文量上来看, 我国生态学工作者的贡献还存在一定差距。图1
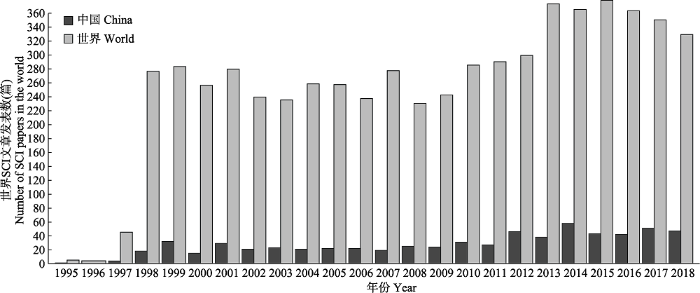
图1中国与世界CO2浓度升高对陆地生态系统影响研究的SCI文章数量变化情况(1990-2018)。
Fig. 1Changes in the number of SCI articles in terms of the impact of rising CO2 concentrations on terrestrial ecosystems in China and the world (1990-2018).
1.2 主要研究热点
统计1990-2018年CO2浓度升高对生态影响研究的SCI发文的学科领域(图2)和关键词(表1), 分别列出发文量前1%的学科领域和前50位的关键词。从研究的学科领域上看(图2), 集中在环境科学、生态学、植物科学、农学、生物多样性保护和林学, 占据了大约71%的总发文数。从出现频次居前10位的关键词(表1)来看, 依次是CO2升高(elevated CO2)、气候变化(climate change)、二氧化碳(carbon dioxide)、光合作用(photosynthesis)、全球变化(global change)、FACE系统(FACE)、氮(nitrogen)、CO2富集(CO2 enrichment)、气孔导度(stomatal conductance)、生长(growth)。图2
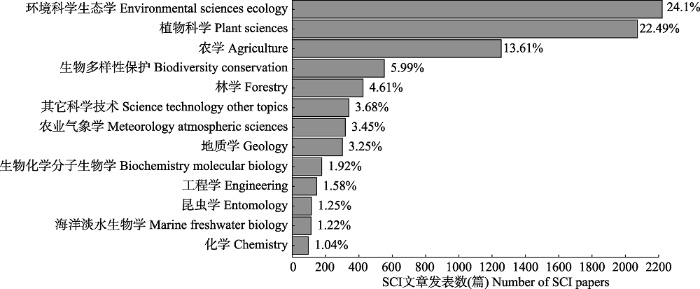
图2CO2浓度升高对生态系统影响的SCI文章发文的学科领域。
Fig. 2Discipline distribution of SCI articles in terms of the impact of rising CO2 concentrations on terrestrial ecosystems.
Table 1
表1
表1CO2浓度升高对生态系统影响的SCI文章出现频次最多的前50个关键词
Table 1
序号 Number | 关键词 Keyword | 频次 Frequency | 序号 Number | 关键词 Keyword | 频次 Frequency |
---|---|---|---|---|---|
1 | CO2升高 Elevated CO2 | 1 148 | 26 | 全球变暖 Global warming | 75 |
2 | 气候变化 Climate change | 769 | 27 | 叶绿素荧光 Chlorophyll fluorescence | 74 |
3 | 二氧化碳 Carbon dioxide | 729 | 28 | 产量 Yield | 73 |
4 | 光合作用 Photosynthesis | 524 | 29 | 蒸腾作用 Transpiration | 67 |
5 | 全球变化 Global change | 310 | 30 | 增温 Warming | 63 |
6 | FACE系统 FACE | 244 | 31 | 植食 Herbivory | 62 |
7 | 氮 Nitrogen | 228 | 32 | 碳水化合物 Carbohydrates | 61 |
8 | CO2富集 CO2 enrichment | 188 | 33 | 竞争 Competition | 58 |
9 | 气孔导度 Stomatal conductance | 186 | 34 | 磷 Phosphorus | 57 |
10 | 生长 Growth | 178 | 35 | 大气CO2 Atmospheric CO2 | 56 |
11 | 干旱 Drought | 154 | 36 | 水分胁迫 Water stress | 56 |
12 | 温度 Temperature | 140 | 37 | 碳循环 Carbon cycle | 54 |
13 | 生物量 Biomass | 109 | 38 | 分解 Decomposition | 53 |
14 | 臭氧 Ozone/O3 | 102 | 39 | 温度升高 Elevated temperature | 52 |
15 | 自由大气CO2富集 Free-air CO2 enrichment | 100 | 40 | 蒸散 Evapotranspiration | 52 |
16 | 呼吸 Respiration | 94 | 41 | 土壤湿度 Soil moisture | 52 |
17 | 二磷酸核酮糖羧化酶 Rubisco | 83 | 42 | 气孔 Stomata | 52 |
18 | 土壤呼吸 Soil respiration | 83 | 43 | 淀粉 Starch | 51 |
19 | 驯化 Acclimation | 82 | 44 | 土壤有机质 Soil organic matter | 49 |
20 | 碳固定 Carbon sequestration | 82 | 45 | 氮沉降 Nitrogen deposition | 48 |
21 | 水分利用效率 Water use efficiency | 80 | 46 | 大豆 Soybean | 47 |
22 | 草地 Grassland | 79 | 47 | 碳 Carbon | 45 |
23 | 气体交换 Gas exchange | 78 | 48 | 全球气候变化 Global climate change | 45 |
24 | 小麦 Wheat | 77 | 49 | 根际 Rhizosphere | 45 |
25 | 水稻 Rice | 76 | 50 | Meta分析 Meta-analysis | 44 |
新窗口打开|下载CSV
2 历史回顾
CO2浓度升高对植物影响的模拟实验研究可以追溯到20世纪60年代(Terashima et al., 2014)。经过几十年的发展, CO2浓度升高的研究手段包括了长期观测与野外调查(如通量观测), 大型野外控制实验(密闭生长箱、田间开顶箱(open top chamber, OTC), 开放体系的CO2浓度增高系统(free air CO2 enrichment, FACE)), 生态系统过程模型, 遥感反演等。研究对象从单一的植物个体发展到不同生态系统(Kimball et al., 2001; Norby et al., 2001b; Feng et al., 2015), 对草地和森林生态系统研究较多, 而农田、湿地、湖泊生态系统研究较少。FACE系统是目前研究CO2浓度升高对地上-地下生态系统影响及其机理最为理想的方法。FACE系统相比其他模拟手段不会限制植物的根系, 也不会对风、光照和辐射等产生影响, 因此能够满足长期持续地研究CO2浓度升高对整个生态系统的影响(Long, 2006; Bunce, 2016)。首次FACE实验成功运行, 始于1989年在美国亚利桑那州的农田(棉花Gossypium spp.) FACE。此后, 全世界范围内先后建立了近40个FACE, 包括森林(如Duke FACE、ASPEN FACE、EucFACE、POPFACE、Bangor FACE等), 草地(BioCON、TasFACE、MEGARICH、Swiss FACE等), 农田(SoyFACE、中国水稻/小麦FACE、Potato FACE、Tsukuba FACE等), 热带稀树草原(OzFACE), 湿地(BERI), 荒漠(NDFF)。这些实验站中CO2浓度升高的模拟设施大同小异, 基本上都是圆形或者多边形的试验区, CO2从周边沿着顺风方向被释放到试验区, 大部分处理都是将CO2浓度设定在550 μL·L-1或者比对照增加200 μL·L-1。中国于2001年在江苏无锡安镇建立了水稻-小麦轮作的农田FACE系统。通过数十年来对高CO2浓度条件下植物叶片气孔、光合作用、物质分配、生育期、作物产量以及生产力等方面的大量观测, 发现FACE研究结果与密闭和半密闭箱室的结果存在差异(Ainsworth & Long, 2005; Norby & Zak, 2011; Jones et al., 2014)。通过长期(大于5年)的FACE研究发现, 大气CO2浓度增加对生态系统净生产力的促进作用可能并没有想象的那么高, 存在短期显著促进但长期的促进作用会削弱的效果(Norby & Zak, 2011)。FACE的研究结果虽然不能简单上推到全球尺度的评估, 但为模型的建立提供了相对可靠的数据, 也为后续尺度上推到区域和全球提供了坚实基础。
3 重大进展及存在的主要问题
3.1 CO2浓度升高对粮食产量及品质的影响
水稻(Yang et al., 2009; Hasegawa et al., 2013), 小麦(Tausz-Posch et al., 2015; Bunce, 2017), 大豆(Bishop et al., 2014)和扁豆(Bourgault et al., 2017) FACE的研究结果表明, 在CO2浓度升高条件下作物产量均显著增加, 但不同作物品种的增产幅度存在显著差异。作物产量及构成要素对CO2浓度升高响应的品种差异涉及叶片光合生理(Chen et al., 2014), 籽粒灌浆动态(Zhang et al., 2015), 植株氮分配(Muryono et al., 2017; Oikawa et al., 2017), 源库结构与植物形态(Tausz-Posch et al., 2015; Nakano et al., 2017), 水分利用(Ikawa et al., 2018), 根系生长和氮吸收(Wu et al., 2018)等多个方面。还有研究表明, 不同水稻品种在CO2浓度升高情况下的抗倒伏能力也会发生变化, 间接影响水稻的产量(Shimono et al., 2007; Zhao et al., 2019)。最新研究发现, 近20年的高CO2浓度处理对C3和C4植物的影响会随着处理时间的延长而发生改变(Hovenden & Newton, 2018; Reich et al., 2018), 说明进行长期实验研究的必要性。增施氮肥可以在一定程度上提高水稻的施肥效应, 进一步提高CO2浓度升高下的产量(Yoshida et al., 2011), 但也有研究发现氮肥与CO2浓度升高对作物的产量没有显著交互作用(Yang et al., 2007; Weigel & Manderscheid, 2012), 而且氮肥增施会进一步增加CO2浓度升高下稻田的甲烷排放(Xu et al., 2004; Xie et al., 2012)。杂草会限制作物的施肥效应。有研究表明, CO2浓度升高虽然会增加小麦30%的产量, 但如果同时受到杂草的影响, 增产则变得不显著(Thompson & Woodward, 1994)。CO2浓度升高(OTC, (570 ± 25) μL·L-1)会提高褐飞虱繁殖能力, 增加其种群个体数量, 从而降低水稻产量(29.9%- 34.9%; Guru-Pirasanna-Pandi et al., 2018)。
CO2浓度升高下作物的品质会受到影响, 包含营养品质、外观品质、加工品质和食味品质。营养品质方面, CO2浓度升高增加植物体内的碳含量, 并对氮、锌、镁等其他元素产生一定的稀释作用(Myers et al., 2014), 而氮含量的相对降低又进一步降低了蛋白质和氨基酸的含量。在CO2浓度升高情况下, 稀释作用只能部分解释氮及蛋白质含量下降这一现象(Taub et al., 2008), 但氮及其他养分的吸收、养分在植物体内运输、各个器官之间的分配都会影响这种现象(Feng et al., 2015)。然而增施氮肥并不能显著改变CO2浓度升高下水稻中氮含量的下降(Yang et al., 2007)。通过FACE模拟实验和APSIM (Agricultural Production System sIMulator)模型模拟研究发现CO2浓度升高下小麦对土壤中氮、钾、镁等养分的吸收能力与蒸腾作用相关, 而且每单位蒸腾水分下的养分吸收也比正常CO2浓度下高, 因此推测得出由蒸腾作用驱动的养分运输是CO2浓度升高减少作物营养成分的机制之一(Houshmandfar et al., 2018)。
除营养品质外, 外观品质也直接影响稻米等作物的加工品质和市场价格。水稻FACE研究发现CO2浓度升高条件下大米的精米率和头等米率显著降低2.0%和23.5%, 精米的垩白籽粒率和垩白度显著增加(Yang et al., 2007; Usui et al., 2016), 籽粒的长度和宽度等形状指标也会发生改变(Jing et al., 2016), 但是不同水稻品种的响应也存在明显差异(Usui et al., 2014)。CO2浓度升高对大米的外观、香味、硬度和黏度等蒸熟品质和食味品质影响不显著(Terao et al., 2005)。
3.2 CO2浓度升高对自然生态系统碳固定的影响
大气CO2浓度升高的“施肥效应”刺激植物光合作用和生产力, 总体上促进陆地植被的碳固定。根据国内外实验数据和模拟预测, CO2浓度倍增后, 中国森林生产力的增幅在12%-35% (Fang, 2002)。但是生态系统的碳汇功能存在很大的争议, 受水分、养分有效性等诸多因素制约。FACE实验结果表明, CO2的施肥效应并非像以往研究那样明显(Long, 2006), 对于气候变化和空气污染引起的生产力的损失也没有像最初模式预测的那样能够得到完全补偿。因此, 尽管大量实验表明大气CO2浓度升高对生态系统有直接施肥效应, 但协同其他胁迫因子如竞争、干旱、氮沉降、O3浓度升高等对陆地生态系统碳汇的影响将非常复杂。CO2浓度升高对整个生态系统碳的影响是非线性的(Gill et al., 2002), 即随着CO2施肥时间的延长, 这种促进的碳汇效应会减弱甚至消失(Oechel et al., 1994; K?rner et al., 2005), 其原因是光适应还是受氮限制目前仍存在争议。一些研究发现CO2浓度升高对生产力的促进作用会受到生态系统氮有效性的限制(即渐进式氮限制, Progressive N Limitation, PNL)(Luo et al., 2004; Reich et al., 2006)。然而Feng等(2015)综合全球8个国家的15个CO2-FACE实验平台在农田、草地和森林生态系统的研究, 发现12年的CO2升高实验对生态系统地上部分碳、氮累积量和植被生产力的促进作用未随时间的延长而显著降低。CO2浓度升高对于土壤“碳汇”功能, 以及对生态系统碳固定(植物和土壤)的综合影响尚不清楚。大气CO2浓度升高既促进地上生物量的增加, 也促进凋落物归还量及地下部分生物量的增加。CO2浓度升高还可增加粗微团聚体形成的稳定性和碳保护功能, 从而提高土壤碳的稳定性(Hoosbeek et al., 2006)。因此, CO2升高可能会导致土壤碳库增加使土壤成为一个潜在的碳汇(Luo et al., 2006)。然而, 在土壤碳输入增加的同时, CO2浓度升高也加速了土壤碳周转, 刺激土壤呼吸(King et al., 2004), 从而限制了土壤碳固定。在橡树林中, CO2倍增实验表明, 植物生长速率增加, 土壤碳含量降低, 主要是由于土壤中真菌丰度提高, 土壤降解酶活性增强, 土壤有机物降解加快, 从而使得潜在的碳库变为碳源(Carney et al., 2007)。因此, CO2浓度升高对土壤碳积累的影响还需要进一步研究。
3.3 CO2浓度升高对生态系统水分利用效率的影响
提高水分利用效率(WUE)是大气CO2浓度升高对植物生长最有利的影响之一。在CO2浓度升高下, 植物出现不同程度的气孔密度和气孔导度下降、Rubisco酶活性提高、羧化效率提高、净光合速率升高, 这一系列的有效生理调控途径导致WUE的提高(Ponton et al., 2006; Keenan et al., 2013), 并且C3植物WUE比C4植物响应程度更大(Wand et al., 1999)。目前, 主要通过便携式光合仪、碳同位素(13C)和涡度相关等手段分别对叶片水平(光合速率/蒸腾速率)、植株水平(干物质量/总蒸腾量)和生态系统水平(干物质量/总蒸散量)的WUE进行观测。表2显示CO2浓度升高显著促进了各种植物的WUE (Cox et al., 2013; Huntingford et al., 2013; Kaminsk et al., 2014; Zscheischler et al., 2014; Sitch et al., 2015), 但物种之间的增加幅度存在较大差异。高氮肥下的WUE增加幅度比低氮肥下的大(Norby & O’Neill, 1991; Cruz et al., 2016)。目前的可能解释为: 在氮供应充足的情况下, CO2浓度升高使叶片的光合速率显著提高, 消耗吸收光能的增幅抵消了因光呼吸降低而导致的吸收光能消耗的降幅, 从而减轻了光抑制, 未出现光适应现象; 而在氮不足时, CO2浓度升高使光合速率下降, 再加上光呼吸降低而导致的吸收光能消耗的降低, 导致光抑制加重, 出现光适应现象(王润佳等, 2010)。因此, CO2浓度升高条件下植物在低氮水平下可能出现光合作用适应性下调, 导致净光合速率增幅变小, 从而导致WUE的增幅变缓。Table 2
表2
表2CO2浓度升高对植物水分利用效率(WUE)的生态正效应
Table 2
植物名称 Plant name | 暴露时间(天) Exposure time (Day) | WUE (%) | 参考文献 Reference |
---|---|---|---|
海岸松 Pinus pinaster | 79 | +48 | Picon et al., 1996 |
垂枝桦 Betula pendula | 45 | +39 | Pettersson et al., 1993 |
北美鹅掌楸 Liriodendron tulipifera | Norby & O’Neill, 1991 | ||
高营养 High nutrition | 168 | +93 | |
低营养 Low nutrition | 168 | +66 | |
Tabebuia rosea | - | +125 | Ziska et al., 1991 |
木瓜 Chaenomeles sinensis | Cruz et al., 2016 | ||
高氮 High nitrogen | 45 | +84 | |
低氮 Low nitrogen | 45 | +30 |
新窗口打开|下载CSV
CO2与其他环境因子复合作用时, WUE的响应会发生显著变化。如干旱促进WUE上升(Kang et al., 2002), 而升温却相反(Hatfield et al., 2011)。因此, 在面对全球变化多因子共存时, 单一考虑CO2对WUE的影响显然不足以准确预测其变化趋势, 需要进一步全面研究WUE与CO2、O3、温度、土壤水分和养分等环境变化因子的相互作用关系。
3.4 CO2浓度升高对生态系统氮利用的影响
CO2浓度升高显著降低植物组织氮含量(Cotrufo et al., 1998), 包括植物氮浓度(-12%)和叶片氮浓度(-15%)(Loladze, 2014; Deng et al., 2015)。但由于CO2浓度升高对生物量的促进作用, 植物氮库并未减小反而显著增加(Deng et al., 2015)。CO2浓度升高条件下叶片氮浓度降低的机理目前还不十分清楚, 可能的解释为生长稀释效应, 叶片碳水化合物累积导致的Rubisco含量降低, 叶片内NO3-同化受抑制, 植物吸氮能力受限, 根系吸收速率的降低, 土壤氮供应不足以及NH3蒸发和分泌物的释放等(Bloom et al., 2002, 2014; Long et al., 2004; Taub & Wang, 2008; Feng et al., 2015)。长期而言, CO2浓度升高对植物生长的刺激作用往往会导致更多的氮被固存在多年生的植物体内和土壤有机质中(Luo et al., 2006), 从而导致植物可利用氮的含量降低, 削弱植物生长对CO2浓度升高的响应, 形成PNL (Luo et al., 2004; Reich et al., 2006; Norby et al., 2010), 这种现象多发生在氮限制的生态系统中, 高浓度CO2条件下土壤中可利用氮含量(NH4+-N和NO3--N)的降低也支持这种观点(Dieleman et al., 2012)。Meta分析表明土壤总氮矿化、硝化作用和NH4+的消耗都未受CO2浓度升高的显著影响(Rutting & Andresen, 2015)。不同生态系统对CO2浓度升高下的PNL响应存在差异。现有研究表明, PNL多发生在草地生态系统(Reich et al., 2006), 而在森林生态系统中仅在ORNL-FACE出现。在氮限制的森林生态系统中, 植物可通过提高总地下碳通量来刺激氮的吸收以使冠层叶面积和冠层氮含量增加从而维持净初级生产力(NPP)对CO2浓度升高的响应(Drake et al., 2011; Phillips et al., 2012)。CO2浓度升高条件下可能存在多种途径来增加氮的吸收、维持NPP的增加以补偿其导致的氮限制, 包括改变根系的构型, 增加光合产物向细根和菌根的分配, 促进根际沉积物的释放, 刺激真菌对氮的矿化等(Phillips et al., 2011; Cheng et al., 2012)。ORNL-FACE与其他森林FACE响应的不同可能是因为不同森林土壤微生物系统的差异(Terrer et al., 2018), 例如外生菌根系统而不是丛枝菌根系统(Drake et al., 2011), 这在下一节中将详细讨论。另一种可能性是目前实验处理时间不够长, 如果处理足够长的时间(>20年), 所有实验可能因为高浓度CO2发生NPP下调的反应(Luo et al., 2004)。
3.5 生态系统土壤微生物对CO2浓度升高的响应
CO2浓度升高会增加根际沉积(Pendall et al., 2004)、凋落物输入和地下部分的碳输入, 进而增加土壤微生物对碳的可利用性(Hungate et al., 1997)。Meta分析显示CO2浓度升高引起土壤微生物生物量碳和氮增加分别占统计样本量的48%和44%, 其余显示降低或者不变(Hu et al., 2006)。一些重要的土壤功能微生物如丛枝菌根真菌(AMF)丰富度在CO2浓度升高下平均增加21% (Alberton et al., 2005)。微生物呼吸速率在CO2浓度升高下平均增加17% (de Graaff et al., 2006); 但微生物酶活性的响应缺乏一致性: CO2浓度升高对土壤中胞外酶活性有促进(Dorodnikov et al., 2009)、抑制(Austin et al., 2009)或者无作用(Billings & Ziegler, 2005)。一般认为CO2浓度升高促进碳输入对真菌的生长更为有利, 但是研究发现真菌细菌的比例随着CO2浓度升高可能出现增加、降低或者不变的响应(Hungate et al., 1997; Jones et al., 1998; Carney et al., 2007); 产生这种不一致的原因尚不清楚, 可能与底物质量和数量、土壤温度、pH、土壤碳氮比等因素有关(Zhang et al., 2005; Fierer et al., 2009)。在大气CO2浓度升高条件下, 土壤微生物通过调控土壤过程对植物生长可能产生正或负效应。短时间的CO2浓度升高条件下微生物促进有机氮矿化, 增加土壤氮的有效性和植物对无机氮的吸收(Hungate et al., 1997; Zak et al., 2000; de Graaff et al., 2006); 但这种正反馈作用可能因为土壤PNL难以持续较长时间(Luo et al., 2004)。因此, 长时间CO2浓度升高条件下土壤微生物通过降低土壤氮的有效供给造成植物缺氮的负反馈效应(Finzi et al., 2006; Reich et al., 2006)。然而, 植物也可能通过与微生物互作的机制来避免PNL的发生, 比如植物自身的根系C:N会增加, 从而促使土壤中的N向C:N高的根系转移(Lichter et al., 2008); 或者植物通过土壤微生物(菌根真菌)直接吸收有机小分子氮的方式来缓解氮限制(Cheng et al., 2012)。Terrer等(2018)最新研究显示, 具有外生菌根(ECM)系统的生态系统可分解难分解凋落物, 直接吸收土壤中的有机氮, 为植物提供充足的有机无机氮, 避免PNL发生, 在CO2浓度升高条件下植物碳库增加, 土壤呼吸增加, 土壤有机碳库降低; 而具有丛枝菌根(AM)系统的生态系统只能为植物提供无机氮, 在从凋落物转化为可供利用的无机氮的复杂过程中, 特别是在CO2浓度升高条件下, 微生物提供的可利用氮库往往跟不上植物对氮的需求, 较易发生PNL, 从而使得土壤呼吸变弱, 生物量积累变小。由于大量的氮来不及被植物利用, 土壤有机碳库增加(Terrer et al., 2018)。目前植物与微生物互馈作用是研究的热点。
CO2浓度升高条件下土壤微生物也可能通过改变土壤中的分解过程对大气CO2浓度产生正或负的反馈作用。一方面, CO2浓度升高可引起微生物活性增加(Zak et al., 2000; de Graaff et al., 2006), 促进微生物呼吸作用, 也促进微生物分解更多的来自植物的碳(如凋落物、根系分泌物); 而且, CO2浓度升高可能会通过激发效应促进微生物对土壤易分解(Carney et al., 2007)和难分解(Langley et al., 2009)有机碳的分解(Terrer et al., 2018), 进而改变土壤碳的长期稳定性, 从而使得更多的碳返回大气, 对大气CO2浓度形成正反馈作用。另一方面, CO2浓度升高也可能通过降低土壤中氮的有效性和植物凋落物的质量(如C:N增加)来抑制土壤微生物的分解速率(Norby et al., 2001a; Booker et al., 2005), 从而减少土壤碳的流失形成负反馈作用。土壤微生物对大气CO2浓度的反馈作用尚无定论, 影响因素包括不同生态系统、不同持续时间、不同实验手段等方面, 仍缺乏区域和全球尺度上的定论。
4 前沿方向展望
理解生态系统对过去、现在和未来CO2浓度变化的响应对于在生态进化的时间尺度上认识和预测全球变化的后果至关重要。过去二十多年来在CO2浓度升高对植物光合和生长、生产力和产量影响等方面取得较大进展, 但是对于整个生态系统结构和功能的响应仍然存在一定挑战和较大的不确定性。Becklin等(2017)举了3个例子强调为什么CO2研究对于全球碳-氮-水循环、食品安全和生态系统服务等方面比以往更加关键。我们仍然面临的巨大挑战是: CO2浓度升高和气候之间的相互作用是如何调控从工业革命前到现代的植物发育、生理和物种相互作用? 对过去环境适应而现存的植物种群、群落和生态系统将如何适应未来CO2浓度升高的环境? CO2浓度升高和气候变化将如何驱动植物性状(及植物拮抗体和共生体性状)的进化? 对于CO2浓度升高的适应有多快? 哪些性状能作为利用额外的碳资源以提高粮食作物产量的潜在目标? 在大的时间尺度和不同的生物组织水平上, 对CO2浓度升高的非线性响应的驱动机制? 我们如何将对CO2浓度升高的非线性反应和性状的变异特征纳入全球变化模型? 植物和其他有机体之间复杂的生物相互作用将如何影响植物对CO2浓度升高的响应轨迹? 这些生态效应如何影响粮食安全和生态系统服务? 我们如何将分子水平的数据尺度转换到生态系统过程模型中来预测有机体到生态系统过程的响应? 这些全球变化研究领域内悬而未决的热点问题, 将有助于我们更深刻理解地球生态系统的响应并与我们的未来命运休戚相关。4.1 地下生态过程与植物-土壤交互作用机制
过去对于土壤微生物的研究多局限于CO2浓度升高对土壤微生物的影响, 而土壤微生物对植物、对气候变化的反馈作用机制研究相对缺乏。其中的研究重点和热点包括: 一、运用稳定同位素、分子与基因组学等相关技术耦合, 定向研究土壤微生物群落以及相关功能微生物, 有助于揭示微生物调控碳氮循环等耦合过程和机理(Cheng et al., 2012)。二、土壤微生物调控的养分(特别是氮)转化机制。在自然或半自然生态系统中, PNL形成及其对植物氮的有效性影响将是关注的重点。在农业生态系统中, 由于大量人工氮肥输入, 关注的重点将是氮在土壤-植物体系中的分配、周转、流失等过程与分子机理及其与碳的耦合机制, 对于未来CO2浓度升高条件下保障粮食安全、水体污染控制和缓解气候变暖都具有重要意义。前沿的热点问题包括: 不同时空尺度的土壤微生物是如何调控不同生态系统碳氮循环, 以及在未来CO2浓度持续升高条件下, 全球土壤是碳库还是碳源? 土壤微生物调控的土壤养分有效性变化是否以及如何影响植物与生态系统对CO2浓度升高的响应? 生态系统PNL形成与土壤微生物-植物互作的相互关系等。4.2 多因子复合研究
全球变化因子间通常不是独立的, 而是相互作用的。目前对CO2浓度升高单因子变化研究取得较大进展, 而多因子交互作用的研究较少, 研究结果存在很大的不确定性。全球变化包括了CO2/O3浓度升高、干旱胁迫、氮沉降和降水格局分布等多因子复合影响, 生态系统在复合因子影响下往往呈现复杂的作用特征。比如O3浓度升高和增温对于植物的负效应往往能抵消一部分CO2浓度升高的“施肥”正效应(Karnosky et al., 2003; Way et al., 2015), 而抵消的强度与生态系统类型、物种、增温幅度、升高的CO2/O3浓度、实验持续时间等因素相关。未来的研究需要结合多种手段, 加强高浓度CO2与多个全球变化因子的交互作用对生态系统过程和功能的综合影响研究, 明确短期响应与长期适应规律, 深入揭示格局背后的环境控制机制和生物驱动机制。4.3 气候变化与粮食安全
随着人们生活水平的提高, 对饮食的需求正在从过去的数量型向现在的营养型转变。21世纪的粮食安全将由以数量为主转向数量与质量并重的方向发展, 不仅要解决全世界人口的温饱问题, 还要对粮食的营养价值给予足够的重视。在全球变化等多因子的复合作用下, CO2浓度升高对作物增产的正效应能被O3浓度升高(Feng & Kobayashi, 2009)和增温(Wang et al., 2015; Chaturvedi et al., 2017)对农作物的伤害和抑制作用所抵消。同时, CO2浓度升高条件下农产品的蛋白质含量普遍下降(Uddling et al., 2018), 作物营养和外观等品质的下降也会在增温复合作用下进一步恶化(Chaturvedi et al., 2017)。这势必将加剧人口饮食中的蛋白质和铁、锌等人体必需微量元素摄入不足造成的“隐性饥饿” (Uddling et al., 2018); 同时进一步增加CH4的排放(Wang et al., 2018), 对气候变暖产生正反馈作用。2050年之前, 世界粮食产量需要至少增加70%才能满足未来人口的需求(Godfray et al., 2010), 而大气CO2浓度将升至550 μL·L-1, 我们急需理解CO2浓度升高对作物的产量与品质的影响机理, 筛选和培养适应的作物品种并调节开发适应未来气候调节的田间管理技术以保障粮食安全。在未来CO2浓度升高情况下, 调整种植期以改变作物物候, 调节种植密度或兼作轮作来优化作物的微气候, 调整肥料施用的数量、频度和方式来提高作物的肥料利用效率, 以及灌溉水、除草、病虫害防治等都需要深入研究。同时, 在不同地区模拟各种环境因素与高浓度CO2对农产品产量和品质影响的交互作用, 这对准确预测全球变化下农作物产量和品质变化趋势及农业生产管理措施的调整等方面具有重要的现实意义。4.4 联网实验
随着全球尺度高密度生态网络观测的发展, 大型联网控制实验系统的完善, 以及大数据时代的多源数据整合分析技术的日臻成熟, CO2研究逐步从单一生态过程研究向多过程耦合研究发展, 从典型生态系统向区域、全球尺度生态系统综合集成研究转变。基于生态系统网络的多尺度、长期观测与联网实验将揭示单个站点所无法回答的大尺度的生态问题。目前陆地生态系统与大气和气候变化研究网络(Terrestrial Ecosystem Responses to Atmosphere and Climate Change, TERACC)在全球已经囊括数百个全球变化控制实验研究站点(Rustad, 2008)。但亚洲国家的研究站点以及加入全球的联网实验仍然缺乏。与全球联网实验的融合将加强我国生态系统与全球其他区域生态系统功能的联合对比研究, 揭示全球CO2浓度升高对不同区域生态系统过程、结构和功能的影响, 有助于开展生态系统地表过程的多途径与多过程模拟分析, 为全球陆地模型降低碳循环预测的不确定性提供了可比性更高的数据支撑。4.5 热带及亚热带区域研究
现有的模拟CO2浓度升高的研究对不同生态系统关注度存在较大的差异。实验系统多建立在温带草原和森林生态系统中, 并且多处在北美和欧洲。对广泛分布于发展中国家和地区的热带和亚热带雨林、稀树草原知之甚少。热带和亚热带生态系统包含了陆地约50%的碳储量, 大气氮沉降和土壤氮有效性与温带、寒温带地区有明显差异(Tian et al., 2018), 对CO2浓度升高的响应也与研究得较为透彻的温带区域(Cernusak et al., 2013; Jones et al., 2014)不同, 而在热带亚热带地区未见有FACE实验站点的报道。理解热带亚热带森林、草原和农田生态系统对于CO2浓度升高的响应, 是减缓气候变化、保障粮食供应的战略重点。加入热带亚热带的研究数据, 也为模型评估未来气候变化对全球固碳、粮食安全的影响提高了预测精度。然而, 由于热带森林生态系统具有高的生物多样性和复杂的地貌特征, 建立一个具有代表性的FACE系统面临着一定挑战, 需要考虑物种-面积的代表性、电力和CO2供应、道路的便利等因素的限制(Cernusak et al., 2013)。4.6 长时间控制实验研究
生态系统对于CO2浓度升高需要时间来响应和与适应。叶片尺度光合速率适应CO2水平的变化可能需要3-4周, 但对于整个群落来说适应性反应时间可能会很长。这样的驯化作用发生在诸如碳分配、水分和氮利用、细根分解和周转等一些功能性状上。这些连锁的功能反应可能在未来几十年后导致自然生态系统结构改变, 对于森林生态系统来说或许需要几十年或更长时间, 然而目前发表的研究都在一个相对短的时间尺度上(10年左右), 短于森林树木的生命周期。因此, 模拟CO2浓度升高对生态系统影响的实验需要长时间控制实验研究, 把所有对CO2浓度变化的群落动力学反应都包括进去。然而, 长时间控制实验存在年际的气候变异问题。这些年际间的气候变化也将影响群落和生态系统过程, 如土壤碳储存和水分关系。除非群落实验年复一年地控制稳定的气候, 否则实验将由于CO2浓度和气候的改变而被混淆。这对于旨在研究CO2浓度单因子的实验, 群落和生态系统水平将是一个严峻挑战, 但也是理解整个生态系统对全球变化响应的关键。卫星遥感被证明是一种有用的工具, 可以扩展对生态系统影响研究的时间尺度和空间尺度。遥感反映的不单单是CO2浓度的变化, 也包括了气候的变化和其他人为因素, 比如大气中O3浓度的增加等。5 总结
CO2浓度升高关注的科学问题主要集中在对植物生长和生产力的影响, CO2浓度升高与碳氮周转, 生态系统PNL形成, CO2浓度升高与其他胁迫因子(O3污染、氮沉降、升温、干旱)之间的交互作用等方面。尽管在数据累积和基础理论上取得一定进展, 但仍然存在大量未知有待解决。新技术手段和方法的应用, 新研究网络的建立, 将有助于我们更好地阐述CO2浓度升高条件下生态系统所面临的一些重要科学问题, 这些问题的解决不仅对于我国面临的一些重大战略需求, 如气候变化、粮食安全和生态文明建设至关重要, 也对整个生态系统健康和可持续发展具有重要意义。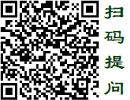
致谢
感谢南京信息工程大学人才启动基金(002992)资助。参考文献 原文顺序
文献年度倒序
文中引用次数倒序
被引期刊影响因子
DOI:10.1111/j.1469-8137.2004.01224.xURLPMID:15720649 [本文引用: 1]

Free-air CO(2) enrichment (FACE) experiments allow study of the effects of elevated [CO(2)] on plants and ecosystems grown under natural conditions without enclosure. Data from 120 primary, peer-reviewed articles describing physiology and production in the 12 large-scale FACE experiments (475-600 ppm) were collected and summarized using meta-analytic techniques. The results confirm some results from previous chamber experiments: light-saturated carbon uptake, diurnal C assimilation, growth and above-ground production increased, while specific leaf area and stomatal conductance decreased in elevated [CO(2)]. There were differences in FACE. Trees were more responsive than herbaceous species to elevated [CO(2)]. Grain crop yields increased far less than anticipated from prior enclosure studies. The broad direction of change in photosynthesis and production in elevated [CO(2)] may be similar in FACE and enclosure studies, but there are major quantitative differences: trees were more responsive than other functional types; C(4) species showed little response; and the reduction in plant nitrogen was small and largely accounted for by decreased Rubisco. The results from this review may provide the most plausible estimates of how plants in their native environments and field-grown crops will respond to rising atmospheric [CO(2)]; but even with FACE there are limitations, which are also discussed.
DOI:10.1111/j.1469-8137.2005.01458.xURLPMID:16101922 [本文引用: 1]

The aim here was to separately assess mycorrhizal fungal and plant responses under elevated atmospheric CO2, and to test a mycocentric model that assumes that increased carbon availability to the fungus will not automatically feed back to enhanced plant growth performance. Meta-analyses were applied across independent studies. Responses were compared in ectomycorrhizal (ECM) and arbuscular mycorrhizal (AM) fungi, and ECM and AM plants. Responses of both mycorrhizal fungi and mycorrhizal plants to elevated CO2 were significantly positive. The response ratio for ECM fungi was 1.34 (an increase of 34%) and for AM fungi 1.21 (21%), indicating a significantly different response. The response ratio for ECM plants was 1.26, similar to that of AM plants (1.25). Fractional colonization proved to be an unsuitable fungal parameter. Evidence was found for the mycocentric view in ECM, but not in AM systems. Fungal identity and plant identity were important parameters that affected response ratios. The need for better descriptors of fungal and plant responses is emphasized.
DOI:10.1016/j.soilbio.2008.12.010URL [本文引用: 1]
DOI:10.1111/nph.14336URLPMID:27891618 [本文引用: 2]

Contents 34 I. 34 II. 36 III. 37 IV. 37 V. 38 38 References 38 SUMMARY: Characterizing plant responses to past, present and future changes in atmospheric carbon dioxide concentration ([CO2 ]) is critical for understanding and predicting the consequences of global change over evolutionary and ecological timescales. Previous CO2 studies have provided great insights into the effects of rising [CO2 ] on leaf-level gas exchange, carbohydrate dynamics and plant growth. However, scaling CO2 effects across biological levels, especially in field settings, has proved challenging. Moreover, many questions remain about the fundamental molecular mechanisms driving plant responses to [CO2 ] and other global change factors. Here we discuss three examples of topics in which significant questions in CO2 research remain unresolved: (1) mechanisms of CO2 effects on plant developmental transitions; (2) implications of rising [CO2 ] for integrated plant-water dynamics and drought tolerance; and (3) CO2 effects on symbiotic interactions and eco-evolutionary feedbacks. Addressing these and other key questions in CO2 research will require collaborations across scientific disciplines and new approaches that link molecular mechanisms to complex physiological and ecological interactions across spatiotemporal scales.
DOI:10.1111/gcb.2005.11.issue-2URL [本文引用: 1]
DOI:10.1111/pce.12443URLPMID:25211487 [本文引用: 1]

Rising atmospheric [CO2] is a uniform, global change that increases C3 photosynthesis and could offset some of the negative effects of global climate change on crop yields. Genetic variation in yield responsiveness to rising [CO2] would provide an opportunity to breed more responsive crop genotypes. A multi-year study of 18 soybean (Glycine max Merr.) genotypes was carried out to identify variation in responsiveness to season-long elevated [CO2] (550 ppm) under fully open-air replicated field conditions. On average across 18 genotypes, elevated [CO2] stimulated total above-ground biomass by 22%, but seed yield by only 9%, in part because most genotypes showed a reduction in partitioning of energy to seeds. Over four years of study, there was consistency from year to year in the genotypes that were most and least responsive to elevated [CO2], suggesting heritability of CO2 response. Further analysis of six genotypes did not reveal a photosynthetic basis for the variation in yield response. Although partitioning to seed was decreased, cultivars with the highest partitioning coefficient in current [CO2 ] also had the highest partitioning coefficient in elevated [CO2]. The results show the existence of genetic variation in soybean response to elevated [CO2], which is needed to breed soybean to the future atmospheric environment.
DOI:10.1038/nclimate2183URL [本文引用: 1]
DOI:10.1073/pnas.022627299URLPMID:11818528 [本文引用: 1]

Simultaneous measurements of CO(2) and O(2) fluxes from wheat (Triticum aestivum) shoots indicated that short-term exposures to elevated CO(2) concentrations diverted photosynthetic reductant from NO(3)(-) or NO(2)(-) reduction to CO(2) fixation. With longer exposures to elevated CO(2), wheat leaves showed a diminished capacity for NO(3)(-) photoassimilation at any CO(2) concentration. Moreover, high bicarbonate levels impeded NO(2)(-) translocation into chloroplasts isolated from wheat or pea leaves. These results support the hypothesis that elevated CO(2) inhibits NO(3)(-) photoassimilation. Accordingly, when wheat plants received NO(3)(-) rather than NH(4)(+) as a nitrogen source, CO(2) enhancement of shoot growth halved and CO(2) inhibition of shoot protein doubled. This result will likely have major implications for the ability of wheat to use NO(3)(-) as a nitrogen source under elevated CO(2).
DOI:10.1111/gcb.2005.11.issue-4URL [本文引用: 1]
DOI:10.1016/j.eja.2017.05.003URL [本文引用: 1]
DOI:10.1016/j.fcr.2015.11.010URL [本文引用: 1]
DOI:10.3390/agronomy7010020URL [本文引用: 1]
DOI:10.1073/pnas.0610045104URLPMID:17360374 [本文引用: 3]

Increased carbon storage in ecosystems due to elevated CO(2) may help stabilize atmospheric CO(2) concentrations and slow global warming. Many field studies have found that elevated CO(2) leads to higher carbon assimilation by plants, and others suggest that this can lead to higher carbon storage in soils, the largest and most stable terrestrial carbon pool. Here we show that 6 years of experimental CO(2) doubling reduced soil carbon in a scrub-oak ecosystem despite higher plant growth, offsetting approximately 52% of the additional carbon that had accumulated at elevated CO(2) in aboveground and coarse root biomass. The decline in soil carbon was driven by changes in soil microbial composition and activity. Soils exposed to elevated CO(2) had higher relative abundances of fungi and higher activities of a soil carbon-degrading enzyme, which led to more rapid rates of soil organic matter degradation than soils exposed to ambient CO(2). The isotopic composition of microbial fatty acids confirmed that elevated CO(2) increased microbial utilization of soil organic matter. These results show how elevated CO(2), by altering soil microbial communities, can cause a potential carbon sink to become a carbon source.
DOI:10.1071/FP12309URL [本文引用: 2]

Elevated atmospheric CO2 concentrations (ca) will undoubtedly affect the metabolism of tropical forests worldwide; however, critical aspects of how tropical forests will respond remain largely unknown. Here, we review the current state of knowledge about physiological and ecological responses, with the aim of providing a framework that can help to guide future experimental research. Modelling studies have indicated that elevated ca can potentially stimulate photosynthesis more in the tropics than at higher latitudes, because suppression of photorespiration by elevated ca increases with temperature. However, canopy leaves in tropical forests could also potentially reach a high temperature threshold under elevated ca that will moderate the rise in photosynthesis. Belowground responses, including fine root production, nutrient foraging and soil organic matter processing, will be especially important to the integrated ecosystem response to elevated ca. Water use efficiency will increase as ca rises, potentially impacting upon soil moisture status and nutrient availability. Recruitment may be differentially altered for some functional groups, potentially decreasing ecosystem carbon storage. Whole-forest CO2 enrichment experiments are urgently needed to test predictions of tropical forest functioning under elevated ca. Smaller scale experiments in the understorey and in gaps would also be informative, and could provide stepping stones towards stand-scale manipulations.
DOI:10.1016/j.fcr.2017.02.018URL [本文引用: 2]
DOI:10.1093/pcp/pcu009URLPMID:24443497 [本文引用: 1]

The development of crops which are well suited to growth under future environmental conditions such as higher atmospheric CO2 concentrations ([CO2]) is essential to meeting the challenge of ensuring food security in the face of the growing human population and changing climate. A high-yielding indica rice variety (Oryza sativa L. cv. Takanari) has been recently identified as a potential candidate for such breeding, due to its high productivity in present [CO2]. To test if it could further increase its productivity under elevated [CO2] (eCO2), Takanari was grown in the paddy field under season-long free-air CO2 enrichment (FACE, approximately 200 micromol mol(-1) above ambient [CO2]) and its leaf physiology was compared with the representative japonica variety 'Koshihikari'. Takanari showed consistently higher midday photosynthesis and stomatal conductance than Koshihikari under both ambient and FACE growth conditions over 2 years. Maximum ribulose-1,5-bisphosphate carboxylation and electron transport rates were higher for Takanari at the mid-grain filling stage in both years. Mesophyll conductance was higher in Takanari than in Koshihikari at the late grain-filling stage. In contrast to Koshihikari, Takanari grown under FACE conditions showed no decrease in total leaf nitrogen on an area basis relative to ambient-grown plants. Chl content was higher in Takanari than in Koshihikari at the same leaf nitrogen level. These results indicate that Takanari maintains its superiority over Koshihikari in regards to its leaf-level productivity when grown in elevated [CO2] and it may be a valuable resource for rice breeding programs which seek to increase crop productivity under current and future [CO2].
DOI:10.1126/science.1224304URLPMID:22936776 [本文引用: 3]

The extent to which terrestrial ecosystems can sequester carbon to mitigate climate change is a matter of debate. The stimulation of arbuscular mycorrhizal fungi (AMF) by elevated atmospheric carbon dioxide (CO(2)) has been assumed to be a major mechanism facilitating soil carbon sequestration by increasing carbon inputs to soil and by protecting organic carbon from decomposition via aggregation. We present evidence from four independent microcosm and field experiments demonstrating that CO(2) enhancement of AMF results in considerable soil carbon losses. Our findings challenge the assumption that AMF protect against degradation of organic carbon in soil and raise questions about the current prediction of terrestrial ecosystem carbon balance under future climate-change scenarios.
DOI:10.1046/j.1365-2486.1998.00101.xURL [本文引用: 1]
DOI:10.1038/nature11882URL [本文引用: 1]

The release of carbon from tropical forests may exacerbate future climate change(1), but the magnitude of the effect in climate models remains uncertain(2). Coupled climate-carbon-cycle models generally agree that carbon storage on land will increase as a result of the simultaneous enhancement of plant photosynthesis and water use efficiency under higher atmospheric CO2 concentrations, but will decrease owing to higher soil and plant respiration rates associated with warming temperatures(3). At present, the balance between these effects varies markedly among coupled climate-carbon-cycle models, leading to a range of 330 gigatonnes in the projected change in the amount of carbon stored on tropical land by 2100. Explanations for this large uncertainty include differences in the predicted change in rainfall in Amazonia(4,5) and variations in the responses of alternative vegetation models to warming(6). Here we identify an emergent linear relationship, across an ensemble of models(7), between the sensitivity of tropical land carbon storage to warming and the sensitivity of the annual growth rate of atmospheric CO2 to tropical temperature anomalies(8). Combined with contemporary observations of atmospheric CO2 concentration and tropical temperature, this relationship provides a tight constraint on the sensitivity of tropical land carbon to climate change. We estimate that over tropical land from latitude 30 degrees north to 30 degrees south, warming alone will release 53 +/- 17 gigatonnes of carbon per kelvin. Compared with the unconstrained ensemble of climate-carbon-cycle projections, this indicates a much lower risk of Amazon forest die-back under CO2-induced climate change if CO2 fertilization effects are as large as suggested by current models(9). Our study, however, also implies greater certainty that carbon will be lost from tropical land if warming arises from reductions in aerosols(10) or increases in other greenhouse gases(11).
DOI:10.1016/j.scienta.2016.02.010URL [本文引用: 1]
DOI:10.1111/gcb.2006.12.issue-11URL [本文引用: 3]
DOI:10.1890/15-0217.1URLPMID:26909440 [本文引用: 2]

Increasing atmospheric CO2 concentrations generally alter element stoichiometry in plants. However, a comprehensive evaluation of the elevated CO2 impact on plant nitrogen: phosphorus (N:P) ratios and the underlying mechanism has not been conducted. We synthesized the results from 112 previously published studies using meta-analysis to evaluate the effects of elevated CO2 on the N:P ratio of terrestrial plants and to explore the underlying mechanism based on plant growth and soil P dynamics. Our results show that terrestrial plants grown under elevated CO2 had lower N:P ratios in both above- and belowground biomass across different ecosystem types. The response ratio for plant N:P was negatively correlated with the response ratio for plant growth in croplands and grasslands, and showed a stronger relationship for P than for N. In addition, the CO2-induced down-regulation of plant N:P was accompanied by 19.3% and 4.2% increases in soil phosphatase activity and labile P, respectively, and a 10.1% decrease in total soil P. Our results show that down-regulation of plant N:P under elevated CO2 corresponds with accelerated soil P cycling. These findings should be useful for better understanding of terrestrial plant stoichiometry in response to elevated CO2 and of the underlying mechanisms affecting nutrient dynamics under climate change.
DOI:10.1111/j.1365-2486.2012.02745.xURLPMID:24501048 [本文引用: 1]

In recent years, increased awareness of the potential interactions between rising atmospheric CO2 concentrations ([ CO2 ]) and temperature has illustrated the importance of multifactorial ecosystem manipulation experiments for validating Earth System models. To address the urgent need for increased understanding of responses in multifactorial experiments, this article synthesizes how ecosystem productivity and soil processes respond to combined warming and [ CO2 ] manipulation, and compares it with those obtained in single factor [ CO2 ] and temperature manipulation experiments. Across all combined elevated [ CO2 ] and warming experiments, biomass production and soil respiration were typically enhanced. Responses to the combined treatment were more similar to those in the [ CO2 ]-only treatment than to those in the warming-only treatment. In contrast to warming-only experiments, both the combined and the [ CO2 ]-only treatments elicited larger stimulation of fine root biomass than of aboveground biomass, consistently stimulated soil respiration, and decreased foliar nitrogen (N) concentration. Nonetheless, mineral N availability declined less in the combined treatment than in the [ CO2 ]-only treatment, possibly due to the warming-induced acceleration of decomposition, implying that progressive nitrogen limitation (PNL) may not occur as commonly as anticipated from single factor [ CO2 ] treatment studies. Responses of total plant biomass, especially of aboveground biomass, revealed antagonistic interactions between elevated [ CO2 ] and warming, i.e. the response to the combined treatment was usually less-than-additive. This implies that productivity projections might be overestimated when models are parameterized based on single factor responses. Our results highlight the need for more (and especially more long-term) multifactor manipulation experiments. Because single factor CO2 responses often dominated over warming responses in the combined treatments, our results also suggest that projected responses to future global warming in Earth System models should not be parameterized using single factor warming experiments.
[本文引用: 1]
DOI:10.1111/j.1461-0248.2011.01593.xURL [本文引用: 2]

P>The earth's future climate state is highly dependent upon changes in terrestrial C storage in response to rising concentrations of atmospheric CO2. Here we show that consistently enhanced rates of net primary production (NPP) are sustained by a C-cascade through the root-microbe-soil system; increases in the flux of C belowground under elevated CO2 stimulated microbial activity, accelerated the rate of soil organic matter decomposition and stimulated tree uptake of N bound to this SOM. This process set into motion a positive feedback maintaining greater C gain under elevated CO2 as a result of increases in canopy N content and higher photosynthetic N-use efficiency. The ecosystem-level consequence of the enhanced requirement for N and the exchange of plant C for N belowground is the dominance of C storage in tree biomass but the preclusion of a large C sink in the soil.
[本文引用: 1]
DOI:10.1016/j.atmosenv.2008.11.033URL [本文引用: 1]
DOI:10.1111/gcb.12938URLPMID:25846203 [本文引用: 5]

A key part of the uncertainty in terrestrial feedbacks on climate change is related to how and to what extent nitrogen (N) availability constrains the stimulation of terrestrial productivity by elevated CO2 (eCO2 ), and whether or not this constraint will become stronger over time. We explored the ecosystem-scale relationship between responses of plant productivity and N acquisition to eCO2 in free-air CO2 enrichment (FACE) experiments in grassland, cropland and forest ecosystems and found that: (i) in all three ecosystem types, this relationship was positive, linear and strong (r(2) = 0.68), but exhibited a negative intercept such that plant N acquisition was decreased by 10% when eCO2 caused neutral or modest changes in productivity. As the ecosystems were markedly N limited, plants with minimal productivity responses to eCO2 likely acquired less N than ambient CO2 -grown counterparts because access was decreased, and not because demand was lower. (ii) Plant N concentration was lower under eCO2 , and this decrease was independent of the presence or magnitude of eCO2 -induced productivity enhancement, refuting the long-held hypothesis that this effect results from growth dilution. (iii) Effects of eCO2 on productivity and N acquisition did not diminish over time, while the typical eCO2 -induced decrease in plant N concentration did. Our results suggest that, at the decennial timescale covered by FACE studies, N limitation of eCO2 -induced terrestrial productivity enhancement is associated with negative effects of eCO2 on plant N acquisition rather than with growth dilution of plant N or processes leading to progressive N limitation.
DOI:10.1111/j.1461-0248.2009.01360.xURLPMID:19674041 [本文引用: 1]

1300 datapoints) to compare belowground plant, microbial and faunal biomass across seven of the major biomes on Earth. We also assembled data to assess biome-level patterns in belowground microbial community composition. Our analysis suggests that variation in microbial biomass is predictable across biomes, with microbial biomass carbon representing 0.6-1.1% of soil organic carbon (r(2) = 0.91) and 1-20% of total plant biomass carbon (r(2) = 0.42). Approximately 50% of total animal biomass can be found belowground and soil faunal biomass represents
DOI:10.1890/04-1748URLPMID:16634293 [本文引用: 1]

A hypothesis for progressive nitrogen limitation (PNL) proposes that net primary production (NPP) will decline through time in ecosystems subjected to a step-function increase in atmospheric CO2. The primary mechanism driving this response is a rapid rate of N immobilization by plants and microbes under elevated CO2 that depletes soils of N, causing slower rates of N mineralization. Under this hypothesis, there is little long-term stimulation of NPP by elevated CO2 in the absence of exogenous inputs of N. We tested this hypothesis using data on the pools and fluxes of C and N in tree biomass, microbes, and soils from 1997 through 2002 collected at the Duke Forest free-air CO2 enrichment (FACE) experiment. Elevated CO2 stimulated NPP by 18-24% during the first six years of this experiment. Consistent with the hypothesis for PNL, significantly more N was immobilized in tree biomass and in the O horizon under elevated CO2. In contrast to the PNL hypothesis, microbial-N immobilization did not increase under elevated CO2, and although the rate of net N mineralization declined through time, the decline was not significantly more rapid under elevated CO2. Ecosystem C-to-N ratios widened more rapidly under elevated CO2 than ambient CO2 indicating a more rapid rate of C fixation per unit of N, a processes that could delay PNL in this ecosystem. Mass balance calculations demonstrated a large accrual of ecosystem N capital. Is PNL occurring in this ecosystem and will NPP decline to levels under ambient CO2? The answer depends on the relative strength of tree biomass and O-horizon N immobilization vs. widening C-to-N ratios and ecosystem-N accrual as processes that drive and delay PNL, respectively. Only direct observations through time will definitively answer this question.
DOI:10.1038/417279aURLPMID:12015601 [本文引用: 1]

Carbon sequestration in soil organic matter may moderate increases in atmospheric CO(2) concentrations (C(a)) as C(a) increases to more than 500 micromol mol(-1) this century from interglacial levels of less than 200 micromol mol(-1) (refs 1 6). However, such carbon storage depends on feedbacks between plant responses to C(a) and nutrient availability. Here we present evidence that soil carbon storage and nitrogen cycling in a grassland ecosystem are much more responsive to increases in past C(a) than to those forecast for the coming century. Along a continuous gradient of 200 to 550 micromol mol(-1) (refs 9, 10), increased C(a) promoted higher photosynthetic rates and altered plant tissue chemistry. Soil carbon was lost at subambient C(a), but was unchanged at elevated C(a) where losses of old soil carbon offset increases in new carbon. Along the experimental gradient in C(a) there was a nonlinear, threefold decrease in nitrogen availability. The differences in sensitivity of carbon storage to historical and future C(a) and increased nutrient limitation suggest that the passive sequestration of carbon in soils may have been important historically, but the ability of soils to continue as sinks is limited.
DOI:10.1126/science.1185383URLPMID:20110467 [本文引用: 1]

Continuing population and consumption growth will mean that the global demand for food will increase for at least another 40 years. Growing competition for land, water, and energy, in addition to the overexploitation of fisheries, will affect our ability to produce food, as will the urgent requirement to reduce the impact of the food system on the environment. The effects of climate change are a further threat. But the world can produce more food and can ensure that it is used more efficiently and equitably. A multifaceted and linked global strategy is needed to ensure sustainable and equitable food security, different components of which are explored here.
DOI:10.18520/cs/v114/i08/1767-1777URL [本文引用: 1]
DOI:10.1071/FP12357URL [本文引用: 1]

There is some evidence that rice cultivars respond differently to elevated CO2 concentrations ([CO2]), but [CO2] x cultivar interaction has never been tested under open-field conditions across different sites. Here, we report on trials conducted at free-air CO2 enrichment (FACE) facilities at two sites in Japan, Shizukuishi (2007 and 2008) and Tsukuba (2010). The average growing-season air temperature was more than 5 degrees C warmer at Tsukuba than at Shizukuishi. For four cultivars tested at both sites, the [CO2] x cultivar interaction was significant for brown rice yield, but there was no significant interaction with site-year. Higher-yielding cultivars with a large sink size showed a greater [CO2] response. The Tsukuba FACE experiment, which included eight cultivars, revealed a wider range of yield enhancement (3-36%) than the multi-site experiment. All of the tested yield components contributed to this enhancement, but there was a highly significant [CO2] x cultivar interaction for percentage of ripened spikelets. These results suggest that a large sink is a prerequisite for higher productivity under elevated [CO2], but that improving carbon allocation by increasing grain setting may also be a practical way of increasing the yield response to elevated [CO2].
DOI:10.2134/agronj2010.0303URL [本文引用: 1]

Changes in temperature, CO2, and precipitation under the scenarios of climate change for the next 30 yr present a challenge to crop production. This review focuses on the impact of temperature, CO2, and ozone on agronomic crops and the implications for crop production. Understanding these implications for agricultural crops is critical for developing cropping systems resilient to stresses induced by climate change. There is variation among crops in their response to CO2, temperature, and precipitation changes and, with the regional differences in predicted climate, a situation is created in which the responses will be further complicated. For example, the temperature effects on soybean [Glycine max (L.) Merr.] could potentially cause yield reductions of 2.4% in the South but an increase of 1.7% in the Midwest. The frequency of years when temperatures exceed thresholds for damage during critical growth stages is likely to increase for some crops and regions. The increase in CO2 contributes significantly to enhanced plant growth and improved water use efficiency (WUE); however, there may be a downscaling of these positive impacts due to higher temperatures plants will experience during their growth cycle. A challenge is to understand the interactions of the changing climatic parameters because of the interactions among temperature, CO2, and precipitation on plant growth and development and also on the biotic stresses of weeds, insects, and diseases. Agronomists will have to consider the variations in temperature and precipitation as part of the production system if they are to ensure the food security required by an ever increasing population.
DOI:10.5194/bg-3-479-2006URL [本文引用: 1]
DOI:10.1111/ppl.12676URLPMID:29205382 [本文引用: 1]

The impact of elevated [CO2 ] (e[CO2 ]) on crops often includes a decrease in their nutrient concentrations where reduced transpiration-driven mass flow of nutrients has been suggested to play a role. We used two independent approaches, a free-air CO2 enrichment (FACE) experiment in the South Eastern wheat belt of Australia and a simulation study employing the agricultural production systems simulator (APSIM), to show that transpiration (mm) and nutrient uptake (g m(-2) ) of nitrogen (N), potassium (K), sulfur (S), calcium (Ca), magnesium (Mg) and manganese (Mn) in wheat are correlated under e[CO2 ], but that nutrient uptake per unit water transpired is higher under e[CO2 ] than under ambient [CO2 ] (a[CO2 ]). This result suggests that transpiration-driven mass flow of nutrients contributes to decreases in nutrient concentrations under e[CO2 ], but cannot solely explain the overall decline.
URLPMID:29674577 [本文引用: 1]
DOI:10.1007/s11104-006-9093-4URL [本文引用: 1]

A major uncertainty in predicting long-term ecosystem C balance is whether stimulation of net primary production will be sustained in future atmospheric CO2 scenarios. Immobilization of nutrients (N in particular) in plant biomass and soil organic matter (SOM) provides negative feedbacks to plant growth and may lead to progressive N limitation (PNL) of plant response to CO2 enrichment. Soil microbes mediate N availability to plants by controlling litter decomposition and N transformations as well as dominating biological N fixation. CO2-induced changes in C inputs, plant nutrient demand and water use efficiency often have interactive and contrasting effects on microbes and microbially mediated N processes. One critical question is whether CO2-induced N accumulation in plant biomass and SOM will result in N limitation of microbes and subsequently cause them to obtain N from alternative sources or to alter the ecosystem N balance. We reviewed the experimental results that examined elevated CO2 effects on microbial parameters, focusing on those published since 2000. These results in general show that increased C inputs dominate the CO2 impact on microbes, microbial activities and their subsequent controls over ecosystem N dynamics, potentially enhancing microbial N acquisition and ecosystem N retention. We reason that microbial mediation of N availability for plants under future CO2 scenarios will strongly depend on the initial ecosystem N status, and the nature and magnitude of external N inputs. Consequently, microbial processes that exert critical controls over long-term N availability for plants would be ecosystem-specific. The challenge remains to quantify CO2-induced changes in these processes, and to extrapolate the results from short-term studies with step-up CO2 increases to native ecosystems that are already experiencing gradual changes in the CO2 concentration.]]>
[本文引用: 3]
DOI:10.1038/NGEO1741URL [本文引用: 1]

How tropical forest carbon stocks might alter in response to changes in climate and atmospheric composition is uncertain. However, assessing potential future carbon loss from tropical forests is important for evaluating the efficacy of programmes for reducing emissions from deforestation and degradation. Uncertainties are associated with different carbon stock responses in models with different representations of vegetation processes on the one hand(1-3), and differences in projected changes in temperature and precipitation patterns on the other hand(4,5). Here we present a systematic exploration of these sources of uncertainty, along with uncertainty arising from different emissions scenarios for all three main tropical forest regions: the Americas (that is, Amazonia and Central America), Africa and Asia. Using simulations with 22 climate models and the MOSES-TRIFFID land surface scheme, we find that only in one(5) of the simulations are tropical forests projected to lose biomass by the end of the twenty-first century-and then only for the Americas. When comparing with alternative models of plant physiological processes(1,2), we find that the largest uncertainties are associated with plant physiological responses, and then with future emissions scenarios. Uncertainties from differences in the climate projections are significantly smaller. Despite the considerable uncertainties, we conclude that there is evidence of forest resilience for all three regions.
DOI:10.1111/gcb.13981URLPMID:29136323 [本文引用: 1]

Achieving higher canopy photosynthesis rates is one of the keys to increasing future crop production; however, this typically requires additional water inputs because of increased water loss through the stomata. Lowland rice canopies presently consume a large amount of water, and any further increase in water usage may significantly impact local water resources. This situation is further complicated by changing the environmental conditions such as rising atmospheric CO2 concentration ([CO2 ]). Here, we modeled and compared evapotranspiration of fully developed rice canopies of a high-yielding rice cultivar (Oryza sativa L. cv. Takanari) with a common cultivar (cv. Koshihikari) under ambient and elevated [CO2 ] (A-CO2 and E-CO2 , respectively) via leaf ecophysiological parameters derived from a free-air CO2 enrichment (FACE) experiment. Takanari had 4%-5% higher evapotranspiration than Koshihikari under both A-CO2 and E-CO2 , and E-CO2 decreased evapotranspiration of both varieties by 4%-6%. Therefore, if Takanari was cultivated under future [CO2 ] conditions, the cost for water could be maintained at the same level as for cultivating Koshihikari at current [CO2 ] with an increase in canopy photosynthesis by 36%. Sensitivity analyses determined that stomatal conductance was a significant physiological factor responsible for the greater canopy photosynthesis in Takanari over Koshihikari. Takanari had 30%-40% higher stomatal conductance than Koshihikari; however, the presence of high aerodynamic resistance in the natural field and lower canopy temperature of Takanari than Koshihikari resulted in the small difference in evapotranspiration. Despite the small difference in evapotranspiration between varieties, the model simulations showed that Takanari clearly decreased canopy and air temperatures within the planetary boundary layer compared to Koshihikari. Our results indicate that lowland rice varieties characterized by high-stomatal conductance can play a key role in enhancing productivity and moderating heat-induced damage to grain quality in the coming decades, without significantly increasing crop water use.
[本文引用: 1]
DOI:10.1002/jsfa.7545URLPMID:26608560 [本文引用: 1]

BACKGROUND: Rising atmospheric CO2 is accompanied by global warming. However, interactive effects of elevated CO2 and temperature have not been well studied on grain quality of rice. A japonica cultivar was grown in the field using a free-air CO2 enrichment facility in combination with a canopy air temperature increase system in 2014. The gas fumigation (200 micromol mol(-1) above ambient CO2 ) and temperature increase (1 degrees C above ambient air temperature) were performed from tillering until maturity. RESULTS: Compared with the control (ambient CO2 and air temperature), elevated CO2 increased grain length and width as well as grain chalkiness but decreased protein concentrations. In contrast, the increase in canopy air temperature had less effect on these parameters except for grain chalkiness. The starch pasting properties of rice flour and taste analysis of cooked rice indicated that the palatability of rice was improved by CO2 and/or temperature elevation, with the combination of the two treatments showing the most significant changes compared with ambient rice. CONCLUSION: It is concluded that projected CO2 in 2050 may have larger effects on rice grain quality than the projected temperature increase. Although deterioration in milling suitability, grain appearance and nutritional quality can be expected, the taste of cooked rice might be better in the future environment. (c) 2015 Society of Chemical Industry.
DOI:10.1016/j.envint.2014.07.021URL [本文引用: 2]

We appraise the present geographical extent and inherent knowledge limits, following two decades of research on elevated CO2 responses in plant communities, and ask whether such research has answered the key question in quantifying the limits of compensatory CO2 uptake in the major biomes. Our synthesis of all ecosystem-scale (between 10 m(2) and 3000 m(2) total experimental plot area) elevated CO2 (eCO(2)) experiments in natural ecosystems conducted worldwide since 1987 (n = 151) demonstrates that the locations of these eCO(2) experiments have been spatially biased, targeting primarily the temperate ecosystems of northern America and Europe. We consider the consequences, suggesting fundamentally that this limits the capacity of the research to understand how the world's major plant communities will respond to eCO(2). Most notably, our synthesis shows that this research lacks understanding of impacts on tropical forests and boreal regions, which are potentially the most significant biomes for C sink and storage activity, respectively. Using a meta-analysis of the available data across all biomes, we show equivocal increases in net primary productivity (NPP) from eCO(2) studies, suggesting that global validation is needed, especially in the most important biomes for C processing. Further, our meta-analysis identifies that few research programs have addressed eCO(2) effects on below-ground C storage, such that at the global scale, no overall responses are discernable. Given the disparity highlighted in the distribution of eCO(2) experiments globally, we suggest opportunities for newly-industrialized or developing nations to become involved in further research, particularly as these countries host some of the most important regions for tropical or subtropical forest systems. Modeling approaches that thus far have attempted to understand the biological response to eCO(2) are constrained with-respect to collective predictions, suggesting that further work is needed, which will link models to in situ eCO(2) experiments, in order to understand how the world's most important regions for terrestrial C uptake and storage will respond to a future eCO(2) atmosphere. Crown Copyright (C) 2014 Published by Elsevier Ltd.
DOI:10.1126/science.280.5362.441URLPMID:9545223 [本文引用: 1]

In model terrestrial ecosystems maintained for three plant generations at elevated concentrations of atmospheric carbon dioxide, increases in photosynthetically fixed carbon were allocated below ground, raising concentrations of dissolved organic carbon in soil. These effects were then transmitted up the decomposer food chain. Soil microbial biomass was unaffected, but the composition of soil fungal species changed, with increases in rates of cellulose decomposition. There were also changes in the abundance and species composition of Collembola, fungal-feeding arthropods. These results have implications for long-term feedback processes in soil ecosystems that are subject to rising global atmospheric carbon dioxide concentrations.
[本文引用: 1]
DOI:10.1023/A:1014244413067URL [本文引用: 1]

Three species, wheat, maize and cotton, were grown in pots and subjected to high (85–100% field capacity, F), medium (65–85% F) and low (45–65% F) soil moisture treatments and high (700 l l–1) and low (350 l l–1) CO2 concentrations. Biomass production, photosynthesis, evapotranspiration and crop water use efficiency were investigated. Results showed that the daily photosynthesis rate was increased more in wheat and cotton at high [CO2] than in maize. In addition, differences were more substantial at low soil water treatment than at high soil water treatment. The daily leaf transpiration was reduced significantly in the three crops at the high CO2 concentration. The decrease at low soil water was smaller than at high soil water. Crop biomass production responses showed a pattern similar to photosynthesis, but the CO2-induced increase was more pronounced in root production than shoot production under all soil water treatments. Low soil water treatment led to more root biomass under high [CO2] than high soil water treatment. CO2 enrichment caused a higher leaf water use efficiency (WUE) of three crops and the increase was more significant in low than in high soil water treatment. Crop community WUE was also increased by CO2 enrichment, but the increase in wheat and cotton was much greater than in maize. We conclude that at least in the short-term, C3 plants such as wheat and cotton may benefit from CO2 enrichment especially under water shortage condition.]]>
DOI:10.1046/j.1365-2435.2003.00733.xURL [本文引用: 1]
DOI:10.1038/nature12291URLPMID:23842499 [本文引用: 1]

Terrestrial plants remove CO2 from the atmosphere through photosynthesis, a process that is accompanied by the loss of water vapour from leaves. The ratio of water loss to carbon gain, or water-use efficiency, is a key characteristic of ecosystem function that is central to the global cycles of water, energy and carbon. Here we analyse direct, long-term measurements of whole-ecosystem carbon and water exchange. We find a substantial increase in water-use efficiency in temperate and boreal forests of the Northern Hemisphere over the past two decades. We systematically assess various competing hypotheses to explain this trend, and find that the observed increase is most consistent with a strong CO2 fertilization effect. The results suggest a partial closure of stomata-small pores on the leaf surface that regulate gas exchange-to maintain a near-constant concentration of CO2 inside the leaf even under continually increasing atmospheric CO2 levels. The observed increase in forest water-use efficiency is larger than that predicted by existing theory and 13 terrestrial biosphere models. The increase is associated with trends of increasing ecosystem-level photosynthesis and net carbon uptake, and decreasing evapotranspiration. Our findings suggest a shift in the carbon- and water-based economics of terrestrial vegetation, which may require a reassessment of the role of stomatal control in regulating interactions between forests and climate change, and a re-evaluation of coupled vegetation-climate models.
[本文引用: 1]
[本文引用: 1]
DOI:10.1126/science.1113977URLPMID:16123297 [本文引用: 1]

Whether rising atmospheric carbon dioxide (CO2) concentrations will cause forests to grow faster and store more carbon is an open question. Using free air CO2 release in combination with a canopy crane, we found an immediate and sustained enhancement of carbon flux through 35-meter-tall temperate forest trees when exposed to elevated CO2. However, there was no overall stimulation in stem growth and leaf litter production after 4 years. Photosynthetic capacity was not reduced, leaf chemistry changes were minor, and tree species differed in their responses. Although growing vigorously, these trees did not accrete more biomass carbon in stems in response to elevated CO2, thus challenging projections of growth responses derived from tests with smaller trees.
DOI:10.1016/j.soilbio.2008.09.016URL [本文引用: 1]
DOI:10.1111/gcb.2008.14.issue-12URL [本文引用: 1]
DOI:10.7554/eLife.02245URLPMID:24867639 [本文引用: 1]

carbon:minerals in C3 plants. The meta-analysis of 7761 observations, including 2264 observations at state of the art FACE centers, covers 130 species/cultivars. The attained statistical power reveals that the shift is systemic and global. Its potential to exacerbate the prevalence of 'hidden hunger' and obesity is discussed.DOI: http://dx.doi.org/10.7554/eLife.02245.001.]]>
URLPMID:16809532 [本文引用: 2]
DOI:10.1146/annurev.arplant.55.031903.141610URLPMID:15377233 [本文引用: 1]

Atmospheric CO(2) concentration ([CO(2)]) is now higher than it was at any time in the past 26 million years and is expected to nearly double during this century. Terrestrial plants with the C(3) photosynthetic pathway respond in the short term to increased [CO(2)] via increased net photosynthesis and decreased transpiration. In the longer term this increase is often offset by downregulation of photosynthetic capacity. But much of what is currently known about plant responses to elevated [CO(2)] comes from enclosure studies, where the responses of plants may be modified by size constraints and the limited life-cycle stages that are examined. Free-Air CO(2) Enrichment (FACE) was developed as a means to grow plants in the field at controlled elevation of CO(2) under fully open-air field conditions. The findings of FACE experiments are quantitatively summarized via meta-analytic statistics and compared to findings from chamber studies. Although trends agree with parallel summaries of enclosure studies, important quantitative differences emerge that have important implications both for predicting the future terrestrial biosphere and understanding how crops may need to be adapted to the changed and changing atmosphere.
[本文引用: 5]
DOI:10.1890/04-1724URLPMID:16634296 [本文引用: 2]

The capability of terrestrial ecosystems to sequester carbon (C) plays a critical role in regulating future climatic change yet depends on nitrogen (N) availability. To predict long-term ecosystem C storage, it is essential to examine whether soil N becomes progressively limiting as C and N are sequestered in long-lived plant biomass and soil organic matter. A critical parameter to indicate the long-term progressive N limitation (PNL) is net change in ecosystem N content in association with C accumulation in plant and soil pools under elevated CO2. We compiled data from 104 published papers that study C and N dynamics at ambient and elevated CO2. The compiled database contains C contents, N contents, and C:N ratio in various plant and soil pools, and root:shoot ratio. Averaged C and N pool sizes in plant and soil all significantly increase at elevated CO2 in comparison to those at ambient CO2, ranging from a 5% increase in shoot N content to a 32% increase in root C content. The C and N contents in litter pools are consistently higher in elevated than ambient CO2 among all the surveyed studies whereas C and N contents in the other pools increase in some studies and decrease in other studies. The high variability in CO2-induced changes in C and N pool sizes results from diverse responses of various C and N processes to elevated CO2. Averaged C:N ratios are higher by 3% in litter and soil pools and 11% in root and shoot pools at elevated relative to ambient CO2. Elevated CO2 slightly increases root:shoot ratio. The net N accumulation in plant and soil pools at least helps prevent complete down-regulation of, and likely supports, long-term CO2 stimulation of C sequestration. The concomitant C and N accumulations in response to rising atmospheric CO2 may reflect intrinsic nature of ecosystem development as revealed before by studies of succession over hundreds to millions of years.
[本文引用: 1]
DOI:10.1038/nature13179URL [本文引用: 2]

Dietary deficiencies of zinc and iron are a substantial global public health problem. An estimated two billion people suffer these deficiencies(1), causing a loss of 63 million life-years annually(2,3). Most of these people depend on C-3 grains and legumes as their primary dietary source of zinc and iron. Here we report that C-3 grains and legumes have lower concentrations of zinc and iron when grown under field conditions at the elevated atmospheric CO2 concentration predicted for the middle of this century. C-3 crops other than legumes also have lower concentrations of protein, whereas C-4 crops seem to be less affected. Differences between cultivars of a single crop suggest that breeding for decreased sensitivity to atmospheric CO2 concentration could partly address these new challenges to global health.
DOI:10.1038/s41598-017-01690-8URLPMID:28500344

The global atmospheric CO2 concentration has been increasing annually. To determine the trait that effectively increases rice (Oryza sativa L.) grain yield under increased atmospheric CO2 concentrations, as predicted in the near future, we grew a chromosome segment substitution line (CSSL) and a near-isogenic line (NIL) producing high spikelet numbers per panicle (CSSL-GN1 and NIL-APO1, respectively) under free-air CO2 enrichment (FACE) conditions and examined the effects of a large sink capacity on grain yield, its components, and growth-related traits under increased atmospheric CO2 concentrations. Under ambient conditions, CSSL-GN1 and NIL-APO1 exhibited a similar grain yield to Koshihikari, as a result of the trade-off between increased spikelet number and reduced grain filling. However, under FACE conditions, CSSL-GN1 and NIL-APO1 had an equal or a higher grain yield than Koshihikari because of the higher number of spikelets and lower reduction in grain filling. Thus, the improvement of source activity by increased atmospheric CO2 concentrations can lead to enhanced grain yield in rice lines that have a large sink capacity. Therefore, introducing alleles that increase sink capacity into conventional varieties represents a strategy that can be used to develop high-yielding varieties under increased atmospheric CO2 concentrations, such as those predicted in the near future.
DOI:10.1007/s004420000615URLPMID:24577644 [本文引用: 1]

The results of published and unpublished experiments investigating the impacts of elevated [CO2] on the chemistry of leaf litter and decomposition of plant tissues are summarized. The data do not support the hypothesis that changes in leaf litter chemistry often associated with growing plants under elevated [CO2] have an impact on decomposition processes. A meta-analysis of data from naturally senesced leaves in field experiments showed that the nitrogen (N) concentration in leaf litter was 7.1% lower in elevated [CO2] compared to that in ambient [CO2]. This statistically significant difference was: (1) usually not significant in individual experiments, (2) much less than that often observed in green leaves, and (3) less in leaves with an N concentration indicative of complete N resorption. Under ideal conditions, the efficiency with which N is resorbed during leaf senescence was found not to be altered by CO2 enrichment, but other environmental influences on resorption inevitably increase the variability in litter N concentration. Nevertheless, the small but consistent decline in leaf litter N concentration in many experiments, coupled with a 6.5% increase in lignin concentration, would be predicted to result in a slower decomposition rate in CO2-enriched litter. However, across the assembled data base, neither mass loss nor respiration rates from litter produced in elevated [CO2] showed any consistent pattern or differences from litter grown in ambient [CO2]. The effects of [CO2] on litter chemistry or decomposition were usually smallest under experimental conditions similar to natural field conditions, including open-field exposure, plants free-rooted in the ground, and complete senescence. It is concluded that any changes in decomposition rates resulting from exposure of plants to elevated [CO2] are small when compared to other potential impacts of elevated [CO2] on carbon and N cycling. Reasons for experimental differences are considered, and recommendations for the design and execution of decomposition experiments using materials from CO2-enrichment experiments are outlined.
[本文引用: 1]
[本文引用: 1]
DOI:10.1073/pnas.1006463107URLPMID:20974944 [本文引用: 1]

Stimulation of terrestrial plant production by rising CO(2) concentration is projected to reduce the airborne fraction of anthropogenic CO(2) emissions. Coupled climate-carbon cycle models are sensitive to this negative feedback on atmospheric CO(2), but model projections are uncertain because of the expectation that feedbacks through the nitrogen (N) cycle will reduce this so-called CO(2) fertilization effect. We assessed whether N limitation caused a reduced stimulation of net primary productivity (NPP) by elevated atmospheric CO(2) concentration over 11 y in a free-air CO(2) enrichment (FACE) experiment in a deciduous Liquidambar styraciflua (sweetgum) forest stand in Tennessee. During the first 6 y of the experiment, NPP was significantly enhanced in forest plots exposed to 550 ppm CO(2) compared with NPP in plots in current ambient CO(2), and this was a consistent and sustained response. However, the enhancement of NPP under elevated CO(2) declined from 24% in 2001-2003 to 9% in 2008. Global analyses that assume a sustained CO(2) fertilization effect are no longer supported by this FACE experiment. N budget analysis supports the premise that N availability was limiting to tree growth and declining over time--an expected consequence of stand development, which was exacerbated by elevated CO(2). Leaf- and stand-level observations provide mechanistic evidence that declining N availability constrained the tree response to elevated CO(2); these observations are consistent with stand-level model projections. This FACE experiment provides strong rationale and process understanding for incorporating N limitation and N feedback effects in ecosystem and global models used in climate change assessments.
[本文引用: 2]
[本文引用: 1]
[本文引用: 1]
[本文引用: 1]
DOI:10.1111/j.1461-0248.2010.01570.xURLPMID:21176050 [本文引用: 1]

The degree to which rising atmospheric CO(2) will be offset by carbon (C) sequestration in forests depends in part on the capacity of trees and soil microbes to make physiological adjustments that can alleviate resource limitation. Here, we show for the first time that mature trees exposed to CO(2) enrichment increase the release of soluble C from roots to soil, and that such increases are coupled to the accelerated turnover of nitrogen (N) pools in the rhizosphere. Over the course of 3 years, we measured in situ rates of root exudation from 420 intact loblolly pine (Pinus taeda L.) roots. Trees fumigated with elevated CO(2) (200 p.p.m.v. over background) increased exudation rates (mug C cm(-1) root h(-1) ) by 55% during the primary growing season, leading to a 50% annual increase in dissolved organic inputs to fumigated forest soils. These increases in root-derived C were positively correlated with microbial release of extracellular enzymes involved in breakdown of organic N (R(2) = 0.66; P = 0.006) in the rhizosphere, indicating that exudation stimulated microbial activity and accelerated the rate of soil organic matter (SOM) turnover. In support of this conclusion, trees exposed to both elevated CO(2) and N fertilization did not increase exudation rates and had reduced enzyme activities in the rhizosphere. Collectively, our results provide field-based empirical support suggesting that sustained growth responses of forests to elevated CO(2) in low fertility soils are maintained by enhanced rates of microbial activity and N cycling fuelled by inputs of root-derived C. To the extent that increases in exudation also stimulate SOM decomposition, such changes may prevent soil C accumulation in forest ecosystems.
[本文引用: 1]
[本文引用: 1]
[本文引用: 5]
[本文引用: 1]
DOI:10.1016/j.scitotenv.2008.04.050URLPMID:18675444 [本文引用: 1]

Accumulating evidence points to an anthropogenic 'fingerprint' on the global climate change that has occurred in the last century. Climate change has, and will continue to have, profound effects on the structure and function of terrestrial ecosystems. As such, there is a critical need to continue to develop a sound scientific basis for national and international policies regulating carbon sequestration and greenhouse gas emissions. This paper reflects on the nature of current global change experiments, and provides recommendations for a unified multidisciplinary approach to future research in this dynamic field. These recommendations include: (1) better integration between experiments and models, and amongst experimental, monitoring, and space-for-time studies; (2) stable and increased support for long-term studies and multi-factor experiments; (3) explicit inclusion of biodiversity, disturbance, and extreme events in experiments and models; (4) consideration of timing vs intensity of global change factors in experiments and models; (5) evaluation of potential thresholds or ecosystem 'tipping points'; and (6) increased support for model-model and model-experiment comparisons. These recommendations, which reflect discussions within the TERACC international network of global change scientists, will facilitate the unraveling of the complex direct and indirect effects of global climate change on terrestrial ecosystems and their components.
[本文引用: 1]
[本文引用: 1]
[本文引用: 1]
[本文引用: 1]
URLPMID:19017124 [本文引用: 2]
[本文引用: 1]
[本文引用: 1]
[本文引用: 2]
[本文引用: 4]
[本文引用: 1]
[本文引用: 2]
DOI:10.1016/j.pbi.2018.06.001URLPMID:29958824 [本文引用: 1]

Crops grown under elevated CO2 (eCO2) typically exhibit enhanced yields but at the same time decreased nutritional quality. The latter effect has often been explained as a growth dilution phenomenon, but this cannot be the only process involved since crop nutrient concentrations are decreased also when production is unaffected by eCO2. We review the current knowledge on eCO2 effects on crop nutritional quality with focus on the current understanding of the possible mechanisms and processes causing these effects. Emphasis is on crop nitrogen (N) and protein concentrations but effects on other nutrients and how they compare with those on N are also covered.
[本文引用: 1]
URLPMID:26463894 [本文引用: 2]
[本文引用: 1]
[本文引用: 1]
[本文引用: 1]
[本文引用: 1]
URLPMID:25737035 [本文引用: 1]
DOI:10.1016/j.eja.2012.05.011URL [本文引用: 1]
URLPMID:32291045 [本文引用: 1]
DOI:10.1007/s10705-012-9523-zURL [本文引用: 1]

To investigate the response of methane (CH4) emissions to an elevated atmospheric carbon dioxide (CO2) concentration (200 +/- A 40 mu mol mol(-1) higher than the ambient atmosphere), we performed a 4-year multi-factorial experiment at a subtropical rice paddy that contained sandy loam soil in the Yangtze River Delta from 2004 to 2007 using free-air CO2 enrichment (FACE) technology. Our results revealed that the elevated atmospheric CO2 increased the seasonal cumulative CH4 emissions by 15 % on average during the 4-year period. The increase was insignificant and much weaker than the previous studies, which might be primarily attributed to the absence of a significant difference in the rice biomass between the two CO2 levels in half of the field treatments. Crop residue incorporation hindered the stimulatory effects induced by the elevated CO2, which were 37, 14 and 6 % for the fields that were incorporated with none, half or all of the wheat straws that were harvested in the preceding winter wheat season, respectively. Nitrogen fertilizers application also hindered the stimulatory effects of the elevated CO2 on the CH4 emissions. The CO2 stimulatory effect was 39 % for the field without nitrogen fertilizers, and reduced to 17, 7 and 5 % for the field with nitrogen fertilization of 125, 250 and 350 kg N ha(-1), respectively. The regulation of nitrogen fertilizers on the CO2 effects in this experiment does not well agree with the previous studies, which might because the soil type was different from those of the previous studies. Thus, further studies are necessary to evaluate the role of soil properties in regulating the effects of elevated atmospheric CO2 on CH4 emissions from managed and natural wetlands. There were no significant interactions between the atmospheric CO2 and the incorporations of nitrogen fertilizer and crop residue. Appropriate experiments are necessary for better understanding of the interact influences of the elevated CO2 and farm managements.
[本文引用: 1]
[本文引用: 1]
[本文引用: 3]
[本文引用: 1]
[本文引用: 3]
[本文引用: 1]
[本文引用: 1]
URLPMID:30077851 [本文引用: 1]
DOI:10.1007/BF00317605URLPMID:28312925 [本文引用: 1]

Seedlings of nine tropical species varying in growth and carbon metabolism were exposed to twice the current atmospheric level of CO2 for a 3 month period on Barro Colorado Island, Panama. A doubling of the CO2 concentration resulted in increases in photosynthesis and greater water use efficiency (WUE) for all species possessing C3 metabolism, when compared to the ambient condition. No desensitization of photosynthesis to increased CO2 was observed during the 3 month period. Significant increases in total plant dry weight were also noted for 4 out of the 5 C3 species tested and in one CAM species, Aechmea magdalenae at high CO2. In contrast, no significant increases in either photosynthesis or total plant dry weight were noted for the C4 grass, Paspallum conjugatum. Increases in the apparent quantum efficiency (AQE) for all C3 species suggest that elevated CO2 may increase photosynthetic rate relative to ambient CO2 over a wide range of light conditions. The response of CO2 assimilation to internal Ci suggested a reduction in either the RuBP and/or Pi regeneration limitation with long term exposure to elevated CO2. This experiment suggests that: (1) a global rise in CO2 may have significant effects on photosynthesis and productivity in a wide variety of tropical species, and (2) increases in productivity and photosynthesis may be related to physiological adaptation(s) to increased CO2.
[本文引用: 1]
What have we learned from 15 years of free-air CO2 enrichment (FACE)? A meta-analytic review of the responses of photosynthesis, canopy properties and plant production to rising CO2
1
2005
... FACE系统是目前研究CO2浓度升高对地上-地下生态系统影响及其机理最为理想的方法.FACE系统相比其他模拟手段不会限制植物的根系, 也不会对风、光照和辐射等产生影响, 因此能够满足长期持续地研究CO2浓度升高对整个生态系统的影响(
Taking mycocentrism seriously: mycorrhizal fungal and plant responses to elevated CO2
1
2005
... CO2浓度升高会增加根际沉积(
Assessment of 10 years of CO2 fumigation on soil microbial communities and function in a sweetgum plantation
1
2009
... CO2浓度升高会增加根际沉积(
CO2 studies remain key to understanding a future world
2
2017
... 工业革命以来, 人类活动导致化石燃料过量使用和土地利用方式改变等, 引发了大气中CO2等温室气体浓度的急剧上升.目前大气中CO2浓度已由1860年的280 μL·L-1升高至目前的410 μL·L-1左右, 并以每年0.45%的速率升高, 预计到2030年将增加到550 μL·L-1 (
... 理解生态系统对过去、现在和未来CO2浓度变化的响应对于在生态进化的时间尺度上认识和预测全球变化的后果至关重要.过去二十多年来在CO2浓度升高对植物光合和生长、生产力和产量影响等方面取得较大进展, 但是对于整个生态系统结构和功能的响应仍然存在一定挑战和较大的不确定性.
Linking microbial activity and soil organic matter transformations in forest soils under elevated CO2
1
2005
... CO2浓度升高会增加根际沉积(
Is there potential to adapt soybean (Glycine max Merr.) to future [CO2]? An analysis of the yield response of 18 genotypes in free-air CO2 enrichment
1
2014
... 水稻(
Nitrate assimilation is inhibited by elevated CO2 in field-grown wheat
1
2014
... CO2浓度升高显著降低植物组织氮含量(
Nitrogen assimilation and growth of wheat under elevated carbon dioxide
1
2002
... CO2浓度升高显著降低植物组织氮含量(
Decomposition of soybean grown under elevated concentrations of CO2 and O3
1
2005
... CO2浓度升高条件下土壤微生物也可能通过改变土壤中的分解过程对大气CO2浓度产生正或负的反馈作用.一方面, CO2浓度升高可引起微生物活性增加(
Yield growth and grain nitrogen response to elevated CO2 in six lentil (Lens culinaris) cultivars grown under Free Air CO2 Enrichment (FACE) in a semi-arid environment
1
2017
... 水稻(
Responses of soybeans and wheat to elevated CO2 in free-air and open top chamber systems
1
2016
... FACE系统是目前研究CO2浓度升高对地上-地下生态系统影响及其机理最为理想的方法.FACE系统相比其他模拟手段不会限制植物的根系, 也不会对风、光照和辐射等产生影响, 因此能够满足长期持续地研究CO2浓度升高对整个生态系统的影响(
Using FACE systems to screen wheat cultivars for yield increases at elevated CO2
1
2017
... 水稻(
Altered soil microbial community at elevated CO2 leads to loss of soil carbon
3
2007
... CO2浓度升高对于土壤“碳汇”功能, 以及对生态系统碳固定(植物和土壤)的综合影响尚不清楚.大气CO2浓度升高既促进地上生物量的增加, 也促进凋落物归还量及地下部分生物量的增加.CO2浓度升高还可增加粗微团聚体形成的稳定性和碳保护功能, 从而提高土壤碳的稳定性(
... CO2浓度升高会增加根际沉积(
... CO2浓度升高条件下土壤微生物也可能通过改变土壤中的分解过程对大气CO2浓度产生正或负的反馈作用.一方面, CO2浓度升高可引起微生物活性增加(
Tropical forest responses to increasing atmospheric CO2: current knowledge and opportunities for future research
2
2013
... 现有的模拟CO2浓度升高的研究对不同生态系统关注度存在较大的差异.实验系统多建立在温带草原和森林生态系统中, 并且多处在北美和欧洲.对广泛分布于发展中国家和地区的热带和亚热带雨林、稀树草原知之甚少.热带和亚热带生态系统包含了陆地约50%的碳储量, 大气氮沉降和土壤氮有效性与温带、寒温带地区有明显差异(
... 供应、道路的便利等因素的限制(
Elevated CO2 and heat stress interactions affect grain yield quality and mineral nutrient composition in rice under field conditions
2
2017
... 随着人们生活水平的提高, 对饮食的需求正在从过去的数量型向现在的营养型转变.21世纪的粮食安全将由以数量为主转向数量与质量并重的方向发展, 不仅要解决全世界人口的温饱问题, 还要对粮食的营养价值给予足够的重视.在全球变化等多因子的复合作用下, CO2浓度升高对作物增产的正效应能被O3浓度升高(
... ), 作物营养和外观等品质的下降也会在增温复合作用下进一步恶化(
Do the rich always become richer? Characterizing the leaf physiological response of the high-yielding rice cultivar Takanari to free-air CO2 enrichment
1
2014
... 水稻(
Arbuscular mycorrhizal fungi increase organic carbon decomposition under elevated CO2
3
2012
... 长期而言, CO2浓度升高对植物生长的刺激作用往往会导致更多的氮被固存在多年生的植物体内和土壤有机质中(
... 在大气CO2浓度升高条件下, 土壤微生物通过调控土壤过程对植物生长可能产生正或负效应.短时间的CO2浓度升高条件下微生物促进有机氮矿化, 增加土壤氮的有效性和植物对无机氮的吸收(
... 过去对于土壤微生物的研究多局限于CO2浓度升高对土壤微生物的影响, 而土壤微生物对植物、对气候变化的反馈作用机制研究相对缺乏.其中的研究重点和热点包括: 一、运用稳定同位素、分子与基因组学等相关技术耦合, 定向研究土壤微生物群落以及相关功能微生物, 有助于揭示微生物调控碳氮循环等耦合过程和机理(
Elevated CO2 reduces the nitrogen concentration of plant tissues
1
1998
... CO2浓度升高显著降低植物组织氮含量(
Sensitivity of tropical carbon to climate change constrained by carbon dioxide variability
1
2013
... 提高水分利用效率(WUE)是大气CO2浓度升高对植物生长最有利的影响之一.在CO2浓度升高下, 植物出现不同程度的气孔密度和气孔导度下降、Rubisco酶活性提高、羧化效率提高、净光合速率升高, 这一系列的有效生理调控途径导致WUE的提高(
Interactive effects between nitrogen fertilization and elevated CO2 on growth and gas exchange of papaya seedlings
1
2016
... 提高水分利用效率(WUE)是大气CO2浓度升高对植物生长最有利的影响之一.在CO2浓度升高下, 植物出现不同程度的气孔密度和气孔导度下降、Rubisco酶活性提高、羧化效率提高、净光合速率升高, 这一系列的有效生理调控途径导致WUE的提高(
Interactions between plant growth and soil nutrient cycling under elevated CO2: a meta-analysis
3
2006
... CO2浓度升高会增加根际沉积(
... 在大气CO2浓度升高条件下, 土壤微生物通过调控土壤过程对植物生长可能产生正或负效应.短时间的CO2浓度升高条件下微生物促进有机氮矿化, 增加土壤氮的有效性和植物对无机氮的吸收(
... CO2浓度升高条件下土壤微生物也可能通过改变土壤中的分解过程对大气CO2浓度产生正或负的反馈作用.一方面, CO2浓度升高可引起微生物活性增加(
Down-regulation of tissue N:P ratios in terrestrial plants by elevated CO2
2
2015
... CO2浓度升高显著降低植物组织氮含量(
... 浓度升高对生物量的促进作用, 植物氮库并未减小反而显著增加(
Simple additive effects are rare: a quantitative review of plant biomass and soil process responses to combined manipulations of CO2 and temperature
1
2012
... 长期而言, CO2浓度升高对植物生长的刺激作用往往会导致更多的氮被固存在多年生的植物体内和土壤有机质中(
Stimulation of microbial extracellular enzyme activities by elevated CO2 depends on soil aggregate size
1
2009
... CO2浓度升高会增加根际沉积(
Increases in the flux of carbon belowground stimulate nitrogen uptake and sustain the long-term enhancement of forest productivity under elevated CO2
2
2011
... 长期而言, CO2浓度升高对植物生长的刺激作用往往会导致更多的氮被固存在多年生的植物体内和土壤有机质中(
... ), 例如外生菌根系统而不是丛枝菌根系统(
Forest productivity in China and its response to global climate change
1
2002
... 大气CO2浓度升高的“施肥效应”刺激植物光合作用和生产力, 总体上促进陆地植被的碳固定.根据国内外实验数据和模拟预测, CO2浓度倍增后, 中国森林生产力的增幅在12%-35% (
Assessing the impacts of current and future concentrations of surface ozone on crop yield with meta-analysis
1
2009
... 随着人们生活水平的提高, 对饮食的需求正在从过去的数量型向现在的营养型转变.21世纪的粮食安全将由以数量为主转向数量与质量并重的方向发展, 不仅要解决全世界人口的温饱问题, 还要对粮食的营养价值给予足够的重视.在全球变化等多因子的复合作用下, CO2浓度升高对作物增产的正效应能被O3浓度升高(
Constraints to nitrogen acquisition of terrestrial plants under elevated CO2
5
2015
... 工业革命以来, 人类活动导致化石燃料过量使用和土地利用方式改变等, 引发了大气中CO2等温室气体浓度的急剧上升.目前大气中CO2浓度已由1860年的280 μL·L-1升高至目前的410 μL·L-1左右, 并以每年0.45%的速率升高, 预计到2030年将增加到550 μL·L-1 (
... CO2浓度升高对植物影响的模拟实验研究可以追溯到20世纪60年代(
... CO2浓度升高下作物的品质会受到影响, 包含营养品质、外观品质、加工品质和食味品质.营养品质方面, CO2浓度升高增加植物体内的碳含量, 并对氮、锌、镁等其他元素产生一定的稀释作用(
... 大气CO2浓度升高的“施肥效应”刺激植物光合作用和生产力, 总体上促进陆地植被的碳固定.根据国内外实验数据和模拟预测, CO2浓度倍增后, 中国森林生产力的增幅在12%-35% (
... CO2浓度升高显著降低植物组织氮含量(
Global patterns in belowground communities
1
2009
... CO2浓度升高会增加根际沉积(
Progressive nitrogen limitation of ecosystem processes under elevated CO2 in a warm-temperate forest
1
2006
... 在大气CO2浓度升高条件下, 土壤微生物通过调控土壤过程对植物生长可能产生正或负效应.短时间的CO2浓度升高条件下微生物促进有机氮矿化, 增加土壤氮的有效性和植物对无机氮的吸收(
Nonlinear grassland responses to past and future atmospheric CO2
1
2002
... 大气CO2浓度升高的“施肥效应”刺激植物光合作用和生产力, 总体上促进陆地植被的碳固定.根据国内外实验数据和模拟预测, CO2浓度倍增后, 中国森林生产力的增幅在12%-35% (
Food security: the challenge of feeding 9 billion people
1
2010
... 随着人们生活水平的提高, 对饮食的需求正在从过去的数量型向现在的营养型转变.21世纪的粮食安全将由以数量为主转向数量与质量并重的方向发展, 不仅要解决全世界人口的温饱问题, 还要对粮食的营养价值给予足够的重视.在全球变化等多因子的复合作用下, CO2浓度升高对作物增产的正效应能被O3浓度升高(
Impact of elevated CO2 on Oryza sativa phenology and brown planthopper, Nilaparvata lugens (Hemiptera: Delphacidae) population
1
2018
... 增施氮肥可以在一定程度上提高水稻的施肥效应, 进一步提高CO2浓度升高下的产量(
Rice cultivar responses to elevated CO2 at two free-air CO2 enrichment (FACE) sites in Japan
1
2013
... 水稻(
Climate impacts on agriculture: implications for crop production
1
2011
... CO2与其他环境因子复合作用时, WUE的响应会发生显著变化.如干旱促进WUE上升(
Effects of Free Atmospheric CO2 Enrichment (FACE), N fertilization and poplar genotype on the physical protection of carbon in the mineral soil of a polar plantation after five years
1
2006
... CO2浓度升高对于土壤“碳汇”功能, 以及对生态系统碳固定(植物和土壤)的综合影响尚不清楚.大气CO2浓度升高既促进地上生物量的增加, 也促进凋落物归还量及地下部分生物量的增加.CO2浓度升高还可增加粗微团聚体形成的稳定性和碳保护功能, 从而提高土壤碳的稳定性(
The relationship between transpiration and nutrient uptake in wheat changes under elevated atmospheric CO2
1
2018
... CO2浓度升高下作物的品质会受到影响, 包含营养品质、外观品质、加工品质和食味品质.营养品质方面, CO2浓度升高增加植物体内的碳含量, 并对氮、锌、镁等其他元素产生一定的稀释作用(
Plant responses to CO2 are a question of time
1
2018
... 水稻(
Progressive N limitation of plant response to elevated CO2: a microbiological perspective
1
2006
... CO2浓度升高会增加根际沉积(
The fate of carbon in grasslands under carbon dioxide enrichment
3
1997
... CO2浓度升高会增加根际沉积(
... 浓度升高可能出现增加、降低或者不变的响应(
... 在大气CO2浓度升高条件下, 土壤微生物通过调控土壤过程对植物生长可能产生正或负效应.短时间的CO2浓度升高条件下微生物促进有机氮矿化, 增加土壤氮的有效性和植物对无机氮的吸收(
Simulated resilience of tropical rainforests to CO2-induced climate change
1
2013
... 提高水分利用效率(WUE)是大气CO2浓度升高对植物生长最有利的影响之一.在CO2浓度升高下, 植物出现不同程度的气孔密度和气孔导度下降、Rubisco酶活性提高、羧化效率提高、净光合速率升高, 这一系列的有效生理调控途径导致WUE的提高(
Increasing canopy photosynthesis in rice can be achieved without a large increase in water use—A model based on free-air CO2 enrichment
1
2018
... 水稻(
1
2013
... 工业革命以来, 人类活动导致化石燃料过量使用和土地利用方式改变等, 引发了大气中CO2等温室气体浓度的急剧上升.目前大气中CO2浓度已由1860年的280 μL·L-1升高至目前的410 μL·L-1左右, 并以每年0.45%的速率升高, 预计到2030年将增加到550 μL·L-1 (
The impact of elevated CO2 and temperature on grain quality of rice grown under open-air field conditions
1
2016
... 除营养品质外, 外观品质也直接影响稻米等作物的加工品质和市场价格.水稻FACE研究发现CO2浓度升高条件下大米的精米率和头等米率显著降低2.0%和23.5%, 精米的垩白籽粒率和垩白度显著增加(
Completing the FACE of elevated CO2 research
2
2014
... FACE系统是目前研究CO2浓度升高对地上-地下生态系统影响及其机理最为理想的方法.FACE系统相比其他模拟手段不会限制植物的根系, 也不会对风、光照和辐射等产生影响, 因此能够满足长期持续地研究CO2浓度升高对整个生态系统的影响(
... 现有的模拟CO2浓度升高的研究对不同生态系统关注度存在较大的差异.实验系统多建立在温带草原和森林生态系统中, 并且多处在北美和欧洲.对广泛分布于发展中国家和地区的热带和亚热带雨林、稀树草原知之甚少.热带和亚热带生态系统包含了陆地约50%的碳储量, 大气氮沉降和土壤氮有效性与温带、寒温带地区有明显差异(
Impacts of rising atmospheric carbon dioxide on model terrestrial ecosystems
1
1998
... CO2浓度升高会增加根际沉积(
Gas-exchange, water use efficiency and yield responses of elite potato (Solanum tuberosum L.) cultivars to changes in atmospheric carbon dioxide concentration, temperature and relative humidity
1
2014
... 提高水分利用效率(WUE)是大气CO2浓度升高对植物生长最有利的影响之一.在CO2浓度升高下, 植物出现不同程度的气孔密度和气孔导度下降、Rubisco酶活性提高、羧化效率提高、净光合速率升高, 这一系列的有效生理调控途径导致WUE的提高(
Benefits of CO2 enrichment on crop plants are modified by soil water status
1
2002
... CO2与其他环境因子复合作用时, WUE的响应会发生显著变化.如干旱促进WUE上升(
Tropospheric O3 moderates responses of temperate hardwood forests to elevated CO2: a synthesis of molecular to ecosystem results from the Aspen FACE project
1
2003
... 全球变化因子间通常不是独立的, 而是相互作用的.目前对CO2浓度升高单因子变化研究取得较大进展, 而多因子交互作用的研究较少, 研究结果存在很大的不确定性.全球变化包括了CO2/O3浓度升高、干旱胁迫、氮沉降和降水格局分布等多因子复合影响, 生态系统在复合因子影响下往往呈现复杂的作用特征.比如O3浓度升高和增温对于植物的负效应往往能抵消一部分CO2浓度升高的“施肥”正效应(
Increase in forest water- use efficiency as atmospheric carbon dioxide concentrations rise
1
2013
... 提高水分利用效率(WUE)是大气CO2浓度升高对植物生长最有利的影响之一.在CO2浓度升高下, 植物出现不同程度的气孔密度和气孔导度下降、Rubisco酶活性提高、羧化效率提高、净光合速率升高, 这一系列的有效生理调控途径导致WUE的提高(
Wheat grain quality as affected by elevated CO2, drought, and soil nitrogen
1
2001
... CO2浓度升高对植物影响的模拟实验研究可以追溯到20世纪60年代(
A multiyear synthesis of soil respiration responses to elevated atmospheric CO2 from four forest FACE experiments
1
2004
... CO2浓度升高对于土壤“碳汇”功能, 以及对生态系统碳固定(植物和土壤)的综合影响尚不清楚.大气CO2浓度升高既促进地上生物量的增加, 也促进凋落物归还量及地下部分生物量的增加.CO2浓度升高还可增加粗微团聚体形成的稳定性和碳保护功能, 从而提高土壤碳的稳定性(
Carbon flux and growth in mature deciduous forest trees exposed to elevated CO2
1
2005
... 大气CO2浓度升高的“施肥效应”刺激植物光合作用和生产力, 总体上促进陆地植被的碳固定.根据国内外实验数据和模拟预测, CO2浓度倍增后, 中国森林生产力的增幅在12%-35% (
Priming depletes soil carbon and releases nitrogen in a scrub-oak ecosystem exposed to elevated CO2
1
2009
... CO2浓度升高条件下土壤微生物也可能通过改变土壤中的分解过程对大气CO2浓度产生正或负的反馈作用.一方面, CO2浓度升高可引起微生物活性增加(
Soil carbon sequestration in a pine forest after 9 years of atmospheric CO2 enrichment
1
2008
... 在大气CO2浓度升高条件下, 土壤微生物通过调控土壤过程对植物生长可能产生正或负效应.短时间的CO2浓度升高条件下微生物促进有机氮矿化, 增加土壤氮的有效性和植物对无机氮的吸收(
Hidden shift of the ionome of plants exposed to elevated CO2 depletes minerals at the base of human nutrition
1
2014
... CO2浓度升高显著降低植物组织氮含量(
Food for thought: lower-than-expected crop yield stimulation with rising CO2 concentrations
2
2006
... FACE系统是目前研究CO2浓度升高对地上-地下生态系统影响及其机理最为理想的方法.FACE系统相比其他模拟手段不会限制植物的根系, 也不会对风、光照和辐射等产生影响, 因此能够满足长期持续地研究CO2浓度升高对整个生态系统的影响(
... 大气CO2浓度升高的“施肥效应”刺激植物光合作用和生产力, 总体上促进陆地植被的碳固定.根据国内外实验数据和模拟预测, CO2浓度倍增后, 中国森林生产力的增幅在12%-35% (
Rising atmospheric carbon dioxide: plants face the future
1
2004
... CO2浓度升高显著降低植物组织氮含量(
Progressive nitrogen limitation of ecosystem responses to rising atmospheric carbon dioxide
5
2004
... 工业革命以来, 人类活动导致化石燃料过量使用和土地利用方式改变等, 引发了大气中CO2等温室气体浓度的急剧上升.目前大气中CO2浓度已由1860年的280 μL·L-1升高至目前的410 μL·L-1左右, 并以每年0.45%的速率升高, 预计到2030年将增加到550 μL·L-1 (
... 大气CO2浓度升高的“施肥效应”刺激植物光合作用和生产力, 总体上促进陆地植被的碳固定.根据国内外实验数据和模拟预测, CO2浓度倍增后, 中国森林生产力的增幅在12%-35% (
... 长期而言, CO2浓度升高对植物生长的刺激作用往往会导致更多的氮被固存在多年生的植物体内和土壤有机质中(
... 下调的反应(
... 在大气CO2浓度升高条件下, 土壤微生物通过调控土壤过程对植物生长可能产生正或负效应.短时间的CO2浓度升高条件下微生物促进有机氮矿化, 增加土壤氮的有效性和植物对无机氮的吸收(
Elevated CO2 stimulates net accumulations of carbon and nitrogen in land ecosystems: a meta-analysis
2
2006
... CO2浓度升高对于土壤“碳汇”功能, 以及对生态系统碳固定(植物和土壤)的综合影响尚不清楚.大气CO2浓度升高既促进地上生物量的增加, 也促进凋落物归还量及地下部分生物量的增加.CO2浓度升高还可增加粗微团聚体形成的稳定性和碳保护功能, 从而提高土壤碳的稳定性(
... 长期而言, CO2浓度升高对植物生长的刺激作用往往会导致更多的氮被固存在多年生的植物体内和土壤有机质中(
Nitrogen distribution in leaf canopies of high-yielding rice cultivar Takanari
1
2017
... 水稻(
Increasing CO2 threatens human nutrition
2
2014
... 水稻(
... CO2浓度升高下作物的品质会受到影响, 包含营养品质、外观品质、加工品质和食味品质.营养品质方面, CO2浓度升高增加植物体内的碳含量, 并对氮、锌、镁等其他元素产生一定的稀释作用(
Quantitative trait loci for large sink capacity enhance rice grain yield under free-air CO2 enrichment conditions
2017
Elevated CO2: litter chemistry, and decomposition: a synthesis
1
2001
... CO2浓度升高条件下土壤微生物也可能通过改变土壤中的分解过程对大气CO2浓度产生正或负的反馈作用.一方面, CO2浓度升高可引起微生物活性增加(
Rising CO2 future ecosystems
1
2001
... CO2浓度升高对植物影响的模拟实验研究可以追溯到20世纪60年代(
Leaf area compensation and nutrient interactions in CO2-enriched seedlings of yellow- poplar (Liriodendron tulipifera L.)
1
1991
... 提高水分利用效率(WUE)是大气CO2浓度升高对植物生长最有利的影响之一.在CO2浓度升高下, 植物出现不同程度的气孔密度和气孔导度下降、Rubisco酶活性提高、羧化效率提高、净光合速率升高, 这一系列的有效生理调控途径导致WUE的提高(
CO2 enhancement of forest productivity constrained by limited nitrogen availability
1
2010
... 长期而言, CO2浓度升高对植物生长的刺激作用往往会导致更多的氮被固存在多年生的植物体内和土壤有机质中(
Ecological lessons from Free-Air CO2 Enrichment (FACE) experiments
2
2011
... FACE系统是目前研究CO2浓度升高对地上-地下生态系统影响及其机理最为理想的方法.FACE系统相比其他模拟手段不会限制植物的根系, 也不会对风、光照和辐射等产生影响, 因此能够满足长期持续地研究CO2浓度升高对整个生态系统的影响(
... 浓度增加对生态系统净生产力的促进作用可能并没有想象的那么高, 存在短期显著促进但长期的促进作用会削弱的效果(
Transient nature of CO2 fertilization in Arctic tundra
1
1994
... 大气CO2浓度升高的“施肥效应”刺激植物光合作用和生产力, 总体上促进陆地植被的碳固定.根据国内外实验数据和模拟预测, CO2浓度倍增后, 中国森林生产力的增幅在12%-35% (
Nitrogen resorption in senescing leaf blades of rice exposed to free-air CO2 enrichment (FACE) under different N fertilization levels
1
2017
... 水稻(
Below-ground process responses to elevated CO2 and temperature: a discussion of observation, measurement methods, and models
1
2004
... CO2浓度升高会增加根际沉积(
Response of small birch plants (Betula pendula Roth.) to elevated CO2 and nitrogen supply
1993
Enhanced root exudation induces microbial feedbacks to N cycling in a pine forest under long-term CO2 fumigation
1
2011
... 长期而言, CO2浓度升高对植物生长的刺激作用往往会导致更多的氮被固存在多年生的植物体内和土壤有机质中(
Roots and fungi accelerate carbon and nitrogen cycling in forests exposed to elevated CO2
1
2012
... 长期而言, CO2浓度升高对植物生长的刺激作用往往会导致更多的氮被固存在多年生的植物体内和土壤有机质中(
Leaf gas exchange and carbon isotope composition responses to drought in a drought-avoiding (Pinus pinaster) and a drought-tolerant (Quercus petraea) species under present and elevated atmospheric CO2 concentrations
1996
Comparison of ecosystem water-use efficiency among Douglas-fir forest, aspen forest and grassland using eddy covariance and carbon isotope techniques
1
2006
... 提高水分利用效率(WUE)是大气CO2浓度升高对植物生长最有利的影响之一.在CO2浓度升高下, 植物出现不同程度的气孔密度和气孔导度下降、Rubisco酶活性提高、羧化效率提高、净光合速率升高, 这一系列的有效生理调控途径导致WUE的提高(
Nitrogen limitation constrains sustainability of ecosystem response to CO2
5
2006
... 工业革命以来, 人类活动导致化石燃料过量使用和土地利用方式改变等, 引发了大气中CO2等温室气体浓度的急剧上升.目前大气中CO2浓度已由1860年的280 μL·L-1升高至目前的410 μL·L-1左右, 并以每年0.45%的速率升高, 预计到2030年将增加到550 μL·L-1 (
... 大气CO2浓度升高的“施肥效应”刺激植物光合作用和生产力, 总体上促进陆地植被的碳固定.根据国内外实验数据和模拟预测, CO2浓度倍增后, 中国森林生产力的增幅在12%-35% (
... 长期而言, CO2浓度升高对植物生长的刺激作用往往会导致更多的氮被固存在多年生的植物体内和土壤有机质中(
... 浓度升高下的PNL响应存在差异.现有研究表明, PNL多发生在草地生态系统(
... 在大气CO2浓度升高条件下, 土壤微生物通过调控土壤过程对植物生长可能产生正或负效应.短时间的CO2浓度升高条件下微生物促进有机氮矿化, 增加土壤氮的有效性和植物对无机氮的吸收(
Unexpected reversal of C3 versus C4 grass response to elevated CO2 during a 20-year field experiment
1
2018
... 水稻(
The response of terrestrial ecosystems to global climate change: towards an integrated approach
1
2008
... 随着全球尺度高密度生态网络观测的发展, 大型联网控制实验系统的完善, 以及大数据时代的多源数据整合分析技术的日臻成熟, CO2研究逐步从单一生态过程研究向多过程耦合研究发展, 从典型生态系统向区域、全球尺度生态系统综合集成研究转变.基于生态系统网络的多尺度、长期观测与联网实验将揭示单个站点所无法回答的大尺度的生态问题.目前陆地生态系统与大气和气候变化研究网络(Terrestrial Ecosystem Responses to Atmosphere and Climate Change, TERACC)在全球已经囊括数百个全球变化控制实验研究站点(
Nitrogen cycle responses to elevated CO2 depend on ecosystem nutrient status
1
2015
... 长期而言, CO2浓度升高对植物生长的刺激作用往往会导致更多的氮被固存在多年生的植物体内和土壤有机质中(
Lodging in rice can be alleviated by atmospheric CO2 enrichment
1
2007
... 水稻(
Recent trends and drivers of regional sources and sinks of carbon dioxide
1
2015
... 提高水分利用效率(WUE)是大气CO2浓度升高对植物生长最有利的影响之一.在CO2浓度升高下, 植物出现不同程度的气孔密度和气孔导度下降、Rubisco酶活性提高、羧化效率提高、净光合速率升高, 这一系列的有效生理调控途径导致WUE的提高(
Effects of elevated CO2 on the protein concentration of food crops: a meta-analysis
1
2008
... CO2浓度升高下作物的品质会受到影响, 包含营养品质、外观品质、加工品质和食味品质.营养品质方面, CO2浓度升高增加植物体内的碳含量, 并对氮、锌、镁等其他元素产生一定的稀释作用(
Why are nitrogen concentrations in plant tissues lower under elevated CO2? A critical examination of the hypotheses
2
2008
... 水稻(
... CO2浓度升高显著降低植物组织氮含量(
Does a freely tillering wheat cultivar benefit more from elevated CO2 than a restricted tillering cultivar in a water-limited environment?
1
2015
... 水稻(
Influence of free-air CO2 enrichment (FACE) on the eating quality of rice
1
2005
... 除营养品质外, 外观品质也直接影响稻米等作物的加工品质和市场价格.水稻FACE研究发现CO2浓度升高条件下大米的精米率和头等米率显著降低2.0%和23.5%, 精米的垩白籽粒率和垩白度显著增加(
Plant responses to CO2: background and perspectives
2
2014
... 工业革命以来, 人类活动导致化石燃料过量使用和土地利用方式改变等, 引发了大气中CO2等温室气体浓度的急剧上升.目前大气中CO2浓度已由1860年的280 μL·L-1升高至目前的410 μL·L-1左右, 并以每年0.45%的速率升高, 预计到2030年将增加到550 μL·L-1 (
... CO2浓度升高对植物影响的模拟实验研究可以追溯到20世纪60年代(
Ecosystem responses to elevated CO2 governed by plant-soil interactions and the cost of nitrogen acquisition
4
2018
... 长期而言, CO2浓度升高对植物生长的刺激作用往往会导致更多的氮被固存在多年生的植物体内和土壤有机质中(
... 在大气CO2浓度升高条件下, 土壤微生物通过调控土壤过程对植物生长可能产生正或负效应.短时间的CO2浓度升高条件下微生物促进有机氮矿化, 增加土壤氮的有效性和植物对无机氮的吸收(
... 浓度升高条件下, 微生物提供的可利用氮库往往跟不上植物对氮的需求, 较易发生PNL, 从而使得土壤呼吸变弱, 生物量积累变小.由于大量的氮来不及被植物利用, 土壤有机碳库增加(
... CO2浓度升高条件下土壤微生物也可能通过改变土壤中的分解过程对大气CO2浓度产生正或负的反馈作用.一方面, CO2浓度升高可引起微生物活性增加(
Some influences of CO2 enrichment nitrogen nutrition and competition on grain yield and quality in spring wheat and barley
1
1994
... 增施氮肥可以在一定程度上提高水稻的施肥效应, 进一步提高CO2浓度升高下的产量(
Responses of forest ecosystems to increasing N deposition in China: a critical review
2
2018
... 随着人们生活水平的提高, 对饮食的需求正在从过去的数量型向现在的营养型转变.21世纪的粮食安全将由以数量为主转向数量与质量并重的方向发展, 不仅要解决全世界人口的温饱问题, 还要对粮食的营养价值给予足够的重视.在全球变化等多因子的复合作用下, CO2浓度升高对作物增产的正效应能被O3浓度升高(
... 现有的模拟CO2浓度升高的研究对不同生态系统关注度存在较大的差异.实验系统多建立在温带草原和森林生态系统中, 并且多处在北美和欧洲.对广泛分布于发展中国家和地区的热带和亚热带雨林、稀树草原知之甚少.热带和亚热带生态系统包含了陆地约50%的碳储量, 大气氮沉降和土壤氮有效性与温带、寒温带地区有明显差异(
Crop quality under rising atmospheric CO2
1
2018
... 随着人们生活水平的提高, 对饮食的需求正在从过去的数量型向现在的营养型转变.21世纪的粮食安全将由以数量为主转向数量与质量并重的方向发展, 不仅要解决全世界人口的温饱问题, 还要对粮食的营养价值给予足够的重视.在全球变化等多因子的复合作用下, CO2浓度升高对作物增产的正效应能被O3浓度升高(
Heat-tolerant rice cultivars retain grain appearance quality under free-air CO2 enrichment
1
2014
... 除营养品质外, 外观品质也直接影响稻米等作物的加工品质和市场价格.水稻FACE研究发现CO2浓度升高条件下大米的精米率和头等米率显著降低2.0%和23.5%, 精米的垩白籽粒率和垩白度显著增加(
Rice grain yield and quality responses to free-air CO2 enrichment combined with soil and water warming
2
2016
... 除营养品质外, 外观品质也直接影响稻米等作物的加工品质和市场价格.水稻FACE研究发现CO2浓度升高条件下大米的精米率和头等米率显著降低2.0%和23.5%, 精米的垩白籽粒率和垩白度显著增加(
... 提高水分利用效率(WUE)是大气CO2浓度升高对植物生长最有利的影响之一.在CO2浓度升高下, 植物出现不同程度的气孔密度和气孔导度下降、Rubisco酶活性提高、羧化效率提高、净光合速率升高, 这一系列的有效生理调控途径导致WUE的提高(
Responses of wild C4 and C3 grass (Poaceae) species to elevated atmospheric CO2 concentration: a meta-analytic test of current theories and perceptions
1999
Responses of yield CH4 and N2O emissions to elevated atmospheric temperature and CO2 concentration in a double rice cropping system
1
2018
... 随着人们生活水平的提高, 对饮食的需求正在从过去的数量型向现在的营养型转变.21世纪的粮食安全将由以数量为主转向数量与质量并重的方向发展, 不仅要解决全世界人口的温饱问题, 还要对粮食的营养价值给予足够的重视.在全球变化等多因子的复合作用下, CO2浓度升高对作物增产的正效应能被O3浓度升高(
Response of rice production to elevated [CO2] and its interaction with rising temperature or nitrogen supply: a meta- analysis
1
2015
... 随着人们生活水平的提高, 对饮食的需求正在从过去的数量型向现在的营养型转变.21世纪的粮食安全将由以数量为主转向数量与质量并重的方向发展, 不仅要解决全世界人口的温饱问题, 还要对粮食的营养价值给予足够的重视.在全球变化等多因子的复合作用下, CO2浓度升高对作物增产的正效应能被O3浓度升高(
高大气CO2浓度下C3植物叶片水分利用效率升高的研究进展
1
2010
... 提高水分利用效率(WUE)是大气CO2浓度升高对植物生长最有利的影响之一.在CO2浓度升高下, 植物出现不同程度的气孔密度和气孔导度下降、Rubisco酶活性提高、羧化效率提高、净光合速率升高, 这一系列的有效生理调控途径导致WUE的提高(
高大气CO2浓度下C3植物叶片水分利用效率升高的研究进展
1
2010
... 提高水分利用效率(WUE)是大气CO2浓度升高对植物生长最有利的影响之一.在CO2浓度升高下, 植物出现不同程度的气孔密度和气孔导度下降、Rubisco酶活性提高、羧化效率提高、净光合速率升高, 这一系列的有效生理调控途径导致WUE的提高(
The space-time continuum: the effects of elevated CO2 and temperature on trees and the importance of scaling
1
2015
... 全球变化因子间通常不是独立的, 而是相互作用的.目前对CO2浓度升高单因子变化研究取得较大进展, 而多因子交互作用的研究较少, 研究结果存在很大的不确定性.全球变化包括了CO2/O3浓度升高、干旱胁迫、氮沉降和降水格局分布等多因子复合影响, 生态系统在复合因子影响下往往呈现复杂的作用特征.比如O3浓度升高和增温对于植物的负效应往往能抵消一部分CO2浓度升高的“施肥”正效应(
Crop growth responses to free air CO2 enrichment and nitrogen fertilization: rotating barley ryegrass, sugar beet and wheat
1
2012
... 增施氮肥可以在一定程度上提高水稻的施肥效应, 进一步提高CO2浓度升高下的产量(
Dynamic analysis of the impact of free-air CO2 enrichment (FACE) on biomass and N uptake in two contrasting genotypes of rice
1
2018
... 水稻(
Influences of free-air CO2 enrichment (FACE) nitrogen fertilizer and crop residue incorporation on CH4 emissions from irrigated rice fields
1
2012
... 增施氮肥可以在一定程度上提高水稻的施肥效应, 进一步提高CO2浓度升高下的产量(
Effects of elevated CO2 and N fertilization on CH4 emissions from paddy rice fields
1
2004
... 增施氮肥可以在一定程度上提高水稻的施肥效应, 进一步提高CO2浓度升高下的产量(
Yield formation of CO2-enriched inter- subspecific hybrid rice cultivar Liangyoupeijiu under fully open-air field condition in a warm sub-tropical climate
1
2009
... 水稻(
The impact of free-air CO2 enrichment (FACE) and nitrogen supply on grain quality of rice
3
2007
... 增施氮肥可以在一定程度上提高水稻的施肥效应, 进一步提高CO2浓度升高下的产量(
... CO2浓度升高下作物的品质会受到影响, 包含营养品质、外观品质、加工品质和食味品质.营养品质方面, CO2浓度升高增加植物体内的碳含量, 并对氮、锌、镁等其他元素产生一定的稀释作用(
... 除营养品质外, 外观品质也直接影响稻米等作物的加工品质和市场价格.水稻FACE研究发现CO2浓度升高条件下大米的精米率和头等米率显著降低2.0%和23.5%, 精米的垩白籽粒率和垩白度显著增加(
Simulation of the effects of genotype and N availability on rice growth and yield response to an elevated atmospheric CO2 concentration
1
2011
... 增施氮肥可以在一定程度上提高水稻的施肥效应, 进一步提高CO2浓度升高下的产量(
Elevated atmospheric CO2, fine roots and the response of soil microorganisms: a review and hypothesis
3
2000
... 工业革命以来, 人类活动导致化石燃料过量使用和土地利用方式改变等, 引发了大气中CO2等温室气体浓度的急剧上升.目前大气中CO2浓度已由1860年的280 μL·L-1升高至目前的410 μL·L-1左右, 并以每年0.45%的速率升高, 预计到2030年将增加到550 μL·L-1 (
... 在大气CO2浓度升高条件下, 土壤微生物通过调控土壤过程对植物生长可能产生正或负效应.短时间的CO2浓度升高条件下微生物促进有机氮矿化, 增加土壤氮的有效性和植物对无机氮的吸收(
... CO2浓度升高条件下土壤微生物也可能通过改变土壤中的分解过程对大气CO2浓度产生正或负的反馈作用.一方面, CO2浓度升高可引起微生物活性增加(
Grain growth of different rice cultivars under elevated CO2 concentrations affects yield and quality
1
2015
... 水稻(
Soil microbial responses to experimental warming and clipping in a tallgrass prairie
1
2005
... CO2浓度升高会增加根际沉积(
Elevated CO2 improves lodging resistance of rice by changing physicochemical properties of the basal internodes
1
2019
... 水稻(
Growth and photosynthetic response of nine tropical species with long-term exposure to elevated carbon dioxide
1
1991
... Positive ecological effect of the rising CO2 concentrations on water use efficiency (WUE) of plants
植物名称 Plant name | 暴露时间(天) Exposure time (Day) | WUE (%) | 参考文献 Reference |
---|---|---|---|
海岸松 Pinus pinaster | 79 | +48 | Picon et al., 1996 |
垂枝桦 Betula pendula | 45 | +39 | Pettersson et al., 1993 |
北美鹅掌楸 Liriodendron tulipifera | Norby & O’Neill, 1991 | ||
高营养 High nutrition | 168 | +93 | |
低营养 Low nutrition | 168 | +66 | |
Tabebuia rosea | - | +125 | |
木瓜 Chaenomeles sinensis | Cruz et al., 2016 | ||
高氮 High nitrogen | 45 | +84 | |
低氮 Low nitrogen | 45 | +30 |
Carbon cycle extremes during the 21st century in CMIP5 models: future evolution and attribution to climatic drivers
1
2014
... 提高水分利用效率(WUE)是大气CO2浓度升高对植物生长最有利的影响之一.在CO2浓度升高下, 植物出现不同程度的气孔密度和气孔导度下降、Rubisco酶活性提高、羧化效率提高、净光合速率升高, 这一系列的有效生理调控途径导致WUE的提高(