
Responses of ecosystem carbon exchange to multi-level water addition in an alpine meadow in Namtso of Qinghai-Xizang Plateau, China
GENG Xiao-Dong1,2, Xu Ri
通讯作者:
编委: 杨元合
责任编辑: 王葳
基金资助: |
Online:2018-03-20
Supported by: | SupportedbytheNationalNaturalScienceFoundationofChina( |

摘要
关键词:
Abstract
Keywords:
PDF (1210KB)摘要页面多维度评价相关文章导出EndNote|Ris|Bibtex收藏本文
引用本文
耿晓东, 旭日, 刘永稳. 青藏高原纳木错高寒草甸生态系统碳交换对多梯度增水的响应. 植物生态学报, 2018, 42(3): 397-405 doi:10.17521/cjpe.2015.0395
GENG Xiao-Dong, Xu Ri, LIU Yong-Wen.
陆地生态系统碳交换过程包括总生态系统生产力(GEP)和生态系统呼吸(ER), 两者平衡的结果为净生态系统碳交换(NEE)。水分是陆地生态系统碳循环过程的重要驱动因子(Reichstein et al., 2013; Williamson, 2013)。大量研究揭示增加水分促进陆地生态系统GEP和ER (Wu et al., 2011), NEE是这两个过程平衡后的结果, 决定了陆地生态系统对大气CO2的吸收能力。
青藏高原被称作“地球第三极”, 平均海拔4 000 m以上, 面积约257万km2, 占中国陆地面积的26.8%左右(张镱锂等, 2002)。过去几十年来, 青藏高原降水量整体上表现为增加(Gao et al., 2009; 杨凯等, 2010)。其中, 生长季降水量每10年增加14%, 增幅高于非生长季(Piao et al., 2010)。模型预测认为, 未来青藏高原降水仍以增加为主, 并将出现更多的极端气候(张人禾等, 2015)。总体上, 青藏高原未来将变得更加湿润(Li et al., 2010)。
高寒草甸生态系统是青藏高原重要的草地生态系统类型, 约占青藏高原总面积的35% (Zheng & Zhu, 2000)。受气候变化影响, 20世纪末青藏高原从较弱的碳排放或碳中和转变为碳吸收(Zhuang et al., 2010), 其中高寒草甸贡献了较大比例的碳吸收(约89.33%), 是青藏高原重要的碳汇(Yan et al., 2015)。研究表明, 在青藏高原高寒草甸, 降水是生态系统碳交换的主要驱动因子(Hu et al., 2013)。在青藏高原海北地区的观测结果(张法伟等, 2008)表明降水强度对日间NEE影响较弱, 但可降低夜间ER; 而那曲地区的研究结果表明, 增水20%的情况下, GEP和ER均升高, GEP升高的幅度高于ER, 因而降水增加促进了高寒草甸的NEE (干珠扎布, 2013), 当雄地区的观测结果(Fu et al., 2009)表明, 降水造成的土壤有效水分变化是影响碳交换的主要因素。因此, 未来的降水变化将对高寒草甸碳交换产生重要影响。
高寒草甸在青藏高原分布广泛, 而目前有关高寒草甸的降水变化与碳交换关系的研究较少, 且主要集中在增水20%左右, 更大幅度的降水增加对碳交换具有怎样的影响? 相关研究较为匮乏。我们在纳木错高寒草甸设置了6个梯度人工增水实验(最高增水100%), 获取青藏高原中部高寒草甸碳交换的观测数据, 探究未来不同尺度降水量增加的情况下, 纳木错高寒草甸碳交换的变化, 以及高寒草甸碳交换对降水增加的响应是否存在阈值。
1 材料和方法
1.1 研究区概况
本研究依托中国科学院纳木错多圈层综合观测研究站(30.77° N, 90.99° E, 海拔4 730 m, 以下简称纳木错站), 2009-2013年生长季日平均温度为7.13 ℃, 2009、2010、2011、2012和2013年年降水量分别为327、494、402、312和397 mm。研究样地位于青藏高原当雄县念青唐古拉山北麓与纳木错湖东南岸之间(30.73° N, 91.02° E), 为典型高寒草甸生态系统, 海拔4 900 m, 距离纳木错站西南约10 km, 2012-2013年生长季日平均温度7.5 ℃, 2012和2013年年降水量分别为311和489 mm (Wei et al., 2015)。高寒草甸地上生物量为89.1 g·m-2, 0-10 cm土壤有机碳含量为29.1 mg·g-1, 土壤全氮值为2.0 mg·g-1, 土壤pH值为7.4 (刘永稳, 2014)。1.2 试验设计
在高寒草甸研究样地设置增水0% (对照)、增水20%、增水40%、增水60%、增水80%、增水100%共6个梯度的水分处理, 每个处理设置4个重复(图1)。每个重复的小区域内6个不同处理装置均随机分布。图1表示增水处理在整个样地的位置以及分布情况, 不代表各不同处理的实际位置。采用立方体不锈钢集雨装置收集样地附近的雨水, 通过带孔橡胶管均匀洒入样地, 以此增加样地的实际降水量。例如, 对于20%增水处理, 集雨装置的有效集雨面积为处理样地面积的20%, 因此降水结束后, 该处理样地可以得到额外的20%的降水, 其余处理以此类推。在每个处理样地扣入一个正方形不锈钢底座 (50 cm × 50 cm), 用以观测CO2通量。在2012年冬季完成整套实验装置的安装, 并于2013年8月14日和16日开始进行观测, 2014年分别在7月14日、19日、25日、31日, 8月2日、17日、18日, 9月6日和8日进行观测。图1
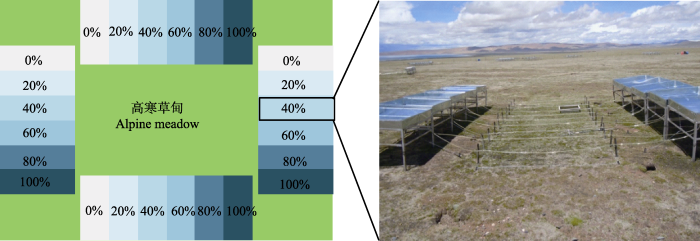
图1增水样地设计分布与增水装置现场。0%-100%表示降水增加的百分比。
Fig. 1Layout of water addition treatments and of the field experimental site. Values 0%-100% represent the percentage of increases in precipitation.
1.3 数据观测处理
采用红外CO2分析仪(LI-840, LI-COR, Lincoln, USA)观测不同处理样地的NEE和ER。观测时间为北京时间10:00到12:00, 此时段当地碳吸收通常达到最大值(徐玲玲等, 2005)。1.3.1 NEE测定
将可透光线塑料箱(50 cm × 50 cm × 50 cm, 箱有5个面, 箱内有2个电动小型风扇)扣在底座上, 用铁夹固定, 通过空气导管连接箱内与红外分析仪, 观测时打开空气泵与风扇, 空气泵可输送箱内空气样本进入分析仪, 风扇可加速箱内空气混合。分析仪每秒记录一次箱内CO2浓度, 记录约120 s即完成一次观测。
1.3.2 ER测定
完成NEE观测后, 从底座上移开明箱, 待箱内通风至空气基本与大气本底值接近时, 扣于底座上固定, 并罩上遮光布, 打开红外分析仪, 记录约120 s, 记录频率同样是每秒1次。完成数据观测后, 参考Jasoni等(2005)的文献, NEE和ER的计算公式如下:
$Flux=\frac{V\times P\times (1000-W)}{R\times S\times (T+273)}\times \frac{d{C}'}{dt}$
Flux表示NEE和ER的通量(μmol·m-2·s-1); V是箱内所包含的气室体积(m3); P是观测时箱内平均大气压(kPa); W是观测时箱内平均水汽分压(mmol·mol-1); R为标准气体常数(8.314 J·mol-1·K-1); S是底座面积(m2); T是观测期间箱内平均气温(℃); C?是经过水汽校正的CO2浓度, C? = C/(1 - W/1000), C是红外分析仪直接观测时记录的箱内CO2浓度(μmol·mol-1); dC?/dt是箱内CO2浓度随时间变化的速率。GEP的计算公式为GEP = -NEE + ER (Flanagan et al., 2002)。
1.4 气象数据获取
在每一个处理样地中各埋设一个微型环境气象记录仪HOBO (H21, Onset Corp, Pocasset, USA), 其2个探头埋入处理样地地下5 cm处, 记录土壤温度和土壤体积含水量的动态变化。使用雨量筒(RG- 3, Onset, Pocasset, USA)收集降水信息; 同时利用电子温度计(JM-624, 今明仪器有限公司, 天津)和简易手持湿度计(Delta-T Devices, Cambridge, UK)测定土壤5 cm深度的温度和体积含水量。1.5 统计分析
采用单因素方差分析, 检验NEE、GEP、ER、土壤温度以及水分在处理之间的差异显著性。统计分析采用SPSS 19.0完成, 图表使用Origin 9.1绘制。2 结果
2.1 降水与梯度增水处理效应
从生长季的降水分布来看, 2013年降水主要集中在8月上半月和9月, 全年降水量为489 mm; 2014年生长季降水主要集中在7月底和8月下旬, 全年降水量为320 mm。2013和2014年的HOBO数据记录了土壤含水量(本文指代土壤体积含水量)对降水变化的响应, 基本上, 降水量较大时对应的土壤含水量也较大。多梯度增水处理之间的土壤含水量出现差异(图2)。但是微型自动气象站只有一个水分探头, 所测数据为一个固定样点的土壤含水量动态变化, 在代表性上存在缺陷, 如2014年8月18日到23日增水20%处理的土壤含水量出现异常变化, 因此在碳交换观测的同时, 使用手持湿度计对各处理样地的土壤含水量进行多点重复测量。根据湿度计的数据, 2013年各处理之间土壤含水量由低到高依次是: 增水20%、对照、增水40%、增水60%、增水80%、增水100%; 2014年各处理土壤含水量由低到高依次是: 对照、增水20%、增水40%、增水60%、增水80%、增水100% (图3A、3B)。
图2
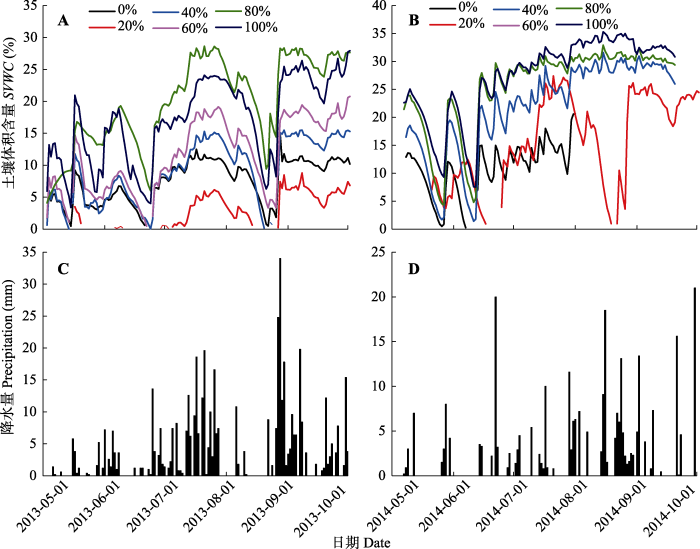
图22013年和2014年高寒草甸生长季不同增水处理地下5 cm处土壤体积含水量(A, B)以及降水量(C, D)的动态变化。0%-100%分别表示不同增水处理。
Fig. 2Seasonal variations in soil volumetric water content (SVWC) at 5 cm depth under different water addition treatments (A, B) and precipitation for alpine meadow (C, D) in 2013 and 2014. Values 0%-100% represent different levels of water addition treatments.
图3
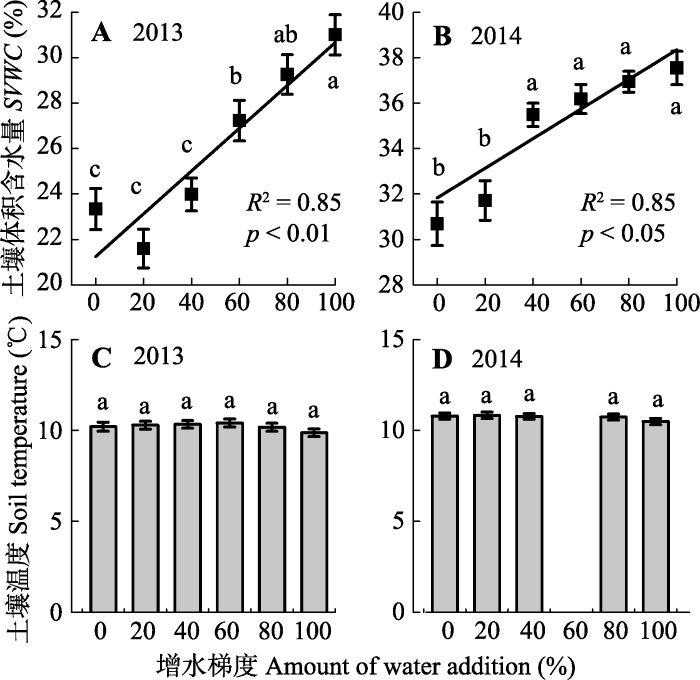
图32013年和2014年生长季不同增水处理地下5 cm处土壤体积含水量(A, B)和土壤温度(C, D) (平均值±标准误差)。相同的字母代表增水处理之间最小显著差异(LSD)法多重比较结果差异不显著(p > 0.05), 不同字母表示处理间差异显著(p < 0.05), 其中2014年增水60%处理的温度值缺失。
Fig. 3Soil volumetric water content (SVWC) (A, B) and temperature (C, D) at 5 cm depth under different water addition treatments in the growing seasons of 2013 and 2014 (mean ± SE). Same letters indicate a non-significant difference (p > 0.05) according to the least significant difference (LSD) test, and different letters indicate significant differences (p < 0.05) among treatments. Temperature data were not available for the water addition treatment of 60% in 2014.
2013和2014年多梯度增水处理后, 各处理的土壤含水量发生显著变化(p < 0.05)。2013年, 较之对照, 增水20%、增水40%、增水60%、增水80%、增水100%导致土壤含水量分别增加-7.46%、2.75%、16.67%、25.35%、32.85%; 2014年, 较之对照, 增水20%、增水40%、增水60%、增水80%、增水100%后, 土壤含水量分别增加3.33%、15.62%、17.87%、20.35%、22.32%。线性拟合的结果表明, 随着模拟增水量的增加, 总体上土壤含水量呈持续增加的趋势(图3 A、3B)。
2013年, 对照、增水20%、增水40%、增水60%、增水80%、增水100%等6个增水处理样地生长季平均地下5 cm土壤温度分别为10.21、10.29、10.34、10.41、10.18和9.87 ℃; 由于微型自动气象站(HOBO)出现故障, 2014年增水60%处理土壤温度数据缺失, 对照、增水20%、增水40%、增水80%、增水100%等处理梯度的温度平均值分别为10.79、10.83、10.77、10.74和10.49 ℃。各处理梯度之间土壤温度没有显著差异(2013年p = 0.298, 2014年p = 0.692) (图3C、3D)。
2.2 NEE的季节动态
2013年NEE的观测仅在8月份进行, 相对于整个生长季(5-9月)时间尺度来说较为短暂。我们采用2014年的观测数据(7、8、9三个月), 探究高寒草甸NEE (对照处理)在时间尺度上的变化趋势。2014年的降水量与地下5 cm温度变化有密切关系, 总体上降水较多的时期温度也较高。从整个生长季来看, 高寒草甸NEE均为负值, 表示生态系统对CO2净吸收, 在生长季表现为碳汇。NEE在温度较高以及降水较多的8月初期和9月达到较低值, 在降水较少的时期达到较高值(图4)。图4
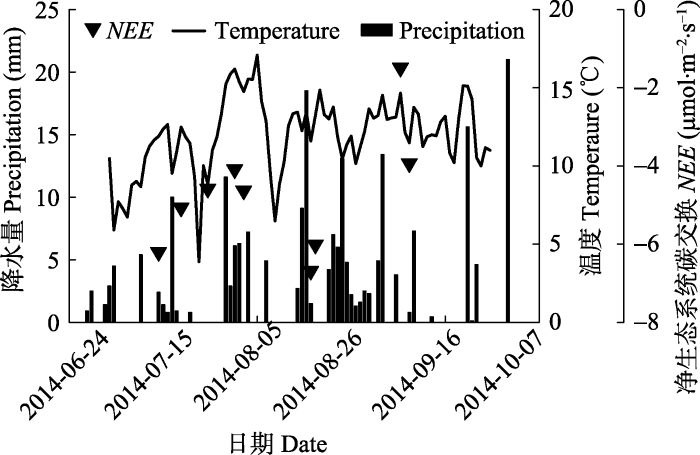
图42014年净生态系统碳交换(NEE, ▼) (对照处理)、降水(柱状图)和温度(线图)的季节变化, NEE值采用每日多次测定平均值; 温度为地下5 cm温度。
Fig. 4Seasonal dynamics of net ecosystem carbon exchange (NEE, ▼) (control), precipitation (column) and temperature (line) in 2014. The NEE value is the mean of multiple investigations in a day, and the temperature refers to the soil temperature at 5 cm depth.
2.3 增水处理后各梯度碳通量的变化
如图5A、5B所示, 较之于对照, 增水20%处理的NEE相对较高, 增水40%以及随后的处理对应的NEE较之于增水20%处理逐渐降低。2013年8月的观测结果表明, 增水处理后最大NEE平均值为-5.27 μmol·m-2·s-1; 2014年在7月、8月、9月都进行观测, 增水处理的最大NEE平均值为-6.34 μmol·m-2·s-1。2013年, 与对照相比, 5个不同梯度增水处理的NEE均未发生显著变化(p > 0.05)。然而, 从增水20%开始, 随着水分不断增加, 净碳吸收受到抑制, 80%和100%增水处理与增水20%处理的NEE具有显著差异(p < 0.05)。2014年增水20%的NEE与对照、增水60%、增水80%、增水100%等处理具有显著性差异(p < 0.05)。图5
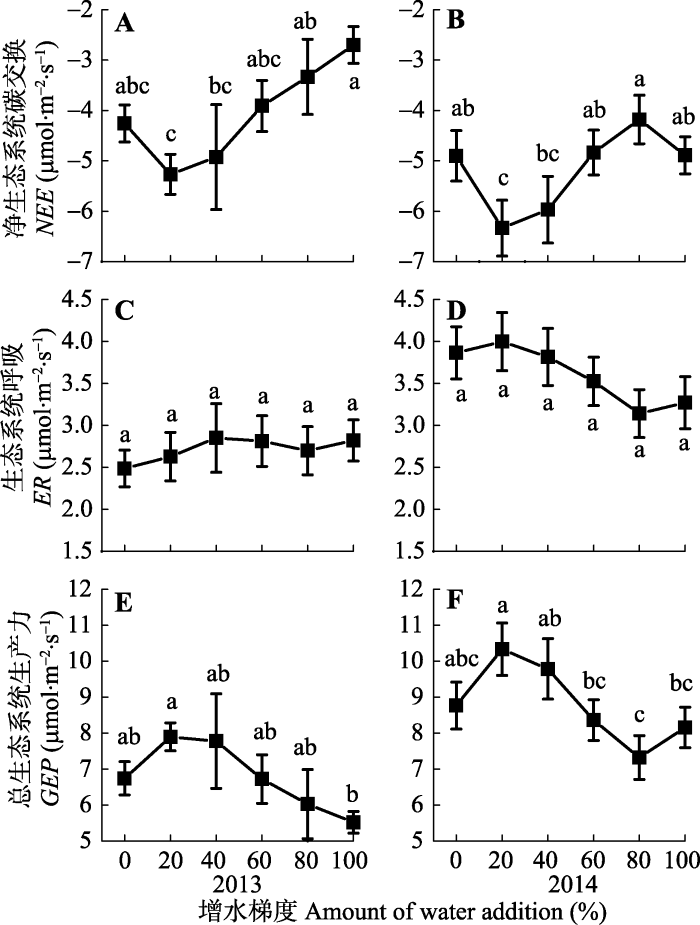
图52013-2014年生长季不同增水处理下的净生态系统碳交换(NEE) (A, B)、生态系统呼吸(ER) (C, D)以及总生态系统生产力(GEP) (E, F), (平均值±标准误差)。相同的字母代表不同处理之间最小显著差异(LSD)法多重比较结果差异不显著(p > 0.05), 不同字母表示处理间差异显著(p < 0.05)。
Fig. 5Net ecosystem carbon exchange (NEE) (A, B), ecosystem respiration (ER) (C, D) and gross ecosystem production (GEP) (E, F) under different water addition treatments in the growing seasons of 2013 and 2014 (mean ± SE). Same letters indicate a non-significant difference (p > 0.05) according to the least significant difference (LSD) test, and different letters indicate significant differences (p < 0.05) among treatments.
2013和2014年, 不同梯度增水处理后, ER没有发生显著的变化(2013年p = 0.948; 2014年p = 0.309)。2013年ER的观测集中在8月份, 而2014年的观测跨越7、8、9月3个月, 其ER的平均值总体上大于2013年(图5C、5D)。
2013年, 随着增水梯度的增加, GEP先增加然后逐渐降低, 并于增水20%处理达到最大值, 但只有20%和100%增水处理之间具有显著差异(p < 0.05)。2014年, 随增水梯度增加, GEP先增加然后逐渐降低, 在增水20%处理达到最大值, 增加更多的降水后GEP逐渐降低, 但在增水100%处理中开始略有增加的趋势。多重比较结果显示, 与增水20%处理相比, 60%、80%、100%增水处理GEP具有显著差异(p < 0.05) (图5E、5F)。2013和2014年的观测结果均表明, 当20%增水处理后, 高寒草甸生态系统GEP和NEE达到最大值。
3 讨论
3.1 模拟降水增加对GEP的影响
中国大部分陆地生态系统的观测研究表明, 年降水量变化会对GEP造成显著影响(Yu et al., 2013)。在青藏高原高寒草甸, GEP依赖于草甸植被的光合作用。由于增水促进光合有效辐射(Zhu et al., 2016), 因而本研究中, 较之于对照, 增加20%增水处理后GEP有所增加, 但增水过量(超过20%)未能进一步促进GEP (图5E、5F)。在干旱生态系统, 水分是限制植物生长和GEP的重要因素(Weltzin et al., 2003)。例如, 在内蒙古草原的观测表明增水促进GEP (Niu et al., 2009; Bai et al., 2010)。这表明在受水分胁迫的生态系统, GEP对增水具有正反馈。相对于半干旱的草原, 温度对高寒生态系统尤为重要; 青藏高原高寒草甸生态系统较高寒草原更为湿润(Yang et al., 2011), 因此水分不是高寒草甸最主要的限制因子。当土壤水分过量时, 植物的生理生态过程会受到负面影响, 如代谢底物供应不足、产生有毒物质等(Jackson & Colmer, 2005), 这将导致植物光合效率的降低(Xu & Zhou, 2011), 因此, 在高寒草甸适度增加降水会促进GEP, 而水分过量时, 这种促进作用会受到抑制, 这表明纳木错高寒草甸GEP存在水分胁迫, 且20%以内的增水对GEP具有促进作用。3.2 模拟降水增加对ER的影响
降水变化会直接或间接地对植物生长和微生物过程造成影响, 继而影响ER (Wan et al., 2007)。对干旱地区的研究发现增水能够促进ER (Niu et al., 2009), 这主要是由于增水促进了植物的生长(Huxman et al., 2004)和微生物的活性等(Austin et al., 2004)。而在水分相对充裕的海北高寒草甸(土壤含水量达到39.1%), ER与土壤含水量为负相关关系(Hu et al., 2008)。本研究试验地比内蒙古干旱草原湿润, 但土壤含水量低于海北地区(图3A、3B)。增水处理后, ER并没有发生显著的变化(图5C、5D)。这说明在纳木错地区高寒草甸, 降水增加对ER的影响较弱。这可能是由于生态系统呼吸作用相关过程对降水增加的响应相互抵消。当水分明显增加时, 植物和微生物的生理过程不再受到水分胁迫, 但土壤含水量的变化改变了土壤通透性, 影响了土壤和大气之间的气体交换(Curry, 2007; Zhuang et al., 2013)。并且, 土壤含水量较高一方面促进了微生物所需的有机质的流通, 另一方面也降低了土壤O2浓度(Skopp et al., 1990)。另外, 土壤含水量也通过改变土壤呼吸的温度敏感性, 控制土壤的CO2排放(Zhou et al., 2014; Shen et al., 2015)。通过以上调节机制, 高寒草甸维持ER在水分变化中的稳定, 对增水的敏感性低于GEP。3.3 模拟降水增加对NEE的影响
由于增加降水对ER的影响较弱, NEE的变化主要取决于GEP (图5)。2013-2014年的观测结果表明, 适度增加降水(20%增水)促进高寒草甸NEE, 这主要是由于增水对GEP的促进超过对ER的促进作用。过量增加降水则导致植物的光合作用降低(Xu & Zhou, 2011), 因而削弱GEP可抑制生态系统对碳的吸收, 因此过量增水(>20%增水)不会导致NEE继续增加(图5A、5B)。这是高寒草甸生态系统碳交换应对气候变化的负反馈调节的重要环节(Marcolla et al., 2011)。2013-2014年的降水情况并不一致, 如降水强度和分布存在差异, 但是我们的观测结果表明在20%范围内的增水促进高寒草甸的碳吸收。Chen等(2009)在内蒙古草原的研究认为, 干旱生态系统碳吸收的能力受降水时间分布的影响比受降水量的影响更大。我们在高寒草甸的研究结果支持这一结论(图5A、5B)。目前青藏高原高寒草甸生态系统被认为是自然条件下较弱的碳汇(Gu et al., 2003; Kato et al., 2006; Zhao et al., 2006)。由于未来的气候变化有利于GEP的增加(Wu et al., 2011), 且低温导致高寒生态系统有机质分解较慢(Ni, 2002), 高寒草甸的固碳能力具有一定的提升空间。我们的结果表明未来降水增加会促进高寒草甸生长季在白天对碳的吸收。3.4 模拟增加降水设计及存在的问题
准确了解降水变化对生态系统的影响需要建立更加科学合理的实验设计, 这需要考虑包括降水的频率、大小以及与其他因素的相互作用等(Beier et al., 2012)。不同的生态系统面临的实际情况与问题存在差别, 国内外在草地生态系统进行的增水实验也得出不同的处理效应, 例如在内蒙古草原进行的多梯度人工增水试验, 模拟增加0、5、10、25和75 mm的降水, 处理后的土壤含水量从4.88%增加到13% (Chen et al., 2009); 在加拿大亚伯达地区矮草草原(short grassland)进行的增水50%的试验, 同时也设置了减水50%和对照处理, 但它们的土壤含水量没有发生显著变化(Flanagan et al., 2013); 在美国田纳西州人工草地进行的“少增水”(每周增加2 mm降水)和“多增水”(每周增加25 mm降水)试验表明其土壤含水量差异显著, 相应的土壤含水量分别为21.7%和26.2% (Wan et al., 2007)。高寒草甸海拔较高(平均4 000 m以上), 人烟稀少, 夏季平均温度低于8 ℃, 降水形式多样, 包括雨水、冰雹、降雪等。由于受自然条件的限制, 目前在该区域进行的增水试验较少。我们在纳木错进行的增水试验采用等面积收集雨水并均匀洒入样地的方式(详见试验设计), 一方面可使增水过程自动化, 另一方面也在一定程度上保证了增水的自然效果, 经过处理, 2013年和2014年最大土壤含水量分别增加了32.8%和22.3% (图3A、3B)。已有的研究通常采用一个增水标准(Beier et al., 2012), 而我们在高寒草甸进行6个梯度的增水, 可以较全面地研究降水变化(增加)对碳交换的影响。我们的装置存在两个方面的问题: 一是增水导致水分蒸散增加(Gu et al., 2008)以及引起一些土壤结构与温度等其他环境因子的改变(van der Molen et al., 2011), 二是高寒草甸生长季降水分布不均, 因此不同观测日期所对应的土壤含水量存在差异。
4 结论
在青藏高原高寒草甸生态系统, 增水显著改变土壤含水量, 并对生态系统碳交换产生重要影响。其中, 降水变化对ER影响较弱, 适度增加降水可以促进GEP, 并最终表现为对NEE的促进, 因此适量的降水增加(20%-40%)有利于高寒草甸生态系统碳吸收。作为青藏高原的碳汇, 未来高寒草甸生态系统在降水增加的情况下将会吸收更多的CO2。致谢 感谢中国科学院纳木错多圈层综合观测站提供的支持, 感谢中国科学院青藏高原研究所张国帅博士提供2014年的降水数据。
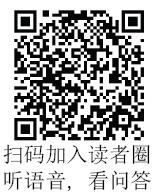
参考文献 原文顺序
文献年度倒序
文中引用次数倒序
被引期刊影响因子
DOI:10.1007/s00442-004-1519-1URLPMID:14986096 [本文引用: 1]

The episodic nature of water availability in arid and semiarid ecosystems has significant consequences on belowground carbon and nutrient cycling. Pulsed water events directly control belowground processes through soil wet-dry cycles. Rapid soil microbial response to incident moisture availability often results in almost instantaneous C and N mineralization, followed by shifts in C/N of microbially available substrate, and an offset in the balance between nutrient immobilization and mineralization. Nitrogen inputs from biological soil crusts are also highly sensitive to pulsed rain events, and nitrogen losses, particularly gaseous losses due to denitrification and nitrate leaching, are tightly linked to pulses of water availability. The magnitude of the effect of water pulses on carbon and nutrient pools, however, depends on the distribution of resource availability and soil organisms, both of which are strongly affected by the spatial and temporal heterogeneity of vegetation cover, topographic position and soil texture. The nverse texture hypothesis for net primary production in water-limited ecosystems suggests that coarse-textured soils have higher NPP than fine-textured soils in very arid zones due to reduced evaporative losses, while NPP is greater in fine-textured soils in higher rainfall ecosystems due to increased water-holding capacity. With respect to belowground processes, fine-textured soils tend to have higher water-holding capacity and labile C and N pools than coarse-textured soils, and often show a much greater flush of N mineralization. The result of the interaction of texture and pulsed rainfall events suggests a corollary hypothesis for nutrient turnover in arid and semiarid ecosystems with a linear increase of N mineralization in coarse-textured soils, but a saturating response for fine-textured soils due to the importance of soil C and N pools. Seasonal distribution of water pulses can lead to the accumulation of mineral N in the dry season, decoupling resource supply and microbial and plant demand, and resulting in increased losses via other pathways and reduction in overall soil nutrient pools. The asynchrony of resource availability, particularly nitrogen versus water due to pulsed water events, may be central to understanding the consequences for ecosystem nutrient retention and long-term effects on carbon and nutrient pools. Finally, global change effects due to changes in the nature and size of pulsed water events and increased asynchrony of water availability and growing season will likely have impacts on biogeochemical cycling in water-limited ecosystems.
DOI:10.1111/gcb.2010.16.issue-4URL [本文引用: 1]
DOI:10.1111/j.1461-0248.2012.01793.xURLPMID:22553898 [本文引用: 2]

Climatic changes, including altered precipitation regimes, will affect key ecosystem processes, such as plant productivity and biodiversity for many terrestrial ecosystems. Past and ongoing precipitation experiments have been conducted to quantify these potential changes. An analysis of these experiments indicates that they have provided important information on how water regulates ecosystem processes. However, they do not adequately represent global biomes nor forecasted precipitation scenarios and their potential contribution to advance our understanding of ecosystem responses to precipitation changes is therefore limited, as is their potential value for the development and testing of ecosystem models. This highlights the need for new precipitation experiments in biomes and ambient climatic conditions hitherto poorly studied applying relevant complex scenarios including changes in precipitation frequency and amplitude, seasonality, extremity and interactions with other global change drivers. A systematic and holistic approach to investigate how soil and plant community characteristics change with altered precipitation regimes and the consequent effects on ecosystem processes and functioning within these experiments will greatly increase their value to the climate change and ecosystem research communities. Experiments should specifically test how changes in precipitation leading to exceedance of biological thresholds affect ecosystem resilience and acclimation.
DOI:10.1111/j.1365-2486.2009.01879.xURL [本文引用: 1]

Abstract Precipitation pulses play an important role in regulating ecosystem carbon exchange and balance of semiarid steppe ecosystems. It has been predicted that the frequency of extreme rain events will increase in the future, especially in the arid and semiarid regions. We hypothesize that large rain pulses favor carbon sequestration, while small ones cause more carbon release in the semiarid steppes. To understand the potential response in carbon sequestration capacity of semiarid steppes to the changes in rain pulse size, we conducted a manipulative experiment with five simulated rain pulse sizes (0, 5, 10, 25, and 75mm) in Inner Mongolia steppe. Our results showed that both gross ecosystem productivity (GEP) and ecosystem respiration ( R e ) responded rapidly (within 24h) to rain pulses and the initial response time was independent of pulse size. However, the time of peak GEP was 1 3 days later than that of R e , which depended on pulse size. Larger pulses caused greater magnitude and longer duration of variations in GEP and R e . Differences in the response time of microbes and plants to wetting events constrained the response pattern of heterotrophic ( R h ) and autotrophic ( R a ) components of R e following a rain event. R h contributed more to the increase of R e in the early stage of rain pulse response, while R a played an more important role later, and determined the duration of pulse response, especially for large rain events of >10mm. The distinct responses of ecosystem photosynthesis and respiration to increasing pulse sizes led to a threshold in rain pulse size between 10 and 25mm, above which post wetting responses favored carbon sequestration. The disproportionate increase of the primary productivity of higher plants, compared with those in the activities of microbial decomposers to larger pulse events suggests that the carbon sequestration capacity of Inner Mongolia steppes will be sensitive to changes in precipitation size distribution rather than just precipitation amount.
DOI:10.1029/2006GB002818URL [本文引用: 1]

[1] A simple scheme for the soil consumption of atmospheric methane, based on an exact solution of the one-dimensional diffusion-reaction equation in the near-surface soil layer, is described. The model includes a parameterization of biological oxidation that is sensitive to soil temperature, moisture content, and land cultivation fraction. The scheme was incorporated in the Canadian Land Surface Scheme (CLASS), with forcing provided by a 21-a, global land meteorological data set, and was calibrated using multiyear field measurements. Application of the scheme on the global scale gives an annual mean sink strength of 28 Tg CH4 a0908081, with an estimated uncertainty range of 909000947 Tg CH4 a0908081. A strong seasonality is present at Northern Hemisphere high latitudes, with enhanced uptake during the summer months. Under the specified surface forcings, the oxidation parameterization is more sensitive to soil moisture than to temperature. Compared to the previous work of Ridgwell et al. (1999), our empirically based water stress parameterization reduces uptake more rapidly with decreasing soil moisture, resulting in a decrease of 09080450% in the potential global sink strength. Analysis of the geographical distribution of methane consumption shows that subtropical and dry tropical ecosystems account for over half of the global uptake.
DOI:10.1016/j.agrformet.2013.01.002URL [本文引用: 1]

The interacting effects of altered temperature and precipitation are expected to have significant consequences for ecosystem net carbon storage. Here we report the results of an experiment that evaluated the effects of elevated temperature and altered precipitation, alone and in combination, on plant biomass production and soil respiration rates in a northern Great Plains grassland, near Lethbridge, Alberta Canada. Open-top chambers and rain shelters were used to establish an experiment in 2011 with two temperature treatments (warmed and control), each combined with three precipitation treatments (minus 50%, ambient (no manipulation), and plus 50%). A smaller experiment with only the two temperature treatments was conducted in 2012, a year with less rain than 2011. Our objectives were to determine the sensitivity of plant biomass production and soil respiration to temperature and moisture manipulations, and to test for direct and indirect effects of the environmental changes on soil respiration rates. The experimental manipulations resulted primarily in a significant increase in air temperature in the warmed treatment. There were no significant temperature or precipitation treatment effects on soil moisture content. Aboveground biomass was not significantly affected by the experimental manipulations, but the warmed plots of the ambient precipitation treatment showed an increase in root biomass relative to the control plots in 2011. The warmed treatment increased the cumulative loss of carbon in soil respiration (July–September) compared to the control by 49702g02C02m 612 during 2011, and by 18502g02C02m 612 during 2012. This higher soil respiration rate in both years was not directly caused by significant differences among treatments in soil temperature or soil moisture, but was likely an indirect result of increased carbon substrate availability in the warmed relative to the control treatment.
DOI:10.1046/j.1365-2486.2002.00491.xURL [本文引用: 1]

Abstract Net ecosystem carbon dioxide (CO 2 ) exchange (NEE) was measured in a northern temperate grassland near Lethbridge, Alberta, Canada for three growing seasons using the eddy covariance technique. The study objectives were to document how NEE and its major component processes—gross photosynthesis (GPP) and total ecosystem respiration (TER)—vary seasonally and interannually, and to examine how environmental and physiological factors influence the annual C budget. The greatest difference among the three study years was the amount of precipitation received. The annual precipitation for 1998 (481.7mm) was significantly above the 1971–2000 mean (±SD, 377.9±97.0mm) for Lethbridge, whereas 1999 (341.3mm) was close to average, and 2000 (275.5mm) was significantly below average. The high precipitation and soil moisture in 1998 allowed a much higher GPP and an extended period of net carbon gain relative to 1999 and 2000. In 1998, the peak NEE was a gain of 5gCm 612 d 611 (day 173). Peak NEE was lower and also occurred earlier in the year on days 161 (3.2gCm 612 d 611 ) and 141 (2.4gCm 612 d 611 ) in 1999 and 2000, respectively. Change in soil moisture was the most important ecological factor controlling C gain in this grassland ecosystem. Soil moisture content was positively correlated with leaf area index (LAI). Gross photosynthesis was strongly correlated with changes in both LAI and canopy nitrogen (N) content. Maximum GPP ( A max : value calculated from a rectangular hyperbola fitted to the relationship between GPP and incident photosynthetic photon flux density (PPFD)) was 27.5, 12.9 and 8.608molm 612 s 611 during 1998, 1999 and 2000, respectively. The apparent quantum yield also differed among years at the time of peak photosynthetic activity, with calculated values of 0.0254, 0.018 and 0.018 during 1998, 1999 and 2000, respectively. The ecosystem accumulated a total of 111.9gCm 612 from the time the eddy covariance measurements were initiated in June 1998 until the end of December 2000, with most of that C gained during 1998. There was a net uptake of almost 21gCm 612 in 1999, whereas a net loss of 18gCm 612 was observed in 2000. The net uptake of C during 1999 was the combined result of slightly higher GPP (287.2 vs. 272.3gCm 612 year 611 ) and lower TER (266.6 vs. 290.4gCm 612 year 611 ) than occurred in 2000.
DOI:10.5194/bgd-6-8007-2009URL [本文引用: 1]

Chinese Academy of Sciences [KZCX2-YW-432, CXTD-Z2005-1]; National Natural Science Foundation of China [30700110, 30721140307, 30590381]; Institute of Geographic Sciences and Natural Resources Research [O66U0604SZ]
URL [本文引用: 1]

近年来,气候因子改变引起的区域性和全球性气候变化对陆地生态系统带来极大的冲击,对生态系统组成、产量、功能组织均有不同程度的影响。草地生态系统作为最重要、分布最广的陆地生态系统类型,在陆地碳循环中发挥着重要的作用。藏北地区草地面积巨大,具有海拔高、温度低、自然条件严酷的特点,是气候变化高敏感区域。小嵩草草甸生态系统是藏北地区主要的草地类型之一,相关研究数据显示该区域气候将在未来一段时间内呈暖湿化趋势,这必将对藏北小嵩草草甸生态系统结构和功能产生重大的影响。研究增温增雨对藏北小嵩草草甸生态系统碳交换的影响有助于确定在未来气候变化条件下草地生态系统碳储存能力,并明确气候变化对草地生态系统生产力、生物多样性的影响,有助于全方位监测草地生态系统对气候变化的响应机理。 本文以藏北地区小嵩草草甸草原为研究对象,利用开顶箱(Open top chamber, OTC)对草地进行被动增温,并结合生长季增加降雨措施模拟区域性气候变化对该地区的影响。试验包括四个处理,分别为对照(CK)、增温(Warming, W)、限制性增温(通过风扇控制OTC增温过高, LimitedWarming, LW)和限制性增温+增雨(添加20%的自然降雨, Limited Warming and PrecipitationEnhancement, LWP)。测定了增温增雨条件下小嵩草草甸土壤温湿度(-5cm、-15cm、-30cm)、空气温度(20cm)、草地生物量、生物多样性;系统的测定了小嵩草草甸碳交换各组分,包括生态系统净交换(Net ecosystem exchange, NEE)、生态系统呼吸(Ecosystem respiration, ER)、土壤呼吸(Soil respiration, SR)、蒸腾速率(Ecosystem evapotranspiration, ET)以及水分利用效率(Wateruse efficiency, WUE),分析了增温增雨条件下生态系统碳交换以及生产力和生物多样性的变化。主要结论如下: (1)生长季内,W处理5cm土壤温度增加1.02℃;W、LW和LWP平均气温分别增加1.91、1.53和1.37℃。LW和LWP处理以及W处理15cm以下土壤温度无显著变化。土壤湿度方面,OTC中5cm的土壤湿度降低,其中只有W处理达到显著性,OTC对15cm以下的土壤湿度无影响。 (2) OTC内物种地上生物量显著升高,W、LW和LWP处理生物量分别达到72.08、97.50和75.78g/m~2,显著高于对照处理(54.38g/m~2)。OTC中群落高度和盖度均有不同程度的提高,其中高度增加更为明显,W、LW和LWP处理群落高度分别增加78.3%、100.0%。和56.5%。物种重要值方面,禾本科植物早熟禾重要值在增温条件下显著升高,其他物种变化并不明显,可以认为早熟禾对增温的敏感性最高。增温条件下草地物种Shannon-Weiner多样性指数呈现增加的趋势,Simpson多样性指数和E.Pielou均匀度指数无显著变化。 (3)增温增雨对小嵩草草甸生态系统碳交换具有较强的促进作用。OTC内各处理GEP和ER均呈现出不同程度的升高,但GEP的升高幅度更大,因此小嵩草草甸NEE在增温条件下也得到了明显的促进。由于温度增加,小嵩草草甸生态系统蒸腾作用明显加强,这也导致了OTC内WUE明显降低,尤其在生长季初期的W和LW处理中较为明显。 (4)环境温湿度对生态系统碳交换包括NEE、GEP、ER影响显著,除了GEP与土壤温度,ER与土壤湿度无关外,其他指标均与土壤温湿度存在显著相关性,温湿度升高对碳交换具有促进作用。禾本科和莎草科植物生物量与各指标间也存在相关性,尤其是GEP与NEE,达到极显著水平(P 0.001),因此认为增温导致的光合产物增加量大多分配到了禾本科和莎草科植物中。 (5)增温增雨促进土壤呼吸增强,并且其相对增加幅度大于ER,使得SR在ER中的比例显著提高,生长季平均值最高达到51%,高于CK(36%)。与ER不同,SR与土壤水分密切相关,其温度敏感性低于ER。增温条件下ER和SR的土壤温度敏感性均呈现升高趋势。 (6)增温增雨条件下,生物量和生物多样性与气温之间存在线性相关,与土壤温湿度并无显著相关。生物量和生物多样性之间关系较为复杂,不同测度之间结果有所不同,物种多样性指数与生物量间的关系为单峰型,而均匀度指数的关系为U型。也从另一方面说明了禾本科和莎草科植物在小嵩草草甸生态系统中的主导地位在增温条件下进一步得到巩固。 增温增雨对藏北小嵩草草甸生态系统禾本科和莎草科植物的生长具有明显的促进作用。增温增雨条件下光合作用和呼吸作用增加的同时生态系统净吸收也显著升高,有助于小嵩草草甸生态系统碳储存,提高生态系统固碳能力。
URL [本文引用: 1]

近年来,气候因子改变引起的区域性和全球性气候变化对陆地生态系统带来极大的冲击,对生态系统组成、产量、功能组织均有不同程度的影响。草地生态系统作为最重要、分布最广的陆地生态系统类型,在陆地碳循环中发挥着重要的作用。藏北地区草地面积巨大,具有海拔高、温度低、自然条件严酷的特点,是气候变化高敏感区域。小嵩草草甸生态系统是藏北地区主要的草地类型之一,相关研究数据显示该区域气候将在未来一段时间内呈暖湿化趋势,这必将对藏北小嵩草草甸生态系统结构和功能产生重大的影响。研究增温增雨对藏北小嵩草草甸生态系统碳交换的影响有助于确定在未来气候变化条件下草地生态系统碳储存能力,并明确气候变化对草地生态系统生产力、生物多样性的影响,有助于全方位监测草地生态系统对气候变化的响应机理。 本文以藏北地区小嵩草草甸草原为研究对象,利用开顶箱(Open top chamber, OTC)对草地进行被动增温,并结合生长季增加降雨措施模拟区域性气候变化对该地区的影响。试验包括四个处理,分别为对照(CK)、增温(Warming, W)、限制性增温(通过风扇控制OTC增温过高, LimitedWarming, LW)和限制性增温+增雨(添加20%的自然降雨, Limited Warming and PrecipitationEnhancement, LWP)。测定了增温增雨条件下小嵩草草甸土壤温湿度(-5cm、-15cm、-30cm)、空气温度(20cm)、草地生物量、生物多样性;系统的测定了小嵩草草甸碳交换各组分,包括生态系统净交换(Net ecosystem exchange, NEE)、生态系统呼吸(Ecosystem respiration, ER)、土壤呼吸(Soil respiration, SR)、蒸腾速率(Ecosystem evapotranspiration, ET)以及水分利用效率(Wateruse efficiency, WUE),分析了增温增雨条件下生态系统碳交换以及生产力和生物多样性的变化。主要结论如下: (1)生长季内,W处理5cm土壤温度增加1.02℃;W、LW和LWP平均气温分别增加1.91、1.53和1.37℃。LW和LWP处理以及W处理15cm以下土壤温度无显著变化。土壤湿度方面,OTC中5cm的土壤湿度降低,其中只有W处理达到显著性,OTC对15cm以下的土壤湿度无影响。 (2) OTC内物种地上生物量显著升高,W、LW和LWP处理生物量分别达到72.08、97.50和75.78g/m~2,显著高于对照处理(54.38g/m~2)。OTC中群落高度和盖度均有不同程度的提高,其中高度增加更为明显,W、LW和LWP处理群落高度分别增加78.3%、100.0%。和56.5%。物种重要值方面,禾本科植物早熟禾重要值在增温条件下显著升高,其他物种变化并不明显,可以认为早熟禾对增温的敏感性最高。增温条件下草地物种Shannon-Weiner多样性指数呈现增加的趋势,Simpson多样性指数和E.Pielou均匀度指数无显著变化。 (3)增温增雨对小嵩草草甸生态系统碳交换具有较强的促进作用。OTC内各处理GEP和ER均呈现出不同程度的升高,但GEP的升高幅度更大,因此小嵩草草甸NEE在增温条件下也得到了明显的促进。由于温度增加,小嵩草草甸生态系统蒸腾作用明显加强,这也导致了OTC内WUE明显降低,尤其在生长季初期的W和LW处理中较为明显。 (4)环境温湿度对生态系统碳交换包括NEE、GEP、ER影响显著,除了GEP与土壤温度,ER与土壤湿度无关外,其他指标均与土壤温湿度存在显著相关性,温湿度升高对碳交换具有促进作用。禾本科和莎草科植物生物量与各指标间也存在相关性,尤其是GEP与NEE,达到极显著水平(P 0.001),因此认为增温导致的光合产物增加量大多分配到了禾本科和莎草科植物中。 (5)增温增雨促进土壤呼吸增强,并且其相对增加幅度大于ER,使得SR在ER中的比例显著提高,生长季平均值最高达到51%,高于CK(36%)。与ER不同,SR与土壤水分密切相关,其温度敏感性低于ER。增温条件下ER和SR的土壤温度敏感性均呈现升高趋势。 (6)增温增雨条件下,生物量和生物多样性与气温之间存在线性相关,与土壤温湿度并无显著相关。生物量和生物多样性之间关系较为复杂,不同测度之间结果有所不同,物种多样性指数与生物量间的关系为单峰型,而均匀度指数的关系为U型。也从另一方面说明了禾本科和莎草科植物在小嵩草草甸生态系统中的主导地位在增温条件下进一步得到巩固。 增温增雨对藏北小嵩草草甸生态系统禾本科和莎草科植物的生长具有明显的促进作用。增温增雨条件下光合作用和呼吸作用增加的同时生态系统净吸收也显著升高,有助于小嵩草草甸生态系统碳储存,提高生态系统固碳能力。
[本文引用: 1]
DOI:10.1029/2007JD009173URL [本文引用: 1]

to characterize evapotranspiration (et) over grasslands on the qinghai-tibetan plateau, we examined et and its relevant environmental variables in a kobresia meadow from 2002 to 2004 using the eddy covariance method. the annual precipitation changed greatly, with 554, 706, and 666 mm a(-1) for the three consecutive calendar years. the annual et varied correspondingly to the annual precipitation with 341, 407, and 426 mm a(-1). the annual et was, however, constant at about 60% of the annual precipitation. about 85% annual et occurred during the growing season from may to september, and the averaged et for this period was 1.90, 2.23, and 2.22 mm/d, respectively for the three consecutive years. the averaged et was, however, very low (< 0.40 mm/d) during the nongrowing season from october to april. the annual canopy conductance (gc) and the priestley-taylor coefficient (a) showed the lowest values in the year with the lowest precipitation. this study first demonstrates that the alpine meadow ecosystem is characterized by a low ratio of annual et to precipitation and that the interannual variation of et is determined by annual precipitation.
DOI:10.1029/2003JD003584 [本文引用: 1]
DOI:10.1371/journal.pone.0075503URLPMID:24069425 [本文引用: 1]

Abstract The Tibetan Plateau (TP) is predicted to experience increases in air temperature, increases in snowfall, and decreases in monsoon rains; however, there is currently a paucity of data that examine the ecological responses to such climate changes. In this study, we examined the effects of increased air temperature and snowfall on: 1) water use partitioning by different plant functional groups, and 2) ecosystem CO2 fluxes throughout the growing season. At the individual plant scale, we used stable hydrogen isotopes ( D) to partition water use between shallow- and deep-rooted species. Prior to the arrival of summer precipitation (typically mid-July), snowmelt was the main water source in the soils. During this time, shallow and deep-rooted species partitioned water use by accessing water from shallow and deep soils, respectively. However, once the monsoon rains arrived, all plants used rainwater from the upper soils as the main water source. Snow addition did not result in increased snowmelt use throughout the growing season; instead, snowmelt water was pushed down into deeper soils when the rains arrived. At the larger plot scale, CO2 flux measurements demonstrated that rain was the main driver for net ecosystem productivity (NEP). NEP rates were low during June and July and reached a maximum during the monsoon season in August. Warming decreased NEP through a reduction in gross primary productivity (GPP), and snow additions did not mitigate the negative effects of warming by increasing NEP or GPP. Both the isotope and CO2 flux results suggest that rain drives productivity in the Nam Tso region on the TP. This also suggests that the effects of warming-induced drought on the TP may not be mitigated by increased snowfall. Further decreases in summer monsoon rains may affect ecosystem productivity, with large implications for livestock-based livelihoods.
DOI:10.1111/j.1744-7909.2007.00617.xURLPMID:18713359 [本文引用: 1]

From 30 June to 24 September in 2003 ecosystem respiration (Re) in two alpine meadows on the Tibetan Plateau were measured using static chamber- and gas chromatography- (GC) based techniques. Simultaneously, plant removal treatments were set to partition Re into plant autotrophic respiration (Ra) and microbial heterotrophic respiration (Rh). Results indicated that Re had clear diurnal and seasonal variation pattems in both of the meadows. The seasonal variability of Re at both meadow sites was caused mainly by changes in Ra, rather than Rh. Moreover, at the Kobresia humilis meadow site (K_site), Ra and Rh accounted for 54% and 46% of Re, respectively. While at the Potentilla fruticosa scrub meadow (P_site), the counterparts accounted for 61% and 39%, respectively. T test showed that there was significant difference in Re rates between the two meadows (t=2.387, P=0.022). However, no significant difference was found in Rh rates, whereas a significant difference was observed in Ra rates between the two meadows. Thus, the difference in Re rate between the two meadows was mainly attributed to plant autotrophic respirations. During the growing season, the two meadows showed relatively low Q10 values, suggesting that Re, especially Rh was not sensitive to temperature variation in the growing season. Additionally, Re and Rh at the K_site, as well as Rh at the P_site was negatively correlated with soil moisture, indicating that soil moisture would also play an important role in respirations.
DOI:10.1038/nature02561URLPMID:15190350 [本文引用: 1]

Abstract Water availability limits plant growth and production in almost all terrestrial ecosystems. However, biomes differ substantially in sensitivity of aboveground net primary production (ANPP) to between-year variation in precipitation. Average rain-use efficiency (RUE; ANPP/precipitation) also varies between biomes, supposedly because of differences in vegetation structure and/or biogeochemical constraints. Here we show that RUE decreases across biomes as mean annual precipitation increases. However, during the driest years at each site, there is convergence to a common maximum RUE (RUE(max)) that is typical of arid ecosystems. RUE(max) was also identified by experimentally altering the degree of limitation by water and other resources. Thus, in years when water is most limiting, deserts, grasslands and forests all exhibit the same rate of biomass production per unit rainfall, despite differences in physiognomy and site-level RUE. Global climate models predict increased between-year variability in precipitation, more frequent extreme drought events, and changes in temperature. Forecasts of future ecosystem behaviour should take into account this convergent feature of terrestrial biomes.
DOI:10.1093/aob/mci205URLPMID:4247020 [本文引用: 1]

Stress on plants imposed by flooding of the soil and deeper submergence constitutes one of the major abiotic constraints on growth, species' distribution and agricultural productivity. Flooding stress is also a strong driver of adaptive evolution. This has resulted in a wide range of biochemical, molecular and morphological adaptations that sanction growth and reproductive success under episodic or permanently flooded conditions that are highly damaging to the majority of plant species. However, even seemingly poorly adapted species possess some short-term resilience that is important for overall success of these plants in various habitats. The papers contained in this Special Issue address these topics and emphasize molecular, biochemical and developmental processes that impact on flooding tolerance. Most of the articles are based on lectures given to the 8th Conference of the International Society for Plant Anaerobiosis (ISPA), held at the University of Western Australia, Perth, 20-24 September, 2004. Reviews and research papers are presented from the leading laboratories currently working on plant responses to flooding stress.
DOI:10.1111/j.1365-2486.2005.00948.xURL [本文引用: 1]

Abstract Arid ecosystems, which occupy about 35% of the Earth's terrestrial surface area, are believed to be among the most responsive to elevated [CO 2 ]. Net ecosystem CO 2 exchange (NEE) was measured in the eighth year of CO 2 enrichment at the Nevada Desert Free-Air CO 2 Enrichment (FACE) Facility between the months of December 2003–December 2004. On most dates mean daily NEE (24h) (μmolCO 2 m 612 s 611 ) of ecosystems exposed to elevated atmospheric CO 2 were similar to those maintained at current ambient CO 2 levels. However, on sampling dates following rains, mean daily NEEs of ecosystems exposed to elevated [CO 2 ] averaged 23 to 56% lower than mean daily NEEs of ecosystems maintained at ambient [CO 2 ]. Mean daily NEE varied seasonally across both CO 2 treatments, increasing from about 0.1μmolCO 2 m 612 s 611 in December to a maximum of 0.5–0.6μmolCO 2 m 612 s 611 in early spring. Maximum NEE in ecosystems exposed to elevated CO 2 occurred 1 month earlier than it did in ecosystems exposed to ambient CO 2 , with declines in both treatments to lowest seasonal levels by early October (0.09±0.03μmolCO 2 m 612 s 611 ), but then increasing to near peak levels in late October (0.36±0.08μmolCO 2 m 612 s 611 ), November (0.28±0.03μmolCO 2 m 612 s 611 ), and December (0.54±0.06μmolCO 2 m 612 s 611 ). Seasonal patterns of mean daily NEE primarily resulted from larger seasonal fluctuations in rates of daytime net ecosystem CO 2 uptake which were closely tied to plant community phenology and precipitation. Photosynthesis in the autotrophic crust community (lichens, mosses, and free-living cyanobacteria) following rains were probably responsible for the high NEEs observed in January, February, and late October 2004 when vascular plant photosynthesis was low. Both CO 2 treatments were net CO 2 sinks in 2004, but exposure to elevated CO 2 reduced CO 2 sink strength by 30% (positive net ecosystem productivity=127±17gCm 612 yr 611 ambient CO 2 and 90±11gCm 612 yr 611 elevated CO 2 , P =0.011). This level of net C uptake rivals or exceeds levels observed in some forested and grassland ecosystems. Thus, the decrease in C sequestration seen in our study under elevated CO 2 – along with the extensive coverage of arid and semi-arid ecosystems globally – points to a significant drop in global C sequestration potential in the next several decades because of responses of heretofore overlooked dryland ecosystems.
DOI:10.1137/040614359URL [本文引用: 1]

Three years of eddy covariance measurements were used to characterize the seasonal and interannual variability of the CO2 fluxes above an alpine meadow (3250 m a.s.l.) on the Qinghai-Tibetan Plateau, China. This alpine meadow was a weak sink for atmospheric CO2, with a net ecosystem production (NEP) of 78.5, 91.7, and 192.5 g C m(-2) yr(-1) in 2002, 2003, and 2004, respectively. The prominent, high NEP in 2004 resulted from the combination of high gross primary production (GPP) and low ecosystem respiration (R-e) during the growing season. The period of net absorption of CO2 in 2004, 179 days, was 10 days longer than that in 2002 and 5 days longer than that in 2003. Moreover, the date on which the mean air temperature first exceeded 5.0 degrees C was 10 days earlier in 2004 (DOY110) than in 2002 or 2003. This date agrees well with that on which the green aboveground biomass (Green AGB) started to increase. The relationship between light-use efficiency and Green AGB was similar among the three years. In 2002, however, earlier senescence possibly caused low autumn GPP, and thus the annual NEP, to be lower. The low summertime R-e in 2004 was apparently caused by lower soil temperatures and the relatively lower temperature dependence of R-e in comparison with the other years. These results suggest that (1) the Qinghai-Tibetan Plateau plays a potentially significant role in global carbon sequestration, because alpine meadow covers about one-third of this vast plateau, and (2) the annual NEP in the alpine meadow was comprehensively controlled by the temperature environment, including its effect on biomass growth.
DOI:10.1657/1938-4246-42.4.449URL [本文引用: 1]

In this study, we apply temperature, precipitation, and other data from 66 Chinese meteorological stations including Xining and Lhasa to analyze the extreme climate events and their impacting factors over the Qinghai-Tibet Plateau during the period 1961-2007. We focus on the spatial and temporal features of extreme climate events and their long-term changes over five climate zones of alpine grassland, meadow, and desert areas. Results show that, during the past decades, the changes in climate over the Qinghai-Tibet Plateau present trends towards warm and wet conditions. These changes in temperature and precipitation are evident in both seasonal means and extreme events, and the changes in precipitation are apparent in both precipitation amount and number of precipitation days. Clearly, warm and wet events increase, but cold and dry events decrease over the plateau region. Features of the warming climate are relatively consistent in spatial and seasonal distributions, with the most significant changes in winter and autumn and at nighttime. Northern Qinghai exhibits the greatest and most significant decrease in the frequency of extremely low-temperature events. However, the wetting trend shows more distinctive spatial features and is more seasonally dependent. While the trends in both precipitation amount and the number of precipitation days are positive in all climate zones for winter and spring, both positive and insignificant negative trends appear in summer and autumn. The largest decrease in the frequency of severely dry events is found over southeastern Tibet and western Sichuan.
URL [本文引用: 1]
URL [本文引用: 1]
DOI:10.1016/j.agrformet.2011.04.015URL [本文引用: 1]

Seven years of continuous eddy covariance measurements at an alpine meadow were used to investigate the impacts of climate drivers and ecosystem responses on the inter-annual variability (IAV) of the net ecosystem exchange (NEE). The annual cumulative value of NEE was positive (source) in 2003, 2005 and 2009 (50, 15 and 112 g m 612 respectively) and negative (sink) in 2004, 2006, 2007 and 2008 (29, 75, 110 and 28 g m 612 respectively). The IAV of carbon dioxide fluxes builds up in two phenological phases: the onset of the growing season (triggered by snow melting) and the canopy re-growth after mowing. Respiratory fluxes during the non-growing season were observed to increase IAV, while growing season uptake dampened it. A novel approach was applied to factor out the two main sources of IAV: climate drivers’ variability and changes in the ecosystem responses to climate. Annual values of carbon dioxide fluxes were calculated assuming (a) variable climate and variable ecosystem response among years, (b) variable climate and constant ecosystem response and (c) constant climate and variable ecosystem response. The analysis of flux variances calculated under these three assumptions indicates the occurrence of an important negative feedback between climate and ecosystem responses. Due to this feedback, the observed IAV of NEE is lower than one would expect for a given climate variability, because of the counteracting changes in ecosystem responses. This alpine meadow therefore demonstrates the ability to acclimatise and to limit the IAV of carbon fluxes induced by climate variability.
DOI:10.1006/jare.2001.0902URL [本文引用: 1]

Carbon storage in grasslands of China was estimated by the carbon density method and based on a nationwide grassland resource survey finished by 1991. The grasslands in China were classified into 18 types, which are distributed mostly in the temperate region and on the Tibetan Plateau, and scattered in the warm-temperate and tropical regions. Based on the median estimate, vegetation, soil and total carbon storage of grasslands in China were 3·06, 41·03 and 44·09 Pg C, respectively. Vegetation had low carbon storage and most carbon was stored in soils. Of the four types of regions that have grasslands, alpine region (54·5%) and temperate region (31·6%) hold more than 85% of the total grassland carbon (in both vegetation and soils) in China. Considering specific types within these two regions, three grassland types, alpine meadow (25·6%), alpine steppe (14·5%) and temperate steppe (11%) constituted more than half of all carbon stored in China's grasslands. In general and regardless of regional vegetation types, steppes (38·6%) and meadows (38·2%) made up more than 2/3 of total grassland carbon. The carbon storage in alpine grasslands may have a significant and long-lived effect on global C cycles. This study estimated more carbon storage in vegetation and less in soils than previous studies. The differences of grassland carbon between this study and two previous studies were due probably to four reasons, i.e. different estimation methods, different classification systems of grasslands, different areas of grasslands, and different carbon densities. China's grasslands cover only 6–8% of total world grassland area and have 9–16% of total carbon in the world grasslands. They make a big contribution to the world carbon storage and may have significant effects on carbon cycles, both in global and in arid lands.
DOI:10.1890/0012-9658(2001)082[1387:EOFOSP]2.0.CO;2URL [本文引用: 2]

Changes in precipitation and nitrogen (N) deposition can influence ecosystem carbon (C) cycling and budget in terrestrial biomes, with consequent feedbacks to climate change. However, little is known about the main and interactive effects of water and N additions on net ecosystem C exchange (NEE). In a temperate steppe of northern China, a field-manipulated experiment was conducted to evaluate the responses of NEE and its components to improve N and water availability from 2005 to 2008. The results showed that both water and N additions stimulated gross ecosystem productivity (GEP), ecosystem respiration (ER), and NEE. Water addition increased GEP by 17%, ER by 24%, and NEE by 11% during the experimental period, whereas N addition increased GEP by 17%, ER by 16%, and NEE by 19%. The main effects of both water and N additions changed with time, with the strongest water stimulation in the dry year and a diminishing N stimulation over time. When water and N were added in combination, there were non-additive effects of water and N on ecosystem C fluxes, which could be explained by the changes in species composition and the shifts of limiting resources from belowground (water or N) to aboveground (light). The positive water and N additions effects indicate that increasing precipitation and N deposition in the future will favor C sequestration in the temperate steppe. The non-additive effects of water and N on ecosystem C fluxes suggest that multifactor experiments are better able to capture complex interactive processes, thus improving model simulations and projections.
DOI:10.1038/nature09364URLPMID:20811450 [本文引用: 1]

Abstract China is the world's most populous country and a major emitter of greenhouse gases. Consequently, much research has focused on China's influence on climate change but somewhat less has been written about the impact of climate change on China. China experienced explosive economic growth in recent decades, but with only 7% of the world's arable land available to feed 22% of the world's population, China's economy may be vulnerable to climate change itself. We find, however, that notwithstanding the clear warming that has occurred in China in recent decades, current understanding does not allow a clear assessment of the impact of anthropogenic climate change on China's water resources and agriculture and therefore China's ability to feed its people. To reach a more definitive conclusion, future work must improve regional climate simulations-especially of precipitation-and develop a better understanding of the managed and unmanaged responses of crops to changes in climate, diseases, pests and atmospheric constituents.
DOI:10.1038/nature12350URLPMID:23955228 [本文引用: 1]

Abstract The terrestrial biosphere is a key component of the global carbon cycle and its carbon balance is strongly influenced by climate. Continuing environmental changes are thought to increase global terrestrial carbon uptake. But evidence is mounting that climate extremes such as droughts or storms can lead to a decrease in regional ecosystem carbon stocks and therefore have the potential to negate an expected increase in terrestrial carbon uptake. Here we explore the mechanisms and impacts of climate extremes on the terrestrial carbon cycle, and propose a pathway to improve our understanding of present and future impacts of climate extremes on the terrestrial carbon budget.
DOI:10.1016/j.apsoil.2015.01.015URL [本文引用: 1]

A warming and precipitation pulses experiment was conducted in an alpine meadow of Tibet over the growing season (June鈥揝eptember) in 2013. Soil respiration ( R s ) was measured biweekly from early July to early September 2013. The effect of experimental warming on R s varied with water availability. The effect of precipitation pulses on R s depended on pulses sizes, antecedent precipitation and soil moisture conditions. Precipitation pulses increased the temperature sensitivity of R s , which was not affected by experimental warming. Our findings suggest that water availability regulates the response of soil respiration to warming.
DOI:10.2136/sssaj1990.03615995005400060018xURL [本文引用: 1]
DOI:10.1016/j.agrformet.2011.01.018URL [本文引用: 1]

Drought as an intermittent disturbance of the water cycle interacts with the carbon cycle differently than the ‘gradual’ climate change. During drought plants respond physiologically and structurally to prevent excessive water loss according to species-specific water use strategies. This has consequences for carbon uptake by photosynthesis and release by total ecosystem respiration. After a drought the disturbances in the reservoirs of moisture, organic matter and nutrients in the soil and carbohydrates in plants lead to longer-term effects in plant carbon cycling, and potentially mortality. Direct and carry-over effects, mortality and consequently species competition in response to drought are strongly related to the survival strategies of species. Here we review the state of the art of the understanding of the relation between soil moisture drought and the interactions with the carbon cycle of the terrestrial ecosystems. We argue that plant strategies must be given an adequate role in global vegetation models if the effects of drought on the carbon cycle are to be described in a way that justifies the interacting processes.
DOI:10.1111/j.1365-2486.2007.01433.xURL [本文引用: 2]

Abstract Responses of soil respiration to atmospheric and climatic change will have profound impacts on ecosystem and global carbon (C) cycling in the future. This study was conducted to examine effects on soil respiration of the concurrent driving factors of elevated atmospheric CO 2 concentration, air warming, and changing precipitation in a constructed old-field grassland in eastern Tennessee, USA. Model ecosystems of seven old-field species were established in open-top chambers and treated with factorial combinations of ambient or elevated (+300ppm) CO 2 concentration, ambient or elevated (+3 C) air temperature, and high or low soil moisture content. During the 19-month experimental period from June 2003 to December 2004, higher CO 2 concentration and soil water availability significantly increased mean soil respiration by 35.8% and 15.7%, respectively. The effects of air warming on soil respiration varied seasonally from small reductions to significant increases to no response, and there was no significant main effect. In the wet side of elevated CO 2 chambers, air warming consistently caused increases in soil respiration, whereas in the other three combinations of CO 2 and water treatments, warming tended to decrease soil respiration over the growing season but increase it over the winter. There were no interactive effects on soil respiration among any two or three treatment factors irrespective of time period. Treatment-induced changes in soil temperature and moisture together explained 49%, 44%, and 56% of the seasonal variations of soil respiration responses to elevated CO 2 , air warming, and changing precipitation, respectively. Additional indirect effects of seasonal dynamics and responses of plant growth on C substrate supply were indicated. Given the importance of indirect effects of the forcing factors and plant community dynamics on soil temperature, moisture, and C substrate, soil respiration response to climatic warming should not be represented in models as a simple temperature response function, and a more mechanistic representation including vegetation dynamics and substrate supply is needed.
[本文引用: 1]
DOI:10.1641/0006-3568(2003)053[0941:ATROTE]2.0.CO;2URL [本文引用: 1]

Changes in Earth's surface temperatures caused by anthropogenic emissions of greenhouse gases are expected to affect global and regional precipitation regimes. Interactions between changing precipitation regimes and other aspects of global change are likely to affect natural and managed terrestrial ecosystems as well as human society. Although much recent research has focused on assessing the responses of terrestrial ecosystems to rising carbon dioxide or temperature, relatively little research has focused on understanding how ecosystems respond to changes in precipitation regimes. Here we review predicted changes in global and regional precipitation regimes, outline the consequences of precipitation change for natural ecosystems and human activities, and discuss approaches to improving understanding of ecosystem responses to changing precipitation. Further, we introduce the Precipitation and Ecosystem Change Research Network (PrecipNet), a new interdisciplinary research network assembled to encourage and foster communication and collaboration across research groups with common interests in the impacts of global change on precipitation regimes, ecosystem structure and function, and the human enterprise. [References: 60]
DOI:10.4155/cmt.13.54URL [本文引用: 1]
DOI:10.1111/j.1365-2486.2010.02302.xURL [本文引用: 2]

Abstract Global mean temperature is predicted to increase by 2 7 C and precipitation to change across the globe by the end of this century. To quantify climate effects on ecosystem processes, a number of climate change experiments have been established around the world in various ecosystems. Despite these efforts, general responses of terrestrial ecosystems to changes in temperature and precipitation, and especially to their combined effects, remain unclear. We used meta-analysis to synthesize ecosystem-level responses to warming, altered precipitation, and their combination. We focused on plant growth and ecosystem carbon (C) balance, including biomass, net primary production (NPP), respiration, net ecosystem exchange (NEE), and ecosystem photosynthesis, synthesizing results from 85 studies. We found that experimental warming and increased precipitation generally stimulated plant growth and ecosystem C fluxes, whereas decreased precipitation had the opposite effects. For example, warming significantly stimulated total NPP, increased ecosystem photosynthesis, and ecosystem respiration. Experimentally reduced precipitation suppressed aboveground NPP (ANPP) and NEE, whereas supplemental precipitation enhanced ANPP and NEE. Plant productivity and ecosystem C fluxes generally showed higher sensitivities to increased precipitation than to decreased precipitation. Interactive effects of warming and altered precipitation tended to be smaller than expected from additive, single-factor effects, though low statistical power limits the strength of these conclusions. New experiments with combined temperature and precipitation manipulations are needed to conclusively determine the importance of temperature recipitation interactions on the C balance of terrestrial ecosystems under future climate conditions.
DOI:10.3321/j.issn:1000-0933.2005.08.017URL [本文引用: 1]

高寒草甸是青藏高原广泛分布的植被类型之一,面积约120万km2,地处青藏高原腹地的当雄草原站即位于 该类植被的典型分布区.以2003年8~10月中旬在该站用涡度相关法连续观测的CO2通量数据资料为基础,分析了高寒草甸生态系统8~10月份净二氧化 碳交换量(NEE)的日变化规律,及其与光合有效辐射、降水、温度等环境因子之间的关系.结果表明,8~10月份的日均NEE有明显的日变化,表现为单峰 型,通常在地方时11:00~12:00左右达到碳吸收的最大值,平均为-0.2680 mg CO2/(m2·s)(-6.0800μmol CO2/(m2·s)).白天的NEE与光合有效辐射之间符合很好的直角双曲线关系,表观量子产额平均为0.0203μmol CO2/μmol PAR,表观最大光合速率平均为 9.7411 μmolCO2/(m2·s).夜晚的NEE与5cm地温有很好的指数函数关系.
DOI:10.3321/j.issn:1000-0933.2005.08.017URL [本文引用: 1]

高寒草甸是青藏高原广泛分布的植被类型之一,面积约120万km2,地处青藏高原腹地的当雄草原站即位于 该类植被的典型分布区.以2003年8~10月中旬在该站用涡度相关法连续观测的CO2通量数据资料为基础,分析了高寒草甸生态系统8~10月份净二氧化 碳交换量(NEE)的日变化规律,及其与光合有效辐射、降水、温度等环境因子之间的关系.结果表明,8~10月份的日均NEE有明显的日变化,表现为单峰 型,通常在地方时11:00~12:00左右达到碳吸收的最大值,平均为-0.2680 mg CO2/(m2·s)(-6.0800μmol CO2/(m2·s)).白天的NEE与光合有效辐射之间符合很好的直角双曲线关系,表观量子产额平均为0.0203μmol CO2/μmol PAR,表观最大光合速率平均为 9.7411 μmolCO2/(m2·s).夜晚的NEE与5cm地温有很好的指数函数关系.
DOI:10.1186/1471-2229-11-21URLPMID:3037845 [本文引用: 2]

Background Changing water condition represents a dramatic impact on global terrestrial ecosystem productivity, mainly by limiting plant functions, including growth and photosynthesis, particularly in...
DOI:10.1016/j.jclepro.2015.04.140URL [本文引用: 1]

Grasslands in Tibetan Plateau play an important role in carbon emission reduction. Accurately evaluating the carbon budget of alpine grassland ecosystems is of great importance. Based on the parameterization and validation of a process-based ecosystem model (Terrestrial Ecosystem Model, TEM5.0), we analyze temporal and spatial dynamics and patterns of grassland ecosystem carbon emission and sequestration of Tibetan Plateau in China from 1961 to 2010. Alpine grasslands act as a carbon sink with mean annual value of 10.12TgCyr 611 during the past 50 years. The alpine meadow contributes most to the sink at 9.04TgCyr 611 , while the alpine steppe only contributes 2.03TgCyr 611 . 83.7% of the total area trends to be carbon sink and only 0.2% of the region showed no significant trend. Furthermore, the annual net ecosystem productivity shows significantly positive relationship with increasing temperature and atmosphere CO 2 concentration, but it shows no significant relationship with precipitation. Alpine grasslands in Qinghai-Tibetan Plateau is a carbon sink and sequestrate totally 520TgC from 1961 to 2010. The carbon budget of alpine experienced dramatically spatial and temporal dynamics during past 50 years. Inter-annual variability of the net ecosystem productivity mainly depends on different sensitivities of net primary productivity and heterotrophic respiration to temperature and precipitation variability. Temperature and atmosphere CO 2 concentration are main driving forces of carbon budget dynamics of grassland ecosystems in Qinghai-Tibetan Plateau during 1961–2010.
[本文引用: 1]
[本文引用: 1]
DOI:10.1007/s12665-010-0716-yURL [本文引用: 1]

Permafrost degradation has the potential to significantly change soil moisture. The objective of this study was to assess the variability of soil moisture in a permafrost region using geostatistical techniques. The experiment was conducted in August 2008 in alpine steppe and meadow located in the Qinghai-Tibetan Plateau permafrost region. Four soil depths (0–10, 10–20, 20–30 and 30–4002cm) were analyzed using frequency domain reflectometry, and sampling made of 80 points in a 1002m02×021002m grid were sampled. Soil moisture was analyzed using classical statistics to appropriately describe central tendency and dispersion, and then using geostatistics to describe spatial variability. Classical statistical method indicated that soil moisture in the permafrost region had a normal distribution pattern. Mean surface soil moisture in alpine meadow was higher than that in alpine steppe. The semivariograms showed that soil moisture variability in alpine cold steppe was larger than that in alpine meadow, which decreased with depths. Nugget values in alpine steppe were low (0.1–4.5), in contrast to alpine cold meadow. Soil moisture in alpine steppe had highly structured spatial variability with more than 93.4% spatial heterogeneity, and the range decreased with depth. Soil moisture content in alpine cold meadow had a moderate spatial dependence with a range of 51.3–169.202m, increasing with depth.
DOI:10.1111/gcb.12079URLPMID:23504837 [本文引用: 1]

Understanding the dynamics and underlying mechanism of carbon exchange between terrestrial ecosystems and the atmosphere is one of the key issues in global change research. In this study, we quantified the carbon fluxes in different terrestrial ecosystems in China, and analyzed their spatial variation and environmental drivers based on the long-term observation data of ChinaFLUX sites and the published data from other flux sites in China. The results indicate that gross ecosystem productivity (GEP), ecosystem respiration (ER), and net ecosystem productivity (NEP) of terrestrial ecosystems in China showed a significantly latitudinal pattern, declining linearly with the increase of latitude. However, GEP, ER, and NEP did not present a clear longitudinal pattern. The carbon sink functional areas of terrestrial ecosystems in China were mainly located in the subtropical and temperate forests, coastal wetlands in eastern China, the temperate meadow steppe in the northeast China, and the alpine meadow in eastern edge of Qinghai-Tibetan Plateau. The forest ecosystems had stronger carbon sink than grassland ecosystems. The spatial patterns of GEP and ER in China were mainly determined by mean annual precipitation (MAP) and mean annual temperature (MAT), whereas the spatial variation in NEP was largely explained by MAT. The combined effects of MAT and MAP explained 79%, 62%, and 66% of the spatial variations in GEP, ER, and NEP, respectively. The GEP, ER, and NEP in different ecosystems in China exhibited ositive coupling correlation in their spatial patterns. Both ER and NEP were significantly correlated with GEP, with 68% of the per-unit GEP contributed to ER and 29% to NEP. MAT and MAP affected the spatial patterns of ER and NEP mainly by their direct effects on the spatial pattern of GEP.
[本文引用: 1]
[本文引用: 1]
DOI:10.1360/n972014-01296URL [本文引用: 1]

本文回顾了21世纪青藏高原区域多种气候和环境要素变化预估研究的进展,包括气温、降水、极端天气气候事件、冻土、积雪、冰川、径流和植被等,预估结果主要来自于SRES和RCP情景下气候模式的预估以及物理统计模型的预估.结果表明,未来青藏高原地面气温将升高,21世纪后期增温更显著.总体来说21世纪高原降水以增加为主,极端天气气候事件增加.高原未来冻土面积缩小,冻土活动层厚度增加,积雪日数和积雪深度减少,冰川将以退缩为主.径流的未来变化较复杂,不同流域之间的差异较大,径流在不同流域表现为增加和减少并存.青藏高原植被对气候变化的响应敏感而脆弱,21世纪中后期青藏高原的生长季长度增加,常绿林/森林出现在高原东部和南部,灌丛植被类型将会扩展并入侵高寒草原.根据已有的研究结果,本文对这些气候与环境要素在21世纪中期(2030~2050年)和后期(2080~2100年)的变化进行了综合集成,给出了它们在21世纪中期和后期的可能变化范围.
DOI:10.1360/n972014-01296URL [本文引用: 1]

本文回顾了21世纪青藏高原区域多种气候和环境要素变化预估研究的进展,包括气温、降水、极端天气气候事件、冻土、积雪、冰川、径流和植被等,预估结果主要来自于SRES和RCP情景下气候模式的预估以及物理统计模型的预估.结果表明,未来青藏高原地面气温将升高,21世纪后期增温更显著.总体来说21世纪高原降水以增加为主,极端天气气候事件增加.高原未来冻土面积缩小,冻土活动层厚度增加,积雪日数和积雪深度减少,冰川将以退缩为主.径流的未来变化较复杂,不同流域之间的差异较大,径流在不同流域表现为增加和减少并存.青藏高原植被对气候变化的响应敏感而脆弱,21世纪中后期青藏高原的生长季长度增加,常绿林/森林出现在高原东部和南部,灌丛植被类型将会扩展并入侵高寒草原.根据已有的研究结果,本文对这些气候与环境要素在21世纪中期(2030~2050年)和后期(2080~2100年)的变化进行了综合集成,给出了它们在21世纪中期和后期的可能变化范围.
DOI:10.3321/j.issn:1000-0585.2002.01.001URL [本文引用: 1]

长期以来 ,种种因素导致****们对青藏高原确切范围的认识和理解存在差异。根据青藏高原相关领域研究的新成果和多年野外实践 ,从地理学角度 ,充分讨论了确定青藏高原范围和界线的原则与涉及的问题 ,结合信息技术方法对青藏高原范围与界线位置进行了精确的定位和定量分析。得出 :青藏高原在中国境内部分西起帕米尔高原 ,东至横断山脉 ,横跨 31个经度 ,东西长约 2 94 5km ;南自喜马拉雅山脉南缘 ,北迄昆仑山 -祁连山北侧 ,纵贯约 13个纬度 ,南北宽达 15 32km ;范围为 2 6°0 0′12″N~ 39°4 6′5 0″N ,73°18′5 2″E~ 10 4°4 6′5 9″E ,面积为 2 5 72 4× 10 3km2 ,占我国陆地总面积的 2 6 8%。
DOI:10.3321/j.issn:1000-0585.2002.01.001URL [本文引用: 1]

长期以来 ,种种因素导致****们对青藏高原确切范围的认识和理解存在差异。根据青藏高原相关领域研究的新成果和多年野外实践 ,从地理学角度 ,充分讨论了确定青藏高原范围和界线的原则与涉及的问题 ,结合信息技术方法对青藏高原范围与界线位置进行了精确的定位和定量分析。得出 :青藏高原在中国境内部分西起帕米尔高原 ,东至横断山脉 ,横跨 31个经度 ,东西长约 2 94 5km ;南自喜马拉雅山脉南缘 ,北迄昆仑山 -祁连山北侧 ,纵贯约 13个纬度 ,南北宽达 15 32km ;范围为 2 6°0 0′12″N~ 39°4 6′5 0″N ,73°18′5 2″E~ 10 4°4 6′5 9″E ,面积为 2 5 72 4× 10 3km2 ,占我国陆地总面积的 2 6 8%。
DOI:10.1111/j.1365-2486.2006.01197.xURL [本文引用: 1]

Abstract Thus far, grassland ecosystem research has mainly been focused on low-lying grassland areas, whereas research on high-altitude grassland areas, especially on the carbon budget of remote areas like the Qinghai-Tibetan plateau is insufficient. To address this issue, flux of CO 2 were measured over an alpine shrubland ecosystem (37°36′N, 101°18′E; 325 above sea level [a. s. l.]) on the Qinghai-Tibetan Plateau, China, for 2 years (2003 and 2004) with the eddy covariance method. The vegetation is dominated by formation Potentilla fruticosa L. The soil is Mol–Cryic Cambisols. To interpret the biotic and abiotic factors that modulate CO 2 flux over the course of a year we decomposed net ecosystem CO 2 exchange (NEE) into its constituent components, and ecosystem respiration ( R eco ). Results showed that seasonal trends of annual total biomass and NEE followed closely the change in leaf area index. Integrated NEE were 6158.5 and 6175.5 g Cm 612 , respectively, for the 2003 and 2004 years. Carbon uptake was mainly attributed from June, July, August, and September of the growing season. In July, NEE reached seasonal peaks of similar magnitude (4–5gCm 612 day 611 ) each of the 2 years. Also, the integrated night-time NEE reached comparable peak values (1.5–2gCm 612 day 611 ) in the 2 years of study. Despite the large difference in time between carbon uptake and release (carbon uptake time < release time), the alpine shrubland was carbon sink. This is probably because the ecosystem respiration at our site was confined significantly by low temperature and small biomass and large day/night temperature difference and usually soil moisture was not limiting factor for carbon uptake. In general, R eco was an exponential function of soil temperature, but with season-dependent values of Q 10 . The temperature-dependent respiration model failed immediately after rain events, when large pulses of R eco were observed. Thus, for this alpine shrubland in Qinghai-Tibetan plateau, the timing of rain events had more impact than the total amount of precipitation on ecosystem R eco and NEE.
[本文引用: 1]
DOI:10.1371/journal.pone.0092531URLPMID:3960259 [本文引用: 1]

The temperature sensitivity (Q10) of soil heterotrophic respiration (Rh) is an important ecological model parameter and may vary with temperature and moisture. While Q10 generally decreases with increasing temperature, the moisture effects on Q10 have been controversial. To address this, we conducted a 90-day laboratory incubation experiment using a subtropical forest soil with a full factorial combination of five moisture levels (20%, 40%, 60%, 80%, and 100% water holding capacity - WHC) and five temperature levels (10, 17, 24, 31, and 38 C). Under each moisture treatment, Rh was measured several times for each temperature treatment to derive Q10 based on the exponential relationships between Rh and temperature. Microbial biomass carbon (MBC), microbial community structure and soil nutrients were also measured several times to detect their potential contributions to the moisture-induced Q10 variation. We found that Q10 was significantly lower at lower moisture levels (60%, 40% and 20% WHC) than at higher moisture level (80% WHC) during the early stage of the incubation, but became significantly higher at 20%WHC than at 60% WHC and not significantly different from the other three moisture levels during the late stage of incubation. In contrast, soil Rh had the highest value at 60% WHC and the lowest at 20% WHC throughout the whole incubation period. Variations of Q10 were significantly associated with MBC during the early stages of incubation, but with the fungi-to-bacteria ratio during the later stages, suggesting that changes in microbial biomass and community structure are related to the moisture-induced Q10 changes. This study implies that global warming impacts on soil CO2 emission may depend upon soil moisture conditions. With the same temperature rise, wetter soils may emit more CO2 into the atmosphere via heterotrophic respiration.
DOI:10.1016/j.ecolind.2015.11.028URL [本文引用: 1]

Analyzing the approaches that climatic factors affect the spatial variation of annual gross primary productivity (GPPyr) would improve our understanding on its spatial pattern. Based on network eddy covariance measurements and published data in literature, we separated GPPyrinto radiation use efficiency (RUE) and annual absorbed photosynthesis active radiation (APARyr), where APARyrcan be regarded as the product of the fraction of absorbed annual photosynthesis active radiation (FPARyr) and annual PAR (PARyr). Given that PARyraffects the spatial variation of GPPyrdirectly through itself, we investigated factors affecting the spatial variations of RUE and FPARyr, to reveal how climatic factors affect the spatial variation of GPPyr. Results suggest that the spatial variation of RUE was directly affected by annual mean air temperature (MAT) and annual mean CO2mass concentration ( cyr). The increasing MAT and cyrdirectly enhanced RUE. The increasing annual precipitation (MAP) directly prompted FPARyr. Therefore, MAT and cyraffected the spatial variation of GPPyrthrough altering RUE while the effect of MAP was achieved through altering FPARyr. Our study could also provide an alternative way for regional GPPyrassessment.
[本文引用: 1]
DOI:10.1002/gbc.20057URL [本文引用: 1]

Soil consumption of atmospheric methane plays an important secondary role in regulating the atmospheric CH4 budget, next to the dominant loss mechanism involving reaction with the hydroxyl radical (OH). Here we used a process-based biogeochemistry model to quantify soil consumption during the 20th and 21st centuries. We estimated that global soils consumed 32–36 Tg CH4 yr611 during the 1990s. Natural ecosystems accounted for 84% of the total consumption, and agricultural ecosystems only consumed 5 Tg CH4 yr611 in our estimations. During the twentieth century, the consumption rates increased at 0.03–0.20 Tg CH4 yr612 with seasonal amplitudes increasing from 1.44 to 3.13 Tg CH4 month611. Deserts, shrublands, and xeric woodlands were the largest sinks. Atmospheric CH4 concentrations and soil moisture exerted significant effects on the soil consumption while nitrogen deposition had a moderate effect. During the 21st century, the consumption is predicted to increase at 0.05-1.0 Tg CH4 yr612, and total consumption will reach 45–140 Tg CH4 yr611 at the end of the 2090s, varying under different future climate scenarios. Dry areas will persist as sinks, boreal ecosystems will become stronger sinks, mainly due to increasing soil temperatures. Nitrogen deposition will modestly reduce the future sink strength at the global scale. When we incorporated the estimated global soil consumption into our chemical transport model simulations, we found that nitrogen deposition suppressed the total methane sink by 26 Tg during the period 1998–2004, resulting in 6.6 ppb higher atmospheric CH4 mixing ratios compared to without considering nitrogen deposition effects. On average, a cumulative increase of every 1 Tg soil CH4 consumption decreased atmospheric CH4 mixing ratios by 0.26 ppb during the period 1998–2004.
Water pulses and biogeochemical cycles in arid and semiarid ecosystems
1
2004
... id="C31">降水变化会直接或间接地对植物生长和微生物过程造成影响, 继而影响ER (
Increased temperature and precipitation interact to affect root production, mortality, and turnover in a temperate steppe: Implications for ecosystem C cycling
1
2010
... id="C30">中国大部分陆地生态系统的观测研究表明, 年降水量变化会对GEP造成显著影响(
Precipitation manipulation experiments—Challenges and recommendations for the future
2
2012
... id="C33">准确了解降水变化对生态系统的影响需要建立更加科学合理的实验设计, 这需要考虑包括降水的频率、大小以及与其他因素的相互作用等(
... 、3B).已有的研究通常采用一个增水标准(
Dependence of carbon sequestration on the differential responses of ecosystem photosynthesis and respiration to rain pulses in a semiarid steppe
1
2009
... id="C33">准确了解降水变化对生态系统的影响需要建立更加科学合理的实验设计, 这需要考虑包括降水的频率、大小以及与其他因素的相互作用等(
Modeling the soil consumption of atmospheric methane at the global scale
1
2007
... id="C31">降水变化会直接或间接地对植物生长和微生物过程造成影响, 继而影响ER (
Response of plant biomass and soil respiration to experimental warming and precipitation manipulation in a Northern Great Plains grassland
1
2013
... id="C33">准确了解降水变化对生态系统的影响需要建立更加科学合理的实验设计, 这需要考虑包括降水的频率、大小以及与其他因素的相互作用等(
Seasonal and interannual variation in carbon dioxide exchange and carbon balance in a northern temperate grassland
1
2002
... id="C19">Flux表示NEE和ER的通量(μmol·m-2·s-1); V是箱内所包含的气室体积(m3); P是观测时箱内平均大气压(kPa); W是观测时箱内平均水汽分压(mmol·mol-1); R为标准气体常数(8.314 J·mol-1·K-1); S是底座面积(m2); T是观测期间箱内平均气温(℃); C?是经过水汽校正的CO2浓度, C? = C/(1 - W/1000), C是红外分析仪直接观测时记录的箱内CO2浓度(μmol·mol-1); dC?/dt是箱内CO2浓度随时间变化的速率.GEP的计算公式为GEP = -NEE + ER (
Environmental influences on carbon dioxide fluxes over three grassland ecosystems in China
1
2009
... id="C8">高寒草甸生态系统是青藏高原重要的草地生态系统类型, 约占青藏高原总面积的35% (
增温增雨对藏北小嵩草草甸生态系统碳交换的影响
1
2013
... id="C8">高寒草甸生态系统是青藏高原重要的草地生态系统类型, 约占青藏高原总面积的35% (
增温增雨对藏北小嵩草草甸生态系统碳交换的影响
1
2013
... id="C8">高寒草甸生态系统是青藏高原重要的草地生态系统类型, 约占青藏高原总面积的35% (
Dynamics of alpine grassland
1
2009
... id="C7">青藏高原被称作“地球第三极”, 平均海拔4 000 m以上, 面积约257万km2, 占中国陆地面积的26.8%左右(
Characterizing evapotranspiration over a meadow ecosystem on the Qinghai-Tibetan Plateau
1
2008
... id="C34">我们的装置存在两个方面的问题: 一是增水导致水分蒸散增加(
Short-term variation of CO2 flux in relation to environmental controls in an alpine meadow on the Qinghai-Tibetan Plateau
1
2003
... id="C32">由于增加降水对ER的影响较弱, NEE的变化主要取决于GEP (
Climate change and water use partitioning by different plant functional groups in a grassland on the Tibetan Plateau
1
2013
... id="C8">高寒草甸生态系统是青藏高原重要的草地生态系统类型, 约占青藏高原总面积的35% (
Growing season ecosystem respirations and associated component fluxes in two alpine meadows on the Tibetan Plateau
1
2008
... id="C31">降水变化会直接或间接地对植物生长和微生物过程造成影响, 继而影响ER (
Convergence across biomes to a common rain-use efficiency
1
2004
... id="C31">降水变化会直接或间接地对植物生长和微生物过程造成影响, 继而影响ER (
Response and adaptation by plants to flooding stress—Preface
1
2005
... id="C30">中国大部分陆地生态系统的观测研究表明, 年降水量变化会对GEP造成显著影响(
Net ecosystem CO2 exchange in Mojave Desert shrublands during the eighth year of exposure to elevated CO2
1
2005
... id="C17">完成NEE观测后, 从底座上移开明箱, 待箱内通风至空气基本与大气本底值接近时, 扣于底座上固定, 并罩上遮光布, 打开红外分析仪, 记录约120 s, 记录频率同样是每秒1次.完成数据观测后,
Temperature and biomass influences on interannual changes in CO2 exchange in an alpine meadow on the Qinghai-Tibetan Plateau
1
2006
... id="C32">由于增加降水对ER的影响较弱, NEE的变化主要取决于GEP (
Evidence of warming and wetting climate over the Qinghai-Tibet Plateau
1
2010
... id="C7">青藏高原被称作“地球第三极”, 平均海拔4 000 m以上, 面积约257万km2, 占中国陆地面积的26.8%左右(
青藏高原氮湿沉降现状及典型放牧草原对氮施肥的响应
1
2014
... id="C11">本研究依托中国科学院纳木错多圈层综合观测研究站(30.77° N, 90.99° E, 海拔4 730 m, 以下简称纳木错站), 2009-2013年生长季日平均温度为7.13 ℃, 2009、2010、2011、2012和2013年年降水量分别为327、494、402、312和397 mm.研究样地位于青藏高原当雄县念青唐古拉山北麓与纳木错湖东南岸之间(30.73° N, 91.02° E), 为典型高寒草甸生态系统, 海拔4 900 m, 距离纳木错站西南约10 km, 2012-2013年生长季日平均温度7.5 ℃, 2012和2013年年降水量分别为311和489 mm (
青藏高原氮湿沉降现状及典型放牧草原对氮施肥的响应
1
2014
... id="C11">本研究依托中国科学院纳木错多圈层综合观测研究站(30.77° N, 90.99° E, 海拔4 730 m, 以下简称纳木错站), 2009-2013年生长季日平均温度为7.13 ℃, 2009、2010、2011、2012和2013年年降水量分别为327、494、402、312和397 mm.研究样地位于青藏高原当雄县念青唐古拉山北麓与纳木错湖东南岸之间(30.73° N, 91.02° E), 为典型高寒草甸生态系统, 海拔4 900 m, 距离纳木错站西南约10 km, 2012-2013年生长季日平均温度7.5 ℃, 2012和2013年年降水量分别为311和489 mm (
Climatic controls and ecosystem responses drive the inter-annual variability of the net ecosystem exchange of an alpine meadow
1
2011
... id="C32">由于增加降水对ER的影响较弱, NEE的变化主要取决于GEP (
Carbon storage in grasslands of China
1
2002
... id="C32">由于增加降水对ER的影响较弱, NEE的变化主要取决于GEP (
Non-additive effects of water and nitrogen addition on ecosystem carbon exchange in a temperate steppe
2
2009
... id="C30">中国大部分陆地生态系统的观测研究表明, 年降水量变化会对GEP造成显著影响(
... id="C31">降水变化会直接或间接地对植物生长和微生物过程造成影响, 继而影响ER (
The impacts of climate change on water resources and agriculture in China
1
2010
... id="C7">青藏高原被称作“地球第三极”, 平均海拔4 000 m以上, 面积约257万km2, 占中国陆地面积的26.8%左右(
Climate extremes and the carbon cycle
1
2013
... id="C6">陆地生态系统碳交换过程包括总生态系统生产力(GEP)和生态系统呼吸(ER), 两者平衡的结果为净生态系统碳交换(NEE).水分是陆地生态系统碳循环过程的重要驱动因子(
Response of soil respiration to short-term experimental warming and precipitation pulses over the growing season in an alpine meadow on the Northern Tibet
1
2015
... id="C31">降水变化会直接或间接地对植物生长和微生物过程造成影响, 继而影响ER (
Steady-state aerobic microbial activity as a function of soil-water content
1
1990
... id="C31">降水变化会直接或间接地对植物生长和微生物过程造成影响, 继而影响ER (
Drought and ecosystem carbon cycling
1
2011
... id="C34">我们的装置存在两个方面的问题: 一是增水导致水分蒸散增加(
Responses of soil respiration to elevated CO2, air warming, and changing soil water availability in a model old-field grassland
2
2007
... id="C31">降水变化会直接或间接地对植物生长和微生物过程造成影响, 继而影响ER (
... id="C33">准确了解降水变化对生态系统的影响需要建立更加科学合理的实验设计, 这需要考虑包括降水的频率、大小以及与其他因素的相互作用等(
Considerable methane uptake by alpine grasslands despite the cold climate:
1
2015
... id="C11">本研究依托中国科学院纳木错多圈层综合观测研究站(30.77° N, 90.99° E, 海拔4 730 m, 以下简称纳木错站), 2009-2013年生长季日平均温度为7.13 ℃, 2009、2010、2011、2012和2013年年降水量分别为327、494、402、312和397 mm.研究样地位于青藏高原当雄县念青唐古拉山北麓与纳木错湖东南岸之间(30.73° N, 91.02° E), 为典型高寒草甸生态系统, 海拔4 900 m, 距离纳木错站西南约10 km, 2012-2013年生长季日平均温度7.5 ℃, 2012和2013年年降水量分别为311和489 mm (
Assessing the response of terrestrial ecosystems to potential changes in precipitation
1
2003
... id="C30">中国大部分陆地生态系统的观测研究表明, 年降水量变化会对GEP造成显著影响(
Researchers illustrate the impact of climate extremes on the carbon cycle
1
2013
... id="C6">陆地生态系统碳交换过程包括总生态系统生产力(GEP)和生态系统呼吸(ER), 两者平衡的结果为净生态系统碳交换(NEE).水分是陆地生态系统碳循环过程的重要驱动因子(
Responses of terrestrial ecosystems to temperature and precipitation change: A meta-analysis of experimental manipulation
2
2011
... id="C6">陆地生态系统碳交换过程包括总生态系统生产力(GEP)和生态系统呼吸(ER), 两者平衡的结果为净生态系统碳交换(NEE).水分是陆地生态系统碳循环过程的重要驱动因子(
... id="C32">由于增加降水对ER的影响较弱, NEE的变化主要取决于GEP (
青藏高原高寒草甸生态系统净二氧化碳交换量特征
1
2005
... id="C13">采用红外CO2分析仪(LI-840, LI-COR, Lincoln, USA)观测不同处理样地的NEE和ER.观测时间为北京时间10:00到12:00, 此时段当地碳吸收通常达到最大值(
青藏高原高寒草甸生态系统净二氧化碳交换量特征
1
2005
... id="C13">采用红外CO2分析仪(LI-840, LI-COR, Lincoln, USA)观测不同处理样地的NEE和ER.观测时间为北京时间10:00到12:00, 此时段当地碳吸收通常达到最大值(
Responses of photosynthetic capacity to soil moisture gradient in perennial rhizome grass and perennial bunchgrass
2
2011
... id="C30">中国大部分陆地生态系统的观测研究表明, 年降水量变化会对GEP造成显著影响(
... id="C32">由于增加降水对ER的影响较弱, NEE的变化主要取决于GEP (
The spatial and temporal dynamics of carbon budget in the alpine grasslands on the Qinghai-Tibetan Plateau using the Terrestrial Ecosystem Model
1
2015
... id="C8">高寒草甸生态系统是青藏高原重要的草地生态系统类型, 约占青藏高原总面积的35% (
气候变化对藏北地区草地生产力的影响模拟
1
2010
... id="C7">青藏高原被称作“地球第三极”, 平均海拔4 000 m以上, 面积约257万km2, 占中国陆地面积的26.8%左右(
气候变化对藏北地区草地生产力的影响模拟
1
2010
... id="C7">青藏高原被称作“地球第三极”, 平均海拔4 000 m以上, 面积约257万km2, 占中国陆地面积的26.8%左右(
Spatial variability of soil moisture at typical alpine meadow and steppe sites in the Qinghai-Tibetan Plateau permafrost region
1
2011
... id="C30">中国大部分陆地生态系统的观测研究表明, 年降水量变化会对GEP造成显著影响(
Spatial patterns and climate drivers of carbon fluxes in terrestrial ecosystems of China
1
2013
... id="C30">中国大部分陆地生态系统的观测研究表明, 年降水量变化会对GEP造成显著影响(
一次降水过程对青藏高原高寒草甸CO2通量和热量输送的影响
1
2008
... id="C8">高寒草甸生态系统是青藏高原重要的草地生态系统类型, 约占青藏高原总面积的35% (
一次降水过程对青藏高原高寒草甸CO2通量和热量输送的影响
1
2008
... id="C8">高寒草甸生态系统是青藏高原重要的草地生态系统类型, 约占青藏高原总面积的35% (
青藏高原21世纪气候和环境变化预估研究进展
1
2015
... id="C7">青藏高原被称作“地球第三极”, 平均海拔4 000 m以上, 面积约257万km2, 占中国陆地面积的26.8%左右(
青藏高原21世纪气候和环境变化预估研究进展
1
2015
... id="C7">青藏高原被称作“地球第三极”, 平均海拔4 000 m以上, 面积约257万km2, 占中国陆地面积的26.8%左右(
论青藏高原范围与面积
1
2002
... id="C7">青藏高原被称作“地球第三极”, 平均海拔4 000 m以上, 面积约257万km2, 占中国陆地面积的26.8%左右(
论青藏高原范围与面积
1
2002
... id="C7">青藏高原被称作“地球第三极”, 平均海拔4 000 m以上, 面积约257万km2, 占中国陆地面积的26.8%左右(
Diurnal, seasonal and annual variation in net ecosystem CO2 exchange of an alpine shrub land on Qinghai-Tibetan Plateau
1
2006
... id="C32">由于增加降水对ER的影响较弱, NEE的变化主要取决于GEP (
1
2000
... id="C8">高寒草甸生态系统是青藏高原重要的草地生态系统类型, 约占青藏高原总面积的35% (
Effects of soil moisture on the temperature sensitivity of soil heterotrophic respiration: A laboratory incubation study
1
2014
... id="C31">降水变化会直接或间接地对植物生长和微生物过程造成影响, 继而影响ER (
Approaches of climate factors affecting the spatial variation of annual gross primary productivity among terrestrial ecosystems in China
1
2016
... id="C30">中国大部分陆地生态系统的观测研究表明, 年降水量变化会对GEP造成显著影响(
Carbon dynamics of terrestrial ecosystems on the Tibetan Plateau during the 20th century: An analysis with a process-based biogeochemical model
1
2010
... id="C8">高寒草甸生态系统是青藏高原重要的草地生态系统类型, 约占青藏高原总面积的35% (
Response of global soil consumption of atmospheric methane to changes in atmospheric climate and nitrogen deposition
1
2013
... id="C31">降水变化会直接或间接地对植物生长和微生物过程造成影响, 继而影响ER (
Copyright © 2021 版权所有 《植物生态学报》编辑部
地址: 北京香山南辛村20号, 邮编: 100093
Tel.: 010-62836134, 62836138; Fax: 010-82599431; E-mail: apes@ibcas.ac.cn, cjpe@ibcas.ac.cn
备案号: 京ICP备16067583号-19