0 引言
【研究意义】大豆疫霉菌(Phytophthora sojae)引起的大豆疫霉根腐病(Phytophthora root rot,PRR)是一种毁灭性的土传病害,在全球范围内均有发生,造成巨大的产量和经济损失[1]。WRKY转录因子在植物生长发育和逆境胁迫方面有着十分重要的作用[2]。因此,研究WRKY在大豆疫霉根腐病抗性方面的功能,对于大豆抗病分子育种有重大意义。【前人研究进展】WRKY蛋白能够与抗病相关基因启动子的W-box结合,激活下游抗病基因的表达,从而开启植物的抗病防卫系统[3,4,5,6]。WRKY转录因子的下游靶标基因和上游调节基因之间的相互作用构成了复杂的WRKY转录因子调控网络[7]。HAN等[8]研究发现,在拟南芥中,AtWRKY44蛋白可以与TOE1(miRNA172的一个靶标蛋白)进行互作,GI-miRNA172-WRKY44可能通过糖信号通路来调节拟南芥的干旱胁迫。丝裂原活化蛋白激酶(mitogen-activated protein kinase,MAPK)信号级联存在于所有真核生物中,并在ABA参与的防御反应的下游信号传导中起作用[9,10]。LI等[11]研究发现拟南芥AtWRKY22和AtWRKY29是MAPK介导的对病原体抗性通路的重要组成部分。AtWRKY29的同源基因在拟南芥叶片中的瞬时表达,可以增强其对细菌和真菌病原体的抗性。DANQUAH等[12]研究表明水稻OsWRKY30通过MAPK磷酸化级联反应能够增强水稻的抗旱性。WRKY转录因子也参与植物激素信号转导。JIANG等[13]研究表明SA可诱导杨树PtrWRKY89过表达,从而加速PR蛋白的表达,提高对杨树黑斑病的抗性。ZHANG等[14]发现在冷应激处理后,过表达黄瓜CsWRKY46的转基因拟南芥在冷胁迫处理时具有较高的幼苗存活率,且种子萌发期间对ABA具有较高的敏感性。各种非生物胁迫,包括热胁迫、氧化应激、干旱和营养缺乏会对植物的生理和生化过程产生不利影响[15,16]。在拟南芥中,过表达棉花GhWRKY17可上调与衰老相关的基因AtWRKY53、AtSAG12和AtSAG13的表达,有助于转基因拟南芥植株延迟叶子的衰老[17]。WU等[18]通过对过表达水稻OsWRKY11的转基因株系进行热处理,转基因株系表现出显著的高温耐受性,如叶子萎蔫的更慢、受损部位更小。HE等[19]研究发现小麦TaWRKY33转基因株系的耐热性与野生型相比明显增强。JIA等[20]发现过表达GhWRKY68棉花植株在干旱和盐胁迫条件下,对氧化应激的耐受性降低,这与活性氧(reactive oxygen species,ROS)的积累、酶活性的降低、MDA含量的升高以及ROS相关基因表达的改变相关。在抵御生物胁迫方面,WRKY转录因子的大多数功能已经明确,但调控机理的研究还不够充分。在大麦(Hordeum vulgare L.)中白粉病病原菌(Blumeria graminis)的无毒基因A10所编码的效应因子能够激活大麦的CC-NBS-LRR类抗病蛋白HvMLA10。HvMLA10与HvWRKY1互作,解除HvWRKY1对HvMYB6的抑制,从而启动防卫反应[21]。拟南芥AtWRKY52转录因子又称为RRS1,除WRKY结构域外,还包含TIR(toll and interleukin-1 receptor)、NBS和LRR结构域,介导拟南芥对病原菌青枯病菌(R. solanacearum)的抗病性[22]。CHENG等[23]研究证明OsWRKY22是水稻抗稻瘟病正调控因子。WANG等[24]研究证明TaWRKY70在小麦高温苗木(HTSP)抗条锈菌(Puccinia striiformis)的过程中可能激活了SA和ET信号通路。【本研究切入点】关于大豆WRKY基因家族己有一些报道,但WRKY基因对大豆疫霉根腐病抗性的研究鲜有报道。【拟解决的关键问题】本研究拟从大豆中克隆出GmWRKY148的CDS全长序列,对其进行组织表达分析和大豆疫霉菌诱导表达分析,利用发根农杆菌介导的遗传转化体系获得大豆发状根,对过表达GmWRKY148的大豆阳性发状根的抗病性进行鉴定,为进一步探究大豆与大豆疫霉菌互作过程的作用机理提供理论依据。1 材料与方法
试验于2017年3月至2018年3月在南京农业大学农学院完成。1.1 试验材料
大豆栽培品种Williams和Williams 82由南京农业大学国家大豆改良中心提供。大豆疫霉菌P6497和P6497R(携带有RFP),由南京农业大学植物保护学院王源超教授提供。植物表达载体pBinGFP2由植物保护学院窦道龙教授提供。发根农杆菌菌株K599由南京农业大学农学院邢邯教授实验室保存。1.2 诱导处理
采用根部接种的方法进行疫霉菌诱导处理。将大豆Williams和Williams 82的种子播种于恒温25℃、16 h/8 h光周期的光照培养箱中。待其生长至两片真叶完全展开时,在根茎交界处划一个约1 cm的伤口。用培养4—5 d的大豆疫霉菌P6497对伤口进行接种。分别在接种后0、6、12和24 h取伤口上下2 cm长的根部组织,液氮速冻并于-80℃保存。1.3 实时荧光定量PCR分析
利用植物总RNA提取试剂盒(DP419,TIANGEN)提取大豆各组织的总RNA,并反转录获得cDNA。根据基因序列设计qPCR引物,GmActin作为内参基因。使用SYBR Green Master Mix(Q111-02,Vazyme)试剂进行实时荧光定量PCR,利用2-ΔΔCT方法计算相对表达量,并用GraphPad prism 5软件作图。1.4 基因克隆及过表达载体构建
提取大豆Williams 82根部组织的RNA,反转录得到cDNA。以此为模板进行PCR扩增, 获得目的基因,与pEASY-Blunt载体连接、鉴定,用于构建pBinGFP2过表达载体。1.5 亚细胞定位
提取质粒,浓缩使其浓度≥1 000 ng·μL-1。洋葱内表皮摆放于MS培养基上,暗培养4 h。通过基因枪技术将包裹了重组质粒的球状金粉转入洋葱表皮细胞,之后暗培养16—20 h,用激光共聚焦显微镜进行观察。1.6 大豆发状根的获得
1.6.1 电击转化农杆菌K599感受态细胞 100 μL K599感受态加1.5 μL质粒装入冰浴的电击杯中,电击转化。加入1 mL YEP培养基,菌液扩培4—6 h。4 500 r/min,2 min离心,弃上清,吸取50 μL涂在YEP加抗生素(YEP+RIF+Kan)的固体培养基上,2 d后挑单克隆,并进行PCR验证。1.6.2 发根农杆菌介导的遗传转化 将20 μL GmWRKY148-GFP加入4 mL(YEP+RIF+Kan)液体培养基中,28℃振荡培养,12—16 h后涂板。挑选颗粒饱满无破损的大豆Willams用氯气消毒。把灭菌的大豆种脐向下种于GM固体培养基中(一皿25—35个),过夜,使大豆充分吸胀。
将农杆菌加入CCM液体培养基中,充分混匀,测OD值(0.6—1.0)。将吸胀的大豆(沿培根对半剖开)浸入菌液中,90 r/min振荡30 min。然后暗培养4—5 d,放入white培养基中继续生长,获得大豆发状根。
1.7 大豆转基因发状根抗性的鉴定
将培养约3周龄的大豆转基因发状根在荧光体视显微镜下进行荧光筛选,对有绿色荧光的大豆转基因发状根进行标记,并扩培。大豆疫霉2号生理小种P6497,在V8固体培养基上培养4 d后进行接种(电子附图1)。切取约3 mm×3 mm的大豆疫霉菌丝块,接种于阳性大豆根毛伸长区,侵染24 h后,利用游标卡尺测量病斑长度。游动孢子接种(电子附图2):选择新鲜的大豆疫霉菌P6497,于25℃黑暗条件下过夜(约10 h)培养,诱导产生游动孢子。带有荧光的大豆转基因发状根放入10 mL离心管中,加入游动孢子过滤液,25℃黑暗培养,接种后24、36和48 h取样。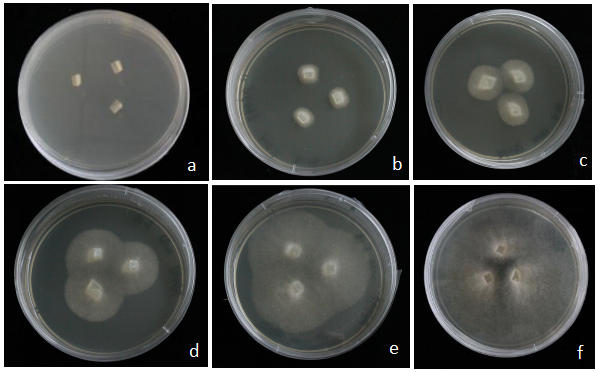
附图1大豆疫霉菌P6497的培养
a:新鲜的菌丝边缘,切成小块贴于固体V8培养基,进行扩繁。b—f:培养2—6 d时,大豆疫霉菌P649的生长情况
-->Fig. S1Formation of mycelium of P6497
a: Choose fresh mycelium edges, then cut them into small pieces, to carry on the propagation. b-f:Growth situation of P6497, after 2 to 6 days
-->
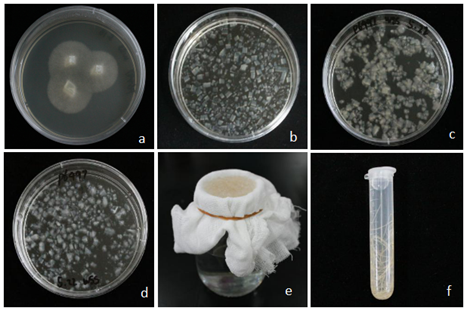
附图2大豆疫霉菌P6497游动孢子的培养
a:扩繁后4 d,大豆疫霉菌P6497的生长情况。b:取培养4d的大豆疫霉菌边缘部分,切成碎块放入液体V8培养基中进行培养。c:灭菌清水充分清洗,诱导产生游动孢子。d:继续培养10 h后,P6497的生长情况。e:过滤,得到透明的游动孢子液。f:大豆阳性发状根浸入游动孢子液
-->Fig. S2Formation of zoospores of P6497
a: After 4 days of propagation, the growth situation of P6497. b: Choose fresh mycelium edges, cut them into tiny pieces, then put them into liquid V8 medium. c: Thoroughly clean the debris with sterile water. d: Growth situation of zoospores of P6497, 10h later. e: Strain the liquid through a piece of gauze, in order to get transparent zoospores fluid. f: Put positive soybean hairy roots in to zoospores fluid
-->
大豆转基因发状根的侵染及侵染水平分析:将经过GFP荧光筛选后得到的阳性大豆转基因发状根(电子附图3)接种大豆疫霉菌P6497,于24、36和48 h后取样,利用荧光体视显微镜(OLYMPUS MVX10,JAPAN)观察游动孢子对阳性大豆发状根的侵染以及卵孢子的萌发情况,并计数。利用qRT-PCR方法检测疫霉的相对积累量(引物序列见电子附表1),以疫霉内参基因GmEF1β 与大豆内参基因GmActin相对表达量的比值来衡量。
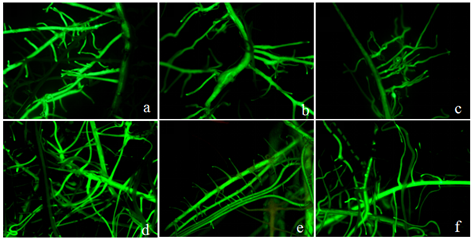
附图S3扩繁的阳性发状根GFP荧光检测图片
a、b、c:GFP转化大豆Williams的发状根。d、e、f:过表达GmWRKY148的大豆Williams发状根
-->Fig. S3GFP fluorescence in enlarged positive transgenic soybean hairy roots
a, b, c: GFP Williams hairy roots. d, e, f : OE-GmWRKY148 Williams hairy roots
-->
Table S1
附表1
附表1试验所用引物序列
Table S1Primers used in this study
基因Gene | 引物序列 Sequence of primer (5'-3') | 引物用途 Function of primer |
---|---|---|
GmWRKY148 | F:GCAGCTTCTAACTCTGGCGA | GmWRKY148克隆 Cloning of GmWRKY148 |
R:TTTATTATAAGTGATAAGATCTACAAGCAA | ||
GmWRKY148-MQ | F:CGGGGTACCATGCCTTTCCGAAATTCCAATATGA | |
R:CGCGGATCCTCATATTTCATTTGGATATTGAGGA | ||
GmWRKY148-2 | F:ATGCACACAACCCACTTGCT | GmWRKY148实时荧光定量PCR qRT-PCR of GmWRKY148 |
R:CAGATGAAGAGTTTCTCTTC | ||
GmEF1β | F:GTTGAAAAGCCAGGGGACA | 疫霉积累量实时荧光定量内参 Reference gene in qRT-PCR |
R:TCTTACCCCTTGAGCGTGG | ||
GmActin | F:GGTGGTTCTATCTTGGCATC | 实时荧光定量PCR内参 Reference gene in qRT-PCR |
R:CTTTCGCTTCAATAACCCTA | ||
pBinGFP2 | F:AAGACCCCAACGAGAAGC | pBinGFP2载体引物 Primer of vector |
R:GAACCCTAATTCCCTTATCTG |
新窗口打开
1.8 GmWRKY148蛋白进化树分析
用GmWRKY148的氨基酸序列在NCBI进行BLAST比对,下载同源性高的蛋白质序列,保存为fasta格式。先用Clustal X软件进行多重序列比对,再利用MEGA 7.0软件进行进化树作图。2 结果
2.1 GmWRKY148蛋白进化树分析
利用MEGA 7.0软件对GmWRKY148与其他植物的WRKY氨基酸序列构建系统进化树(图1)。结果表明,GmWRKY148蛋白属于大豆WRKY转录因子家族基因,与苜蓿(Medicago truncatula)、菜豆(Phaseolus vulgaris)等植物亲缘关系十分相近。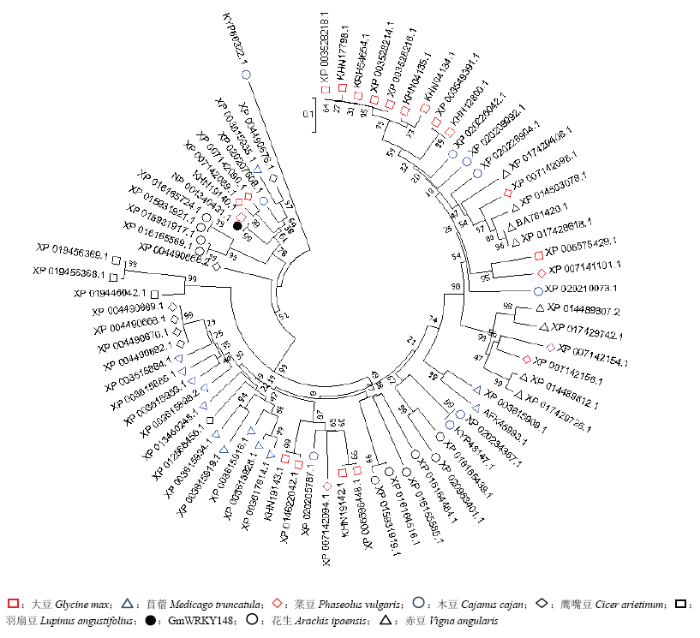
图1GmWRKY148进化树分析
-->Fig. 1Phylogenetic tree analysis of GmWRKY148
-->
2.2 GmWRKY148的表达分析
2.2.1 GmWRKY148的组织表达分析 从Williams 82的根中克隆得到GmWRKY148,利用荧光定量PCR方法,分析大豆GmWRKY148在根、茎、叶和子叶中的表达量(图2)。结果表明,GmWRKY148在大豆的根中的表达量最高,在茎和叶中次之,在子叶中的表达量最低。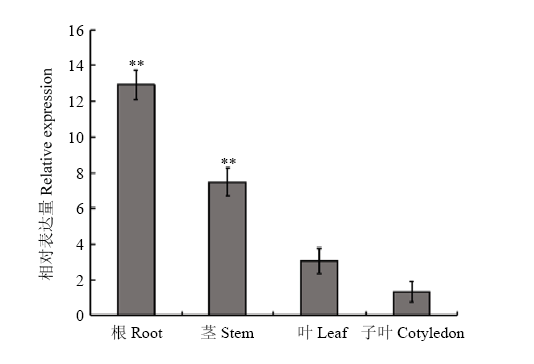
图2GmWRKY148的组织表达分析
**表示在P < 0.01水平差异极显著。下同
-->Fig. 2Tissue-specific expression of GmWRKY148
Asterisks indicate statistically significant differences at P < 0.01. The same as below
-->
2.2.2 GmWRKY148的诱导表达分析 利用荧光定量PCR分析大豆Williams和Williams 82(含有Rps1k)根部接种疫霉菌后GmWRKY148的转录水平。在感病品种Williams中,GmWRKY148的表达量在接种后12 h开始上调,在24 h达到最大值,大约是接种前的10倍(图3-A)。在抗病品种Williams 82中,GmWRKY148的表达在接种后6 h显著上调,12 h下调表达,之后在接种后24 h再次上调,达到最大值(图3-B)。从图中可以看出,GmWRKY148在抗病品种Williams 82中的相对表达量显著高于感病品种Williams,推测GmWRKY148可能参与大豆对疫霉菌的响应。
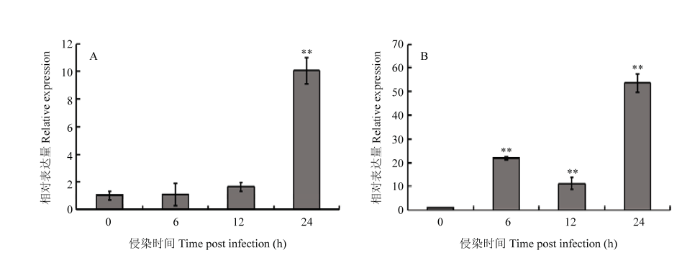
图3GmWRKY148在大豆品种Williams和Williams82中受疫霉诱导下的表达模式
A:疫霉诱导下大豆栽培品种Williams中GmWRKY148的表达模式。B:疫霉诱导下大豆栽培品种Williams 82中GmWRKY148的表达模式
-->Fig. 3Expression pattern of GmWRKY148 response to P. sojae in Williams and Williams82
A: Expression pattern of GmWRKY148 response to P. sojae in Williams. B: Expression pattern of GmWRKY148 response to P. sojae in Williams 82
-->
2.3 GmWRKY148蛋白的亚细胞定位
利用基因枪法将35S-GmWRKY148::GFP重组质粒转化到洋葱表皮细胞中,暗培养16—20 h,用激光共聚焦荧光显微镜观察绿色荧光蛋白的分布。结果显示,在转化空载体35S::GFP的洋葱表皮细胞中,细胞膜、细胞质以及细胞核中均有绿色荧光分布,而在转化重组质粒的洋葱表皮细胞中,绿色荧光主要分布于细胞核部位(图4)。表明GmWRKY148是一个核定位蛋白。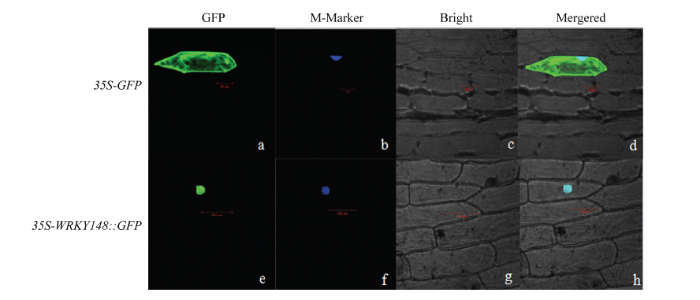
图4GmWRKY148蛋白在洋葱表皮细胞定位分析
a、b、c、d:35S-GFP定位;e、f、g、h:35S-WRKY148::GFP融合蛋白定位
-->Fig. 4Subcellular localization analysis of GmWRKY148 protein in onion epidermal cells
a, b, c, d: 35S-GFP alone location; e, f, g, h: 35S-WRKY148::GFP location
-->
2.4 OE-GmWRKY148大豆发状根的获得
为了探究GmWRKY148在大豆对大豆疫霉菌抗性中的功能,将GmWRKY148在大豆发状根中进行过表达分析,并利用荧光体视显微镜进行初步筛选(图5),将阳性大豆发状根在White培养基上扩大培养。为了进一步检测GmWRKY148的上调表达倍数,将分别扩培的过表达GmWRKY148(OE-GmWRKY148)和转pBinGFP2空载体(EV)的大豆发状根提取RNA,检测GmWRKY148的表达情况(图6),与空载体对照相比,其上调表达倍数达到4—6倍,可用于后续的功能研究。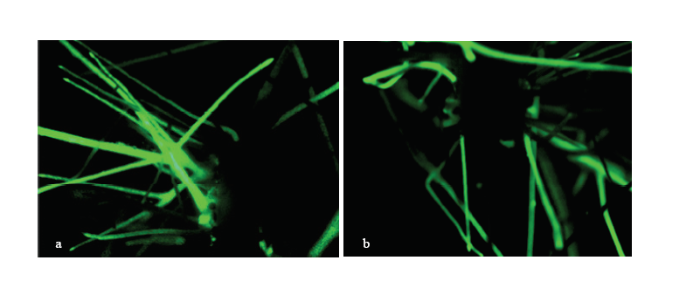
图5转基因阳性发状根的荧光筛选
a:GFP转化大豆Williams的发状根。b:过表达GmWRKY148的大豆Williams发状根
-->Fig. 5GFP fluorescence in transgenic hairy roots
a: GFP Williams hairy roots. b: OE-GmWRKY148 Williams hairy roots
-->
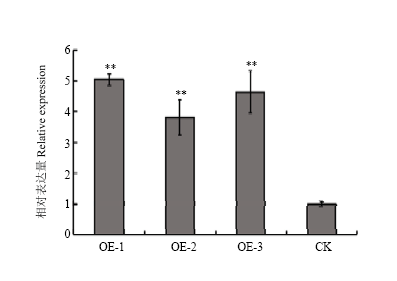
图6Williams阳性发状根的上调表达倍数检测
-->Fig. 6Analysis of over expression efficiency in Williams transgenic soybean hairy roots
-->
2.5 GmWRKY148可提高大豆对大豆疫霉菌的抗性
在大豆Williams中过表达GmWRKY148,获得OE-GmWRKY148大豆发状根。同时将空载体pBinGFP2转入大豆Williams作为对照。通过GFP荧光筛选得到阳性大豆发状根,在阳性大豆发状根上接种新鲜的大豆疫霉P6497菌丝块,24 h后观察接种部位病斑扩展情况。病斑长度统计结果显示,过表达GmWRKY148的大豆转基因发状根(W-OE-W148)接种大豆疫霉菌P6497后的病斑长度明显比Williams空载体对照(W-EV)短(图7-A、图7-B、图7-C)。将过表达GmWRKY148的大豆Williams阳性发状根与对照在white培养基上扩大培养,选取根尖长势一致的大豆转基因发状根接种大豆疫霉游动孢子,结果显示,过表达GmWRKY148的大豆发状根中的疫霉累积量明显低于空载体对照(图7-D)。综上所述,过表达GmWRKY148使Williams对大豆疫霉菌P6497的抗性增强。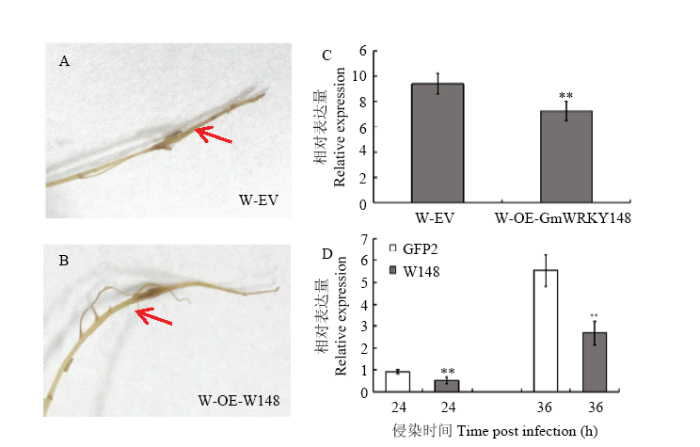
图7GmWRKY148可提高感病大豆品种Williams对大豆疫霉菌的抗性
A:转pBinGFP2空载体的Williams病斑表型。B:过表达GmWRKY148的Williams病斑表型。C:疫霉菌P6497侵染大豆发状根24 h后,大豆Williams中空载对照和OE-GmWRKY148发状根病斑长度统计。D:P6497游动孢子接种后24和36 h,过表达GmWRKY148的大豆Williams发状根和对照中疫霉相对生物积累量的分析
-->Fig. 7GmWRKY148 could improve the resistance to P. sojae in soybean Williams
A:Disease phenotypes of GFP Williams hairy roots after inoculated with P6497 at 24 hpi. B:Disease phenotypes of OE-GmWRKY148 Williams hairy roots after inoculated with P6497 at 24 hpi. C: P6497 infected hairy roots 24h later, the lesion length of GFP Williams hairy roots and OE-GmWRKY148 Williams hairy roots. D: P. sojae biomass was determined by qPCR in inoculated Williams hairy roots OE-GmWRKY148 or EV at 24 and 36 hpi
-->
2.6 OE-GmWRKY148的大豆发状根和对照中菌丝的侵染及卵孢子的萌发情况
利用荧光体视显微镜观察大豆转基因发状根中菌丝的侵染及卵孢子的萌发情况(图8)。接种后24 h,对照EV大豆转基因发状根有35%被侵染,15%产生了卵孢子;而OE-GmWRKY148的大豆发状根只有15%被侵染,且没有萌发的卵孢子。接种后36 h,对照EV大豆发状根,有65%被侵染,30%的大豆转基因发状根能观察到萌发的卵孢子;而对于OE-GmWRKY148的大豆发状根,仅有25%被侵染,10%的阳性大豆发状根能够观察到萌发的卵孢子。接种后48 h,对照EV大豆发状根80% 被侵染,60%能观察到萌发的卵孢子;而OE-GmWRKY148的大豆发状根仅有45%被侵染,20%的阳性大豆发状根能够观察到萌发的卵孢子(电子附表2)。接种大豆疫霉菌P6497的游动孢子24、 36和48 h后,OE-GmWRKY148的大豆Williams发状根与对照EV相比,侵染率以及卵孢子萌发率都较低。综上所述,过表达GmWRKY148能够显著提高大豆对大豆疫霉菌的抗性。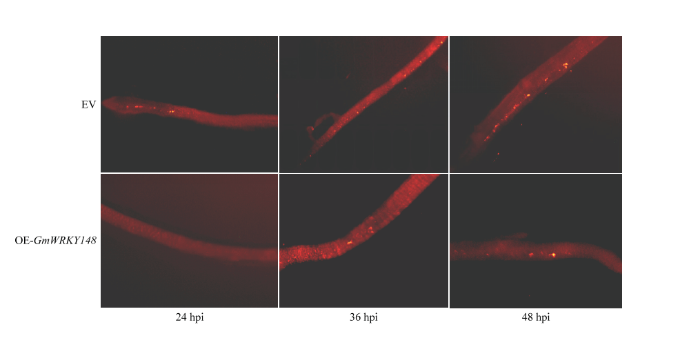
图8大豆Williams发状根中疫霉侵染的显微观察
-->Fig. 8Microscopic analysis of P. sojae infection in soybean Williams hairy roots
-->
Table S2
附表2
附表2附表2
Table S2Table S2 Summary of the numbers of roots that were successfully infected by P. sojae or oospores development
24 hpi | 36 hpi | 48 hpi | ||||
---|---|---|---|---|---|---|
GFP | OE-GmWRKY148 | GFP | OE-GmWRKY148 | GFP | OE-GmWRKY148 | |
被成功侵染的大豆发状根数 Number of roots infected | 7/20 | 3/20 | 13/20 | 5/20 | 16/20 | 9/20 |
萌发卵孢子的大豆发状根数 Number of roots developed oospores | 3/20 | 0/20 | 6/20 | 2/20 | 12/20 | 4/20 |
新窗口打开
3 讨论
大豆疫霉菌(Phytophthora sojae Kaufmann & Gerdemann)可在条件适宜时侵染大豆,有多个生理小种,难以防治[25]。YANG等[26]研究表明采用多种防治措施相结合的综合防治技术是根本有效的防治途径,其中应用抗病品种防治大豆疫霉根腐病最为有效。本研究使用大豆疫霉菌P6497以及感病品种Williams和携带Rps1-k的抗病品种Williams 82作为试验材料。陶恺[27]研究发现大豆疫霉菌侵入后扩展阶段,绝大多数休止孢在接种后6 h已经成功入侵寄主。GmWRKY148在抗病品种Williams 82和感病品种Williams中的表达情况存在差异(图3),接种后6 h在Williams 82 中该基因上调表达倍数更高,说明GmWRKY148受到大豆疫霉菌P6497的诱导,很有可能与大豆抵御大豆疫霉的侵染过程有关。为了进一步研究GmWRKY148在大豆抗疫霉菌侵染中的功能,将其在大豆Williams进行过表达。MOY等[28]研究表明大豆疫霉菌在侵染初期以菌丝体形式扩展定殖,在12–24 h形成病斑,因此选择在侵染后24 h测量病斑长度。转OE- GmWRKY148的大豆Williams发状根与对照EV的大豆Williams发状根相比病斑长度明显变短(图7-A、图7-B、图7-C)。大豆疫霉是典型的土传卵菌,卵孢子能够在土壤中存活数年,成为次年病害发生的初侵染源[29,30]。SINCLAIR[31]发现卵孢子萌发后,产生孢子囊继而释放出游动孢子是病害能够发生的必要条件。HARDHAM等[32,33]研究证明游动孢子感知寄主信号,聚集形成休止孢,同时分泌粘性物质让休止孢粘在寄主根的表面,进而萌发芽管,芽管直接通过细胞间隙和根的垂周壁直接侵入寄主或形成附着孢侵入寄主。张鑫[34]研究发现大部分致病疫霉等的孢子囊可以脱落并直接作为侵染源,而另外一些则不能脱落,以游动孢子为侵染源,如大豆疫霉。综上所述,卵孢子的萌发情况与游动孢子的数量是大豆疫霉根腐病发生的重要指标。
为了研究GmWRKY148在大豆疫霉根腐病中的功能,将OE-GmWRKY148的阳性大豆发状根浸入大豆疫霉游动孢子液中,24、36和48 h取样进行观察。大豆疫霉游动孢子接种大豆发状根后,转OE-GmWRKY148的大豆Williams发状根与对照EV相比,疫霉的积累量在接种后24和36 h,都显著低于对照EV(图7-D)。游动孢子侵染发状根24、36和48 h后,转OE-GmWRKY148的大豆Williams发状根与对照相比,侵染率以及卵孢子萌发率都较低(图8、电子附表2)。以上结果表明,转OE-GmWRKY148的大豆发状根对大豆疫霉的抗性明显增强,过表达GmWRKY148能够提高Williams对大豆疫霉菌的抗性。但是GmWRKY148的调控机制还有待于更进一步研究。
4 结论
克隆获得GmWRKY148,其CDS为999 bp。GmWRKY148在Williams和Williams 82中受疫霉诱导产生表达差异,过表达GmWRKY148可以提高大豆品种Williams对大豆疫霉菌P6497的抗性。The authors have declared that no competing interests exist.
参考文献 原文顺序
文献年度倒序
文中引用次数倒序
被引期刊影响因子
[1] | [D]. [D]. |
[2] | . Sesame (Sesamum indicumL.) is one of the world most important oil crops. However, it is susceptible to abiotic stresses in general, and to waterlogging and drought stresses in particular. The molecular mechanisms of abiotic stress tolerance in sesame have not yet been elucidated. The WRKY domain transcription factors play significant roles in plant growth, development, and responses to stresses. However, little is known about the number, location, structure, molecular phylogenetics, and expression of theWRKYgenes in sesame. We performed a comprehensive study of theWRKYgene family in sesame and identified 71SiWRKYs. In total, 65 of these genes were mapped to 15 linkage groups within the sesame genome. A phylogenetic analysis was performed using a related species (Arabidopsis thaliana) to investigate the evolution of the sesameWRKYgenes. Tissue expression profiles of theWRKYgenes demonstrated that sixSiWRKYgenes were highly expressed in all organs, suggesting that these genes may be important for plant growth and organ development in sesame. Analysis of theSiWRKYgene expression patterns revealed that 33 and 26SiWRKYsrespond strongly to waterlogging and drought stresses, respectively. Changes in the expression of 12SiWRKYgenes were observed at different times after the waterlogging and drought treatments had begun, demonstrating that sesame gene expression patterns vary in response to abiotic stresses. In this study, we analyzed the WRKY family of transcription factors encoded by the sesame genome. Insight was gained into the classification, evolution, and function of theSiWRKYgenes, revealing their putative roles in a variety of tissues. Responses to abiotic stresses in different sesame cultivars were also investigated. The results of our study provide a better understanding of the structures and functions of sesameWRKYgenes and suggest that manipulating theseWRKYscould enhance resistance to waterlogging and drought. The online version of this article (10.1186/s12870-017-1099-y) contains supplementary material, which is available to authorized users. |
[3] | . PR1 is a pathogenesis-related protein encoded in the parsley genome by a family of three genes (PR1-1, PR1-2 and PR1-3), Loss- and gain-of-function experiments in a transient expression system demonstrated the presence of two fungal elicitor responsive elements in each of the PR1-1 and PR1-2 promoters, These elements, W1, W2 and W3, contain the sequence (T)TGAC(C) and mutations that disrupt this sequence abolish function, Gel shift experiments demonstrated that W1, W2 and W3 are bound specifically by similar nuclear proteins, Three cDNA clones encoding sequence-specific DNA-binding proteins were isolated by South-Western screening and these proteins, designated WRKY1, 2 and 3, also bind specifically to W1, W2 and W3. WRKY1, 2 and 3 are members of the family of sequence-specific DNA-binding proteins, which we call the WRKY family, Treatment of parsley cells with the specific oligopeptide elicitor Pep25 induced a transient and extremely rapid increase in mRNA levels of WRKY1 and 3, WRKY2 mRNA levels in contrast showed a concomitant transient decrease, These rapid changes in WRKY mRNA levels in response to a defined signal molecule suggest that WRKY1, 2 and 3 play a key role in a signal transduction pathway that leads from elicitor perception to PR1 gene activation. |
[4] | . Transcripts of SFR2, a member of the S family of receptor kinase genes, accumulate rapidly in Brassica oleracea leaves in response to wounding, bacterial infection and following treatment with salicylic acid (SA). Expression of a chimeric gene consisting of the SFR2 5 flanking sequence fused to the gusA reporter gene is also induced in wounded and SA-treated Arabidopsis plants indicating that the observed response is conferred by the SFR2 promoter. We show here that, in Arabidopsis plants carrying the salicylate hydroxylase ( NahG) transgene, wound induction of the SFR2 promoter gusA reporter fusion was abolished, indicating that, as has previously been shown for the response to bacterial infection, SA is required for the response to wounding. Deletion analysis of the SFR2 promoter identified a region necessary for full expression following SA treatment. This region, which includes two putative W-boxes, is conserved in the promoter of the Arabidopsis SFR2 homologue, ARK3. Deletion of a 12 bp region containing the two W-box motifs reduced the response to SA treatment. Tandem repeats of the W-box-containing element fused upstream of a CaMV 35S minimal promoter enhanced reporter gene expression in transgenic Arabidopsis both in the absence and presence of SA. Gel-mobility shift assays showed that Arabidopsis leaf extracts contained factors that bound to a fragment of the promoter spanning the putative W-boxes and that a fragment in which these motifs were mutated was unable to compete for binding. In summary, induction of the SFR2 promoter in response to bacterial infection and wounding requires SA, and full expression of the induced gene requires the presence of a functional element containing W-box motifs in the SFR2 promoter. The involvement of two W-boxes indicates that transcription factors of the WRKY family may play a key role in mediating these responses. |
[5] | . Mitogen-activated protein kinase (MAPK) cascades are important signaling modules in eukaryotic cells that convert signals generated from the receptors/sensors to cellular responses. Upon activation, MAPKs can be translocated into nuclei where they phosphorylate transcription factors, which in turn activate gene expression. We recently identified NtMEK2, a tobacco MAPK kinase, as the upstream kinase of SIPK and WIPK, two well-characterized tobacco stress-responsive MAPKs. In the conditional gain-of-function NtMEK2 DD transgenic tobacco plants, the activation of endogenous SIPK and WIPK by NtMEK2 DD induces several groups of defense genes, including 3-hydroxy-3-methlyglutaryl CoA reductase ( HMGR ), basic pathogenesis related ( PR ) genes, systemic acquired resistance gene 8.2 ( Sar 8.2 ), and harpin-induced gene1 ( Hin1 ). To identify the transcription factor(s) involved in the activation of these defense genes, we performed gel-mobility shift assays using nuclear extracts from NtMEK2 DD plants. Among the common cis -acting elements present in the promoters of defense-related genes, we observed a strong increase in the binding activity to the W box in nuclear extracts from the NtMEK2 DD plants but not the control NtMEK2 KR plants. The elevated W-box-binding activity in the nuclear extracts cannot be reversed by phosphatase treatment, excluding the possibility of a direct phosphorylation regulation of WRKY transcription factors by SIPK/WIPK. Instead, we observed a rapid increase in the expression of several WRKY genes in the NtMEK2 DD plants. These results suggest that the increase in W-box-binding activity after SIPK/WIPK activation is a result of WRKY gene activation, and the NtMEK2 IPK/WIPK cascade is involved in regulating the expression of genes ranging from transcription factors to defense genes further downstream during plant defense responses. |
[6] | . ABSTRACT Plants constantly face a plethora of abiotic and biotic stresses in their natural habitat. Adapting to such changes requires a great degree of phenotypic plasticity that is mainly determined by the plant's genome. We currently do not know how plants are able to integrate the multitude of partly synergistic/partly antagonistic environmental signals that enable them to respond properly under any given condition. What has become apparent, however, is that plants are capable of extensive reprogramming of their transcriptome in a highly dynamic and temporal manner. This regulation in response, leading to adaptive plasticity of plants in highly variable environments, is mainly achieved by enforcement of a network of various transcription factors (TFs). WRKY TFs are a large family of regulatory proteins forming such a network (Eulgem and Somssich, 2007). They are involved in various plant processes but most notably in coping with diverse biotic and abiotic stresses. In this update, we will restrict our attention to the role of WRKY TFs in plant immunity. |
[7] | . WRKY proteins are emerging players in plant signaling and have been thoroughly reported to play important roles in plants under biotic stress like pathogen attack. However, recent advances in this field do reveal the enormous significance of these proteins in eliciting responses induced by abiotic stresses. WRKY proteins act as major transcription factors, either as positive or negative regulators. Specific WRKY factors which help in the expression of a cluster of stress-responsive genes are being targeted and genetically modified to induce improved abiotic stress tolerance in plants. The knowledge regarding the signaling cascade leading to the activation of the WRKY proteins, their interaction with other proteins of the signaling pathway, and the downstream genes activated by them are altogether vital for justified targeting of the WRKY genes. WRKY proteins have also been considered to generate tolerance against multiple abiotic stresses with possible roles in mediating a cross talk between abiotic and biotic stress responses. In this review, we have reckoned the diverse signaling pattern and biological functions of WRKY proteins throughout the plant kingdom along with the growing prospects in this field of research. |
[8] | . [This corrects the article DOI: 10.1371/journal.pone.0073541.]. |
[9] | . Abiotic stresses impact average yield in agriculture by more than 50% globally. Since ABA is a key regulator of abiotic stress responses, an understanding of its functioning at the molecular level is essential for plant breeding. Although the ABA core signaling pathway has been unraveled, several downstream events are still unclear. MAPKs are involved in most plant developmental stages and in response to stresses. Several members of the MAPK family were shown to be directly or indirectly activated by the ABA core signaling pathway. Recent evidence shows that the complete MAP3K17/18-MKK3-MPK1/2/7/14 module is under the control of ABA, whose members are under the transcriptional and post-translational control of the ABA core signaling pathway. |
[10] | . |
[11] | . |
[12] | . |
[13] | . |
[14] | . 61CsWRKY46gene responds to cold and ABA treatment in cucumber.61CsWRKY46 overexpression regulates freezing and chilling resistance.61CsWRKY46 is localized in the nucleus, and CsWRKY46 bind theABI5promoter.61WRKY46-OE lines were more hypersensitive to ABA during seed germination.61Cold-responsive and ABA-responsive genes were up-regulated in the WRKY46-OE plants. |
[15] | . Increasing vulnerability of plants to a variety of stresses such as drought, salt and extreme temperatures poses a global threat to sustained growth and productivity of major crops. Of these stresses, drought represents a considerable threat to plant growth and development. In view of this, developing staple food cultivars with improved drought tolerance emerges as the most sustainable solution toward improving crop productivity in a scenario of climate change. In parallel, unraveling the genetic architecture and the targeted identification of molecular networks using modern “OMICS” analyses, that can underpin drought tolerance mechanisms, is urgently required. Importantly, integrated studies intending to elucidate complex mechanisms can bridge the gap existing in our current knowledge about drought stress tolerance in plants. It is now well established that drought tolerance is regulated by several genes, including transcription factors (TFs) that enable plants to withstand unfavorable conditions, and these remain potential genomic candidates for their wide application in crop breeding. These TFs represent the key molecular switches orchestrating the regulation of plant developmental processes in response to a variety of stresses. The current review aims to offer a deeper understanding of TFs engaged in regulating plant’s response under drought stress and to devise potential strategies to improve plant tolerance against drought. |
[16] | . HEAT SHOCK TRANSCRIPTION FACTOR A1s (HsfA1s) are the master transcriptional regulators of the HSR. A transcriptional network comprising many TFs and other transcriptional regulators controls the expression of HS-inducible genes throughout the HSR. The activity of HsfA1s and DEHYDRATION-RESPONSIVE ELEMENT BINDING PROTEIN 2A (DREB2A) is tightly controlled through post-translational regulation. Small RNAs, histone modifiers, and transposons are involved in transcriptional regulation and stress memory in the HSR. Ca2+and reactive oxygen species are involved in a signaling pathway that connects HS sensors and transcriptional regulators. |
[17] | . WRKY transcription factors play important roles in plant defense, stress response, leaf senescence, and plant growth and development. Previous studies have revealed the important roles of the group IIaGhWRKYgenes in cotton. To comprehensively analyze the group IIaGhWRKYgenes in upland cotton, we identified 15 candidate group IIaGhWRKYgenes in theGossypium hirsutumgenome. The phylogenetic tree, intron-exon structure, motif prediction and Ka/Ks analyses indicated that most group IIaGhWRKYgenes shared high similarity and conservation and underwent purifying selection during evolution. In addition, we detected the expression patterns of several group IIaGhWRKYgenes in individual tissues as well as during leaf senescence using public RNA sequencing data and real-time quantitative PCR. To better understand the functions of group IIaGhWRKYsin cotton,GhWRKY17(KF669857) was isolated from upland cotton, and its sequence alignment, promotercis-acting elements and subcellular localization were characterized. Moreover, the over-expression ofGhWRKY17inArabidopsisup-regulated the senescence-associated genesAtWRKY53,AtSAG12andAtSAG13, enhancing the plant susceptibility to leaf senescence. These findings lay the foundation for further analysis and study of the functions of WRKY genes in cotton. |
[18] | . Abstract00020002An OsWRKY11 gene, which encodes a transcription factor with the WRKY domain, was identified as one of the genes that was induced by both heat shock and drought stresses in seedlings of rice (Oryza sativa L.). To determine if overexpression of OsWRKY11 confers heat and drought tolerance, OsWRKY11 cDNA was fused to the promoter of HSP101 of rice and introduced into a rice cultivar Sasanishiki. Overexpression of OsWRKY11 was induced by heat treatment. After heat pretreatment, the transgenic lines showed significant heat and drought tolerance, as indicated by the slower leaf-wilting and less-impaired survival rate of green parts of plants. They also showed significant desiccation tolerance, as indicated by the slower water loss in detached leaves. Our results indicate that the OsWRKY11 gene plays a role in heat and drought stress response and tolerance, and might be useful for improvement of stress tolerance. |
[19] | . Drought stress is one of the major causes of crop loss. WRKY transcription factors, as one of the largest transcription factor families, play important roles in regulation of many plant processes, including drought stress response. However, far less information is available on drought-responsive WRKY genes in wheat (Triticum aestivumL.), one of the three staple food crops. Forty eight putative drought-induced WRKY genes were identified from a comparison betweende novotranscriptome sequencing data of wheat without or with drought treatment.TaWRKY1andTaWRKY33from WRKY Groups III and II, respectively, were selected for further investigation. Subcellular localization assays revealed that TaWRKY1 and TaWRKY33 were localized in the nuclei in wheat mesophyll protoplasts. Various abiotic stress-relatedcis-acting elements were observed in the promoters ofTaWRKY1andTaWRKY33. Quantitative real-time PCR (qRT-PCR) analysis showed thatTaWRKY1was slightly up-regulated by high-temperature and abscisic acid (ABA), and down-regulated by low-temperature.TaWRKY33was involved in high responses to high-temperature, low-temperature, ABA and jasmonic acid methylester (MeJA). Overexpression ofTaWRKY1andTaWRKY33activated several stress-related downstream genes, increased germination rates, and promoted root growth inArabidopsisunder various stresses.TaWRKY33transgenicArabidopsislines showed lower rates of water loss thanTaWRKY1transgenicArabidopsislines and wild type plants during dehydration. Most importantly,TaWRKY33transgenic lines exhibited enhanced tolerance to heat stress. The functional roles highlight the importance of WRKYs in stress response. The online version of this article (doi:10.1186/s12870-016-0806-4) contains supplementary material, which is available to authorized users. |
[20] | . |
[21] | . The nucleotide binding domain and Leucine-rich repeat (NLR)-containing proteins in plants and animals mediate pathogen sensing inside host cells and mount innate immune responses against microbial pathogens. The barley (Hordeum vulgare) mildew A (MLA) locus encodes coiled-coil (CC)-type NLRs mediating disease resistance against the powdery mildew pathogen Blumeria graminis. Here, we report direct interactions between MLA and two antagonistically acting transcription factors, MYB6 and WRKY1. The N-terminal CC signaling domain of MLA interacts with MYB6 to stimulate its DNA binding activity. MYB6 functions as a positive regulator of basal and MLA-mediated immunity responses to B. graminis. MYB6 DNA binding is antagonized by direct association with WRKY1 repressor, which in turn also interacts with the MLA CC domain. The activated form of full-length MLA10 receptor is needed to release MYB6 activator from WRKY1 repression and to stimulate MYB6-dependent gene expression. This implies that, while sequestered by the WRKY1 repressor in the presence of the resting immune receptor, MYB6 acts as an immediate and positive postactivation signaling component of the active state of MLA during transcriptional reprogramming for innate immune responses. |
[22] | . |
[23] | . . |
[24] | . |
[25] | . ABSTRACT The effect of calcium compounds [Ca(HCOO)(2)-A and Ca(NO(3))(2)] on the incidence of Phytophthora stem rot of soybean (Glycine max) cv. Tanbakuro was investigated in the field. Disease incidence in control plants in three fields naturally infested with Phytophthora sojae ranged from 11.7 to 52.0% at 140 days after transplanting. Independent of the pathotype diversity, 4 and 10 mM of the calcium compounds applied twice (prior to transplanting and 14 days after transplanting) significantly suppressed disease incidence and delayed onset. Ca(HCOO)(2)-A (Suicaru) was more effective than calcium nitrate for reducing disease incidence. In most cases, the calcium amendments increased plant height, number of nodes and pods, and seed yields, and reduced low-quality seeds. Scanning electron microscopy with fresh samples showed increased accumulation of calcium crystals around the cambium and xylem elements of soybean plants treated with 10-mM Ca(HCOO)(2)-A and Ca(NO(3))(2). Mycelial penetration was inhibited at these sites. These results indicated that calcium-rich areas may be more resistant to invasion by P sojae, and the calcium crystals may play an important role in calcium ion storage and its availability for those tissues to maintain long-term field resistance. |
[26] | . . |
[27] | [D]. [D]. |
[28] | . |
[29] | . The possibility that jets fragment with limited momentum transverse not to the jet momentum but to a color-anticolor axis is considered. This broadens jets somewhat in multijet events and leads to secondary maxima in the laboratory distributions of jet fragments. |
[30] | . |
[31] | . |
[32] | . Motile spores of the phytopathogen Phytophthora cinnamomi are chemotactically attracted to the roots of potential host plants. We have observed that after approaching the root, the zoospores become aligned so that their ventral surface faces the root. This alignment is maintained as the flagella are detached and the cell becomes immobile. Adhesion of the spore to the root ensues rapidly and is a result of the secretion of adhesive material from internal stores confined to the cytoplasm beneath the ventral surface. The germ tube emerges from this same surface and grows straight towards the root. Thus, both adhesion and the orientation of germ tube outgrowth are clearly dependent on the correct alignment of the zoospores at the root surface. |
[33] | . Species of Phytophthora cause serious soilborne diseases of important crop plants and threaten natural ecosystems on a vast scale. Successful infection is usually initiated by motile, biflagellate zoospores that are chemotactically attracted to nearby roots. The zoospores home in at sites on the root surface that are favourable for subsequent penetration. There they encyst, secreting adhesive that glues them to the root surface. The germ tube that emerges from the cysts penetrates the root epidermis, usually growing intercellularly along the anticlinal cell walls. Intracellular growth also occurs and haustoria can develop in cortical cells. Within 2鈥3 days, the pathogen sporulates with the production of chlamydospores in cortical cells and multinucleate sporangia on the root surface. The sporangia undergo cytokinesis and release motile zoospores into the soil. A number of features of the infection cycle contribute to the rapid dissemination of infective propagules and successful disease establishment by Phytophthora pathogens. While basic aspects of the infection cycle were described 30鈥40 years ago, in the last decade immunocytochemical studies have helped uncover new details of cellular and molecular mechanisms involved in processes such as zoospore osmoregulation, motility and adhesion, and asexual sporulation. The increase in our understanding of Phytophthora pathogenesis that has resulted has already pointed to potential targets for novel inhibitors of Phytophthora diseases. Further investigations of the cell and molecular biology of Phytophthora pathogenicity promise to be an integral part of our development of highly specific and sustainable control measures in the future. |
[34] | [D]. [D]. |