

Characteristics of Soil Enzyme Activities and CO2 and CH4 Emissions from Natural Wetland and Paddy Field in Karst Areas
YUAN Wu

通讯作者:
责任编辑: 李云霞
收稿日期:2019-09-5接受日期:2020-02-16网络出版日期:2020-07-16
基金资助: |
Received:2019-09-5Accepted:2020-02-16Online:2020-07-16
作者简介 About authors
袁武,E-mail:

摘要
关键词:
Abstract
Keywords:
PDF (476KB)元数据多维度评价相关文章导出EndNote|Ris|Bibtex收藏本文
本文引用格式
袁武, 靳振江, 程跃扬, 贾远航, 梁锦桃, 邱江梅, 潘复静, 刘德深. 岩溶湿地和稻田的土壤酶活性与CO2和CH4排放特征[J]. 中国农业科学, 2020, 53(14): 2897-2906 doi:10.3864/j.issn.0578-1752.2020.14.013
YUAN Wu, JIN ZhenJiang, CHENG YueYang, JIA YuanHang, LIANG JinTao, QIU JiangMei, PAN FuJing, LIU DeShen.
0 引言
【研究意义】土壤有机碳是生态系统碳分配与转化的核心[1]。湿地在陆地生态系统碳素储存中起着重要作用[2]。因此,维持湿地系统较高的碳储量对应对气候变化具有重要的意义。土壤微生物是土壤碳循环的主要参与者,其胞外酶的活性高低会影响有机质分解,进而影响土壤碳的固定与排放。微生物活动排放的CH4和CO2也是反映土壤微生物活性的重要指标,是重要的温室气体来源[3]。因此,对温室气体排放与土壤酶活性之间关系的明晰能够促进人们对固碳机制的深入理解。改变湿地的土地利用方式导致地上生物量发生变化,这使得土壤酶活性和土壤呼吸也发生改变,进而影响湿地系统碳收支平衡。我国很多地方的稻田多来自于不同历史时期对天然湿地的垦殖[4]。由于人为耕作和管理等带来的土壤性质差异,稻田土壤的酶活性和温室气体排放也发生不同程度的变化。在我国亚热带地区,水热循环较快,土壤碳库的变化尤为敏感。【前人研究进展】目前,对稻田和湿地土壤酶活性的比较研究多集中在湖北梁子湖湿地[5]、荆江湿地[6]和鄱阳湖湿地[7];对稻田和湿地温室气体的研究主要集中在安徽的皖江湿地[8]、崇明岛湿地[9,10]和洪泽湖湿地[11]。会仙湿地位于我国亚热带南端的桂林地区,是现今我国面积最大的岩溶湿地,分布着众多河流、湖泊和水塘等斑块状湿地,被誉为“桂林之肾”。在会仙岩溶湿地系统中,稻田是一种重要的景观类别和土地利用方式。【本研究切入点】蔗糖酶、几丁质酶、β-葡萄糖苷酶和纤维素酶参与蔗糖、几丁质和纤维素等多糖化合物的分解,影响碳素的循环过程;而脲酶和碱性磷酸酶参与氮和磷的循环过程。我们的前期工作报道了会仙湿地系统特定时间点稻田与湿地酶活性的差异[12]和土壤微生物群落结构的差异[13],但是,水稻整个生育期内稻田的温室气体排放特征及其与土壤酶活性的关系还未见报道。【拟解决的关键问题】本文以会仙湿地系统中的稻田和与其相邻湖泊湿地为研究对象,选取4个与碳循环有关的酶、1个与氮循环有关的酶和1个与磷循环有关的酶指标对湖泊湿地和稻田的土壤酶活性进行对比分析;同时结合田间原位测定,探讨温室气体排放与土壤酶活性之间的关联性,以期为揭示稻田土壤的碳循环机制以及湿地系统的保护提供科学的参考依据。1 材料与方法
1.1 研究区概况
会仙湿地位于广西壮族自治区桂林市临桂区东南部的会仙镇冯家村。桂林市属亚热带季风气候,湿热多雨且雨热基本同季,年平均降雨量、相对湿度和平均气温分别为1 887.6 mm、76%和19.1 °C。研究区内土壤是母岩为石灰岩的黑色石灰土。本研究涉及的稻田的利用超过30年,种植单季稻,水稻生长期为89 d。3个稻田样地的面积分别约为4.44×10-2、6.39×10-2和8.40×10-2 hm2,管理方式一致。其中,浅水灌溉(插秧时)、深水灌溉(分蘖结束)和间隙灌溉(抽穗开花)的田面水层厚度分别约为4—6、8—10和2—4 cm;秧苗移栽前施入240 kg·hm-2氮磷钾复合肥(含量均低于15%)和600 kg·hm-2鸡粪作为底肥,5月26日和7月4日分别施用鸡粪600 kg·hm-2和尿素90 kg·hm-2作为追肥。湖泊面积约为0.91 hm2,存在历史超过70年。湖泊湿地的优势植物为双穗雀稗(Paspalum distichum),采样点植物地上部高度约为13.5—43.3 cm,水位为16.4—34.7 cm。1.2 供试土壤与样品采集
2018年5—8月,选取研究区(25°4′8″—25°6′5″N, 110°11′51″—110°17′40″E)内的3个稻田和与其相邻的湖泊湿地作为研究样地。以湖泊湿地中优势植物类型及其分布特点和常水位特征作为选取采样点的依据,以长和宽均为50 cm的静态暗箱作为野外气体采集装置,随着植株高度的变化,加高箱体。箱体由PVC材料制成,箱体顶部安装有用于混匀箱内气体的风扇和采气接口。在上午9:00—11:00之间用60 mL注射器采集气体,分别在箱体密闭后的0、10、20和30 min时采气。施肥、灌溉或晒田时,增加采样频率。灌水和晒田期间的箱体高度分别由水面至箱顶高度和地面至箱顶高度确定。对每个样地分别采集3个重复样本,土壤与气体样本的采集同时进行,土样的采集深度均为0—20 cm。将随机的3个等量样品均匀混合为一个土壤样本,带回实验室后去除植物根系和碎片等杂物,研磨过0.85 mm筛,一部分暂时贮存于-20°C冰箱中,以用于土壤酶活性分析;另一部分自然风干后研磨,分别过0.85 mm、0.25 mm和0.15 mm筛,用于土壤理化指标以及养分含量的测定。1.3 分析方法
1.3.1 土壤理化指标及养分含量 土壤理化指标及养分含量的测定方法参考《土壤农化分析方法》[14]进行。其中,pH采用无CO2蒸馏水作为浸提剂,按照土水1﹕2.5的比例混匀后,用PHS-3E型pH计(上海仪电科学仪器公司,中国)测定;土壤有机碳(SOC)的测定采用高温外热重铬酸钾氧化-容量法;可溶性有机碳(DOC)采用水土振荡提取法,按水土比10﹕1振荡提取,经0.45 μm纤维滤膜过滤后,用multi N/C 3100总有机碳分析仪(Analytik Jena公司,德国)测定;总氮(TN)采用浓硫酸消煮-凯氏定氮法测定;碱解氮(AN)采用碱解扩散法测定;阳离子交换量(CEC)采用乙酸铵浸提-凯氏蒸馏法测定;总磷(TP)采用碳酸钠熔融法测定;有效磷(AP)采用盐酸-氟化铵法测定;交换性钙和交换性镁用Optima 7000 DV电感耦合等离子发射光谱仪(Perkin Elmer公司,美国)测定。1.3.2 土壤酶活性的测定 土壤酶活性的测定方法参考《土壤酶及其研究法》[15]进行。其中,蔗糖酶、脲酶分别采用3,5-二硝基水杨酸比色法和苯酚-次氯酸钠比色法,活性以24 h后每克干土中葡萄糖和铵态氮的毫克数表示;碱性磷酸酶采用磷酸苯二钠比色法,活性以2 h后每克干土中苯酚的毫克数表示;β-葡萄糖苷酶采用硝基酚比色法,活性以1 h后每克土壤中对硝基酚的微克数表示;纤维素酶采用蒽酮比色法,活性以72 h后10 g土壤释放葡萄糖的毫克数表示;采用N-乙酰葡萄糖胺比色法测定土壤几丁质酶,其活性以18 h后每克土壤产生的N-乙酰葡萄糖胺的微克数表示。酶的归一化方法参考文献[6]进行。
1.3.3 温室气体的测定 将采集的气体样品立即带回实验室,用Agilent 7890 B气相色谱质谱联用仪(Agilent公司,美国)进行定量分析。CO2和CH4使用氢火焰离子检测器(FID),检测下限分别为196和0.36 mg·m-3;N2O使用电子捕获检测器(ECD),检测下限为1.96×10-3 mg·m-3。系统每完成48个样品进行一次外标工作曲线的绘制。气体排放速率(dc/dt)是由4个连续样品浓度值的斜率经线性回归分析得出。温室气体排放通量的计算公式如下:
$F=H\times \frac{MP}{R(273+T)}\times \frac{\text{d}c}{\text{d}t}$ (1)
式中:F为气体的排放通量(mg·m-2·h-1);H为采样箱高度(m);M为气体摩尔质量(g·mol-1);P为采样点气压(Pa);R为普适气体常数(8.314,单位为Pa·m3·mol-1·k-1);T为采样时箱内平均气温(°C);dc/dt为气体排放速率(μL·L-1·min-1)。
异养呼吸(Rh)的计算参考文献[16]进行,公式如下:
NPP=NPP籽粒+NPP秸秆+NPP根系+NPP凋落物+NPP根部沉积物
(2)
GPP=NPP+Ra (3)
Rh=Re-Ra (4)
式(2)中,NPP为植物全生长周期内植物地上、地下部分增加的总碳量(kg·hm-2);NPP籽粒和NPP秸秆以收获植株干燥后的生物量计,NPP根系、NPP凋落物和NPP根部沉积物参考文献[17,18,19]进行估算;其中,水稻和双穗雀稗地上部/根系比分别为1.0/0.1和0.7/0.1,凋落物分别占地上部和根系干燥生物量的5%和8%,而根部沉积物分别占植物总生物量的15%和17%。式(3)中,GPP为总初级生产力,Ra为自养呼吸(kg·hm-2),NPP/GPP为0.58[20]。式(4)中,Re为生态系统呼吸,Rh为异养呼吸(kg·hm-2)。
温室气体增温潜势(GHGs)的计算公式如下:
GHGs= FCO2+ FCH4×28+FN2O×265 (5)
式中,以CO2排放量计,单位质量CH4和N2O的增温潜势分别为CO2的28和265倍[21]。FCO2为减去植物自养呼吸后CO2累积排放量;FCH4为CH4累积排放量;FN2O为N2O累积排放量,这三者的单位均为kg·hm-2。
1.4 数据分析与统计
运用Microsoft Excel 2010对测定的数据进行初步整理。利用多属性决策法[6]对生育期酶活性进行归一化处理,用SPSS 19.0对测定数据进行描述性统计分析,运用Duncan法进行方差分析中的多重比较,采用Pearson法对温室气体通量与酶活性进行相关性分析,用加权平均法计算温室气体排放总量,运用Origin 9.5绘制柱状图。2 结果
2.1 土壤理化指标及养分含量
从表1中可以看出,稻田pH显著低于湖泊湿地,交换性镁、SOC、TN、AN、TP、AP和DOC均显著高于湖泊湿地;而CEC、交换性钙在稻田和湖泊湿地中没有显著差异。Table 1
表1
表1不同湿地类型理化指标及养分含量
Table 1
因子 | 湿地类型Wetland type | |
---|---|---|
Factor | 稻田 | 湖泊湿地 |
Paddy field | Lake wetland | |
pH (H2O) | 7.36±0.02b | 7.63±0.08a |
阳离子交换量CEC (cmol·kg-1) | 22.13±0.25a | 20.05±3.21a |
交换性钙 Exchangeable Ca2+ (cmol·kg-1) | 14.39±2.03a | 14.49±0.59a |
交换性镁Exchangeable Mg2+ (cmol·kg-1) | 1.03±0.11a | 0.55±0.04b |
土壤有机碳SOC (g·kg-1) | 26.62±0.32a | 14.49±8.37b |
可溶性有机碳DOC (mg·kg-1) | 202.30±10.34a | 116.60±47.78b |
全氮TN (g·kg-1) | 2.46±0.01a | 1.42±0.71b |
碱解氮AN (mg·kg-1) | 89.54±2.59a | 45.24±3.36b |
全磷TP (g·kg-1) | 0.87±0.04a | 0.33±0.12b |
有效磷AP (mg·kg-1) | 30.50±2.30a | 3.28±0.18b |
新窗口打开|下载CSV
2.2 水稻生育期不同湿地类型酶活性特征
从表2中可以发现,在水稻整个生育期内所测得的8组数据中,稻田的β-葡萄糖苷酶与纤维素酶活性均显著高于湖泊湿地,高出幅度分别为10.8%—57.2%和15.5%—42.1%。蔗糖酶、几丁质酶和脲酶活性6组数据显著高于湖泊湿地,碱性磷酸酶的3组数据显著高于湖泊湿地,其余的数据无显著差异。因此,总体上看,稻田土壤的β-葡萄糖苷酶、纤维素酶、蔗糖酶、几丁质酶、脲酶和碱性磷酸酶活性高于湖泊湿地。Table 2
表2
表2水稻生育期稻田和湖泊湿地的酶活性
Table 2
日期 | 湿地类型 | β-葡萄糖苷酶 | 纤维素酶 | 蔗糖酶 | 几丁质酶 | 脲酶 | 碱性磷酸酶 |
---|---|---|---|---|---|---|---|
Date | Wetland type | β-glucosidase (μg·g-1·h-1) | Cellulase (mg·10g-1·72h-1) | Invertase (mg·g-1·24h-1) | Chitinase (μg·g-1·18h-1) | Urease (mg·g-1·24h-1) | Alkaline phosphatase (mg·g-1·2h-1) |
5.8 | 稻田 Paddy field | 110.2±1.77a | 5.85±0.08a | 68.63±4.38a | 3.86±0.19a | 1.54±0.07a | 1.12±0.05a |
湖泊湿地Lake wetland | 88.67±6.80b | 2.39±0.35b | 32.92±5.52b | 2.61±0.05b | 1.12±0.33b | 0.47±0.08b | |
5.26 | 稻田Paddy field | 87.90±6.32a | 4.04±0.57a | 167.0±11.15a | 4.08±0.13a | 1.01±0.08a | 0.74±0.04a |
湖泊湿地Lake wetland | 68.64±8.57b | 2.69±0.19b | 49.43±16.26b | 2.18±0.05b | 1.13±0.12a | 0.39±0.07b | |
6.3 | 稻田Paddy field | 104.8±19.81a | 5.26±0.61a | 79.25±4.48a | 4.10±0.38a | 1.32±0.09a | 0.94±0.04a |
湖泊湿地Lake wetland | 28.56±15.34b | 2.87±0.38b | 25.67±3.94b | 1.96±0.09b | 0.42±0.08b | 0.26±0.10b | |
6.18 | 稻田Paddy field | 117.7±12.67a | 4.60±0.15a | 106.0±17.71a | 3.23±0.28a | 1.56±0.18a | 1.04±0.12a |
湖泊湿地Lake wetland | 70.20±18.73b | 2.92±0.19b | 93.19±24.76a | 2.65±0.57a | 0.79±0.22b | 0.95±0.07a | |
7.4 | 稻田Paddy field | 71.14±0.49a | 4.18±0.34a | 47.38±2.37a | 2.23±0.11a | 1.22±0.05a | 0.74±0.08a |
湖泊湿地Lake wetland | 44.91±0.41b | 2.54±0.95b | 46.56±3.99a | 2.21±0.04a | 0.97±0.17b | 0.69±0.13a | |
7.16 | 稻田Paddy field | 125.9±33.66a | 4.97±0.59a | 95.92±6.29a | 4.16±0.10a | 1.76±0.26a | 1.13±0.08a |
湖泊湿地Lake wetland | 71.07±18.67b | 3.63±0.54b | 41.34±3.52b | 2.31±0.63b | 1.00±0.33b | 1.18±0.24a | |
7.29 | 稻田Paddy field | 113.4±12.22a | 6.63±0.30a | 57.30±13.02a | 3.69±0.49a | 2.28±0.20a | 1.10±0.17a |
湖泊湿地Lake wetland | 73.21±6.72b | 3.64±0.51b | 32.98±7.59b | 2.46±0.31b | 1.65±0.51a | 1.36±0.20a | |
8.5 | 稻田Paddy field | 115.3±9.73a | 8.19±0.67a | 120.4±27.58a | 3.65±0.27a | 2.52±0.20a | 1.61±0.11a |
湖泊湿地Lake wetland | 88.86±13.44b | 3.69±0.43b | 54.63±12.73b | 2.82±0.13b | 1.59±0.46b | 1.33±0.53a |
新窗口打开|下载CSV
对生育期酶活性归一化后发现(表3),稻田土壤的6个酶活性均较高,高出幅度为11.8%—32.7%。这个结果与表2中的数据总体是一致的,表明稻田土壤中与主要营养元素循环有关的酶活性总体高于天然湖泊湿地。
Table 3
表3
表3水稻生育期酶活性的归一化
Table 3
湿地类型 Wetland type | β-葡萄糖苷酶 β-glucosidase | 纤维素酶 Cellulase | 蔗糖酶 Invertase | 几丁质酶 Chitinase | 脲酶 Urease | 碱性磷酸酶 Alkaline phosphatase |
---|---|---|---|---|---|---|
稻田Paddy field | 0.61 | 0.64 | 0.66 | 0.60 | 0.60 | 0.56 |
湖泊湿地Lake wetland | 0.39 | 0.36 | 0.34 | 0.40 | 0.40 | 0.44 |
新窗口打开|下载CSV
2.3 水稻生育期不同湿地类型温室气体排放特征
如图1-a和1-b所示,稻田生育期CO2排放通量变化范围为255.9—789.7 mg·m-2·h-1,平均值为445.8 mg·m-2·h-1,低于湖泊湿地的574.4 mg·m-2·h-1;CH4排放通量变化范围为-0.41—1.74 mg·m-2·h-1,平均值为0.42 mg·m-2·h-1,低于湖泊湿地的2.55 mg·m-2·h-1。在水稻整个生育期测得的13组数据中,稻田CO2排放通量8组数据显著低于湖泊湿地,2组数据显著高于湖泊湿地,其余数据无显著差异;CH4排放通量11组数据显著低于湖泊湿地,1组数据显著高于湖泊湿地,1组数据无显著差异。因此,总体上看,稻田的CO2和CH4排放通量低于天然湖泊湿地。图1
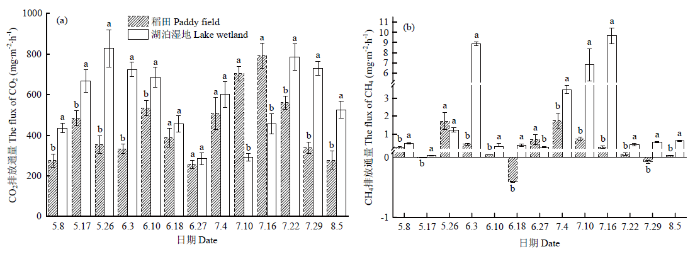
图1稻田和湖泊湿地温室气体排放通量
图中的不同字母表示变量之间差异显著(P<0.05)
Fig. 1Greenhouse gas flux from paddy field and lake wetland
Different letters in the figure indicate significant difference among the variables at P<0.05
从水稻整个生育期来看(表4),稻田CO2、CH4和N2O排放总量均低于湖泊湿地。相比天然湖泊湿地,稻田CO2、CH4和N2O排放总量分别降低了22.3%、83.3%和64.5%。稻田增温潜势(GHGs)比天然湖泊湿地降低了29.6%。
Table 4
表4
表4水稻生育期温室气体排放总量及增温潜势
Table 4
湿地类型 Wetland type | CO2排放总量 Emissions of CO2 (kg·hm-2) | CH4排放总量 Emissions of CH4 (kg·hm-2) | N2O排放总量 Emissions of N2O (kg·hm-2) | GHGs |
---|---|---|---|---|
稻田Paddy field | 9108.4 | 8.38 | 0.38 | 9443.7 |
湖泊湿地Lake wetland | 11722.1 | 50.04 | 1.07 | 13406.8 |
新窗口打开|下载CSV
2.4 温室气体排放与酶活性的相关性分析
从表5中可以看出,生育期的蔗糖酶与β-葡萄糖苷酶、纤维素酶和几丁质酶呈极显著正相关关系(P<0.01);脲酶与碱性磷酸酶、β-葡萄糖苷酶和纤维素酶呈极显著正相关关系,与几丁质酶显著正相关(P<0.05);碱性磷酸酶与β-葡萄糖苷酶极显著正相关,与纤维素酶、几丁质酶显著正相关;此外,β-葡萄糖苷酶、纤维素酶和几丁质酶三者之间极显著正相关。从温室气体排放与酶活性的关系来看,CH4排放通量与6种酶活性多呈极显著或显著的负相关关系,CO2排放通量与β-葡萄糖苷酶、纤维素酶、蔗糖酶和几丁质酶呈极显著或显著的负相关关系。另外,CO2与CH4的排放通量呈显著正相关关系。Table 5
表5
表5温室气体排放与酶活性的相关性
Table 5
β-葡萄糖苷酶 β-glucosidase | 纤维素酶 Cellulase | 蔗糖酶 Invertase | 几丁质酶 Chitinase | 脲酶 Urease | 碱性磷酸酶 Alkaline phosphatase | CO2排放通量 CO2 flux | |
---|---|---|---|---|---|---|---|
纤维素酶 Cellulase | 0.633** | ||||||
蔗糖酶Invertase | 0.433** | 0.433** | |||||
几丁质酶Chitinase | 0.633** | 0.500** | 0.533** | ||||
脲酶Urease | 0.667** | 0.567** | 0.300 | 0.367* | |||
碱性磷酸酶Alkaline phosphatase | 0.433** | 0.400* | 0.233 | 0.367* | 0.533** | ||
CO2排放通量CO2 flux | -0.367* | -0.433** | -0.333* | -0.500** | -0.167 | -0.200 | |
CH4排放通量CH4 flux | -0.683** | -0.417* | -0.483** | -0.483** | -0.550** | -0.350* | 0.317* |
新窗口打开|下载CSV
3 讨论
3.1 稻田利用对生育期土壤酶活性的影响
土壤酶可作为养分循环过程和肥力管理的潜在指标,其在湿地土壤养分的生物地球化学循环中起着重要作用[22]。在本研究中,从整个生育期来看,稻田土壤酶活性总体高于湖泊湿地(表3);这些土壤酶与多个理化指标(SOC、TN、AN、TP和AP)显著正相关[5,6,7],表明在岩溶湿地系统中,稻田利用显著影响了土壤酶活性。主要原因是人为的施肥[23]、地上部凋落物和根系分泌物等的分解归还[1,2]、耕种和对稻田的管理[24],提高了土壤肥力,稻田的SOC、DOC、TN、AN、TP和AP等理化因子发生改变,促进了土壤微生物的生长和微生物酶的分泌,提高了土壤酶活性。本研究整个生育期的结果与我国荆江湿地[6]和鄱阳湖湿地[7]特定时间点的研究结果总体上是一致的。在我们的前期研究中,会仙湿地的河流湿地和稻田的3个土壤酶(蔗糖酶、脲酶和碱性磷酸酶)没有显著差异[12],是因为稻田在经过近3个月的排水休闲以后,土壤有机碳部分降解,多个养分指标下降,与河流湿地的SOC、养分和部分微生物指标没有显著差异。但是,土壤酶与理化性质、微生物指标等呈显著正相关关系的结果表明,土壤酶活性受这些理化指标和微生物特征的明显制约。土壤酶受制于土壤理化指标和微生物特征主要与地上植被的变化和人为干预程度的改变有关[25,26]。湿地植物转变为稻作后,耕作模式和施肥方式等人为活动均会对土壤酶活性产生影响。在本研究的稻田土壤中,水稻植株生物量随生育期逐渐增加,根系分泌物质也增多,土壤有机碳库得以提高[6];而湿地优势植物双穗雀稗其本身的生物量较小,根系归还和地上植被凋落物进入土壤的生物量有限,有机碳库低于人为耕种的稻田。另一方面,人为施用化肥和有机肥使得稻田有机质含量增加,改善了稻田的肥力、质地和营养元素含量[27],导致土壤及水稻根系的微生物种类和数量增加,生长代谢相比湖泊湿地更为活跃,因而稻田的酶活性总体较高[28]。说明人为的施肥管理不仅提高了稻田土壤的酶活性,而且促进了土壤有机碳的积累。此外,稻田较高的有机质输入在为微生物和水稻生长提供新底物的同时,促进了胞外水解酶与老化腐殖物质的吸附结合,而且这种存在形式仍能保持酶的一定活性,具备较强的热稳定性和抗分解能力[28]。因此,与湖泊湿地相比,稻田较高的有机质含量可能伴随着较高的水解酶活性,而有机质的腐殖化程度可能因湿地和植被类型的不同而存在差异。再者,稻田土壤有机质、养分和酶活性的提高带来的可能并不是微生物对有机质的较快分解,而是通过促进微生物对土壤养分利用率的提高进而使土壤有机质得以积累[2,23]。表明湿地的稻田利用显著提高土壤酶活性的同时,也促进了土壤微生物功能活性的演化。因此,相比天然湖泊湿地,施肥、耕作和植被生物量上的差异带来地下部的差异可能是提高稻田生育期酶活性的重要因素。
3.2 稻田利用对CH4和CO2排放的影响
稻田与天然湖泊湿地有着类似的淹水条件,其生育期CH4的排放通量和总量与田间水分条件有着密切的关系[29,30,31]。研究表明,相比烤田处理,持续淹水的稻田CH4排放通量是其12—20倍[29]。在本研究中,天然的湖泊湿地长期淹水,本研究涉及的稻田在水稻抽穗后期无淹水状况(7月16日后),尽管水稻生长后期稻田CH4的排放通量相对前期较低,但仍然显著低于同期的湖泊湿地,表明在生长期水稻根系可能通过增加土壤的通气性抑制了厌氧环境下产甲烷菌的活动。同时,稻田土壤产生的CH4大多被土壤浅层和水稻根系的微生物氧化消耗[32,33],因而较低。生育期稻田CH4的排放不仅与土壤通气性和水分条件的改变有关,更重要的是和水稻的生长过程有关[34]。随着水稻生物量的提高,根系泌氧增加,这促进了CH4的氧化,进而降低了稻田CH4的排放[35]。同时,合理的作物管理模式能改善水稻根系形态和生理性状,在降低稻田CH4排放的同时也能实现水稻增产[36]。另一方面,水稻在种植初期由于人为耕种的原因使得稻苗之间合理有序地排列,这为根系泌氧及甲烷氧化菌的活动提供了良好的空间场所;而湖泊湿地长期淹水,优势植物双穗雀稗地上和地下生物量均比较小,植物及根际间氧气密度低,从而使得CH4的氧化过程受限,有利于产甲烷菌的活动,增加了CH4的排放量。因此,相对于湿地植物双穗雀稗,水稻种植在一定程度上降低了稻田CH4的排放。在本研究中,稻田土壤的CO2排放总量相比天然湖泊湿地低22.3%,表明CO2排放的减少可能是岩溶稻田有利于土壤有机碳积累的一个重要因素。这主要与土壤大团聚体及大团聚体内微团聚体对碳的物理保护和不同粒径颗粒碳的复合以及通过生物化学形成的惰性腐殖质的积累有关[37]。然而,岩溶稻田和湿地的交换性钙含量没有显著差异的结果(表1)表明,通过化学钙键结合的腐殖质并不是造成二者有机碳积累与碳排放分异的主要原因;反而是稻田土壤氧化物结合态碳以及有机质转化为非钙键结合腐殖质比例的变化可能引起稻田土壤碳的积累[38],这主要取决于稻田与湿地土壤有机质内在组分的差异程度。相较湖泊湿地,稻田的利用在土壤团聚体尺度的空间异构性决定了部分微生物在土壤环境中的分布及其对受保护有机质组分的支配度[39]。尽管受矿物复合体保护的颗粒碳在化学上并不趋于稳定,但是由于稻田利用改变了原有土壤空间结构和存在于团聚体界面的充水孔隙,造成土壤微生物与有机质基质分布重组,处于同一位点的微生物与有机质空间位置异构而使有机质变得趋于稳定[39],从而降低了微生物对有机底物的可利用性。这种降低底物可利用性机制与CHI等[40]关于方解石与腐殖质相互作用后,腐殖质颗粒被嵌入和封闭到空腔中的作用机制类似。在本研究中,尽管稻田土壤碳源输入较湖泊湿地多,但是,这并不会激发对稻田原有有机碳的分解,相反,可能会促进其对外源输入碳的积累[39]。另外,稻田常年的水稻耕种和施肥条件改变了源于湿地土壤的“质”,由于土壤温度、水分和通气条件等的微域变化,使得微生物群落结构和对有机质组分的支配发生适应性调整,这会影响微生物和水稻根部的呼吸能力,进而影响稻田CO2的排放。最近我们也发现了稻田与河流湿地土壤微生物群落结构之间的差异性[13]。因此,稻田与湖泊湿地土壤微生物群落结构和土壤有机质的组分差异需要进一步的研究。
在本研究中,β-葡萄糖苷酶、纤维素酶、蔗糖酶和几丁质酶活性与CO2和CH4的排放及其二者之间的相关性结果显示,稻田土壤与碳循环相关酶的活性较高,而CO2和CH4的排放较低;湖泊湿地与碳循环相关酶的活性较低,而CO2和CH4的排放较高。这个结果表明,在会仙岩溶湿地中,土壤酶活性和温室气体的排放受不同机制所控制。而较高土壤酶活性对微生物碳利用率提高的促进作用和较低的碳排放率可能是岩溶稻田土壤有机碳积累的重要原因。因此,在会仙岩溶湿地系统中,加强对稻田实行合理的利用和保护,不仅能促进土壤有机碳的积累,减缓区域的气候变化,也有利于保护独特的会仙岩溶湿地景观。
4 结论
在会仙岩溶湿地系统中,稻田的β-葡萄糖苷酶、纤维素酶、蔗糖酶、几丁质酶、脲酶和碱性磷酸酶活性均显著高于湖泊湿地,高出幅度为11.8%—32.7%。相比天然湖泊湿地,稻田CO2和CH4排放总量分别降低了22.3%和83.3%,而增温潜势(GHGs)降低了29.6%。在本研究中,稻田整个生育期内的6种酶活性整体均高于天然湖泊湿地。以上研究结果表明,在会仙岩溶湿地中,湖泊湿地的稻田利用,能够提高土壤酶活性,降低温室气体的排放。参考文献 原文顺序
文献年度倒序
文中引用次数倒序
被引期刊影响因子
[本文引用: 2]
[本文引用: 2]
[本文引用: 3]
[本文引用: 3]
[本文引用: 1]
[本文引用: 1]
[本文引用: 1]
[本文引用: 1]
[本文引用: 2]
[本文引用: 2]
[本文引用: 6]
[本文引用: 6]
DOI:10.1007/s11368-019-02262-1URL [本文引用: 3]
[本文引用: 1]
[本文引用: 1]
[本文引用: 1]
[本文引用: 1]
[本文引用: 1]
[本文引用: 1]
[本文引用: 1]
[本文引用: 1]
[本文引用: 2]
[本文引用: 2]
[本文引用: 2]
[本文引用: 2]
[本文引用: 1]
[本文引用: 1]
[本文引用: 1]
[本文引用: 1]
[本文引用: 1]
DOI:10.1890/05-1792URLPMID:17494389 [本文引用: 1]

Considerable efforts have been made to assess the contribution of forest and grassland ecosystems to the global carbon budget, while less attention has been paid to agriculture. Net primary production (NPP) of Chinese croplands and driving factors are seldom taken into account in the regional carbon budget. We studied crop NPP by analyzing the documented crop yields from 1950 to 1999 on a provincial scale. Total NPP, including estimates of the aboveground and belowground components, was calculated from harvested yield data by (1) conversion from economic yield of the crop to aboveground mass using the ratio of aboveground residue production to the economic yield, (2) estimation of belowground mass as a function of aboveground mass, and (3) conversion from total dry mass to carbon mass. This approach was applied to 13 crops, representing 86.8% of the total harvested acreage of crops in China. Our results indicated that NPP in Chinese croplands increased markedly during this period. Averaging for each decade, the amount of NPP was 146 +/- 32, 159 +/- 34, 260 +/- 55, 394 +/- 85, and 513 +/- 111 Tg C/yr (mean +/- SD) in the 1950s, 1960s, 1970s, 1980s, and 1990s, respectively. This increase may be attributed to synthetic fertilizer application. A further investigation indicated that the climate parameters of temperature and precipitation determined the spatial variability in NPP. Spatiotemporal variability in NPP can be well described by the consumption of synthetic fertilizer and by climate parameters. In addition, the total amount of residue C and root C retained by the soils was estimated to be 618 Tg, with a range from 300 to 1040 Tg over the 50 years.
[本文引用: 1]
[本文引用: 1]
[本文引用: 1]
[本文引用: 1]
[本文引用: 1]
[D].
[本文引用: 1]
[D].
[本文引用: 1]
[本文引用: 2]
[本文引用: 2]
[本文引用: 1]
[本文引用: 1]
[本文引用: 1]
DOI:10.1371/journal.pone.0172767URLPMID:28263999 [本文引用: 1]

Understanding the effects of external organic and inorganic components on soil fertility and quality is essential for improving low-yielding soils. We conducted a field study over two consecutive rice growing seasons to investigate the effect of applying chemical fertilizer (NPK), NPK plus green manure (NPKG), NPK plus pig manure (NPKM), and NPK plus straw (NPKS) on the soil nutrient status, enzyme activities involved in C, N, P, and S cycling, microbial community and rice yields of yellow clayey soil. Results showed that the fertilized treatments significantly improved rice yields over the first three experimental seasons. Compared with the NPK treatment, organic amendments produced more favorable effects on soil productivity. Notably, the NPKM treatment exhibited the highest levels of nutrient availability, microbial biomass carbon (MBC), activities of most enzymes and the microbial community. This resulted in the highest soil quality index (SQI) and rice yield, indicating better soil fertility and quality. Significant differences in enzyme activities and the microbial community were observed among the treatments, and redundancy analysis showed that MBC and available N were the key determinants affecting the soil enzyme activities and microbial community. The SQI score of the non-fertilized control (0.72) was comparable to that of the NPK (0.77), NPKG (0.81) and NPKS (0.79) treatments but significantly lower compared with NPKM (0.85). The significant correlation between rice yield and SQI suggests that SQI can be a useful to quantify soil quality changes caused by different agricultural management practices. The results indicate that application of NPK plus pig manure is the preferred option to enhance SOC accumulation, improve soil fertility and quality, and increase rice yield in yellow clayey soil.
[本文引用: 1]
[本文引用: 2]
[本文引用: 2]
[本文引用: 2]
[本文引用: 2]
DOI:10.1016/j.jenvman.2018.07.080URLPMID:30119009 [本文引用: 1]

Water drainage is an important mitigation option for reducing CH4 (methane) emissions from residue-amended paddy soils. Several studies have indicated a long-term reduction in CH4 emissions, even after re-flooding, suggesting that the mechanism goes beyond creating temporary oxidized conditions in the soil. In this pot trial, the effects of different drainage patterns on straw-derived CH4 and CO2 (carbon dioxide) emissions were compared to identify the balance between straw-carbon CH4 and CO2 emissions influenced by soil aeration over different periods, including effects of drainage on emissions during re-flooding. The water treatments included were: continuous flooding [C] as the control and five drainage patterns (pre-planting drainage [P], early-season drainage [E], midseason drainage [M], pre-planting plus midseason drainage [PM], early-season-plus-midseason drainage [EM]). An equal amount of (13)C-enriched rice straw was applied to all treatments to identify straw-derived (13)C-gas emissions from soil carbon derived emissions. The highest fluxes of CH4 and delta(13)C-CH4 were recorded from the control treatment in the first week after straw application. The CH4 flux and delta(13)C-CH4 were reduced the most (0.1-0.8mug CH4 g(-1) soil day(-1) and -13 to -34 per thousand) in the pre-planting and pre-planting plus midseason drainage treatments at day one after transplanting. Total and straw-derived CH4 emissions were reduced by 69% and 78% in pre-planting drainage and 77% and 87% in pre-planting plus midseason drainage respectively, compared to control. The early-season, midseason, pre-planting plus midseason and early-season-plus-midseason drainage treatments resulted in higher total and straw-derived CO2 emissions compared to the control and pre-planting drainage treatments. The pre-planting and pre-planting plus midseason drainage treatments lowered the global warming potential by 47-53%, and early-season and early-season-plus-midseason drainage treatments reduced it by 24-31% compared to control. By using labelled crop residues, this experiment demonstrates a direct link between early drainage and reduced CH4 emissions from incorporated crop residues, eventually leading to a reduction in total global warming potential. It is suggested that accelerated decomposition of the residues during early season drainage prolonged the reduction in CH4 emissions. Therefore, it is important to introduce the early drainage as an effective measure to mitigate CH4 emissions from crop residues.
[本文引用: 1]
[本文引用: 1]
URLPMID:14997675 [本文引用: 1]

Methane emission from rice paddy fields is the net result of the combination of many processes, i.e., CH4 production, CH4 oxidation and CH4 transportation in paddy soil. Rice plants play a key role in the CH4 emission from paddy fields, particularly in all the processes involved. The positive and negative effects of rice plants on CH4 emission from paddy fields are well recognized as the main factors influencing the temporal variation of CH4 emission flux in paddy field. Process-based studies about the effects of rice plants on methane emission from paddy fields were summarized, and different roles of rice plants on this emission were discussed. Root exudates and litters of rice plants could serve as the substrate for methanogenesis and enhance the CH4 production of paddy soils, resulting in a high CH4 emission peak, particularly in rice late growing season. Rhizospheric CH4 oxidation induced by rice root-excreted oxygen constitutes a main biogenic sink of CH4, which could account for 36-90% of CH4 produced in paddy soil over the entire growing season of rice. Up to 80% and more of CH4 released from rice field during a growing season could be emitted by rice plant-mediated transport. The fully developed aerenchyma of rice plants could be of importance in CH4 emission during rice growing seasons, and responsible for the CH4 emission peak observed at rice early growing season.
URLPMID:14997675 [本文引用: 1]

Methane emission from rice paddy fields is the net result of the combination of many processes, i.e., CH4 production, CH4 oxidation and CH4 transportation in paddy soil. Rice plants play a key role in the CH4 emission from paddy fields, particularly in all the processes involved. The positive and negative effects of rice plants on CH4 emission from paddy fields are well recognized as the main factors influencing the temporal variation of CH4 emission flux in paddy field. Process-based studies about the effects of rice plants on methane emission from paddy fields were summarized, and different roles of rice plants on this emission were discussed. Root exudates and litters of rice plants could serve as the substrate for methanogenesis and enhance the CH4 production of paddy soils, resulting in a high CH4 emission peak, particularly in rice late growing season. Rhizospheric CH4 oxidation induced by rice root-excreted oxygen constitutes a main biogenic sink of CH4, which could account for 36-90% of CH4 produced in paddy soil over the entire growing season of rice. Up to 80% and more of CH4 released from rice field during a growing season could be emitted by rice plant-mediated transport. The fully developed aerenchyma of rice plants could be of importance in CH4 emission during rice growing seasons, and responsible for the CH4 emission peak observed at rice early growing season.
[本文引用: 1]
[本文引用: 1]
[本文引用: 1]
[本文引用: 1]
[本文引用: 1]
[本文引用: 1]
DOI:10.1016/j.cj.2018.12.011URL [本文引用: 1]
DOI:10.1023/A:1016125726789URL [本文引用: 1]

The relationship between soil structure and the ability of soil to stabilize soil organic matter (SOM) is a key element in soil C dynamics that has either been overlooked or treated in a cursory fashion when developing SOM models. The purpose of this paper is to review current knowledge of SOM dynamics within the framework of a newly proposed soil C saturation concept. Initially, we distinguish SOM that is protected against decomposition by various mechanisms from that which is not protected from decomposition. Methods of quantification and characteristics of three SOM pools defined as protected are discussed. Soil organic matter can be: (1) physically stabilized, or protected from decomposition, through microaggregation, or (2) intimate association with silt and clay particles, and (3) can be biochemically stabilized through the formation of recalcitrant SOM compounds. In addition to behavior of each SOM pool, we discuss implications of changes in land management on processes by which SOM compounds undergo protection and release. The characteristics and responses to changes in land use or land management are described for the light fraction (LF) and particulate organic matter (POM). We defined the LF and POM not occluded within microaggregates (53–250

[本文引用: 1]
[本文引用: 1]
[本文引用: 3]
[本文引用: 3]
DOI:10.1021/acs.est.8b06807URLPMID:31241316 [本文引用: 1]

Global soil carbon cycling plays a key role in regulating and stabilizing the earth's climate change because of soils with amounts of carbon at least three times greater than those of other ecological systems. Soil minerals have also been shown to underlie the persistence of soil organic matter (SOM) through both adsorption and occlusion, but the microscopic mechanisms that control the latter process are poorly understood. Here, using time-resolved in situ atomic force microscopy (AFM) to observe how calcite, a representative mineral in alkaline soils, interacts with humic substances, we show that following adsorption, humic substances are gradually occluded by the advancing steps of spirals on the calcite (1014) face grown in relatively high supersaturated solutions, through the embedment, compression, and closure of humic substance particles into cavities. This occlusion progress is inhibited by phytate at high concentrations (10-100 muM) due to the formation of phytate-Ca precipitates on step edges to prevent the step advancement, whereas phytate at relatively low concentrations (=1 muM) and oxalate at high concentrations (100 muM) have little effect on this process. These in situ observations may provide new insights into the organo-mineral interaction, resulting in the incorporation of humic substances into minerals with a longer storage time to delay degradation in soils. This will improve our understanding of carbon cycling and immobilization in soil ecological systems.