

Molecular Marker-Assisted Identification of Yellow/White Flesh Trait for 122 Peach Cultivars (Lines)
LU ZhenHua

通讯作者:
责任编辑: 赵伶俐
收稿日期:2019-12-25接受日期:2020-05-18网络出版日期:2020-07-16
基金资助: |
Received:2019-12-25Accepted:2020-05-18Online:2020-07-16
作者简介 About authors
鲁振华,E-mail:

摘要
关键词:
Abstract
Keywords:
PDF (998KB)元数据多维度评价相关文章导出EndNote|Ris|Bibtex收藏本文
本文引用格式
鲁振华, 沈志军, 牛良, 潘磊, 崔国朝, 曾文芳, 王志强. 122份栽培桃品种(系)黄白肉性状的分子标记辅助鉴定[J]. 中国农业科学, 2020, 53(14): 2929-2940 doi:10.3864/j.issn.0578-1752.2020.14.016
LU ZhenHua, SHEN ZhiJun, NIU Liang, PAN Lei, CUI GuoChao, ZENG WenFang, WANG ZhiQiang.
0 引言
【研究意义】桃[Prunus persica(L.)Batsch]作为我国第三大落叶果树,是近年来发展最快的树种之一。果肉颜色是果实重要的经济性状之一,由于桃果肉颜色(白/黄)表型特征明显,是桃常见的分类标准。黄肉类型桃富含类胡萝卜素,其风味浓郁、营养丰富[1],而类胡萝卜素又是维生素A合成的前体,和人类健康保健密切相关。欧美国家桃品种选育多以黄肉类型为主,近几年,中国、日本和韩国等逐渐转向黄肉桃类型的品种选育,并有迅速发展的趋势[2]。作为多年生果树,桃树童期较长,果实性状鉴定需要2—3年[3],不利于早期选择,一定程度上延缓了育种进程。同时,由于桃黄肉性状受隐性单基因控制,亲本选配是提高黄肉桃育种效率的前提。【前人研究进展】早在1920年,CONNORS等[4]确定了桃黄肉和白肉是受一对等位基因(Y/y)控制,且白肉相对黄肉为显性遗传。之后研究者对该基因进行研究并分析了不同果肉颜色的代谢成分差异[5,6]。WARBURTON等[7]、ARúS等[8]、CANTíN等[9]和俞明亮等[10]分别获得与Y基因连锁的标记。BRANDI等[5]研究发现黄肉桃品种中的类胡萝卜素含量远高于白肉品种。ADAMIN等[11]通过基因定位发现黄肉桃中调控类胡萝卜素裂解双加氧酶的同源基因CCD4位于桃基因组scaffold 1上,且存在3种等位变异形式:LTR反转录转座子插入,微卫星重复序列差异和A→T碱基替换。CCD4的突变降低了黄肉桃中类胡萝卜素的降解速率,进而在桃黄肉形成中起到至关重要的作用。FALCHI等[12]进一步确定了CCD4不同等位基因控制桃果肉颜色的遗传机制。【本研究切入点】传统的育种方法中,育种工作者常利用一些生物学性状的相关性对杂交后代进行早期鉴定,但鉴定工作繁杂,周期长,随着分子生物学与分子遗传学的发展,在苗期对果肉颜色鉴定已成为可能。【拟解决的关键问题】基于CCD4等位基因不同变异形式,采用InDel、微卫星SSR基因分型和Sanger测序分析对122份栽培品种(系)进行分子鉴定,确定我国主要栽培品种黄白肉性状的等位基因类型,为后续选择相应的标记对子代桃黄/白肉类型的分子鉴定和品种选育奠定基础。1 材料与方法
1.1 材料
试验材料1—79号来源于国家果树种质南京桃资源圃(National Fruit Germplasm Repository of Nanjing,NFGRN),80—122号来源于中国农业科学院郑州果树研究所(Zhengzhou Fruit Research Institute CAAS,ZFRI),具体见表1。全部试验于2017—2018年在中国农业科学院郑州果树研究所的农业农村部果树育种技术重点实验室进行。Table 1
表1
表1CCD4等位基因变异与桃黄白肉表型的关系
Table 1
编号 Code | 品种(系) Cultivar (line) | 来源 Origin | 果肉类型 Flesh color | 微卫星序列 SSR | SNP位点 SNP | CCD4/LTR CCD4/LTR |
---|---|---|---|---|---|---|
1 | 雨花2号 Yuhua 2 | NFGRN | 白 White | 177/177 | AA | 1/1 |
2 | 霞脆 Xiacui | NFGRN | 白 White | 177/177 | AA | 1/1 |
3 | 雨花1号 Yuhua 1 | NFGRN | 白 White | 177/177 | AA | 1/0 |
4 | 瑞光18号 Ruiguang 18 | NFGRN | 黄Yellow | 179/179 | AA | 1/0 |
5 | 瑞光19号 Ruiguang 19 | NFGRN | 白 White | 177/179 | AA | 1/0 |
6 | 早美Zaomei | NFGRN | 白 White | 177/177 | AA | 1/0 |
7 | 红粉佳人 Pinklady | NFGRN | 白 White | 177/177 | AA | 1/0 |
8 | 瑞光美玉 Ruiguangmeiyu | NFGRN | 白 White | 177/179 | AA | 1/0 |
9 | 早花露 Zaohualu | NFGRN | 白 White | 177/177 | AA | 1/0 |
10 | 大久保 Okubo | NFGRN | 白 White | 177/177 | AA | 1/0 |
11 | 金陵黄露 Jinlinghuanglu | NFGRN | 黄 Yellow | 179/179 | AA | 1/0 |
12 | 沪油002号 Huyou 002 | NFGRN | 白 White | 177/179 | AA | 1/0 |
13 | 锦绣 Jinxiu | NFGRN | 黄 Yellow | 177/177 | AA | 0/1 |
14 | 金童7号 Babygold 7 | NFGRN | 黄 Yellow | 177/179 | AA | 1/1 |
15 | 沪油003号 Huyou 003 | NFGRN | 黄 Yellow | 177/179 | AA | 1/1 |
16 | 玉霞蟠桃 Yuxiapantao | NFGRN | 白 White | 177/179 | AA | 1/0 |
17 | 五月火 Mayfire | NFGRN | 黄 Yellow | 179/179 | AA | 1/0 |
18 | 早凤玉 Zaofengyu | NFGRN | 白 White | 177/179 | AA | 1/0 |
19 | 沪021号 Hu 021 | NFGRN | 白 White | 177/177 | AA | 1/1 |
20 | 日川白凤 Richuanbaifeng | NFGRN | 白 White | 177/177 | AA | 1/1 |
21 | 瑞光7号 Ruiguang 7 | NFGRN | 白 White | 177/179 | AA | 1/0 |
22 | 瑞光22号 Ruiguang 22 | NFGRN | 黄 Yellow | 179/179 | AA | 1/0 |
23 | 阿姆肯 Armking | NFGRN | 黄 Yellow | 179/179 | AA | 1/0 |
24 | 霞晖2号 Xiahui 2 | NFGRN | 白 White | 177/177 | AA | 1/0 |
25 | 瑞蟠3号 Ruipan 3 | NFGRN | 白 White | 177/177 | AA | 1/0 |
26 | 早金露 Zaojinlu | NFGRN | 黄 Yellow | 177/179 | AA | 1/1 |
27 | 瑞蟠5号 Ruipan 5 | NFGRN | 白 White | 177/177 | AA | 1/1 |
28 | 晚硕蜜 Wanshuomi | NFGRN | 白 White | 177/177 | AA | 1/0 |
29 | 早露蟠桃 Zaolupantao | NFGRN | 白 White | 177/177 | AA | 1/0 |
30 | 霞晖8号 Xiahui 8 | NFGRN | 白 White | 177/179 | AA | 1/0 |
31 | 晚白花 Wanbaihua | NFGRN | 白 White | 177/177 | AA | 1/1 |
32 | 连黄 Lianhuang | NFGRN | 黄 Yellow | 177/179 | AA | 0/1 |
33 | 春花 Chunhua | NFGRN | 白 White | 177/177 | AA | 1/1 |
34 | 黄露蟠桃 Huanglupantao | NFGRN | 白 White | 177/177 | AA | 1/1 |
35 | 金童5号 Babygold 5 | NFGRN | 黄 Yellow | 177/179 | AA | 1/1 |
36 | 砂子早生 Sunago Wase | NFGRN | 白 White | 177/177 | AA | 1/1 |
37 | 早上海水蜜 Zaoshanghaishuimi | NFGRN | 白 White | 177/177 | AA | 1/0 |
38 | 金童9号 Babygold 9 | NFGRN | 黄 Yellow | 177/177 | AA | 0/1 |
39 | 紫金红2号 Zijinghong 2 | NFGRN | 黄 Yellow | 179/179 | AA | 1/0 |
40 | 银花露 Yinhualu | NFGRN | 白 White | 177/177 | AA | 1/1 |
编号 Code | 品种(系) Cultivar (line) | 来源 Origin | 果肉类型 Flesh color | 微卫星序列 SSR | SNP位点 SNP | CCD4/LTR CCD4/LTR |
41 | 弗雷德里克 Frederica | NFGRN | 黄 Yellow | 179/179 | AA | 1/0 |
42 | 新白凤 Early Hakuho | NFGRN | 白 White | 177/177 | AA | 1/0 |
43 | 奉化玉露早 Fenghuayuluzao | NFGRN | 白 White | 177/177 | AA | 1/0 |
44 | 霞晖4号 Xiahui 4 | NFGRN | 白 White | 177/179 | AA | 1/0 |
45 | 早硕蜜 Zaoshuomi | NFGRN | 白 White | 177/177 | AA | 1/0 |
46 | 早魁蜜 Zaokuimi | NFGRN | 白 White | 177/177 | AA | 1/0 |
47 | 霞光 Xiaguang | NFGRN | 黄 Yellow | 177/179 | AA | 1/1 |
48 | 瑞蟠4号 Ruipan 4 | NFGRN | 白 White | 177/177 | AA | 1/0 |
49 | 京玉 Jingyu | NFGRN | 白 White | 177/177 | AA | 1/1 |
50 | 金霞蟠桃 Jinxiapantao | NFGRN | 黄 Yellow | 179/179 | AA | 1/1 |
51 | 京红 Jinghong | NFGRN | 白 White | 177/177 | AA | 1/0 |
52 | 银河 Galaxy | NFGRN | 白 White | 177/179 | AA | 1/0 |
53 | 瑞光28号 Ruiguang 28 | NFGRN | 黄 Yellow | 179/179 | AA | 1/0 |
54 | 瑞蟠2号 Ruipan 2 | NFGRN | 白 White | 177/177 | AA | 1/0 |
55 | 金晖 Jinhui | NFGRN | 黄 Yellow | 177/177 | AA | 0/1 |
56 | 雨花露 Yuhualu | NFGRN | 白 White | 177/177 | AA | 1/0 |
57 | 白花水蜜 Baihuashuilu | NFGRN | 白 White | 177/177 | AA | 1/1 |
58 | 丰黄 Fenghuang | NFGRN | 黄 Yellow | 177/177 | AA | 0/1 |
59 | 晖雨露 Huiyulu | NFGRN | 白 White | 177/177 | AA | 1/0 |
60 | 奉化玉露 Fenghuayulu | NFGRN | 白 White | 177/177 | AA | 1/0 |
61 | 花玉露 Huayulu | NFGRN | 白 White | 177/177 | AA | 1/0 |
62 | 瑞光23 号 Ruiguang 23 | NFGRN | 白 White | 177/179 | AA | 1/0 |
63 | 瑞蟠1号 Ruipan 1 | NFGRN | 白 White | 177/177 | AA | 1/0 |
64 | 霞晖3号 Xiahui 3 | NFGRN | 白 White | 177/177 | AA | 1/0 |
65 | 金童8号 Babygold 8 | NFGRN | 白 White | 177/177 | AA | 0/1 |
66 | 霞晖1号 Xiahui 1 | NFGRN | 白 White | 177/177 | AA | 1/0 |
67 | 雨花3号 Yuhua 3 | NFGRN | 白 White | 177/177 | AA | 1/1 |
68 | 金陵锦桃 Jinlingjintao | NFGRN | 白 White | 177/177 | AA | 1/0 |
69 | 源东白桃 Yuandongbaitao | NFGRN | 白 White | 177/177 | AA | 1/1 |
70 | 新白花 Xinbaihua | NFGRN | 白 White | 177/177 | AA | 1/1 |
71 | 金童6号 Babygold 6 | NFGRN | 黄 Yellow | 177/179 | AA | 1/1 |
72 | 紫金红3号 Zijinhong 3 | NFGRN | 黄 Yellow | 179/179 | AA | 1/0 |
73 | 金山早红 Jinshanzaohong | NFGRN | 黄 Yellow | 179/179 | AA | 1/0 |
74 | 早红港 Early Redhaven | NFGRN | 黄 Yellow | 177/177 | AA | 0/1 |
75 | 霞晖5号 Xiahui 5 | NFGRN | 白 White | 177/177 | AA | 0/0 |
76 | 南山甜桃 Nanshantiantao | NFGRN | 白 White | 177/177 | AA | 1/0 |
77 | 锦香 Jinxiang | NFGRN | 黄 Yellow | 177/177 | AA | 0/1 |
78 | 弗尔蒂尼莫蒂尼 Fertilia Morettini | NFGRN | 黄 Yellow | 177/179 | AT | 1/0 |
79 | 京春 Jingchun | NFGRN | 白 White | 177/177 | AA | 1/0 |
80 | 中蟠1号 Zhongpan 1 | ZFRI | 白 White | 177/179 | AA | 1/0 |
81 | 99-54-36 | ZFRI | 白 White | 177/179 | AA | 1/0 |
编号 Code | 品种(系) Cultivar (line) | 来源 Origin | 果肉类型 Flesh color | 微卫星序列 SSR | SNP位点 SNP | CCD4/LTR CCD4/LTR |
82 | 36-3 | ZFRI | 白 White | 177/179 | AA | 1/0 |
83 | 黄金蜜1号 Huangjinmi 1 | ZFRI | 黄 Yellow | 179/179 | AA | 1/0 |
84 | 09南9-22 09nan9-22 | ZFRI | 白 White | 177/177 | AA | 1/0 |
85 | 09南10-5 09nan10-5 | ZFRI | 白 White | 177/179 | AA | 1/0 |
86 | 4-3-11 | ZFRI | 黄 Yellow | 179/179 | AA | 1/0 |
87 | 5-3-7 | ZFRI | 黄 Yellow | 179/179 | AA | 1/0 |
88 | 中油桃13号 Zhongyoutao 13 | ZFRI | 白 White | 177/179 | AA | 1/0 |
89 | 春美 Chunmei | ZFRI | 白 White | 177/179 | AA | 1/0 |
90 | 中桃9号 Zhongtao 9 | ZFRI | 白 White | 177/179 | AA | 1/0 |
91 | 中桃10号 Zhogntao 10 | ZFRI | 黄 Yellow | 179/179 | AA | 1/0 |
92 | 08北-12-1 08bei-12-1 | ZFRI | 白 White | 177/177 | AA | 1/0 |
93 | 双喜红 Shuangxihong | ZFRI | 黄 Yellow | 179/179 | AA | 1/0 |
94 | 中油20号 Zhognyou 20 | ZFRI | 白 White | 177/179 | AA | 1/0 |
95 | 中桃红玉 Zhongtaohongyu | ZFRI | 白 White | 177/179 | AA | 1/0 |
96 | 06-3-113 | ZFRI | 黄 Yellow | 179/179 | AA | 1/0 |
97 | 05-3-102 | ZFRI | 黄 Yellow | 179/179 | AA | 1/0 |
98 | 晚油桃 Wanyoutao | ZFRI | 黄 Yellow | 179/179 | AA | 1/0 |
99 | 中油18号 Zhongyou 18 | ZFRI | 白 White | 177/179 | AA | 1/0 |
100 | 1区井蟠 Yiqujingpan | ZFRI | 白 White | 177/179 | AA | 1/0 |
101 | 中桃22号 Zhongtao 22 | ZFRI | 白 White | 177/179 | AA | 1/0 |
102 | 春瑞 Chunrui | ZFRI | 白 White | 177/179 | AA | 1/0 |
103 | 9-6-180 | ZFRI | 白 White | 177/179 | AA | 1/0 |
104 | 04-7-13 | ZFRI | 白 White | 177/179 | AA | 1/0 |
105 | 中桃白玉 Zhongtaobaiyu | ZFRI | 白 White | 177/177 | AA | 1/0 |
106 | 中油19号 Zhongyou 19 | ZFRI | 黄 Yellow | 179/179 | AA | 1/0 |
107 | 中油27号 Zhongyou 27 | ZFRI | 白 White | 177/179 | AA | 1/0 |
108 | 中油12号 Zhongyou 12 | ZFRI | 白 White | 177/179 | AA | 1/0 |
109 | 4-1-6 | ZFRI | 黄 Yellow | 177/179 | AA | 1/1 |
110 | 枣油桃 Zaoyoutao | ZFRI | 白 White | 177/179 | AA | 1/0 |
111 | 红不软 Hongburuan | ZFRI | 白 White | 177/177 | AA | 1/0 |
112 | 6-7-6 | ZFRI | 白 White | 177/179 | AA | 1/0 |
113 | 13-33 | ZFRI | 白 White | 177/179 | AA | 1/0 |
114 | 4-9-16 | ZFRI | 白 White | 177/179 | AA | 1/0 |
115 | 小花红芒果 Xiaohuahongmangguo | ZFRI | 黄 Yellow | 179/179 | AA | 1/0 |
116 | 中桃8号 Zhongtao 8 | ZFRI | 白 White | 177/179 | AA | 1/0 |
117 | 中油15号 Zhongyou 15 | ZFRI | 白 White | 177/179 | AA | 1/0 |
118 | 红芒果 Hongmangguo | ZFRI | 黄 Yellow | 179/179 | AA | 1/0 |
119 | 中蟠2号 Zhongpan 2 | ZFRI | 黄 Yellow | 179/179 | AA | 1/0 |
120 | 春蜜 Chunmi | ZFRI | 白 White | 177/179 | AA | 1/0 |
121 | 中农金辉 Zhongnongjinhui | ZFRI | 黄 Yellow | 179/179 | AA | 1/0 |
122 | 中桃5号 Zhongtao 5 | ZFRI | 白 White | 177/179 | AA | 1/0 |
新窗口打开|下载CSV
1.2 基因组DNA提取
每份桃品种(系)材料取幼嫩叶片约30 mg,液氮研磨,然后用高通量提取叶片基因组DNA,具体提取方法参考张南南等 [13]。提取基因组DNA后利用1%琼脂糖凝胶电泳和NanoDrop 1000 spectrophotometer(Themo Scientific)紫外分光光度计检测DNA浓度和纯度,然后将DNA浓度稀释到工作液浓度(约20—50 ng·μL-1),用于后续的基因分型。1.3 引物设计及PCR扩增
引物序列根据重测序数据设计和参考FUKAMATSU等[14],具体序列信息请见表2,引物和Rox荧光标记引物在上海生工生物工程技术服务有限公司合成。PCR反应体系为2×Taq Master Mix(Mg2+)10 μL,浓度为10 μmol·L-1的正、反向引物各0.2 μL,20—50 ng·μL-1的DNA模板1 μL,使用Eppendorf Mastercycler PCR扩增仪进行DNA扩增。PCR反应程序为95℃ 3 min;95℃ 15 s,55℃ 15 s,72℃ 40 s,共35个循环;72℃ 5 min。Table 2
表2
表2桃中CCD4等位基因序列测序引物
Table 2
名称 Code | 引物序列 Primer sequence | 退火温度 Annealing temperature (℃) | 片段长度 Size (bp) |
---|---|---|---|
CCD4-F | 5'-ACCACCTGTTTGACGGAGAC-3' | 55 | 594 |
CCD4-R | 5'-TGCTCATGAAGAGCTTGCCA-3' | ||
LTR-F(CCD4) | 5'-TACCTGAGAGCTTCTCGTGC-3' | 55 | 729 |
CCD4-SSR-F | 5'- ROX -CCCATTTTGCAGTGAAGGGC-3' | 55 | 177 |
CCD4-SSR-R | 5'-GCTGTGGTGCTTTTGTGGAG-3' | ||
CCD4-SNP-F | 5′-GGGTGATCCAATGCCTAAGA-3′ | 55 | 510 |
CCD4-SNP-R | 5′-GGCTCTCTAGCCACGAAAAA-3′ |
新窗口打开|下载CSV
1.4 CCD4序列、微卫星序列和反转录转座子插入分析
根据控制桃黄肉基因的等位基因形式,CCD4目的片段和LTR反转录转座子插入突变的目的片段在PCR扩增后经1%琼脂糖凝胶电泳检测;CCD44-SSR扩增产物在ABI 3730XL测序仪(擎科生物技术有限公司)进行毛细管电泳分析,然后采用GeneMapper 4.0软件分析SSR标记在122份桃品种(系)中的基因型信息;CCD4-SNP扩增产物进行Sanger测序,利用ContigExpress软件分析测序结果,检测CCD4序列是否发生了碱基A→T替换。2 结果
2.1 CCD4等位基因的LTR插入分析
研究发现[11],桃成熟时由于黄桃肉中的CCD4发生突变而降低了果肉类胡萝卜素的降解速率,从而使桃果肉颜色由白色变为黄色,其中一种突变形式是黄肉桃中CCD4的内含子有一个6 254 bp的LTR反转录转座子插入。本研究利用引物CCD4-F/R和LTR-F(CCD4)/CCD4-R对122份桃栽培品种(系)中的CCD4等位基因进行扩增(图1),分别扩增片段大小为594 bp(图2-A)和729 bp(图2-B),其中729 bp的片段为LTR插入片段。图1
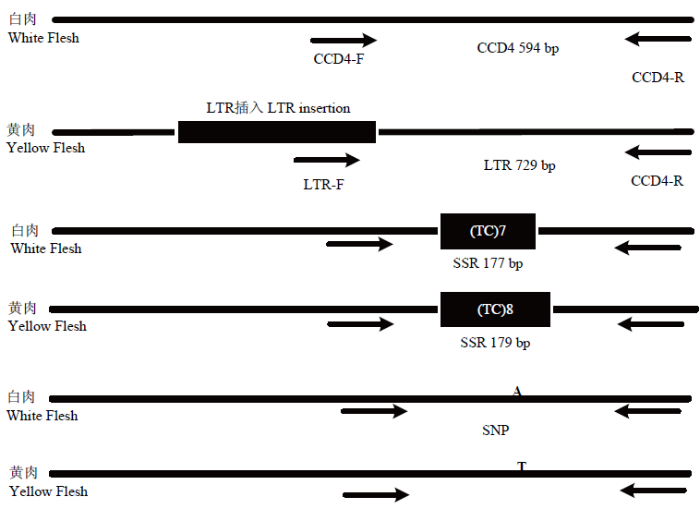
图1检测3种CCD4等位基因的分子标记
Fig. 1Molecular marker of three alleles of CCD4 detecting
图2
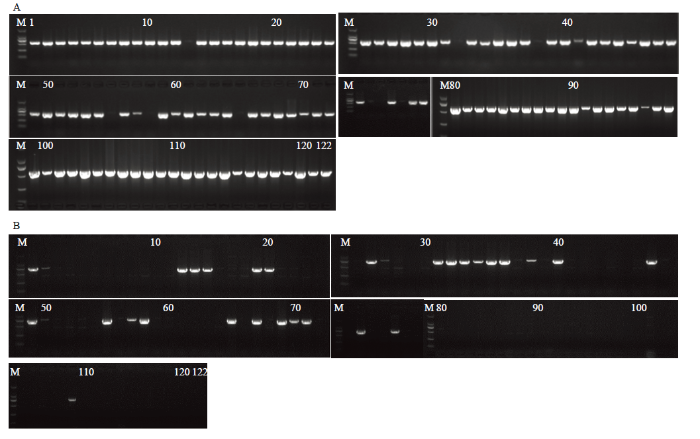
图2122份桃品种(系)CCD4的LTR插入突变检测
M:DL2000 DNA marker。A:122份桃品种(系)引物CCD4-F/R扩增的琼脂糖检测;B:122份桃品种(系)引物CCD4 LTR扩增的琼脂糖检测
Fig. 2CCD4 gene and LTR insertion mutation detected in 122 peach cultivars
M:DL2000 DNA marker。A: Agarose assay for CCD4 F/R amplification in 122 peach cultivars (lines); B: Agarose assay for LTR amplification of CCD4 in 122 peach cultivars (lines)
统计发现在122份桃品种(系)材料中有8份材料中的CCD4存在LTR插入纯合突变,31份材料存在LTR插入杂合突变,存在LTR插入的品种(系)占比相对较高,为25%。由于桃黄/白肉颜色中,白肉性状为显性,因此在LTR杂合突变的材料中存在其他突变类型,从而调控桃果肉黄色性状。
2.2 CCD4等位基因的SSR微卫星分析
黄肉桃品种中存在一种CCD4的微卫星重复序列由(TC)7突变为(TC)8,即二者存在2 bp的差异,从而使CCD4蛋白翻译时提前终止而丧失功能。利用SSR分子标记结合荧光毛细管电泳技术,准确分析了122份桃栽培品种(系)中CCD4的第一个外显子区域SSR重复序列,发现在黄肉桃品种中CCD4等位基因序列中的微卫星重复序列比白肉桃多2 bp的碱基,如‘霞脆’为纯合白肉桃,扩增片段大小为177 bp;‘瑞光22号’为纯合黄肉桃,扩增片段为179 bp;‘春美’为杂合白肉桃,扩增片段为177/179 bp(图3)。本研究所采用的122份材料中有25份材料存在SSR纯合突变,68份材料存在SSR杂合突变,占总材料的55.7%,可能与该类型突变材料在实际育种中作为亲本的比例较高有关。图3
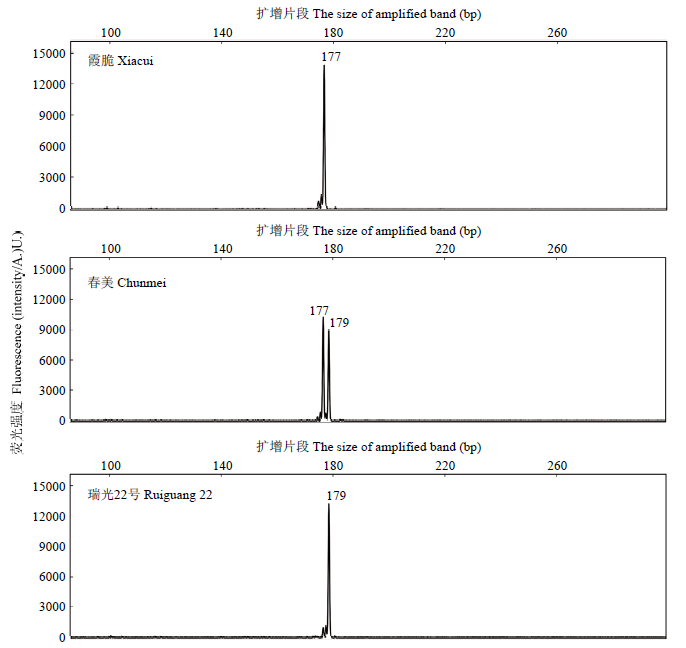
图3CCD4 3种不同的SSR基因型
Fig. 3Three types of SSR fingerprints in CCD4 locus
2.3 CCD4等位基因序列SNP位点检测
结合Sanger测序对122份桃栽培品种(系)中的CCD4等位基因序列进行分析,发现在122份材料中仅有‘弗尔蒂尼莫蒂尼’一份材料CCD4等位基因发生了A→T替换突变(图4),说明在黄肉桃中CCD4等位基因发生核苷酸替换的突变率极低或者该品种极少用于品种选育的亲本。图4
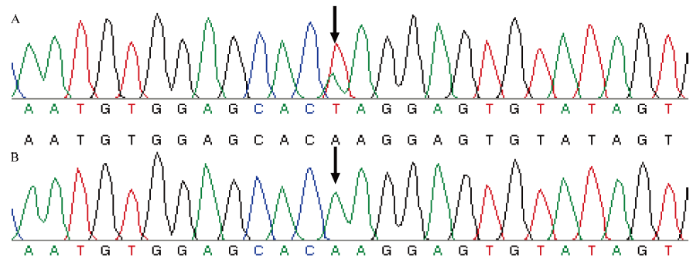
图4基于Sanger测序的SNP基因分型(A:杂合SNP位点;B:纯合SNP位点)
Fig. 4Genotyping results using Sanger sequencing (A: Heterozygous SNP locus; B: Homozygous SNP)
2.4 122份桃品种(系)的等位基因类型
利用CCD4等位基因片段扩增、微卫星基因分型分析、Sanger测序分析结果对122份桃品种(系)材料中的果肉黄白颜色进行区分。在122份桃种质材料中可以扩增出CCD4目的基因及LTR插入的目的片段记为1,无法扩增的记为0,统计结果和SSR基因分型及SNP位点测序结果如表1所示。发现SSR基因型为179/179时,对应桃品种的果肉颜色为黄色;LTR扩增片段为0/1时,对应桃品种的果肉颜色为黄色;SSR基因型为177/179,CCD4和LTR扩增片段为0/1时,对应材料的果肉颜色为黄色;SSR基因型为177/179,SNP位点基因型为A/T时,对应桃品种的果肉颜色为黄色,其余组合对应材料的果肉颜色为白色(表1)。通过分析3种不同类型等位基因发现,纯合CT插入导致果肉颜色为黄色的品种(系)为25个;纯合LTR插入导致果肉颜色为黄色的品种(系)为8个;LTR插入和CT插入变异导致果肉颜色为黄色的品种(系)为7个;CT插入和SNP替代导致果肉颜色为黄色的品种(系)为1个(表3),分析结果与122份桃品种(系)果肉黄白颜色性状的田间调查结果一致。说明可以综合利用CCD4等位基因扩增、SSR基因分型和Sanger测序等分子标记,准确又快速地对桃的杂交后代幼苗进行黄/白肉性状的区分。Table 3
表3
表3不同黄肉桃品种(系)CCD的等位基因类型
Table 3
基因型 Genotype | 品种(系) Cultivar (line) |
---|---|
CT插入 CT insertion | 瑞光18号 Ruiguang 18 |
金陵黄露 Jinlinghuanglu | |
五月火 Mayfire | |
瑞光22号 Ruiguang 22 | |
阿姆肯 Armking | |
连黄 Lianhuang | |
紫金红2号 Zijinghong 2 | |
弗雷德里克 Frederica | |
金霞蟠桃Jinxiapantao | |
瑞光28号 Ruiguang 28 | |
紫金红3号 Zijinhong 3 | |
金山早红 Jinshanzaohong | |
黄金蜜1号 Huangjinmi 1 | |
4-3-11 | |
5-3-7 | |
中桃10号 Zhongtao 10 | |
双喜红 Shuangxihong | |
06-3-113 | |
05-3-102 | |
晚油桃 Wanyoutao | |
中油19号 Zhongyou 19 | |
小花红芒果 Xiaohuahongmangguo | |
红芒果 Hongmangguo | |
中蟠2号 Zhongpan 2 | |
中农金辉 Zhongnongjinhui | |
LTR插入 LTR insertion | 锦绣 Jinxiu |
连黄 Lianhuang | |
金童9号 Babygold 9 | |
金晖 Jinhui | |
丰黄 Fenghuang | |
早红港 Early Redhaven | |
锦香 Jinxiang 金童8号 Babygold 8 | |
CT重复+LTR插入 CT insertion + LTR insertion | 金童7号 Babygold 7 |
沪油003号 Huyou 003 | |
早金露 Zaojinlu | |
金童5号 Babygold 5 | |
霞光 Xiaguang | |
金童6号 Babygold 6 | |
04-7-13 | |
CT插入+SNP替换 CT insertion +SNP subsitution | 弗尔蒂尼莫蒂尼 Fertilia Morettini |
新窗口打开|下载CSV
3 讨论
桃栽培品种为二倍体,其基因组小、童期短,相对其他多年生木本植物遗传改良周期较短[15]。桃全基因组的测序和高质量的组装[16],加速了对目标性状基因的定位,为桃的分子辅助选种和果树遗传改良奠定了重要的基础[17]。基因定位是实现目标性状分子鉴定的前提,而确定控制目标性状的基因则可实现表型的直接分子鉴定。采用分子标记进行辅助选种可以通过两种途径;(1)通过对控制性状的基因序列开发分子标记可以直接完成表型鉴定;(2)根据亲本基因型,在目标性状位点两侧开发分子标记,进而实现表型的鉴定。其中第一种可实现100%的表型预测,第二种准确性主要取决于定位的区间大小。目前,桃上已经克隆多个控制质量性状的候选基因,包括分枝角度[18,19]、矮化[20,21]、黏离核[22]、果形[23]、桃果实毛/油[24]、肉质[25]以及桃果肉颜色(红、黄和白肉)等基因[11-12,26],实现了对表型性状的直接分子鉴定。在植物长期的进化和人工选择过程中产生不同类型的等位变异,包括LTR插入、微卫星重复序列和单碱基变异等。其中逆转录转座子(retrotransposons)LTR和non-LTR是真核植物基因组中常见的变异类型之一,特别是长末端重复序列(long terminal repeat,LTR)的插入导致基因的突变在作物中最为常见,可改变植物基因表达和转录完整性[27]。如葡萄的果皮颜色[28]、苹果果肉颜色[29]和柑橘颜色[30]等均是由于LTR的插入导致。微卫星在植物基因组分布较为广泛,微卫星重复数改变多见于内含子区,有些也发生在基因编码区。在对已报到的3种CCD4等位变异形式进行基因型鉴定发现,白肉CCD4包括3个外显子、1个内含子、7个短的CT重复。在黄肉类型中,由于8个CT重复导致翻译提前终止,果肉表现黄色。自然界植物中也存在单碱基的突变导致表型的改变[31],如LEE等[32]发现编码区一个单个碱基A突变为G,进而导致水稻胚大小的改变。在桃和番茄中也发现了单个碱基的突变导致编码蛋白提前终止,从而使植株产生突变表型[33]。
本研究对122份品种(系)的基因型分析发现,LTR插入和微卫星重复数变化所占比例较高,仅有1个品种是A→T替换突变导致果肉颜色由白色变为黄色,该结果与FUKAMATSU等[14]研究结果一致,即对39份日本桃品种进行分析,并未检测到CCD4等位基因A→T替换突变类型。综合分析认为,可能CT重复数差异突变的单株最早作为亲本用于品种的选育,主导了现有品种的谱系,才导致该类型占比较多,也可能多个单株在重组过程中,出现相同的变异类型。微卫星重复数量差异和LTR插入是引起桃果肉颜色表型自然突变的主要途径。
4 结论
利用CCD4等位基因扩增、微卫星SSR基因分型分析和Sanger测序分析对122份桃种质资源进行果肉黄/白CCD4等位基因突变鉴定,明确了苗期可以快速区分桃子代果肉颜色的分子鉴定方法,加快了桃品种选育及后代果肉颜色鉴定进程,为桃品种的选育及改良提供了重要的参考依据。参考文献 原文顺序
文献年度倒序
文中引用次数倒序
被引期刊影响因子
[本文引用: 1]
[本文引用: 1]
DOI:10.1007/s11295-012-0523-6URL [本文引用: 1]
[本文引用: 1]
DOI:10.1186/1471-2229-11-24URLPMID:21269483 [本文引用: 2]

BACKGROUND: Carotenoids are plant metabolites which are not only essential in photosynthesis but also important quality factors in determining the pigmentation and aroma of flowers and fruits. To investigate the regulation of carotenoid metabolism, as related to norisoprenoids and other volatile compounds in peach (Prunus persica L. Batsch.), and the role of carotenoid dioxygenases in determining differences in flesh color phenotype and volatile composition, the expression patterns of relevant carotenoid genes and metabolites were studied during fruit development along with volatile compound content. Two contrasted cultivars, the yellow-fleshed 'Redhaven' (RH) and its white-fleshed mutant 'Redhaven Bianca' (RHB) were examined. RESULTS: The two genotypes displayed marked differences in the accumulation of carotenoid pigments in mesocarp tissues. Lower carotenoid levels and higher levels of norisoprenoid volatiles were observed in RHB, which might be explained by differential activity of carotenoid cleavage dioxygenase (CCD) enzymes. In fact, the ccd4 transcript levels were dramatically higher at late ripening stages in RHB with respect to RH. The two genotypes also showed differences in the expression patterns of several carotenoid and isoprenoid transcripts, compatible with a feed-back regulation of these transcripts. Abamine SG - an inhibitor of CCD enzymes - decreased the levels of both isoprenoid and non-isoprenoid volatiles in RHB fruits, indicating a complex regulation of volatile production. CONCLUSIONS: Differential expression of ccd4 is likely to be the major determinant in the accumulation of carotenoids and carotenoid-derived volatiles in peach fruit flesh. More in general, dioxygenases appear to be key factors controlling volatile composition in peach fruit, since abamine SG-treated 'Redhaven Bianca' fruits had strongly reduced levels of norisoprenoids and other volatile classes. Comparative functional studies of peach carotenoid cleavage enzymes are required to fully elucidate their role in peach fruit pigmentation and aroma.
[本文引用: 1]
DOI:10.1007/BF00224094URLPMID:24162426 [本文引用: 1]

The identification of molecular markers linked to economically important traits for use in crop improvement is very important in long-lived perennial species. Three-hundred-and-sixty RAPD primers were used with bulked segregant analysis to identify markers linked to loci of specific interest in peach [(Prunus persica) L. Batch] and peach x almond [(Prunus dulcis) Batch] crosses. The traits analyzed included flesh color, adhesion, and texture; pollen fertility; plant stature; and three isozyme loci. The Mendelian behavior of the RAPD loci was established, and RAPD markers were mapped relative to the loci controlling flesh color, adhesion, and texture, and the isozyme loci Mdh-1, 6Pgd-2 and Aat-1, as well as the existing RFLP genetic linkage map constructed previously using a peach x almond F2 population. This technique has facilitated rapid identification of RAPD and RFLP markers that are linked to the traits under study. Loci controlling these traits mapped predominantly to linkage groups 2 and 3 of the peach genetic linkage map. Linkages to genes with both dominant and co-dominant alleles were identified, but linkages to dominant genes were more difficult to find. In several crosses, RAPD marker bands proved to be allelic. One co-dominant RAPD formed a heteroduplex band in heterozygous individuals and in mixtures of alternate homozygotes. The Mendelian behavior of the RAPD loci studied was established and the results suggest that RAPD markers will be useful for plant improvement in peach.
[本文引用: 1]
[本文引用: 1]
[本文引用: 1]
[本文引用: 1]
[本文引用: 3]
DOI:10.1111/tpj.12283URLPMID:23855972 [本文引用: 2]

Peach flesh color (white or yellow) is among the most popular commercial criteria for peach classification, and has implications for consumer acceptance and fruit nutritional quality. Despite the increasing interest in improving cultivars of both flesh types, little is known about the genetic basis for the carotenoid content diversity in peach. Here we describe the association between genotypes at a locus encoding the carotenoid cleavage dioxygenase 4 (PpCCD4), localized in pseudomolecule 1 of the Prunus persica reference genome sequence, and the flesh color for 37 peach varieties, including two somatic revertants, and three ancestral relatives of peach, providing definitive evidence that this locus is responsible for flesh color phenotype. We show that yellow peach alleles have arisen from various ancestral haplotypes by at least three independent mutational events involving nucleotide substitutions, small insertions and transposable element insertions, and that these mutations, despite being located within the transcribed portion of the gene, also result in marked differences in transcript levels, presumably as a consequence of differential transcript stability involving nonsense-mediated mRNA decay. The PpCCD4 gene provides a unique example of a gene for which humans, in their quest to diversify phenotypic appearance and qualitative characteristics of a fruit, have been able to select and exploit multiple mutations resulting from a variety of mechanisms.
[本文引用: 1]
[本文引用: 1]
[本文引用: 2]
DOI:10.1186/1471-2156-11-69URLPMID:20646280 [本文引用: 1]

BACKGROUND: Peach [Prunus persica (L.) Batsch] is one of the most economically important fruit crops that, due to its genetic and biological characteristics (small genome size, taxonomic proximity to other important species and short juvenile period), has become a model plant in genomic studies of fruit trees. Our aim was an in-depth study of the extent, distribution and structure of peach genetic variation in North American and European commercial varieties as well as old Spanish varieties and several founders used in the early USA peach breeding programmes. For this we genotyped 224 peach cultivars using 50 SSRs evenly distributed along the 8 linkage groups of the Prunus reference map. RESULTS: Genetic distance analysis based on SSRs divided the peach cultivars in three main groups based mainly on their fruit characteristics: melting flesh peaches, melting flesh nectarines and non-melting varieties. Whereas non-melting flesh peaches had a higher number of alleles than melting peaches and nectarines, they were more homozygous. With some exceptions ('Admiral Dewey', 'Early Crawford' and 'Chinese Cling'), the founder US cultivars clustered together with the commercial melting peaches, indicating that their germplasm is well represented in modern cultivars. Population structure analysis showed a similar subdivision of the sample into subpopulations. Linkage disequilibrium (LD) analysis in three unstructured, or barely structured, subpopulations revealed a high level of LD conservation in peach extending up to 13-15 cM. CONCLUSIONS: Using a much larger set of SSRs, our results confirm previous observations on peach variability and population structure and provide additional tools for breeding and breeders' rights enforcement. SSR data are also used for the estimation of marker mutation rates and allow pedigree inferences, particularly with founder genotypes of the currently grown cultivars, which are useful to understand the evolution of peach as a crop. Results on LD conservation can be explained by the self-pollinating nature of peach cultivated germplasm and by a bottleneck that occurred at the beginning of modern breeding practices. High LD suggests that the development of whole-genome scanning approaches is suitable for genetic studies of agronomically important traits in peach.
DOI:10.1038/ng.2586URLPMID:23525075 [本文引用: 1]

Rosaceae is the most important fruit-producing clade, and its key commercially relevant genera (Fragaria, Rosa, Rubus and Prunus) show broadly diverse growth habits, fruit types and compact diploid genomes. Peach, a diploid Prunus species, is one of the best genetically characterized deciduous trees. Here we describe the high-quality genome sequence of peach obtained from a completely homozygous genotype. We obtained a complete chromosome-scale assembly using Sanger whole-genome shotgun methods. We predicted 27,852 protein-coding genes, as well as noncoding RNAs. We investigated the path of peach domestication through whole-genome resequencing of 14 Prunus accessions. The analyses suggest major genetic bottlenecks that have substantially shaped peach genome diversity. Furthermore, comparative analyses showed that peach has not undergone recent whole-genome duplication, and even though the ancestral triplicated blocks in peach are fragmentary compared to those in grape, all seven paleosets of paralogs from the putative paleoancestor are detectable.
[本文引用: 1]
DOI:10.1111/tpj.12234URLPMID:23663106 [本文引用: 1]

Trees are capable of tremendous architectural plasticity, allowing them to maximize their light exposure under highly competitive environments. One key component of tree architecture is the branch angle, yet little is known about the molecular basis for the spatial patterning of branches in trees. Here, we report the identification of a candidate gene for the br mutation in Prunus persica (peach) associated with vertically oriented growth of branches, referred to as 'pillar' or 'broomy'. Ppa010082, annotated as hypothetical protein in the peach genome sequence, was identified as a candidate gene for br using a next generation sequence-based mapping approach. Sequence similarity searches identified rice TAC1 (tiller angle control 1) as a putative ortholog, and we thus named it PpeTAC1. In monocots, TAC1 is known to lead to less compact growth by increasing the tiller angle. In Arabidopsis, an attac1 mutant showed more vertical branch growth angles, suggesting that the gene functions universally to promote the horizontal growth of branches. TAC1 genes belong to a gene family (here named IGT for a shared conserved motif) found in all plant genomes, consisting of two clades: one containing TAC1-like genes; the other containing LAZY1, which contains an EAR motif, and promotes vertical shoot growth in Oryza sativa (rice) and Arabidopsis through influencing polar auxin transport. The data suggest that IGT genes are ancient, and play conserved roles in determining shoot growth angles in plants. Understanding how IGT genes modulate branch angles will provide insights into how different architectural growth habits evolved in terrestrial plants.
DOI:10.1073/pnas.1704515115URLPMID:29712856 [本文引用: 1]

Plant shoots typically grow upward in opposition to the pull of gravity. However, exceptions exist throughout the plant kingdom. Most conspicuous are trees with weeping or pendulous branches. While such trees have long been cultivated and appreciated for their ornamental value, the molecular basis behind the weeping habit is not known. Here, we characterized a weeping tree phenotype in Prunus persica (peach) and identified the underlying genetic mutation using a genomic sequencing approach. Weeping peach tree shoots exhibited a downward elliptical growth pattern and did not exhibit an upward bending in response to 90 degrees reorientation. The causative allele was found to be an uncharacterized gene, Ppa013325, having a 1.8-Kb deletion spanning the 5' end. This gene, dubbed WEEP, was predominantly expressed in phloem tissues and encodes a highly conserved 129-amino acid protein containing a sterile alpha motif (SAM) domain. Silencing WEEP in the related tree species Prunus domestica (plum) resulted in more outward, downward, and wandering shoot orientations compared to standard trees, supporting a role for WEEP in directing lateral shoot growth in trees. This previously unknown regulator of branch orientation, which may also be a regulator of gravity perception or response, provides insights into our understanding of how tree branches grow in opposition to gravity and could serve as a critical target for manipulating tree architecture for improved tree shape in agricultural and horticulture applications.
DOI:10.1111/nph.13772URLPMID:26639453 [本文引用: 1]

Little is known about the genetic factors controlling tree size and shape. Here, we studied the genetic basis for a recessive brachytic dwarfism trait (dw) in peach (Prunus persica) that has little or no effect on fruit development. A sequencing-based mapping strategy positioned dw on the distal end of chromosome 6. Further sequence analysis and fine mapping identified a candidate gene for dw as a non-functional allele of the gibberellic acid receptor GID1c. Expression of the two GID1-like genes found in peach, PpeGID1c and PpeGID1b, was analyzed. GID1c was predominantly expressed in actively growing vegetative tissues, whereas GID1b was more highly expressed in reproductive tissues. Silencing of GID1c in plum via transgenic expression of a hairpin construct led to a dwarf phenotype similar to that of dw/dw peaches. In general, the degree of GID1c silencing corresponded to the degree of dwarfing. The results suggest that PpeGID1c serves a primary role in vegetative growth and elongation, whereas GID1b probably functions to regulate gibberellic acid perception in reproductive organs. Modification of GID1c expression could provide a rational approach to control tree size without impairing fruit development.
[本文引用: 1]
[本文引用: 1]
URLPMID:26850878 [本文引用: 1]
[本文引用: 1]
DOI:10.1371/journal.pone.0090574URLPMID:24595269 [本文引用: 1]

Nectarines play a key role in peach industry; the fuzzless skin has implications for consumer acceptance. The peach/nectarine (G/g) trait was described as monogenic and previously mapped on chromosome 5. Here, the position of the G locus was delimited within a 1.1 cM interval (635 kb) based on linkage analysis of an F2 progeny from the cross 'Contender' (C, peach) x 'Ambra' (A, nectarine). Careful inspection of the genes annotated in the corresponding genomic sequence (Peach v1.0), coupled with variant discovery, led to the identification of MYB gene PpeMYB25 as a candidate for trichome formation on fruit skin. Analysis of genomic re-sequencing data from five peach/nectarine accessions pointed to the insertion of a LTR retroelement in exon 3 of the PpeMYB25 gene as the cause of the recessive glabrous phenotype. A functional marker (indelG) developed on the LTR insertion cosegregated with the trait in the CxA F2 progeny and was validated on a broad panel of genotypes, including all known putative donors of the nectarine trait. This marker was shown to efficiently discriminate between peach and nectarine plants, indicating that a unique mutational event gave rise to the nectarine trait and providing a useful diagnostic tool for early seedling selection in peach breeding programs.
DOI:10.1093/jxb/erv400URLPMID:26307136 [本文引用: 1]

High concentrations of indole-3-acetic acid (IAA) are required for climacteric ethylene biosynthesis to cause fruit softening in melting flesh peaches at the late ripening stage. By contrast, the fruits of stony hard peach cultivars do not soften and produce little ethylene due to the low IAA concentrations. To investigate the regulation of IAA accumulation during peach ripening [the transition from stage S3 to stage S4 III (climacteric)], a digital gene expression (DGE) analysis was performed. The expression patterns of auxin-homeostasis-related genes were compared in fruits of the melting flesh peach 'Goldhoney 3' and the stony hard flesh peach 'Yumyeong' during the ripening stage. It is revealed here that a YUCCA flavin mono-oxygenase gene (PpYUC11, ppa008176m), a key gene in auxin biosynthesis, displayed an identical differential expression profile to the profiles of IAA accumulation and PpACS1 transcription: the mRNA transcripts increased at the late ripening stage in melting flesh peaches but were below the limit of detection in mature fruits of stony hard peaches. In addition, the strong association between intron TC microsatellite genotypes of PpYUC11 and the flesh texture (normal or stony hard) is described in 43 peach varieties, indicating that this locus may be responsible for the stony hard phenotype in peach. These findings support the hypothesis that PpYUC11 may play an essential role in auxin biosynthesis during peach fruit ripening and is a candidate gene for the control of the stony hard phenotype in peach.
DOI:10.1007/s11295-013-0649-1URL [本文引用: 1]
DOI:10.1038/nrg3374URLPMID:23247435 [本文引用: 1]

For decades, transposable elements have been known to produce a wide variety of changes in plant gene expression and function. This has led to the idea that transposable element activity has played a key part in adaptive plant evolution. This Review describes the kinds of changes that transposable elements can cause, discusses evidence that those changes have contributed to plant evolution and suggests future strategies for determining the extent to which these changes have in fact contributed to plant adaptation and evolution. Recent advances in genomics and phenomics for a range of plant species, particularly crops, have begun to allow the systematic assessment of these questions.
DOI:10.1126/science.1095011URLPMID:15143274 [本文引用: 1]
[本文引用: 1]
DOI:10.1105/tpc.111.095232URLPMID:22427337 [本文引用: 1]

Traditionally, Sicilian blood oranges (Citrus sinensis) have been associated with cardiovascular health, and consumption has been shown to prevent obesity in mice fed a high-fat diet. Despite increasing consumer interest in these health-promoting attributes, production of blood oranges remains unreliable due largely to a dependency on cold for full color formation. We show that Sicilian blood orange arose by insertion of a Copia-like retrotransposon adjacent to a gene encoding Ruby, a MYB transcriptional activator of anthocyanin production. The retrotransposon controls Ruby expression, and cold dependency reflects the induction of the retroelement by stress. A blood orange of Chinese origin results from an independent insertion of a similar retrotransposon, and color formation in its fruit is also cold dependent. Our results suggest that transposition and recombination of retroelements are likely important sources of variation in Citrus.
DOI:10.1016/S1673-8527(08)60098-3URLPMID:19232310 [本文引用: 1]

Green-revertible albino is a novel type of chlorophyll deficiency in rice (Oryza sativa L.), which is helpful for further research in chlorophyll synthesis and chloroplast development to illuminate their molecular mechanism. In the previous study, we had reported a single recessive gene, gra(t), controlling this trait on the long arm of chromosome 2. In this paper, we mapped the gra(t) gene using 1,936 recessive individuals with albino phenotype in the F(2) population derived from the cross between themo-photoperiod-sensitive genic male-sterile (T/PGMS) line Pei'ai 64S and the spontaneous mutant Qiufeng M. Eventually, it was located to a confined region of 42.4 kb flanked by two microsatellite markers RM2-97 and RM13553. Based on the annotation results of RiceGAAS system, 11 open reading frames (ORFs) were predicted in this region. Among them, ORF6 was the most possible gene related to chloroplast development, which encoded the chloroplast protein synthesis elongation factor Tu in rice. Therefore, we designated it as the candidate gene of gra(t). Sequence analysis indicated that only one base substitution C to T occurred in the coding region, which caused a missense mutation (Thr to Ile) in gra(t) mutant. These results are very valuable for further study on gra(t) gene.
DOI:10.1186/s12284-019-0277-yURLPMID:30972509 [本文引用: 1]

BACKGROUND: Although embryo accounts for only 2-3% of the total weight of a rice grain, it is a good source of various nutrients for human health. Because enlarged embryo size causes increase of the amount of nutrients and bioactive compounds stored within rice grain, giant embryo mutants of rice (Oryza sativa L.) are excellent genetic resources for improving the nutritional value of rice grains. RESULTS: Three giant embryo mutants, including large embryo (le), giant embryo (ge) and super-giant embryo (ge(s)), with variable embryo size were used in this study. We investigated whether genes controlling embryo size in these mutants (le, ge and ge(s)) were allelic to each other. Although ge and ge(s) was allelic to GIANT EMBRY (GE), le was not allelic to ge and ge(s) in allelism test. The GE gene carried a unique nucleotide substitution in each of the two mutants (ge and ge(s)), resulting in non-synonymous mutations in exon 2 of GE in both mutants. However, the GE gene of the le mutant did not carry any mutation, suggesting that the enlarged embryo phenotype of le was governed by another gene. Using map-based cloning, we mapped the LE gene to the short arm of chromosome 3. The le mutant showed mild enlargement in embryo size, which resulted from an increase in the size of scutellar parenchyma cells. The LE encodes a C3HC4-type RING finger protein and was expressed to relatively high levels in seeds at a late developmental stage. Knockdown of LE expression using RNA interference increased the embryo size of rice grains, confirming the role of LE in determining the embryo size. CONCLUSION: Overall, we identified a new gene controlling embryo size in rice. Phenotypic and molecular characterization results suggest that the le mutant will serve as a valuable resource for developing new rice cultivars with large embryos and nutrient-dense grains.
DOI:10.1093/jxb/ery076URLPMID:29509915 [本文引用: 1]

A mutant line, bifurcate flower truss (bif), was recovered from a tomato genetics programme. Plants from the control line produced a mean of 0.16 branches per truss, whereas the value for bif plants was 4.1. This increase in branching was accompanied by a 3.3-fold increase in flower number and showed a significant interaction with exposure to low temperature during truss development. The control line and bif genomes were resequenced and the bif gene was mapped to a 2.01 Mbp interval on chromosome 12; all coding region polymorphisms in the interval were surveyed, and five candidate genes displaying altered protein sequences were detected. One of these genes, SlMAPK1, encoding a mitogen-activated protein (MAP) kinase, contained a leucine to stop codon mutation predicted to disrupt kinase function. SlMAPK1 is an excellent candidate for bif because knock-out mutations of an Arabidopsis orthologue MPK6 were reported to have increased flower number. An introgression browser was used to demonstrate that the origin of the bif genomic DNA at the BIF locus was Solanum galapagense and that the SlMAPK1 null mutant is a naturally occurring allele widespread only on the Galapagos Islands. This work strongly implicates SlMAPK1 as part of the network of genes controlling inflorescence branching in tomato.