

Functional Markers Reveal Genetic Variations in Wheat Improved Cultivars and Landraces from Xinjiang
JIANDa-Wei

通讯作者:
收稿日期:2017-08-3
接受日期:2018-01-8
网络出版日期:2018-01-26
版权声明:2018作物学报编辑部作物学报编辑部
基金资助:
作者简介:
-->
展开
摘要
关键词:
Abstract
Keywords:
-->0
PDF (1336KB)元数据多维度评价相关文章收藏文章
本文引用格式导出EndNoteRisBibtex收藏本文-->
小麦是新疆的主要粮食作物, 约占全省主要粮食作物种植面积的40%~60%[1]。经过几十年的努力, 新疆小麦的产量和品质在近七、八十年取得了突破性进展, 单产水平由1949年的870 kg hm-2 [2]提高到2016年的6488 kg hm-2 (http://www.feedtrade.com.cn/ yumi/yumi_china), 目前主推小麦品种大部分达到中筋水平[3]。品种推广也经历了几轮更换, 从20世纪50年代以地方品种为主[2], 到21世纪一大批优质、高产、广适性改良品种的推广, 小麦优良品种在不同时期对新疆小麦生产都发挥了重要的作用。育种是一个优异基因不断聚合和选择的过程, 这也是导致优异基因频率不断提高的原因[4]。随着育种和生产的发展, 新疆地方品种逐渐被改良品种取代, 这种品种演替是否与改良品种在主要农艺性状相关的基因上所经历的强选择压有关, 有待研究。因此, 有必要全面了解新疆改良品种与地方品种间在与适应性、品质和产量等性状相关基因上的遗传变异。
功能标记(functional marker)是依据基因序列的多态性开发的, 这些基因的不同等位变异与表型直接相关[5]。截至2012年, 已开发了96个小麦功能标记, 涉及30多个基因座93个等位变异[6]。抽穗期是小麦的重要农艺性状之一, 对小麦适应不同生态环境具有重要的作用。它主要受春化基因(vernalization, Vrn)、光周期基因(photoperiod, Ppd)和早熟性本身基因(earliness per se, Eps)[7]控制, 其中, Vrn-A1、Vrn-B1、Vrn-D1、Vrn-B3、Ppd-A1、Ppd-B1和Ppd-D1是决定春化和光周期反应的主要基因座[8,9,10,11]。“绿色革命”基因“Rht”主要包括Rht-B1和Rht-D1, 其中, 矮秆等位变异Rht-B1a和Rht-D1a被广泛用于降低小麦株高, 提高抗倒伏能力和产量[12]。小麦-黑麦T1BL·1RS易位系广泛用于产量、适应性、抗病性和其他农艺性状改良, 在小麦生产上发挥了重要作用[5]。小麦的加工品质主要与高、低分子量麦谷蛋白亚基(high- and low-molecular weight glutenin subunits)、脂肪氧化酶(lipoxygenase, LOX)活性、籽粒硬度(puroindoline b, Pinb)基因、多酚氧化酶(polyphenol oxidase, PPO)活性和黄色素含量相关基因八氢番茄红素合酶(phytoene synthase, PSY)密切相关[13,14,15,16,17,18,19]。粒重是小麦籽粒产量重要构成因素之一, 由多基因控制, 细胞壁转化酶(cell wall invertase, CWI)基因、谷氨酰胺合酶(glutamine synthetase, GS)基因、籽粒大小(grain size, GS)基因、蔗糖合酶(sucrose synthase, Sus2)基因和粒重相关(IAA-glucose hydrolase gene, TaTGW6)基因是控制粒重和籽粒大小的重要基因, 目前已经从小麦上克隆了这些基因的同源基因, 而且已有相应的功能标记[20,21,22,23,24,25,26]。
本研究比较了与适应性、品质和粒重相关基因等位变异在新疆改良品种和地方品种中的频率变化, 揭示了相关基因优异等位变异在不同育种时期频率变化趋势, 并且进行了改良品种和地方品种的遗传多样性和群体结构分析。研究结果不仅可以解释改良品种取代地方品种的原因, 而且可以为育种者提供理想的育种材料和聚合优异等位基因提供重要的参考信息。
1 材料与方法
1.1 供试品种
包括新疆小麦资源136份, 其中79份改良品种(附表1), 57份地方品种(附表2)。前者包括35份春性品种和44份冬性品种, 都是1966年至2015年育成或审定的新疆主推品种。后者包括50份春性地方品种和7份冬性地方品种。Supplementary table 1
附表1
附表179份新疆小麦育成品种基本信息
Supplementary table 1General information on 79 wheat improved cultivars from Xinjiang
编号 Code | 名称 Name | 系谱 Pedigree | 冬/春性 Winter/ spring | 来源 Origin | 审定或 育成年份 Released/ bred year |
---|---|---|---|---|---|
1 | 新冬2号 | 热依木夏/亥恩·亥德 | 冬 | 新疆农业科学院粮食作物研究所 | 1966 |
2 | 新冬5号 | 巴克甫克/北京7号 | 冬 | 新疆农业科学院粮食作物研究所 | 1969 |
3 | 新冬7号 | 新冬2号/敖萨德3号 | 冬 | 新疆农业科学院粮食作物研究所 | 1969 |
4 | 新冬14 | 热衣木夏/乌克兰83 | 冬 | 新疆农垦科学院作物研究所 | 1984 |
5 | 新冬15 | 新冬2号/中引5号 | 冬 | 新疆农业科学院粮食作物研究所 | 1989 |
6 | 新冬16 | (巴克甫克/亥恩·亥德)/70-4 | 冬 | 新疆生产建设兵团农七师农业科学研究所 | 1988 |
7 | 新冬17 | 新冬14/安选5号 | 冬 | 新疆农垦科学院作物研究所 | 1994 |
8 | 新冬18 | N.S11-33/新冬3号 | 冬 | 新疆农业科学院粮食作物研究所 | 1994 |
9 | 新冬19 | (170/阿夫乐尔)/H-矮82-6 F0种子辐照 | 冬 | 新疆农业科学院核技术生物技术研究所 | 1995 |
10 | 新冬20 | 冀875018 | 冬 | 新疆农业科学院粮食作物研究所 | 1995 |
11 | 新冬21 | 318/88122 | 冬 | 新疆农业科学院核技术生物技术研究所 | 1997 |
12 | 新冬22 | (诺斯塔/花春84-1)/(76-4/洛夫林13) | 冬 | 新疆生产建设兵团农七师农业科学研究所 | 1996 |
13 | 新冬23 | 美国引进F1分离群体/花培品系88-136 | 冬 | 新疆农业科学院核技术生物技术研究所 | 2000 |
14 | 新冬24 | 9245/冀6159 | 冬 | 新疆农业科学院粮食作物研究所 | 2003 |
15 | 新冬27 | 从中国农业大学引进高代品系系谱选择 | 冬 | 新疆农垦科学院作物研究所 | 2005 |
16 | 新冬28 | 92-45/新冬20 | 冬 | 新疆农业科学院粮食作物研究所 | 2005 |
17 | 新冬29 | PH82-2-2/鲁植79-1 | 冬 | 新疆生产建设兵团农四师农业科学研究所 | 2005 |
18 | 新冬30 | 不详 | 冬 | 新疆农垦科学院作物研究所 | 2007 |
19 | 新冬31 | (4114/新冬14/丰收)/(新冬18/中引85) | 冬 | 新疆农业科学院粮食作物研究所 | 2007 |
20 | 新冬33 | 73-13-36/82-4009 | 冬 | 石河子农业科技开发研究中心粮食油料作物研究所 | 2009 |
21 | 新冬36 | (89(813)/新冬18)/晋农207 | 冬 | 新疆农垦科学院作物研究所 | 2011 |
22 | 新冬37 | 京411/贵农15 | 冬 | 中国农业科学院作物科学研究所 | 2012 |
23 | 新冬38 | (矮秆916/9133)/伊农16 | 冬 | 新疆生产建设兵团农四师农业科学研究所 | 2011 |
24 | 新冬41 | 石冬8号/新冬22 | 冬 | 石河子农业科学研究院 | 2013 |
25 | 新冬48 | (新冬22/M844)/石冬8号 | 冬 | 石河子农业科学研究院 | 2015 |
26 | 新冬51 | (藁城8901优/新冬18)/冀5473 | 冬 | 新疆农垦科学院作物研究所 | 2015 |
27 | 新冬52 | 新冬17/95-7-13-2 | 冬 | 石河子农业科学研究院粮油作物研究所 | 2015 |
28 | 伊农16 | (白壳欧柔/72-829/K2-13)/ (72-629-7141-64/K2-13) | 冬 | 新疆生产建设兵团农四师农业科学研究所 | 1994 |
29 | 伊农18 | [(77224/R2-13)/(7113-9/76-629)]/[(运动1号/00089)/(工农10-3/72-原78)] | 冬 | 新疆生产建设兵团农四师农业科学研究所 | 1999 |
30 | 奎花1号 | 京花1号/奎冬3号 | 冬 | 新疆生产建设兵团农七师农业科学研究所 | 1991 |
31 | 石冬7号 | 87-5048/昌冬5号(76-165) | 冬 | 石河子农业科技开发研究中心 | 1999 |
32 | 石冬8号 | 昌冬5号(76-165)/82-4009 | 冬 | 石河子农业科技开发研究中心 | 2003 |
33 | 石冬9号 | 轮回选择 | 冬 | 新疆农垦科学院作物研究所 | 2003 |
34 | 喀冬1号 | 巴克甫克/亥恩·亥德 | 冬 | 喀什地区农业科学研究所 | 1972 |
35 | 喀冬4号 | 华北187系选 | 冬 | 喀什农业学校 | 1973 |
36 | 新春3号 | 西特·赛洛斯/奇春4号 F0种子辐照 | 春 | 新疆农业科学院核技术生物技术研究所 | 1985 |
37 | 新春4号 | 151/74-6//74-16/沃尔森 | 春 | 新疆农业科学院粮食作物研究所/昌吉农业科学研究所 | 1990 |
38 | 新春5号 | 繁6/6038 | 春 | 新疆农垦科学院作物研究所 | 1990 |
39 | 新春6号 | 中7906/新春2号 | 春 | 新疆农业科学院核技术生物技术研究所 | 1993 |
编号 Code | 名称 Name | 系谱 Pedigree | 冬/春性 Winter/ spring | 来源 Origin | 审定或 育成年份 Released/ bred year |
40 | 新春7号 | 中7906/新春2号 | 春 | 新疆农业科学院核技术生物技术研究所 | 1995 |
41 | 新春8号 | CO7/21-23 | 春 | 石河子大学农学院 | 1997 |
42 | 新春9号 | 引进春麦品系NS-65 | 春 | 新疆农业科学院核技术生物技术研究所 | 1999 |
43 | 新春10号 | 9-3-3/新春4号 | 春 | 新疆农业科学院粮食作物研究所 | 2002 |
44 | 新春11 | 新春2号/86-7 | 春 | 石河子大学农学院 | 2002 |
45 | 新春12 | 8021/77-13 | 春 | 新疆农业科学院粮食作物研究所 | 2003 |
46 | 新春13 | 不详 | 春 | 不详 | 2003 |
47 | 新春14 | 不详 | 春 | 新疆农业科学院核技术生物技术研究所 | 2004 |
48 | 新春15 | F5繁24/85307 | 春 | 新疆农业科学院粮食作物研究所 | 2004 |
49 | 新春16 | 86-6B/93鉴9 | 春 | 新疆农垦科学院作物研究所 | 2004 |
50 | 新春17 | 新春6号/NS64 | 春 | 新疆农业科学院核技术生物技术研究所 | 2005 |
51 | 新春18 | 不详 | 春 | 不详 | 2005 |
52 | 新春19 | 不详 | 春 | 石河子大学农学院 | 2005 |
53 | 新春20 | 昌春6号/墨西哥M85-30 | 春 | 新疆农业科学院粮食作物研究所 | 2006 |
54 | 新春21 | NS-23-3/青海946 | 春 | 新疆生产建设兵团农五师农业科学研究所 | 2006 |
55 | 新春22 | Tal/永1265 | 春 | 新疆农垦科学院作物研究所; 宁夏永宁县小麦育繁所 | 2006 |
56 | 新春23 | CIMMYT引进F2/88-136 | 春 | 新疆农业科学院核技术生物技术研究所 | 2006 |
57 | 新春24 | 不详 | 春 | 不详 | 2006 |
58 | 新春25 | 不详 | 春 | 新疆农业科学院奇台春小麦试验场 | 2006 |
59 | 新春26 | 新春9号/新春6号 | 春 | 新疆农业科学院核技术生物技术研究所 | 2007 |
60 | 新春27 | 91I82299/21-4 | 春 | 新疆农业科学院粮食作物研究所 | 2007 |
61 | 新春28 | CIMMYT引进, 原代号为01-25 | 春 | 新疆农垦科学院作物研究所 | 2007 |
62 | 新春29 | 85-56/25-3 | 春 | 新疆农业科学院粮食作物研究所 | 2008 |
63 | 新春30 | 新春9号/新春6号 | 春 | 新疆农业科学院核技术生物技术研究所 | 2009 |
64 | 新春31 | 12-25/96-5 | 春 | 石河子大学农学院 | 2009 |
65 | 新春32 | 97-18/永良11 | 春 | 新疆生产建设兵团农五师农业科学研究所 | 2009 |
66 | 新春33 | 新春9号/新春6号 | 春 | 新疆农业科学院核技术生物技术研究所 | 2010 |
67 | 新春34 | 88(13)/5×44 | 春 | 新疆农业科学院粮食作物研究所 | 2011 |
68 | 新春35 | 巴96-4870/93鉴29 | 春 | 新疆农垦科学院作物研究所 | 2011 |
69 | 新春36 | 21-6/黑小麦 | 春 | 新疆生产建设兵团农十三师农业科学研究所 | 2011 |
70 | 新春38 | 原212/97-46-3 | 春 | 新疆农垦科学院作物研究所与新疆九禾种业有限责任公司 | 2012 |
71 | 宁春23 | (宁春4号/中7906)/陕农7855 | 春 | 宁夏农林科学院作物科学研究所 | 1995 |
72 | 宁春32 | 建三江- 6918/高代品系1658 | 春 | 宁夏农林科学院作物科学研究所 | 2002 |
73 | 宁春35 | 宁春16/永A71 | 春 | 宁夏农林科学院作物科学研究所 | 2003 |
74 | 宁春37 | 从南非引进 | 春 | 宁夏农林科学院作物科学研究所 | 2005 |
75 | 昌春6号 | (82A3/021)/(78A131/03312) | 春 | 新疆农业科学院奇台春小麦试验场 | 1994 |
76 | 抗旱1号 | H101/C8501 | 春 | 新疆生产建设兵团农四师农业科学研究所 | 2013 |
77 | 青春5号 | 阿勃/欧柔 | 春 | 青海省农业科学研究院 | 1969 |
78 | 新曙光1号 | 阿勃/欧柔 | 春 | 黑龙江省农业科学研究院 | 1971 |
79 | 吐春9号 | (80B63/02126)/(77A7/834) | 春 | 新疆农业科学院奇台春小麦试验场 | 1998 |
新窗口打开
Supplementary table 2
附表2
附表257份新疆小麦地方品种基本信息
Supplementary table 2General information on 57 wheat landraces from Xinjiang
编号 Code | 名称 Name | 冬/春性 Winter/spring | 来源 Origin | 编号 Code | 名称 Name | 冬/春性 Winter/spring | 来源 Origin |
---|---|---|---|---|---|---|---|
80 | 库车白冬麦 | 冬 | 新疆库车 | 109 | 直芒 | 春 | 新疆焉耆 |
81 | 白冬麦 | 冬 | 新疆呼图壁 | 110 | 佛手麦 | 春 | 新疆库车 |
82 | 小白冬麦 | 冬 | 新疆呼图壁 | 111 | 稻麦子 | 春 | 新疆乌什 |
83 | 阿克库孜盖 | 冬 | 新疆库尔勒 | 112 | 高拉山春麦 | 春 | 新疆疏附 |
84 | 热衣木夏 | 冬 | 新疆新和 | 113 | 白芒红麦 | 春 | 新疆叶城 |
85 | 长巴什曼 | 冬 | 新疆叶城 | 114 | 古玛尔汗 | 春 | 新疆墨玉 |
86 | 纳瓦提然 | 冬 | 新疆新和 | 115 | 克拉黑麦 | 春 | 新疆墨玉 |
87 | 阿克脱哈尔 | 春 | 新疆哈巴河 | 116 | 阿及麦 | 春 | 新疆墨玉 |
88 | 其力克 | 春 | 新疆洛浦 | 117 | 白吐里克 | 春 | 新疆墨玉 |
89 | 黑芒 | 春 | 新疆米泉 | 118 | 西藏稻麦子 | 春 | 新疆洛浦 |
90 | 白光头 | 春 | 新疆昌吉 | 119 | 其力克 | 春 | 新疆洛浦 |
91 | 红春麦 | 春 | 新疆昌吉 | 120 | 买甚春麦 | 春 | 新疆于田 |
92 | 木锨棒 | 春 | 新疆昌吉 | 121 | 古尔汗满 | 春 | 新疆于田 |
93 | 疙瘩头 | 春 | 新疆昌吉 | 122 | 阿克禾孜干 | 春 | 新疆于田 |
94 | 金包银 | 春 | 新疆昌吉 | 123 | 吐里克 | 春 | 新疆和田 |
95 | 兰麦 | 春 | 新疆昌吉 | 124 | 克兹买克 | 春 | 新疆和田 |
96 | 黑芒春 | 春 | 新疆米泉 | 125 | 米麦米尔 | 春 | 新疆和田 |
97 | 白春麦 | 春 | 新疆玛纳斯 | 126 | 大头郞 | 春 | 新疆哈密 |
98 | 无芒麦 | 春 | 新疆鄯善 | 127 | 小白芒(白) | 春 | 新疆青河 |
99 | 分枝麦 | 春 | 新疆哈密 | 128 | 小白芒(红) | 春 | 新疆青河 |
100 | 大白麦 | 春 | 新疆哈密 | 129 | 小红芒1 | 春 | 新疆青河 |
101 | 黄库班克 | 春 | 新疆伊宁 | 130 | 小红芒2 | 春 | 新疆青河 |
102 | 比热巴沙尔 | 春 | 新疆霍城 | 131 | 小红麦 | 春 | 新疆巴里坤 |
103 | 波兰麦 | 春 | 新疆吐鲁番 | 132 | 托力克 | 春 | 新疆和田 |
104 | 黑头麦 | 春 | 新疆塔城 | 133 | 金包银(红) | 春 | 新疆伊吾 |
105 | 分枝黑芒 | 春 | 新疆塔城 | 134 | 金包银(白) | 春 | 新疆伊吾 |
106 | 良山麦子 | 春 | 新疆阿勒泰 | 135 | 红穗无芒 | 春 | 新疆阿勒泰 |
107 | 黑库班克 | 春 | 新疆阿勒泰 | 136 | 阿克贾克 | 春 | 新疆和田 |
108 | 兰壳兰芒 | 春 | 新疆阿勒泰 |
新窗口打开
1.2 分子标记检测
取每份供试材料10粒种子放在带有湿润试纸的培养皿中发芽, 室温下生长5 d, 然后从每份材料各个植株上取等量叶片混合用于DNA提取[27]。利用52个与适应性、品质和粒重相关的功能标记对供试材料进行等位变异分析。适应性相关的基因包括Rht (Rht-B1和Rht-D1)[12]、VRN (Vrn-A1、Vrn-B1、Vrn-D1和Vrn-B3)[8-9,28]、Ppd (Ppd-A1、Ppd-B1和Ppd-D1)[10,11]和T1BL·1RS易位系(sec-1)[29]; 与品质相关的基因包括Glu-D1 (Dx5+Dy10)[13]、Lox (TaLox-B1)[14]、Pin (Pinb-D1)[15]、Ppo (Ppo-A1)[17]和Psy (Psy-A1和Psy-B1)[18,19]; 粒重相关基因主要包括CWI (TaCwi-A1、TaCWI-4A和TaCWI-5D)[20,21]、GS1 (TaGS1a)[22]、GS3 (TaGS-D1)[23]和GS5 (TaGS5-A1)[24]、Sus-2 (TaSus2-2B)[25]和TGW6 (TaTGW6-A1)[26]。
PCR在Biometra Thermocycler (Germany)上进行, 总反应体系为20 μL, 包括2 × Taq PCR Master Mix 10 μL, 正反引物(10 μmol L-1)各1 μL, DNA模板(50~100 ng μL-1) 2 μL。PCR程序为94°C预变性5 min, 94°C变性30 s, 57~60°C退火30 s, 72°C延伸1~3 min, 72°C后延伸10 min。PCR扩增产物经2%琼脂糖凝胶电泳检测, 溴化乙锭染色后用凝胶成像系统观察电泳结果, 读取基因型。需要说明的是对于粒重相关基因座TaCWI-4A、TaCWI-5D、TaGS1a、TaGS5-A1和TaTGW6-A1在PCR扩增的基础上, 分别利用限制性内切酶Tai I、BstY I、EcoR I、Bbv I和Dpn II进行酶切, 用2%琼脂糖凝胶电泳检测酶切产物。
1.3 统计分析
采用PowerMarker v3.25软件[30]分析每个基因座等位基因数目、遗传多样性指数和多态性信息含量(PIC)。利用Arlequin v3.5软件[31]对亚组间(改良品种和地方品种)、亚组内个体间以及个体间进行分子方差分析(analysis of molecular variance, AMOVA)。通过NTSYS-pc v2.1软件[32]对供试材料进行基于主成分(PCA)的群体结构分析。为了比较不同时期新疆小麦品种基因频率变化趋势, 按照品种的育成或审定时间将136个小麦资源分成6组, 即地方品种(57份)、1966—1990年(13份)、1991—2000年(18份)、2001—2005年(19份)、2006—2010年(17份)和2011—2015年(12份); 冬性品种和春性品种也分别按上述原则分为6组, 冬性品种各组依次为7、8、11、6、3和7份, 春性品种各组依次为50、5、7、13、14和5份。通过简单线性回归方程y = a + bx分析优异等位变异频率分别在所有供试品种、冬性和春性品种不同时期的变化趋势, 其中, y表示因变量, x表示自变量, a表示回归截距, b表示回归系数。用回归系数b的大小来反映变化趋势, 具体参考Meng等[4]的方法进行回归分析。
2 结果与分析
2.1 适应性、品质和粒重性状基因不同等位变异分布频率
利用52个功能标记对136份新疆小麦资源进行等位变异分型, 部分引物扩增结果见图1。从这些基因不同等位变异分布频率看, 无论是冬性或春性资源, 与适应性相关的矮秆等位变异Rht-B1b和Rht-D1b、光周期不敏感等位变异Ppd-D1a以及T1BL·1RS易位系在改良品种中的分布频率均高于地方品种(表1)。与冬性生长习性密切相关的隐性等位变异vrn-B1、vrn-D1和vrn-B3主要分布在改良品种中; 与春性生长习性相关的显性等位变异Vrn-A1在改良品种中分布频率也明显高于地方品种。矮秆等位基因组合Rht-B1b+Rht-D1b (5.1%)仅在改良品种中出现且在春性改良品种中分布频率更高。相反, 高秆等位变异组合Rht-B1a+Rht-D1a主要分布在地方品种中, 频率高达98.2%, 且这一等位变异组合在冬性地方品种频率高于春性地方品种(图2-A)。春性改良品种的Rht-D1b和Ppd-D1a等位变异以及T1BL·1RS易位系的分布频率高于冬性改良品种。相反, 冬性改良品种Rht-B1b等位变异分布频率高于春性改良品种。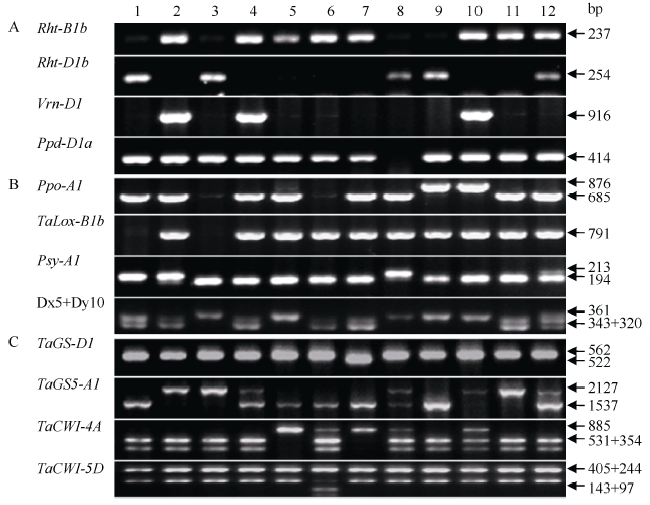
图1部分适应性(A)、品质(B)和粒重(C)性状基因在12个新疆小麦品种中的扩增结果
1: 新春3号; 2: 新春4号; 3: 新春5号; 4: 新春6号; 5: 新春7号; 6: 新春8号; 7: 新春9号; 8: 新春10号; 9: 新春11; 10: 新春12; 11: 新春13; 12: 新春14。
-->Fig. 1PCR products of partial genes associated with adaptation (A), quality (B), and kernel weight (C) in 12 Xinjiang wheat cultivars
1: Xinchun 3; 2: Xinchun 4; 3: Xinchun 5; 4: Xinchun 6; 5: Xinchun 7; 6: Xinchun 8; 7: Xinchun 9; 8: Xinchun 10; 9: Xinchun 11; 10: Xinchun 12; 11: Xinchun 13; 12: Xinchun 14.
-->
Table 1
表1
表1新疆改良品种和地方品种与适应性、品质和粒重性状相关基因等位变异分布频率比较
Table 1Comparison of allele frequencies at the loci associated with adaptation, quality and kernel weight in the wheat cultivars and the landraces from Xinjiang
基因 Gene | 基因座 Locus | 等位基因 Allele | 全部All | 冬性Winter | 春性Spring | |||
---|---|---|---|---|---|---|---|---|
改良品种 Improved cultivar | 地方品种 Landrace | 改良品种 Improved cultivar | 地方品种 Landrace | 改良品种 Improved cultivar | 地方品种 Landrace | |||
适应性 Adaptation | ||||||||
Rht | Rht-B1 | Rht-B1a | 20.3 | 100.0 | 16.1 | 100.0 | 23.3 | 100.0 |
Rht-B1b | 79.7 | 0 | 83.9 | 0 | 76.7 | 0 | ||
Rht-D1 | Rht-D1a | 84.8 | 100.0 | 91.4 | 100.0 | 79.5 | 100.0 | |
Rht-D1b | 15.2 | 0 | 8.6 | 0 | 20.5 | 0 | ||
VRN | Vrn-A1 | Vrn-A1 | 39.2 | 5.8 | 0 | 0 | 70.5 | 93.3 |
vrn-A1 | 60.8 | 94.2 | 100.0 | 100.0 | 29.5 | 6.7 | ||
Vrn-B1 | Vrn-B1 | 39.2 | 44.0 | 0 | 0 | 70.5 | 47.8 | |
vrn-B1 | 60.8 | 56.0 | 100.0 | 100.0 | 29.5 | 52.2 | ||
Vrn-D1 | Vrn-D1a | 20.5 | 80.0 | 0.0 | 0.0 | 37.2 | 93.0 | |
Vrn-D1b | 1.7 | 0 | 2.9 | 0 | 0 | 0 | ||
vrn-D1 | 78.2 | 20.0 | 97.1 | 100.0 | 62.8 | 7.0 | ||
Vrn-B3 | Vrn-B3 | 0 | 0 | 0 | 0 | 0 | 0 | |
vrn-B3 | 100.0 | 100.0 | 100.0 | 100.0 | 100.0 | 100.0 | ||
Ppd | Ppd-A1 | Ppd-A1a | 100.0 | 100.0 | 100.0 | 100.0 | 100.0 | 100.0 |
Ppd-A1b | 0 | 0 | 0 | 0 | 0 | 0 | ||
Ppd-B1 | Ppd-B1a | 0 | 0 | 0 | 0 | 0 | 0 | |
基因 Gene | 基因座 Locus | 等位基因 Allele | 全部All | 冬性Winter | 春性Spring | |||
改良品种 Improved cultivar | 地方品种 Landrace | 改良品种 Improved cultivar | 地方品种 Landrace | 改良品种 Improved cultivar | 地方品种 Landrace | |||
Ppd-B1b | 100.0 | 100.0 | 100.0 | 100.0 | 100.0 | 100.0 | ||
Ppd-D1 | Ppd-D1a | 77.6 | 4.1 | 55.9 | 0 | 95.1 | 4.8 | |
Ppd-D1b | 22.4 | 95.9 | 44.1 | 100.0 | 4.9 | 95.2 | ||
T1BL·1RS | Sec-1 | T1BL·1RS | 16.5 | 0 | 11.4 | 0 | 20.5 | 0 |
Non T1BL·1RS | 83.5 | 100.0 | 88.6 | 100.0 | 79.5 | 100.0 | ||
品质 Quality | ||||||||
Glu-1 | Glu-D1 | Dx5+Dy10 | 35.4 | 1.8 | 22.9 | 16.7 | 45.5 | 0 |
Dx2+Dy12 | 64.6 | 98.2 | 77.1 | 83.3 | 54.5 | 100.0 | ||
Lox | TaLox-B1 | TaLox-B1a | 20.0 | 0 | 37.1 | 0 | 5.0 | 0 |
TaLox-B1b | 80.0 | 100.0 | 62.9 | 100.0 | 95.0 | 100.0 | ||
Pin | Pinb-D1 | Pinb-D1a | 75.0 | 91.7 | 56.3 | 50.0 | 91.7 | 97.6 |
Pinb-D1b | 25.0 | 8.3 | 43.8 | 50.0 | 8.3 | 2.4 | ||
Ppo | Ppo-A1 | Ppo-A1a | 78.1 | 48.6 | 60.9 | 50.0 | 88.1 | 48.5 |
Ppo-A1b | 21.9 | 51.4 | 39.1 | 50.0 | 11.9 | 51.5 | ||
Ppo-D1 | Ppo-D1a | 3.7 | 0 | 0 | 0 | 6.7 | 0 | |
Ppo-D1b | 96.3 | 100.0 | 100.0 | 100.0 | 93.3 | 100.0 | ||
Psy | Psy-A1 | Psy-A1a | 89.6 | 100.0 | 100.0 | 100.0 | 82.6 | 100.0 |
Psy-A1b | 10.4 | 0 | 0 | 0 | 17.4 | 0 | ||
Psy-B1 | Psy-B1c | 58.8 | 55.0 | 100.0 | 100.0 | 30.0 | 52.6 | |
Psy-B1d | 41.2 | 45.0 | 0 | 0 | 70.0 | 47.4 | ||
Psy1-D1 | Psy1-D1a | 84.4 | 55.6 | 80.0 | 100.0 | 88.1 | 48.9 | |
Psy1-D1g | 15.6 | 44.4 | 20.0 | 0 | 11.9 | 51.1 | ||
粒重 Kernel weight | ||||||||
CWI | TaCwi-A1 | TaCwi-A1a | 63.3 | 22.8 | 40.0 | 14.3 | 81.8 | 24.0 |
TaCwi-A1b | 36.7 | 77.2 | 60.0 | 85.7 | 18.2 | 76.0 | ||
TaCWI-4A | Hap-4A-C | 66.2 | 83.7 | 54.5 | 66.7 | 77.1 | 86.0 | |
Hap-4A-T | 33.8 | 16.3 | 45.5 | 33.3 | 22.9 | 14.0 | ||
TaCWI-5D | Hap-5D-C | 93.7 | 85.5 | 88.6 | 71.4 | 97.7 | 87.5 | |
Hap-5D-G | 6.3 | 14.5 | 11.4 | 28.6 | 2.3 | 12.5 | ||
GS1 | TaGS1a | Hap-1 | 22.1 | 39.0 | 40.7 | 0.0 | 9.8 | 42.1 |
Hap-2 | 77.9 | 61.0 | 59.3 | 100.0 | 90.2 | 57.9 | ||
GS3 | TaGS-D1 | TaGS-D1a | 78.5 | 64.0 | 80.0 | 57.1 | 77.3 | 65.1 |
TaGS-D1b | 21.5 | 36.0 | 20.0 | 42.9 | 22.7 | 34.9 | ||
GS5 | TaGS5-A1 | TaGS5-A1a | 50.0 | 88.7 | 39.3 | 83.3 | 58.3 | 89.4 |
TaGS5-A1b | 50.0 | 11.3 | 60.7 | 16.7 | 41.7 | 10.6 | ||
Sus-2 | TaSus2-2B | Hap-L | 86.8 | 100.0 | 66.7 | 100.0 | 100.0 | 100.0 |
Hap-H | 13.2 | 0 | 33.3 | 0 | 0 | 0 | ||
TGW6 | TaTGW6-A1 | TaTGW6-A1a | 92.1 | 55.1 | 87.9 | 20.0 | 95.3 | 59.1 |
TaTGW6-A1b | 7.9 | 44.9 | 12.1 | 80.0 | 4.7 | 40.9 |
新窗口打开
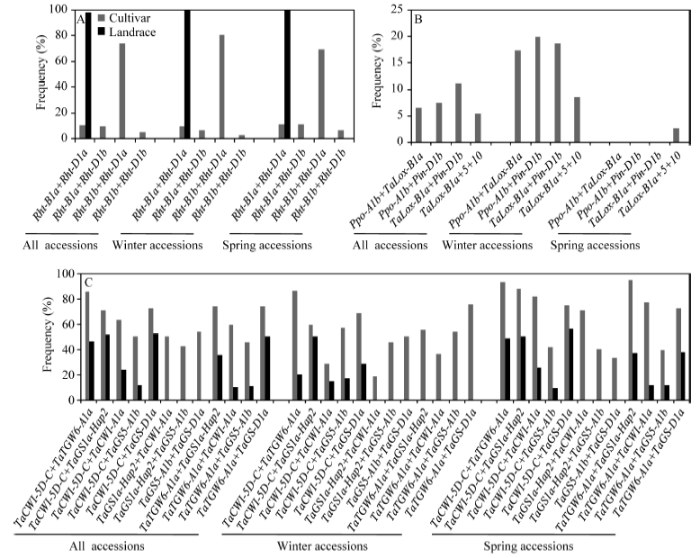
图2影响株高(A)、品质(B)和粒重(C)基因优异等位变异组合在新疆改良品种和地方品种中分布频率
-->Fig. 2Frequencies of favorable allele combinations at the loci associated with plant height (A), quality (B), and kernel weight (C) in the improved cultivars and the landraces from Xinjiang
-->
与品质相关的优质麦谷蛋白亚基Dx5+Dy10、面粉色泽相关的高脂肪氧化酶活性等位变异TaLox-B1a、硬质等位变异Pin-D1b、低多酚氧化酶活性等位变异Ppo-D1a和低黄色素含量等位变异Psy-A1b在全部、冬性和春性改良品种中的分布频率均高于相应的地方品种(表1), 而且只在改良品种中检测到优异等位变异组合Ppo-A1b+TaLox-B1a、Ppo-A1b+Pin-D1b、TaLox-B1a+Pin-D1b和TaLox- B1a+Dx5+Dy10。冬性改良品种TaLox-B1a和Pin-D1b的频率高于春性改良品种; 相反, 春性改良品种Dx5+Dy10、Ppo-D1a和Psy-A1b等位变异的频率高于冬性改良品种, 而且只在春性改良品种中检测到Ppo-D1a和Psy-A1b等位变异。值得注意的是, 除了TaLox-B1a+Dx5+Dy10外, 其他3个优异等位变异组合仅分布于冬性改良品种(图2-B)。
在粒重相关的8个基因座中, 高粒重等位变异TaCwi-A1a、Hap-4A-T (Tacwi-4A)、Hap-5D-C (TaCWI- 5D)、Hap-2 (TaGS1a)、TaGS-D1a、TaGS5-A1b、Hap-H (TaSus-2B)和TaTGW6-A1a在改良品种中的分布频率均高于地方品种(表1)。在14对高粒重等位变异组合中, TaCWI-5D-C+TaTGW-A1a、TaCWI-5D-C+ TaGS-D1a、TaTGW6-A1a+TaGS1a-Hap2和TaTGW6- A1a+TaGS-D1a在改良品种中频率明显高于地方品种, 分别达到82.3%、72.2%、63.3%和70.9% (图2-C)。冬性改良品种高粒重等位变异组合多于春性改良品种。
2.2 适应性、品质和粒重性状基因优异等位变异在不同育种时期的频率变化
在与适应性、品质和粒重性状相关的17个优异等位变异中, 有11个分布频率随育种时期推进呈现不连续性上升的趋势(图3)。矮秆等位变异Rht-B1b在地方品种1991—2000年期间呈现连续上升趋势, 2000年频率达到94.1%; 2001—2005年期间频率下降到73.9%, 2005年以后保持平稳(图3-A)。在冬性改良品种中, Rht-B1b分布频率在2001—2010年期间呈现下降趋势, 2011年后又出现上升趋势; 而春性改良品种变化趋势与所有品种的变化趋势一致。除了在2006—2010期间, 等位变异Rht-D1b分布频率随着育种时期推进呈明显上升趋势(图3-B)。T1BL·1RS易位系仅分布在改良品种中, 在地方品种1991—2000年期间无论是冬性或春性改良品种, 其易位系分布频率均随育种时期推进呈现上升趋势, 2000年以后呈现下降趋势(图3-C)。与面粉色泽相关的高脂肪氧化酶活性等位变异TaLox-B1a分布频率随育种时期推进显著提高, 特别是冬性改良品种呈直线上升趋势, 回归系数达到15.0 (P < 0.01), 且R2达到0.96 (P < 0.01)(图3-D)。优质麦谷蛋白亚基Dx5+Dy10分布频率随育种时期推进呈现不连续的上升趋势, 但在2011年以后的改良品种中出现下降趋势(图3-E)。高千粒重等位变异TaGS-D1a (图3-F)、hap-h (TaSus2-2B) (图3-G)、TaCwi-A1a (图3-H)、TaCWI-4A (图3-I)、TaCWI-5D (图3-J)和TaTGW6-A1a (图3-K)分布频率在地方品种-2000年期间均呈现直线上升趋势, 随着育种时期的推进在2001年以后分布频率呈现不连续上升, 其中, 等位变异TaTGW6-A1a的分布频率在2006年以后的改良品种中达到100%。随着育种时期推进, 6个等位变异在冬、春性改良品种中的分布频率呈现不同的变化趋势, 特别是等位变异hap-h在春性改良品种中没有出现, 而在冬性改良品种中于地方品种-2010年期间呈现直线上升趋势(图3-G)。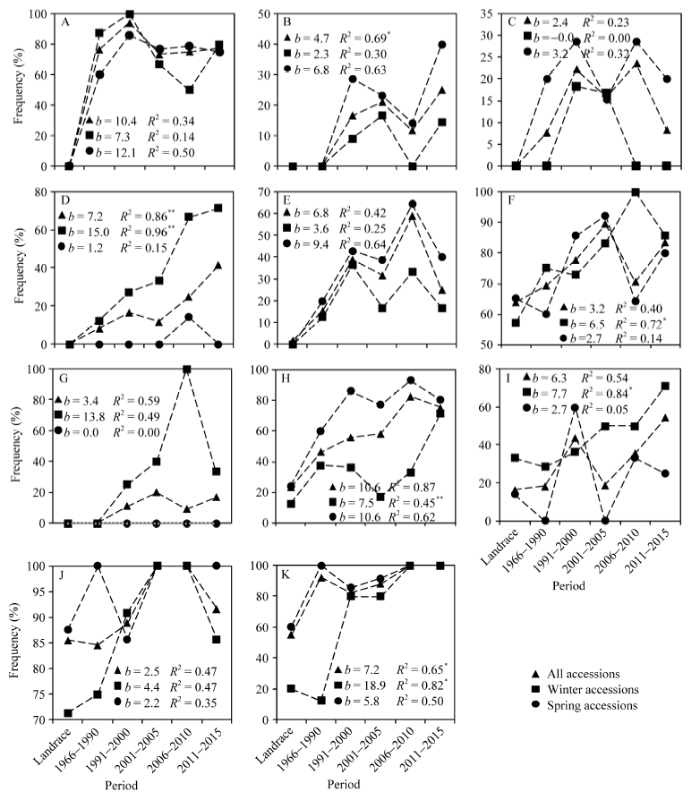
图3适应性、品质和粒重基因优异等位变异在不同育种时期频率变化
A: Rht-B1b等位变异; B: Rht-D1b等位变异; C: TlBL·1RS易位系; D: TaLox-B1a等位变异; E: Dx5+Dy10亚基; F: TaGS-D1a等位变异; G: TaSus2-2B (hap-h等位变异); H: TaCwi-A1a等位变异; I: TaCWI-4A等位变异; J: TaCWI-5D等位变异; K:TaTGW6-A1a等位变异。*和**分别表示R2在0.05和0.01概率水平显著。
-->Fig. 3Variation in the frequency of favorable alleles associated with adaptation, quality, and kernel weight in different breeding periods
A: Rht-B1b allele; B: Rht-D1b allele; C: TlBL·1RS translocation line; D: TaLox-B1a allele; E: Dx5+Dy10 subunits; F: TaGS-D1a allele; G: TaSus2-2B (hap-h allele); H: TaCwi-A1a allele; I: TaCWI-4A allele; J: TaCWI-5D allele; K: TaTGW6-A1a allele. * and ** indicate significance of R2 at the 0.05 and 0.01 probability levels, respectively.
-->
2.3 改良品种和地方品种的遗传多样性比较
除了春性资源与粒重相关基因座的多态性信息含量(PIC)平均值外, 无论是全部、冬性还是春性资源, 改良品种所有基因座的平均等位基因数目和平均PIC均高于地方品种(表2)。春性改良品种在与适应性相关以及品质相关基因座的平均等位基因数目和平均PIC高于冬性改良品种。相反, 冬性改良品种与粒重相关基因座的平均PIC高于春性改良品种。在适应性相关基因的10个基因座中, 春性改良品种的平均等位基因数目(1.70)和PIC (0.20)均高于冬性改良品种(1.50和0.10)。在品质相关基因的8个基因座中, 春性改良品种平均等位基因数目(2.00)和平均PIC (0.23)亦高于冬性改良品种(1.63和0.21)。在粒重相关基因的8个基因座中, 春性和冬性改良品种的平均等位基因数目相等, 而春性改良品种的平均PIC高于冬性改良品种。Table 2
表2
表2新疆改良品种与地方品种适应性、品质和粒重性状相关基因座等位基因数目和多态性信息含量比较
Table 2Comparison of number of alleles and polymorphic information content (PIC) at the loci associated with adaptation, quality and kernel weight in wheat cultivars and landraces from Xinjiang
基因座 Locus | 等位基因数 No. of alleles | 多态性信息量 PIC | ||||||||||
---|---|---|---|---|---|---|---|---|---|---|---|---|
改良品种 Improved cultivar | 地方品种 Landrace | 改良品种 Improved cultivar | 地方品种 Landrace | |||||||||
全部 All | 冬性 Winter | 春性 Spring | 全部 All | 冬性 Winter | 春性 Spring | 全部 All | 冬性 Winter | 春性 Spring | 全部 All | 冬性 Winter | 春性 Spring | |
适应性 Adaptation | ||||||||||||
Rht-B1 | 2 | 2 | 2 | 1 | 1 | 1 | 0.27 | 0.23 | 0.29 | 0 | 0 | 0 |
Rht-D1 | 2 | 2 | 2 | 1 | 1 | 1 | 0.22 | 0.14 | 0.27 | 0 | 0 | 0 |
Vrn-A1 | 2 | 1 | 2 | 2 | 1 | 2 | 0.36 | 0 | 0.33 | 0.10 | 0 | 0.11 |
Vrn-B1 | 2 | 1 | 2 | 2 | 1 | 2 | 0.36 | 0 | 0.33 | 0.37 | 0 | 0.37 |
Vrn-D1 | 3 | 2 | 2 | 2 | 1 | 2 | 0.29 | 0.05 | 0.36 | 0.27 | 0 | 0.12 |
Vrn-B3 | 1 | 1 | 1 | 1 | 1 | 1 | 0 | 0 | 0 | 0 | 0 | 0 |
Ppd-A1 | 1 | 1 | 1 | 1 | 1 | 1 | 0 | 0 | 0 | 0 | 0 | 0 |
Ppd-B1 | 1 | 1 | 1 | 1 | 1 | 1 | 0 | 0 | 0 | 0 | 0 | 0 |
Ppd-D1 | 2 | 2 | 2 | 2 | 1 | 2 | 0.29 | 0.37 | 0.11 | 0.09 | 0 | 0.10 |
T1BL·1RS | 2 | 2 | 2 | 1 | 1 | 1 | 0.24 | 0.18 | 0.27 | 0 | 0 | 0 |
平均Mean | 1.80 | 1.50 | 1.70 | 1.40 | 1.00 | 1.40 | 0.20 | 0.10 | 0.20 | 0.08 | 0 | 0.07 |
品质 Quality | ||||||||||||
Dx5+Dy10 | 2 | 2 | 2 | 2 | 2 | 1 | 0.35 | 0.30 | 0.37 | 0.05 | 0.28 | 0 |
TaLox-B1 | 2 | 2 | 2 | 2 | 2 | 2 | 0.28 | 0.35 | 0.15 | 0.07 | 0.24 | 0.04 |
Pinb-D1 | 2 | 2 | 2 | 2 | 2 | 2 | 0.32 | 0.37 | 0.23 | 0.15 | 0.38 | 0.07 |
Ppo-A1 | 2 | 2 | 2 | 2 | 2 | 2 | 0.29 | 0.36 | 0.21 | 0.37 | 0.38 | 0.37 |
Ppo-D1 | 2 | 1 | 2 | 1 | 1 | 1 | 0.07 | 0 | 0.12 | 0 | 0 | 0 |
Psy-A1 | 2 | 1 | 2 | 1 | 1 | 1 | 0.17 | 0 | 0.25 | 0 | 0 | 0 |
Psy-B1 | 2 | 1 | 2 | 2 | 1 | 2 | 0.37 | 0 | 0.33 | 0.37 | 0 | 0.37 |
Psy1-D1 | 2 | 2 | 2 | 2 | 1 | 2 | 0.23 | 0.27 | 0.19 | 0.37 | 0 | 0.37 |
平均Mean | 2.00 | 1.63 | 2.00 | 1.75 | 1.50 | 1.63 | 0.26 | 0.21 | 0.23 | 0.17 | 0.16 | 0.15 |
粒重 Kernel weight | ||||||||||||
TaCwi-A1 | 2 | 2 | 2 | 2 | 2 | 2 | 0.36 | 0.36 | 0.25 | 0.29 | 0.21 | 0.30 |
TaCWI-4A | 2 | 2 | 2 | 2 | 2 | 2 | 0.35 | 0.37 | 0.32 | 0.28 | 0.35 | 0.26 |
TaCWI-5D | 2 | 2 | 2 | 2 | 2 | 2 | 0.11 | 0.18 | 0.04 | 0.23 | 0.32 | 0.21 |
TaGS1a | 2 | 2 | 2 | 2 | 2 | 2 | 0.29 | 0.37 | 0.17 | 0.36 | 0.19 | 0.37 |
TaGS-D1 | 2 | 2 | 2 | 2 | 2 | 2 | 0.28 | 0.36 | 0.37 | 0.35 | 0.24 | 0.19 |
TaGS5-A1 | 2 | 2 | 2 | 2 | 2 | 2 | 0.38 | 0.27 | 0.29 | 0.20 | 0.37 | 0.35 |
TaSus2-2B | 2 | 2 | 1 | 1 | 1 | 1 | 0.21 | 0.35 | 0 | 0 | 0 | 0 |
TaTGW6-A1 | 2 | 1 | 2 | 2 | 2 | 2 | 0.14 | 0.19 | 0.10 | 0.37 | 0.27 | 0.37 |
平均Mean | 2.00 | 1.88 | 1.88 | 1.85 | 1.88 | 1.88 | 0.27 | 0.31 | 0.19 | 0.26 | 0.24 | 0.26 |
总平均 Total mean | 1.92 | 1.67 | 1.86 | 1.65 | 1.46 | 1.63 | 0.24 | 0.20 | 0.21 | 0.17 | 0.13 | 0.16 |
新窗口打开
2.4 群体结构分析
对供试材料52个等位基因遗传多样性的分子检测结果分析发现, 改良品种和地方品种两类群体间、群体内个体间以及个体间差异都极显著(P < 0.01), 这三者分别解释总变异的23.9%、71.5%和4.7% (表3)。Table 3
表3
表3遗传多样性分子方差分析
Table 3Analysis of molecular variance (AMOVA) based on genetic diversity
变异来源 Source of variance | 自由度 df | 平方和 Sum of square | 方差分量 Variance component | 变异百分比 Percentage of variation (%) |
---|---|---|---|---|
群体间Among populations | 1 | 96.7 | 0.70** | 23.9 |
群体内个体间Among individuals within populations | 134 | 578.4 | 2.09** | 71.5 |
个体间Within individuals | 136 | 18.5 | 0.14** | 4.7 |
总计Total | 271 | 693.6 | 2.92 |
新窗口打开
基于主成分的群体结构分析发现, 通过第1主成分(PC1)和第2主成分(PC2)聚类能够将136份资源划分为改良品种和地方品种两类, 其中冬性改良品种和春性改良品种也各自聚为一类(图4)。
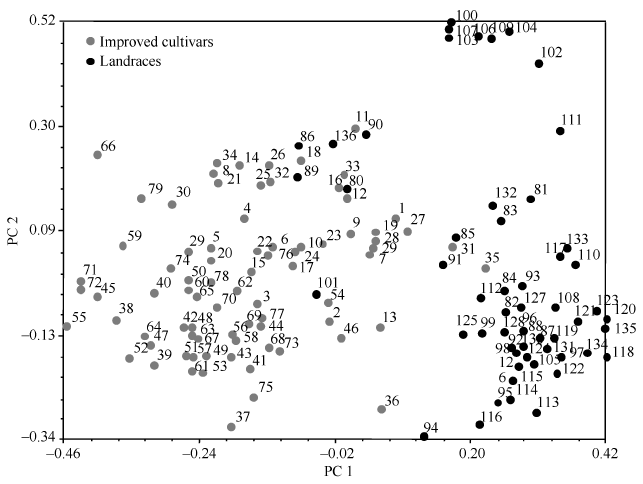
图4136份新疆小麦资源主成分分析
1~79: 改良品种, 其中1~35为冬性品种, 36~79为春性品种; 80~136: 地方品种, 其中80~86为冬性地方品种, 87~136为春性地方品种。图中数字代表的资源名称参考附
-->Fig. 4Principal component (PC) analysis of 136 Xinjiang wheat accessions
1-79: improved cultivars, including winter (1-35) and spring (36-79) accesions; 80-136: landraces, including winter (80-86) and spring (87-136) accesions. Numbers represent germplasm names given in Supplementary Tables 1 and S2.
-->
3 讨论
新疆改良品种比地方品种含有更高的优异等位变异频率, 充分反映了育种是将优异等位基因不断选择和聚合的过程。矮秆等位变异Rht-B1b和Rht-D1b在我国小麦生产上发挥了重要的作用。Rht-B1b和Rht-D1b在新疆改良品种的分布频率分别为79.7%和15.2% (表1), 至少含有其中一个矮秆等位变异的频率达到85%, 表明这2个等位变异已经广泛应用于新疆小麦育成品种。杨松杰等[33]发现, Rht-B1b等位变异在新疆冬春小麦(8份)中的分布频率为62.5%, Rht-D1b等位基因分布频率为12.5%。Meng等[4]证明, 80%以上建国以来中国大面积推广品种(80份)至少含有两个矮秆等位变异中的一个。光周期基因Ppd是影响抽穗期的一类重要基因[7]。在Ppd-A1和Ppd-B1基因座, 新疆改良品种和地方品种没有差异, 都只含有一种等位变异(表1)。究其原因, 一是这2个基因座的功能标记是基于日本小麦品种开发的, 对中国品种可能不适用[10]; 二是引起抽穗期变化的可能是拷贝数, 目前这种推测在Ppd-B1基因座已经被证实[34]。
20世纪80年代后我国育成的小麦品种中38%~43%含有T1RL·1BS [35,36]。新疆小麦品种也不例外, 特别是1990—2010年间, 有20%的新疆小麦育成品种中含有这个易位系。T1RL·1BS易位系在提高抗病性和丰产性的同时, 也可能降低小麦面包加工品质, 这可能是2010年以后新疆小麦改良品种中并没有检测到T1BL·1RS片段的原因之一。与品质相关的优质麦谷蛋白亚基Dx5+Dy10在新疆育种品种中达到33.6%, 相比之下, 在地方品种中仅为1.8% (表1)。与对360份新疆地方品种、育成品种和国内外引进小麦品种分析结果一致, 33.9%的改良品种含有Dx5+Dy10亚基[37], 但这一数值低于黄淮冬麦区的41.1%[38]。与面粉色泽相关的高脂肪氧化酶活性等位变异TaLox-B1a、低多酚氧化酶活性等位变异Ppo-D1a和低黄色素含量等位变异Psy-A1b在改良品种中分布频率均高于地方品种(表1)。尽管等位变异Ppo-D1a在改良品种的频率比地方品种高, 但明显低于王亮等[39]报道的86.9%。
现代育种实践使得重要农艺性状相关的优异等位变异逐渐累积。本研究中, 11个优异等位变异随着育种时期推进, 在新疆小麦改良品种中呈现不同程度的上升趋势(图3), 比如矮秆等位变异、优质相关的等位变异和高粒重等位变异, 这是不难理解的, 这些等位变异对新疆小麦生产是有利的, 因此被不断积累和保留下来。Meng等[4]通过对1949年以来80个大面积推广小麦品种研究同样发现, 与重要农艺性状相关的优异等位变异分布频率随育种时期推进呈现上升趋势。
新疆属于冬、春麦兼种区, 这一特殊生态区域要求北疆以春性品种为主, 而南疆以冬性或者抗寒性较好的弱冬性品种为主[40]。对春化基因的检测发现, 春性改良品种至少含有一个显性等位变异, 而冬性品种在所有春化基因座全部为隐性, 说明该地区小麦品种春化基因组成完全是为适应特定生态环境不断选择的结果[41]。光周期不敏感等位变异Ppd-D1a在新疆冬性地方品种和春性地方品种中分布频率很低, 而在春性和冬性改良品种中频率明显提高(表1), 表明Ppd-D1a可能经历了较强的选择压。值得注意的是, 该等位变异在春性改良品种中分布频率(95.1%)明显高于冬性改良品种(55.9%)。随着育种时期的推进Ppd-D1a分布频率在春性改良品种中呈现直线上升趋势, 而在冬性品种中2010年以前呈现直线上升, 2010年后呈现明显下降趋势, 进一步表明冬性和春性改良品种对该等位变异的选择是不一致的。其原因可能是春播春性品种要求生育期短, 光周期敏感品种会延长生育期, 不能满足生产需要, 这类品种在小麦生产实践过程中自然被淘汰; 而冬性品种在秋季播种需要经历漫长的冬季和春化过程, 这就需要冬性品种具有强的抗寒性, 光周期敏感品种能够延长营养生长过渡到生殖生长的时间, 在一定程度上避开冬季冻害, 增强抗寒性[42]。
本研究发现改良品种的平均等位基因数目、遗传多样性指数和PIC均高于地方品种(表2), 与Rasheed等[43]对巴基斯坦小麦改良品种和地方品种比较结果一致, 但与Hao等[44]对中国小麦的研究有所不同。本研究利用的所有功能标记都是基于改良品种开发的, 这样的标记对地方品种或者野生近缘种的检测效率可能会有一定的影响, 导致在地方品种中检测不到某些稀有或新的等位变异, 这是可能导致地方品种遗传多样性低的一个原因。另外, 与改良品种比较, 供试新疆地方品种没有发现矮秆等位变异Rht-B1b和Rht-D1b、半冬性等位变异Vrn-D1b以及T1BL·1RS易位染色体, 也没有检测到低黄色素含量等位变异Psy-A1b和高千粒重等位变异Hap-H (TaSus2-2B), 这与实际情况是一致的, 比如, 广泛应用的矮秆等位变异Rht-B1b和Rht-D1b主要来自于日本品种农林10号; T1BL·1RS易位系在20世纪70年代才引入我国; 含有Vrn-D1b等位变异的新冬20实际上是从河北引进的品种, 因此, 在新疆地方品种中不可能检测到这些等位变异。由于这些基因对于提高新疆小麦品种适应性、品质和产量具有重要作用, 因此在改良品种中被保留下来, 这可能是导致新疆改良品种比地方品种遗传多样性高的另一个原因。当然, 本研究仅是基于功能标记分析, 如果采用SNP等高通量分子标记, 相信地方品种在遗传多样性上会有变化。
Rasheed等[43]利用功能标记对巴基斯坦228份小麦资源进行聚类分析, 发现改良品种和地方品种被分别聚为一类。同样, 本研究利用52对功能标记就能很好地将136个新疆小麦资源聚为改良品种和地方品种两类(图4)。这充分说明地方品种和改良品种存在巨大的遗传差异。
4 结论
利用功能标记分析发现, 重要农艺性状相关的优异等位变异在新疆改良品种中频率明显高于地方品种, 而且随着育种时期的推进这些优异等位变异频率在改良品种中呈现上升趋势。在适应性和品质相关基因方面, 春性改良品种的优异等位变异频率比冬性改良品种更高。改良品种遗传多样性高于地方品种。136份新疆小麦资源被聚为改良品种和地方品种两类, 冬性和春性改良品种分别聚为一类。The authors have declared that no competing interests exist.
作者已声明无竞争性利益关系。
参考文献 原文顺序
文献年度倒序
文中引用次数倒序
被引期刊影响因子
[1] | . . |
[2] | . . |
[3] | . 新疆粮油质检站对全疆45个粮食主产县约40个小麦品种的品质指标进行检测分析,对其一致性和差异性进行研究.分析结论是:新疆产小麦均为中筋小麦和弱筋小麦,缺乏优质高筋小麦,品质指标不平衡,蛋白质含量偏低. . 新疆粮油质检站对全疆45个粮食主产县约40个小麦品种的品质指标进行检测分析,对其一致性和差异性进行研究.分析结论是:新疆产小麦均为中筋小麦和弱筋小麦,缺乏优质高筋小麦,品质指标不平衡,蛋白质含量偏低. |
[4] | . The modern plant breeding is generally considered to be a practice that leads to a narrowing in genetic diversity of crops. The objective of the present study was to assess whether this practice has l |
[5] | . |
[6] | . Abstract Functional markers (FM) are developed from sequence polymorphisms present in allelic variants of a functional gene at a locus. FMs accurately discriminate alleles of a targeted gene, and are ideal molecular markers for marker-assisted selection in wheat breeding. In this paper, we summarize FMs developed and used in common wheat. To date, more than 30 wheat loci associated with processing quality, agronomic traits, and disease resistance, have been cloned, and 97 FMs were developed to identify 93 alleles based on the sequences of those genes. A general approach is described for isolation of wheat genes and development of FMs based on in silico cloning and comparative genomics. The divergence of DNA sequences of different alleles that affect gene function is summarized. In addition, 14 molecular markers specific for alien genes introduced from common wheat relatives were also described. This paper provides updated information on all FMs and gene-specific STS markers developed so far in wheat and should facilitate their application in wheat breeding programs. |
[7] | . |
[8] | . The broad adaptability of wheat and barley is in part attributable to their flexible growth habit, in that spring forms have recurrently evolved from the ancestral winter growth habit. In diploid wheat and barley growth habit is determined by allelic variation at the VRN-1 and/or VRN-2 loci, whereas in the polyploid wheat species it is determined primarily by allelic variation at VRN-1 . Dominant Vrn-A1 alleles for spring growth habit are frequently associated with mutations in the promoter region in diploid wheat and in the A genome of common wheat. However, several dominant Vrn-A1 , Vrn-B1 , Vrn-D1 (common wheat) and Vrn-H1 (barley) alleles show no polymorphisms in the promoter region relative to their respective recessive alleles. In this study, we sequenced the complete VRN-1 gene from these accessions and found that all of them have large deletions within the first intron, which overlap in a 4-kb region. Furthermore, a 2.8-kb segment within the 4-kb region showed high sequence conservation among the different recessive alleles. PCR markers for these deletions showed that similar deletions were present in all the accessions with known Vrn-B1 and Vrn-D1 alleles, and in 51 hexaploid spring wheat accessions previously shown to have no polymorphisms in the VRN-A1 promoter region. Twenty-four tetraploid wheat accessions had a similar deletion in VRN-A1 intron 1. We hypothesize that the 2.8-kb conserved region includes regulatory elements important for the vernalization requirement. Epistatic interactions between VRN-H2 and the VRN-H1 allele with the intron 1 deletion suggest that the deleted region may include a recognition site for the flowering repression mediated by the product of the VRN-H2 gene of barley. |
[9] | . Winter wheat and barley varieties require an extended exposure to low temperatures to accelerate flowering (vernalization), whereas spring varieties do not have this requirement. In this study, we show that in these species, the vernalization gene VRN3 is linked completely to a gene similar to Arabidopsis FLOWERING LOCUS T (FT). FT induction in the leaves results in a transmissible signal that promotes flowering. Transcript levels of the barley and wheat orthologues, designated as HvFT and TaFT, respectively, are significantly higher in plants homozygous for the dominant Vrn3 alleles (early flowering) than in plants homozygous for the recessive vrn3 alleles (late flowering). In wheat, the dominant Vrn3 allele is associated with the insertion of a retroelement in the TaFT promoter, whereas in barley, mutations in the HvFT first intron differentiate plants with dominant and recessive VRN3 alleles. Winter wheat plants transformed with the TaFT allele carrying the promoter retroelement insertion flowered significantly earlier than nontransgenic plants, supporting the identity between TaFT and VRN-B3. Statistical analyses of flowering times confirmed the presence of significant interactions between vernalization and FT allelic classes in both wheat and barley (P < 0.0001). These interactions were supported further by the observed up-regulation of HvFT transcript levels by vernalization in barley winter plants (P = 0.002). These results confirmed that the wheat and barley FT genes are responsible for natural allelic variation in vernalization requirement, providing additional sources of adaptive diversity to these economically important crops. |
[10] | . |
[11] | . The Ppd-A1 genotype of 240 Japanese wheat cultivars and 40 foreign cultivars was determined using a PCR-based method. Among Japanese cultivars, only 12 cultivars, all of which were Hokkaido winter wheat, carried the Ppd-A1a allele, while this allele was not found in Hokkaido spring wheat cultivars or Tohoku-Kyushu cultivars. Cultivars with a photoperiod-insensitive allele headed 6.9-9.8 days earlier in Kanto and 2.5 days earlier in Hokkaido than photoperiod-sensitive cultivars. The lower effect of photoperiod-insensitive alleles observed in Hokkaido could be due to the longer day-length at the spike formation stage compared with that in Kanto. Pedigree analysis showed that 'Purple Straw' and 'Tohoku 118' were donors of Ppd-A1a and Ppd-D1a in Hokkaido wheat cultivars, respectively. Wheat cultivars recently developed in Hokkaido carry photoperiod-insensitive alleles at a high frequency. For efficient utilization of Ppd-1 alleles in the Hokkaido wheat-breeding program, the effect of Ppd-1 on growth pattern and grain yield should be investigated. Ppd-A1a may be useful as a unique gene source for fine tuning the heading time in the Tohoku-Kyushu region since the effect of Ppd-A1a on photoperiod insensitivity appears to differ from the effect of Ppd-B1a and Ppd-D1a. |
[12] | . |
[13] | . |
[14] | . |
[15] | . The quantitative level of friabilin 15-kDa protein present on the surface of water-washed starch is highly correlated with wheat grain softness. Friabilin is composed primarily, if not exclusively, of the proteins puroindoline a and b. The transcript levels of these two proteins are similar among hard and soft wheat varieties, and the expression of both is controlled by the short arm of chromosome 5D, also the chromosomal location of the Hardness gene. We report here a glycine to serine sequence change in puroindoline b associated with hard grain texture. This amino acid change results from a single nucleotide mutation and resides in a region thought to be important for the lipid-binding properties of puroindolines. No recombination was observed between the serine puroindoline-b mutation, hard grain texture and low levels of starch surface friabilin among a set of 83 homozygous 5D recombinant lines derived from the soft-textured variety ‘Chinese Spring’ and the substitution line ‘Chinese Spring’ containing the 5D chromosome of the hard-textured variety ‘Cheyenne’. The sequence change reported here may adversely affect the lipid-binding properties of puroindoline-b and so effect hard grain texture. The results suggest that grain hardness results from puroindoline-b functionality such that the Hardness gene is a direct manifestation of puroindoline structure. We are suggesting the tentative molecular marker loci designations of Pinb-D1a and Pinb-D1b for the glycine and serine puroindoline-b types, respectively. |
[16] | . The enzyme activity of polyphenol oxidase (PPO) in grain has been related to undersirable brown discoloration of bread wheat ( Triticum aestivum L.) based end-products, particularly for Asian noodles. Breeding wheat cultivars with low PPO activity is the best approach to reduce the undesirable darkening. Molecular markers could greatly improve selection efficiency in breeding programs. Based on the sequences of PPO genes (GenBank Accession Numbers AY596268, AY596269 and AY596270) conditioning PPO activity during kernel development, 28 pairs of primers were designed using the software ‘DNAMAN’. One of the markers from AY596268, designated as PPO18 , can amplify a 685-bp and an 876-bp fragment in the cultivars with high and low PPO activity, respectively. The difference of 191-bp size was detected in the intron region of the PPO gene. The STS marker PPO18 was mapped to chromosome 2AL using a DH population derived from a cross Zhongyou 9507×02CA9632, a set of nulli-tetrasomic lines and ditelosomic line 2AS of Chinese Spring. QTL analysis indicated that the PPO gene co-segregated with the STS marker PPO18 and is closely linked to Xgwm312 and Xgwm294 on chromosome 2AL, explaining 28–43% of phenotypic variance for PPO activity across three environments. A total of 233 Chinese wheat cultivars and advanced lines were used to validate the correlation between the polymorphic fragments of PPO18 and grain PPO activity. The results showed that PPO18 is a co-dominant, efficient and reliable molecular marker for PPO activity and can be used in wheat breeding programs targeted for noodle quality improvement. |
[17] | . Polyphenol oxidase (PPO) activity is highly related to the undesirable browning of wheat-based end products, especially Asian noodles. Characterization of PPO genes and the development of their functional markers are of great importance for marker-assisted selection in wheat breeding. In the present study, complete genomic DNA sequences of two PPO genes, one each located on chromosomes 2A and 2D and their allelic variants were characterized by means of in silico cloning and experimental validation. Sequences were aligned at both DNA and protein levels. Two haplotypes on chromosome 2D showed 95.2% sequence identity at the DNA level, indicating much more sequence diversity than those on chromosome 2A with 99.6% sequence identity. Both of the PPO genes on chromosomes 2A and 2D contain an open reading frame (ORF) of 1,731 bp, encoding a PPO precursor peptide of 577 amino acids with a predicted molecular mass of 64 kD. Two complementary dominant STS markers, PPO16 and PPO29 , were developed based on the PPO gene haplotypes located on chromosome 2D; they amplify a 713-bp fragment in cultivars with low PPO activity and a 490-bp fragment in those with high PPO activity, respectively. The two markers were mapped on chromosome 2DL using a doubled haploid population derived from the cross Zhongyou 9507/CA9632, and a set of nullisomic etrasomic lines and ditelosomic line 2DS of Chinese Spring. QTL analysis indicated that the PPO gene co-segregated with the two STS markers and was closely linked to SSR marker Xwmc41 on chromosome 2DL, explaining from 9.6 to 24.4% of the phenotypic variance for PPO activity across three environments. In order to simultaneously detect PPO loci on chromosomes 2A and 2D, a multiplexed marker combination PPO33/PPO16 was developed and yielded distinguishable DNA patterns in a number of cultivars . The STS marker PPO33 for the PPO gene on chromosome 2A was developed from the same gene sequences as PPO18 that we reported previously, and can amplify a 481-bp and a 290-bp fragment from cultivars with low and high PPO activity, respectively. A total of 217 Chinese wheat cultivars and advanced lines were used to validate the association between the polymorphic fragments and grain PPO activity. The results showed that the marker combination PPO33/PPO16 is efficient and reliable for evaluating PPO activity and can be used in wheat breeding programs aimed for noodle and other end product quality improvement. |
[18] | . |
[19] | . |
[20] | . Cell wall invertase (CWI) is a critical enzyme for sink tissue development and carbon partition, and has a high association with kernel weight. Characterization of Cwi genes and development of functional markers are of importance for marker-assisted selection in wheat breeding. In the present study, the full-length genomic DNA sequence of a Cwi gene located on wheat chromosome 2A, designated TaCwi - A1 , was characterized by in silico cloning and experimental validation. TaCwi - A1 comprises seven exons and six introns, with 3,67602bp in total, and an open reading frame (ORF) of 1,76702bp. A pair of complementary dominant markers, CWI21 and CWI22, was developed based on allelic variations at the TaCwi - A1 locus. A 404-bp PCR fragment was amplified by CWI21 in varieties with lower kernel weights, whereas a 402-bp fragment was generated by CWI22 in the varieties with higher kernel weights. The markers CWI21 and CWI22 were located on chromosome 2AL using a F 2:3 population from a cross Doumai/Shi 4185, and a set of Chinese Spring nullisomic–tetrasomic lines. They were linked to the SSR locus Xbarc15 - 2AL with a genetic distance of 10.902cM. QTL analysis indicated that TaCwi - A1 could explain 4.8% of phenotypic variance for kernel weight over 202years. Two sets of Chinese landraces and two sets of commercial wheat varieties were used to validate the association of CWI21 and CWI22 with kernel weight. The results indicated that the functional markers CWI21 and CWI22 were closely related to kernel weight and could be used in wheat breeding for improving grain yield. |
[21] | . Plant invertase hydrolyzes sucrose into glucose and fructose.Cell wall invertase(CWI),one of the three types of invertase,is essential for development of flowers,seeds,and fruits.Based on isolated TaCWIs on chromosomes 4A,5B and 5D,two SNPs were detected in the promoter region of TaCWI-4A,and four SNPs and two Indels were present in the TaCWISD gene.No polymorphism was detected in TaCWISB coding or promoter regions.CAPS markers caps4 A and caps5 D were developed to discriminate haplotypes of TaCWI-4A and TaCWI-SD,respectively.Marker/trait association analysis indicated that Hap-5D-C at TaCWISD was significantly associated with higher thousand kernel weight(TKW),lower plant height(PH),earlier heading date(HD) and earlier maturity date(MD) in 348 Chinese modern cultivars grown in multiple environments.Geographic distributions and changes over time of favored haplotypes showed that Hap-5D-C was the major haplotype in modern cultivars and was strongly positively selected in six major wheat production regions worldwide.However,selection for haplotypes at TaCWI-4A was not so evident,possibly due to balancing effects of the two haplotypes on TKW and grain number per spike(GN).In rainfed production regions,Hap-4A-C was favored because it brought more seeds,but in well irrigated conditions,Hap-4A-T was favored in modern breeding because of higher TKW.Evolutionary analysis among wheat and its relatives showed that genetic diversity of TaCWI genes on chromosomes 4A and 5D declined dramatically in progression from the diploid level to modern polyploid cultivars.There was strong allelic selection in polyplodization,domestication and breeding. |
[22] | . Glutamine synthetase (GS) is a key enzyme in the formation of the amino acid glutamine during N assimilation. The characterization of GS genes and the development of functional markers are important for marker-assisted selection (MAS) in wheat breeding programs. In the present study, the full-length genomic DNA (gDNA) sequence of TaGS1a was obtained from 60 wheat varieties. TaGS1a comprises 3415bp and has eleven exons and ten introns. Nine single nucleotide polymorphisms (SNPs) and two insertions and deletions of DNA segments (InDels) were detected in introns, resulting two haplotypes: Hap 1 and Hap 2. A cleaved amplified polymorphic sequence (CAPS) marker was developed to distinguish the two haplotypes. The TaGS1a-CAPS marker was located on chromosome 6D using Chinese Spring nullisomic鈥搕etrasomic lines, and mapped at 2.5 cM from the SSR marker barc1121b in a RIL population. The completely corresponding results between quantitative trait locus (QTL) analysis and association analysis suggested that the TaGS1a gene had functions for grain size traits, including thousand grain weight (TGW), grain width (GW), grain height (GH), GL/GW ratio (GLW), factor form density (FFD), grain area (GA) and grain volume (GV) during maturity stage, and for the ratio of root/shoot for dry weight and nitrogen, phosphorus, potassium contents (RSDW, RSNC, RSPC and RSKC) during seedling stage. |
[23] | . The OsGS3 gene plays a principal role in controlling grain weight and grain length in rice. However, the function of an orthologous gene TaGS in wheat has not been analyzed to date. In the present study, we cloned the gDNA of TaGS gene, designated TaGS - D1 , with four exons and three introns on chromosome 7DS by a comparative genomics approach. The cDNA of TaGS - D1 is 255 bp, and it encodes 85 amino acids. We also found a plant-specific organ size regulation domain in the deduced polypeptide, indicating that TaGS - D1 , like OsGS3 , does not belong to the PEBP family. DNA sequencing of the TaGS - D1 locus revealed no diversity in the coding sequence of exons, but there was a single nucleotide polymorphism (SNP) in the first intron, and 30 SNPs, a 40-bp InDel and a 3-bp InDel were found in the second intron between genotypes with higher and lower thousand grain weights (TGW). Based on the 40-bp InDel, a co-dominant STS marker, designated GS7D, was developed to discriminate the two alleles. GS7D was 8.0 cM from Xbarc184 located on chromosome 7DS by linkage mapping. A QTL for TGW and grain length at GS7D locus explained up to 16.3 and 7.7 %, respectively, of the phenotypic variances in a RIL population derived from Doumai/Shi 4185 grown in Shijiazhuang and Beijing. One hundred and seventy-five Chinese wheat cultivars were genotyped with GS7D, indicating that TaGS - D1 was significantly associated with grain weight. The allelic distribution at the TaGS - D1 locus showed that the frequencies of TaGS - D1a were high in cultivars from Serbia, Japan, Australia, Canada, and the Northeastern Spring Wheat and Northern Winter Wheat Regions of China. |
[24] | . TaGS5 genes were cloned from bread wheat and were physically mapped on 3AS and 3DS. Sequencing results revealed that a SNP was found in the sixth exon of TaGS5-A1 gene. The SNP resulted in amino acid change from alanine to serine at the 303 bp position of TaGS5-A1. These two alleles were designated as TaGS5-A1a (alanine at the 303 bp position) and TaGS5-A1b genes (serine at the 303-bp position). Analysis of association of TaGS5-A1 alleles with agronomic traits indicated that cultivars with TaGS5-A1b possessed wider kernel width and higher thousand-kernel weight, as well as significantly lower plant height, spike length, and internode length below spike than those of cultivars with TaGS5-A1a over three years. These trait differences between TaGS5-A1a and TaGS5-A1b genotypes were larger in landraces than in modern cultivars. This finding suggested that TaGS5 gene played an important role in modulating yield-related traits in the landraces, which possibly resulted from numerous superior genes gathering in modern cultivars after strong artificial selection. The preferred TaGS5-A1b haplotype underwent very strong positive selection in Chinese modern wheat breeding, but not in Chinese landraces. Expression analysis of the TaGS5-A1 gene indicated that TaGS5-A1b allele possessed significantly higher expression level than TaGS5-A1b allele in differently developmental seeds. This study could provide relatively superior genotype in view of agronomic traits in wheat breeding programs. Likewise, this study could offer important information for the dissection of molecular and genetic basis of yield-related traits. |
[25] | . |
[26] | . |
[27] | . Vernalization requirement in wheat is determined by allelic variation mainly at three loci, Vrn - A1 , Vrn - B1 and Vrn - D1 . Up to 2011 only two alleles at the Vrn - D1 locus were known. The dominant allele, now designated as Vrn - D1a , confers spring growth habit, and the other, recessive allele, vrn - D1 , is associated with the winter growth habit. Recently, we found an additional dominant allele, Vrn - D1b , associated with the facultative growth habit. As facultative wheat cultivars play an important role in wheat production we screened 689 accessions from all over China, with a specific aim of determining the frequency and distribution of Vrn - D1b . The results showed that Vrn - D1a , Vrn - D1b and vrn - D1 were present in 27.3, 20.6 and 52.102% of all accessions, respectively. Vrn - D1a was mostly distributed in the autumn sown spring wheat zone, whereas Vrn - D1b was common in the autumn sown facultative wheat zone. One cultivar (Shiluan 02-1) was heterogeneous at the Vrn - D1 locus. A comparison between the Vrn - D1b genotype and the vrn - D1 genotype from the same cultivar showed that without vernalization the Vrn - D1b genotype reached the double ridge (DR) stage 2702days earlier than the vrn - D1 genotype. Vernalization responses, expressed as a reduction in the number of days to reach the DR stage following cold treatment, at 402°C for 20, 30 and 4002days, were 62, 67 and 7502days, respectively, for the Vrn - D1b genotype, and 74, 87 and 9802days, respectively, for the vrn - D1 genotype, confirming the effect of Vrn - D1b on facultative growth habit. Pedigree analysis indicates that the Vrn - D1b allele originated from Chinese landraces. |
[28] | . |
[29] | . A genome specific DNA sequence that detects Secale africanum chromatin incorporated into wheat was developed in this study. Random amplified polymorphic DNA (RAPD) analysis was used to search for genome specific DNA sequences of S. africanum in lines, R111, “mianyang11” (MY11) and wheat-rye 1RS/1BL translocations R25 and R57. A high copy rye-specific DNA segment pSaD15 940 of the S. africanum genome was obtained. The sequence of pSaD15 did not show any significant homology to other reported sequences in databases and it is therefore a new repetitive sequence of Secale . PCR primers were designed for pSaD15 940 , which amplify a clear 88702bp fragment in S. africanum but not in any wheat. The primers also amplified an 88702bp fragment in other accessions of rye, Chinese Spring-Imperial rye chromosome additions and a diverse range of material carrying different rye chromosomes or chromosomal segments. In02situ hybridization showed that probe pSaD15 940 was specifically hybridized throughout all rye chromosomes arms except for the terminal regions. The advantage of the rye-specific probe developed herein compared to those of previous reports is that it has been shown to be widely applicable to other Secale species. The probe will be useful as a molecular marker for the introgression of S. africanum and other rye chromosome segments into the wheat genome. |
[30] | . PowerMarker delivers a data-driven, integrated analysis environment (IAE) for genetic data. The IAE integrates data management, analysis and visualization in a user-friendly graphical user interface. It accelerates the analysis lifecycle and enables users to maintain data integrity throughout the process. An ever-growing list of more than 50 different statistical analyses for genetic markers has been implemented in PowerMarker. |
[31] | . |
[32] | . |
[33] | . . |
[34] | . |
[35] | . . |
[36] | . . |
[37] | . 为全面了解新疆冬、春麦兼种区小麦品种高分子量麦谷蛋白亚基(HMW-GS)组成与分布情况,给优质小麦品种选育提供理论依据,采用十二烷基硫酸钠-聚丙烯酰胺凝胶电泳(SDS-PAGE)方法对360份新疆农家品种、育成品种(系)及国内外引进品种的HMW-Gs组成进行了分析。结果表明,新疆小麦品种HMW-GS组成存在广泛变异,亚基类型以2、Null、7+8和2+12为主,其频率分别为40.0%、35.3%、51.4%和61.9%。此外,还发现了单亚基2和稀有亚基7、21、7+8、6.1+22、2.2+12,其中2.2+12亚基主要分布在南疆麦区。优质亚基频率偏低是新疆小麦品质差的重要原因。新疆冬、春小麦品种的HMW-GS组成也存在差异,冬小麦品种有17种变异类型,以Null/7+8/2+12为主;春小麦品种有13种变异类型,以2/7+8/2+12为主。育成品种中1、7+9和5+10亚基(对)的频率较农家品种有很大的提高,其中优质亚基5+10的频率呈明显上升趋势,由农家品种的。增加至2000年以后育成品种的33.9%,说明引进并利用外来优异种质有利于提高新疆小麦品种的加工品质。 . 为全面了解新疆冬、春麦兼种区小麦品种高分子量麦谷蛋白亚基(HMW-GS)组成与分布情况,给优质小麦品种选育提供理论依据,采用十二烷基硫酸钠-聚丙烯酰胺凝胶电泳(SDS-PAGE)方法对360份新疆农家品种、育成品种(系)及国内外引进品种的HMW-Gs组成进行了分析。结果表明,新疆小麦品种HMW-GS组成存在广泛变异,亚基类型以2、Null、7+8和2+12为主,其频率分别为40.0%、35.3%、51.4%和61.9%。此外,还发现了单亚基2和稀有亚基7、21、7+8、6.1+22、2.2+12,其中2.2+12亚基主要分布在南疆麦区。优质亚基频率偏低是新疆小麦品质差的重要原因。新疆冬、春小麦品种的HMW-GS组成也存在差异,冬小麦品种有17种变异类型,以Null/7+8/2+12为主;春小麦品种有13种变异类型,以2/7+8/2+12为主。育成品种中1、7+9和5+10亚基(对)的频率较农家品种有很大的提高,其中优质亚基5+10的频率呈明显上升趋势,由农家品种的。增加至2000年以后育成品种的33.9%,说明引进并利用外来优异种质有利于提高新疆小麦品种的加工品质。 |
[38] | . Wheat end-use product quality is highly influenced by the composition and quantity of high- and low-molecular-weight glutenin subunits (HMW-GS and LMW-GS). In the present study, 224 Chinese wheat cultivars and advanced lines were characterised for the HMW-GS and LMW-GS with allele-specific PCR markers and sodium-dodecyl-sulfate polyacrylamide gel electrophoresis (SDS-PAGE). The results showed that 56 cultivars (25.0%) carried the allele Glu-D1-1d (Dx5), while 80 cultivars (35.7%) with the allele Glu-B1-2a (By8) produced a 527-bp specific band. Fourteen genotypes (6.3%) with the allele Glu-B1e (Bx20) yielded a 701-bp amplicon with the marker Mar and a 753-bp specific PCR fragment with the marker ZSBy9aF1/R3. Glu-B1h (Bx14+By15) was present in only 1 genotype, and 2 cultivars contained the allele Glu-B1f (Bx13+By16) identified with the marker ZSBy9F2/R2. Four genotypes (1.8%) with the allele Glu-B1-1d (Bx6) gave 695-bp and 830-bp bands, and 5 genotypes (2.2%) with the allele Glu-B1i (Bx17+By18) amplified a 659-bp fragment using the marker Bx. One hundred and six cultivars (47.3%) had the allele Glu-B1-2b (By9), amplifying a 663-bp fragment with the marker ZSBy9aF1/R3; 34 genotypes (15.8%) contained the allele Glu-B3d, generating a 662-bp PCR fragment with the marker gluB3d. Fifteen cultivars (7.0%) with the allele Glu-B3b yielded 1570-bp and 750-bp PCR amplicons with the markers gluB3b and gluB3bef, respectively. The allele Glu-B3h was found in 7 cultivars, generating a 1022-bp PCR fragment with the marker gluB3h. The genotypes detected by SDS-PAGE were mostly consistent with those identified by molecular markers, confirming the utility of the molecular markers. The information for the HMW-GS and LMW-GS in Chinese wheat cultivars will be useful in wheat breeding programs. |
[39] | . . |
[40] | . 对新疆小麦品质区划研究工作进行了总结.简述了新疆小麦品质生态区划的原则、方法、步骤以及命名方式.通过对比分析不同生态区小麦品质的表现,确定了小麦品质的区划方案.将新疆小麦品质生态区划分为3个主区和7个亚区,并对不同生态区的范围、生态环境状况、小麦品质的形成特点和适宜种植的品种类型进行了评述. . 对新疆小麦品质区划研究工作进行了总结.简述了新疆小麦品质生态区划的原则、方法、步骤以及命名方式.通过对比分析不同生态区小麦品质的表现,确定了小麦品质的区划方案.将新疆小麦品质生态区划分为3个主区和7个亚区,并对不同生态区的范围、生态环境状况、小麦品质的形成特点和适宜种植的品种类型进行了评述. |
[41] | . 为了明确新疆冬春麦区小麦春化和光周期基因的分布特点,利用 STS标记对185份品种(系)的重要春化基因Vrn-A1、Vrn-B1、Vrn-D1、Vrn-B3和光周期基因Ppd-D1住点的等位变异组成进行 了检测和分析.结果表明,在新疆小麦品种中,春化和光周期基因位点显性等位变异分布频率不同.含有春化显性等位变异Vrn-A1的品种47个,占供试品种 (系)的25.4%;Vrn-B1为43个,占23.3%;Vrn-D1为38个,占20.5%;Vrn-B3位点不存在显性等位变异.春化显性等位变异 Vrn-A1、Vrn-B1和Vrn-D1在冬、春性小麦内的分布比例也不同.在春性小麦品种(系)中,显性等位变异Vrn-A1出现的频率较高 (55.3%);其次为Vrn-B1,占50.6%;Vrn-D1占44.7%.在冬性小麦中,仅有显性等位变异Vrn-B1出现,占2.0%.在光周期 基因Ppd-D1位点,80.0%的品种(系)携带光不教感显性等位变异Ppd-D1a;其中在春性和冬性小麦品种(系)中,Ppd-D1a出现的频率分 别为83.5%和77.0%.新疆小麦品种(系)中,存在11种春化和光周期基因显性等位变异组合. . 为了明确新疆冬春麦区小麦春化和光周期基因的分布特点,利用 STS标记对185份品种(系)的重要春化基因Vrn-A1、Vrn-B1、Vrn-D1、Vrn-B3和光周期基因Ppd-D1住点的等位变异组成进行 了检测和分析.结果表明,在新疆小麦品种中,春化和光周期基因位点显性等位变异分布频率不同.含有春化显性等位变异Vrn-A1的品种47个,占供试品种 (系)的25.4%;Vrn-B1为43个,占23.3%;Vrn-D1为38个,占20.5%;Vrn-B3位点不存在显性等位变异.春化显性等位变异 Vrn-A1、Vrn-B1和Vrn-D1在冬、春性小麦内的分布比例也不同.在春性小麦品种(系)中,显性等位变异Vrn-A1出现的频率较高 (55.3%);其次为Vrn-B1,占50.6%;Vrn-D1占44.7%.在冬性小麦中,仅有显性等位变异Vrn-B1出现,占2.0%.在光周期 基因Ppd-D1位点,80.0%的品种(系)携带光不教感显性等位变异Ppd-D1a;其中在春性和冬性小麦品种(系)中,Ppd-D1a出现的频率分 别为83.5%和77.0%.新疆小麦品种(系)中,存在11种春化和光周期基因显性等位变异组合. |
[42] | . Vernalization and photoperiod requirements regulate the timing of the vegetative/reproductive transition in plants. Cereals adapted to cold winter climates regulate this developmental transition mainly through vernalization requirements, which delay transition from the vegetative to the reproductive growth stage. Recent research indicates that vernalization requirements also influence the expression of low-temperature (LT) tolerance genes in cereals exposed to acclimating temperatures. The objective of the present study was to determine if LT tolerance expression was also developmentally regulated by photoperiod response. The nonhardy, short day (SD) sensitive, wheat (Triticum aestivum L. em Thell) cultivar AC Minto, the LT tolerant, highly SD sensitive barley (Hordeum vulgare L.) cultivar Dicktoo, and a barley selection with very low sensitivity to SD were subjected to 8-h (SD) and 20-h (LD) days at cold acclimating temperatures over a period of 98 d. Final leaf number (FLN) was used to measure photoperiod sensitivity and determine the vegetative/reproductive transition point. The LT tolerance of the less SD sensitive barley genotype was similar for LD and SD treatments. In contrast, a delay in the transition from the vegetative to the reproductive stage in AC Minto and Dicktoo grown under SD resulted in an increased level and/or longer retention of LT tolerance. These results support the hypothesis that not only the level, but also the duration of gene expression determines the degree of LT tolerance in cereals. Consequently, any factor that lengthens the vegetative stage, such as vernalization or photoperiod sensitivity, also increases the duration of expression of LT tolerance genes. [References: 11] |
[43] | . We investigated alleles at 31 loci associated with adaptability, yield, and end-use quality in 107 wheat (Triticum aestivum L.) landraces (WLRs) and 121 improved historical wheat cultivars (HWCs) from Pakistan. The WLRs were categorized into two further subgroups: 36 pre-Green-Revolution landraces released as cultivars and 71 geographically spread landraces from all over Pakistan. Alleles Vrn-A1a, TaGW2-6A-A, TaCKX6-D1b, Pinb-D1b, Psy-A1b, and Wx-D1b were absent in WLRs, whereas ample diversity was observed at all other loci. In HWCs, only Wx-D1b and Glu-A3e were absent among the alleles tested, whereas the alleles Ppd-D1a (90%), Rht-B1b or Rht-D1b (83.4%), TaCwi-A1a (95%), TaGW2-6A-G (76%), TaCKX6-D1a (77.3%), Glu-A1b (66.1%), Glu-D1d (61.3%), Pina-D1b (88.2%), Pinb-D1a (90%), Psy-A1a (66.1%), Psy-B1b (81.8%), Psy-D1a (86.5%), Ppo-A1a (70%), TaZds-D1b (73.9%), TaLox-B1b (80.1%), and Wx-D1a (100%) predominated,ndicating significant improvement in adaptability, yield potential, and end-use quality and unconscious selection for favored alleles. Higher frequencies of favored alleles at the TaCwi-A1 and TaCKX6-D1 loci influencing 1000-kernel weight (TKW) in HWCs indicated that selection pressure on these alleles during breeding successfully contributed to cultivar improvement. Wright pairwise fixation index (Fst) statistics indicated greater genetic divergence between HWC and WLR collections (0.16)than HWC and WLR cultivars (0.14). Population structure based on functional markers (FMs) using principal component analysis partitioned the germplasm into two distinct groups. High genetic divergence and low admixture between HWCs and WLRs indicated limited use of landraces in wheat breeding in Pakistan. Our results suggested these collections as rich reservoirsof alleles and haplotype combinations that may be useful in future breeding programs. |
[44] | . |