

Physiological characters and gene mapping of a dwarf and wide-leaf mutant osdwl1 in rice (Oryza sativa L.)
HUANG Yan

通讯作者:
收稿日期:2019-12-22接受日期:2020-09-13网络出版日期:2021-01-12
基金资助: |
Received:2019-12-22Accepted:2020-09-13Online:2021-01-12
Fund supported: |
作者简介 About authors
黄妍, E-mail:

摘要
关键词:
Abstract
Keywords:
PDF (4518KB)元数据多维度评价相关文章导出EndNote|Ris|Bibtex收藏本文
本文引用格式
黄妍, 贺焕焕, 谢之耀, 李丹莹, 赵超越, 吴鑫, 黄福灯, 程方民, 潘刚. 水稻矮化宽叶突变体osdwl1的生理特性和基因定位[J]. 作物学报, 2021, 47(1): 50-60. doi:10.3724/SP.J.1006.2021.92069
HUANG Yan, HE Huan-Huan, XIE Zhi-Yao, LI Dan-Ying, ZHAO Chao-Yue, WU Xin, HUANG Fu-Deng, CHENG Fang-Min, PAN Gang.
水稻是我国最重要的粮食作物之一, 对保障我国粮食安全起重要作用。然而, 随着我国人口数量的持续增长、可耕种面积的逐年减少, 粮食安全形势日益严峻[1,2]。因此, 培育高产稳产水稻新品种依旧是水稻育种工作者的重要目标之一[3]。株高是水稻重要农艺性状之一, 直接影响水稻生物学产量, 与水稻的抗倒伏及抗病虫能力线性相关, 最终影响水稻的丰产性和稳产性[4,5]。传统水稻品种一般表现为高秆, 矮秆则是突变性状[4,5]。遗传学研究显示, 矮秆性状受主效基因或多个微效基因控制, 同时受内源激素及外源光温水气肥的影响[4,5,6]。利用正向或反向遗传学手段, 迄今已克隆调控水稻株高的基因超过70个, 这些基因多数与赤霉素、油菜素内酯、独脚金内酯、生长素等激素生物合成代谢或信号传导有关[4,5]。研究表明, 现已克隆的多数矮秆或半矮秆基因表现出“一因多效”的遗传效应, 即引起水稻植株矮化的同时其他农艺性状也发生改变, 如d3[7]、d10[8]、d14[9]、fc1[10]和htd1[11]具多分蘖特征; sgd2[12]和SRS5[13]与籽粒大小有关; 而DNL1[14]则表现为窄叶; dlt[15]则引起少蘖宽叶。
当然, 叶片是水稻进行光合作用的主要场所, 是最重要的源器官, 其形态直接影响植株形态并最终关系到稻谷产量与品质。而叶片的长、宽、面积、厚、倾角、披垂度、卷曲度等是界定叶形态及空间姿态的重要因素[16], 因此增加叶宽将影响有效光合叶面积, 从而影响单个叶片的光合产物。研究认为, 叶片是沿基-顶轴、近-远轴以及中-边轴3个轴向生长发育, 其中中-边轴调控叶宽[17]。因此, 可以利用窄叶或宽叶突变体研究水稻叶宽调控分子机理, 迄今已克隆诸如NAL1[18]和DNL3[19]等窄叶基因, 但有关宽叶突变体却仅有Wl[20]和dlt[15]等少量报道。
本课题组前期利用60Co辐射诱变中籼恢复系自选1号, 获得一个矮化宽叶突变体, 暂名为osdwl1 (Oryza sativa dwarf and wider-leaf 1, osdwl1)。该突变体在苗期(约3~4片期)就表现为宽叶, 且除上部一片完整展开叶及心叶外, 其他叶片的中上部均表现为黄叶。之后随着叶龄的增加, 除叶片形态发生变化外, 植株还明显矮化。本文对突变体osdwl1的基本表型、生理细胞变化及基因定位等方面进行了研究, 为进一步克隆该基因并揭示其矮化宽叶的分子细胞生理机理奠定基础。
1 材料与方法
1.1 试验材料
矮化宽叶突变体osdwl1来源于籼稻恢复系自选1号(来源于湖北省恩施市农业科学院水稻研究所)的60Co辐射诱变后代, 经杭州和海南连续多代回交和自交, 突变性状已稳定遗传。之后利用突变体osdwl1分别与原始对照自选1号、籼稻恢复系93-11以及常规粳稻02428杂交, 获得osdwl1/自选1号、osdwl1/93-11和osdwl1/02428三个F1并自交获得相应F2群体, 用于突变性状的遗传分析和基因定位。所有材料均种植于浙江大学紫金港农业试验站, 常规肥水管理。水稻幼苗期和抽穗开花期, 分别调查20个单株的幼叶和剑叶宽度。水稻成熟后, 随机选取osdwl1及自选1号各20株, 考查其株高、茎节长、穗长、有效穗数、每穗粒数、结实率和千粒重等主要农艺性状。1.2 生理指标测定
随机选取osdwl1及自选1号处于孕穗期(剑叶叶枕与倒二叶叶枕重合的分蘖)的剑叶、倒二叶和倒三叶的上部叶片, 根据Gong等[21]的方法分别测定叶绿素、可溶性蛋白、H2O2、O2?和MDA含量、以及CAT和SOD酶活。同时, 利用Li-6400XT光合仪以及PAM-2000叶绿素荧光仪分别测定剑叶、倒二叶和倒三叶相应部位的净光合速率及Fv/Fm[22,23]。每个生理指标测定5个生物学重复。1.3 叶片及茎节细胞学观察
取抽穗当天osdwl1及自选1号剑叶的上部边缘叶片, 用刀片切成1 mm × 2 mm的样品, 立即放入预冷的2.5%戊二醛固定液中并抽真空至样品完全浸没, 4℃固定过夜, 根据Yang等[24]的方法制片, 于扫描电镜(Hitachi TM-1000)和透射电镜(JEM-1230)下分别观察叶片表皮细胞和叶肉细胞显微结构并拍照。同时, 选取抽穗当天osdwl1及自选1号相同部位剑叶, 将叶片横剪成2 mm宽的小片段, 立即放入70% FAA固定液并抽真空至样品完全浸没, 4℃固定3 d, 而后依次进行乙醇脱水、二甲苯透明、石蜡包埋、切片以及番红固绿染色, 最后用1︰1的二甲苯和中性树胶封片, 置于42℃恒温箱处理48 h, 后于NIKON E6000显微镜下观察并拍照。此外, 根据曹剑波等[25]的方法, 选取抽穗当天osdwl1及自选1号相同部位倒二节茎段进行树脂半薄切片, 于倒置显微镜(Nikon TE2000-U)下观察细胞形态并照相。1.4 基因遗传分析与定位
大田栽培条件下, 首先观察osdwl1/自选1号及osdwl1/93-11的杂种F1的株高及叶片表型, 确定控制osdwl1矮化宽叶的显隐性; 其次, 根据osdwl1/自选1号及osdwl1/93-11的F2群体中具有野生型表型的单株数与具有突变体表型的单株数比例, 确定控制osdwl1矮化宽叶的基因数。同时, 剪取osdwl1/02428的F2定位群体中具有突变体性状和野生型性状的各10个单株、以及其亲本osdwl1和02428的叶片, 采用CTAB法提取基因组总DNA。选取SSR (2 结果与分析
2.1 突变体osdwl1的表型及主要农艺性状
osdwl1的叶片从苗期(三至四叶期)开始, 除上部1片完整展开叶及心叶外, 其他叶片在完整展开3~4 d后, 叶尖开始变黄并逐步扩展至叶片中上部(图1-A~C)。之后随着叶龄增长, osdwl1植株高度也逐渐矮于野生型对照, 到水稻成熟期(图1-D, E), osdwl1株高仅有野生型对照的64.18% (表1)。进一步分析植株每一个茎节长度, 结果显示osdwl1倒一节、倒二节、倒三节、倒四节和倒五节分别仅有野生型对照的62.86%、75.42%、79.24%、79.93%和77.03% (图1-E, F)。倒二茎节纵切面的树脂半薄切片结果显示, 突变体的细胞长度明显变短, 但细胞数目显著增加(图1-G, H)。由于叶片出现黄化症状, 导致突变体osdwl1的主要农艺性状, 如穗长、每穗粒数和结实率分别比野生型对照下降35.60%、37.35%和42.15%, 而突变体千粒重则极显著高于对照(表1)。图1

图1突变体及其野生型的表型
A: 幼苗期; B: 开花初期; C: 开花初期叶片, 其中F代表剑叶, 2~4分别为倒二叶至倒四叶; D: 成熟期; E: 成熟期穗及茎节, P代表小穗, 1~5分别为倒一节至倒五节; F: 2019年成熟期各茎节长度; G~H: 野生型对照(G)和突变体(H)倒二茎节的纵切面。A~F的标尺为20 cm, G~H的标尺为20 μm。* 表示在0.05水平上差异显著; ** 表示在0.01水平上差异显著(t-test)。
Fig. 1Phenotypes of osdwl1 and its wild-type (WT) plants at the different growth stages
A: seedling stage; B: early-flowering stage; C: leaves at the early-flowering stage, and F means flag leaf and 2-4 means 2nd to 4th leaf from top in order, respectively; D: mature stage; E: panicle and the internodes at the mature stage, P means panicle, 1-4 means the 1st to 5th internode from top, respectively; F: the length of different internodes at the mature stage in 2019; G-H: longitudinal sections of the 2nd internode from top in wild-type plants (G) and osdwl1 (H); Bar = 20 cm in A-F; Bar = 20 μm in G-H. Values marked with * and ** indicate significant differences at P < 0.05 and P < 0.01 by Student’s t-test, respectively.
Table 1
表1
表1突变体及其野生型的主要农艺性状
Table 1
性状 Trait | 2018 | 2019 | ||
---|---|---|---|---|
野生型 WT | 突变体 osdwl1 | 野生型 WT | 突变体 osdwl1 | |
株高 Plant height (cm) | 110.74±2.16 | 65.90±3.62** | 82.91±4.96 | 58.40±2.38** |
穗长 Panicle length (cm) | 24.35±0.31 | 14.87±1.26** | 23.07±0.82 | 15.67±1.25** |
有效穗数 Effective panicle number | 6.20±1.64 | 3.60±1.55** | 11.80±3.56 | 5.20±2.49** |
每穗粒数 Grain number per panicle | 165.96±7.64 | 97.56±12.67** | 177.72±10.51 | 117.74±21.21** |
结实率 Seed-setting rate (%) | 90.92±3.19 | 55.07±1.97** | 79.57±3.01 | 43.57±8.01** |
千粒重 1000-grain weight (g) | 23.17±1.04 | 26.91±1.31** | 22.42±0.67 | 26.06±1.06** |
单株产量 Yield per plant (g) | 19.44±1.34 | 3.27±0.91** | 20.89±2.13 | 4.76±1.00** |
新窗口打开|下载CSV
2.2 突变体osdwl1叶片表型特征
图1-C及图2-A显示突变体osdwl1幼苗期叶片及抽穗开花早期剑叶极显著宽于对照, 分别增宽16.17%和12.88%。剑叶石蜡切片(图2-B)结果显示, osdwl1与野生型对照之间的大维管束数无显著性差异, 但突变体的小维管束数及小维管束之间距均极显著增加, 分别增加10.98%和27.95% (图2-C, D); 而且, 与对照相比, 突变体小维管束间的表皮细胞数显著增加(图2-E), 而其宽度无明显变化(图2-F)。同时, 利用SEM分析突变体剑叶表皮细胞结构特征, 进一步证实突变体小维管束之间的间距极显著增加(图2-G), 此外, 与对照相比, osdwl1剑叶下表皮的小刺毛数也极显著增加, 达3384.85% (图2-G, H); 而其上表皮的大刺毛和小刺毛数则分别增加36.70%和211.08% (图2-G, I)。图2
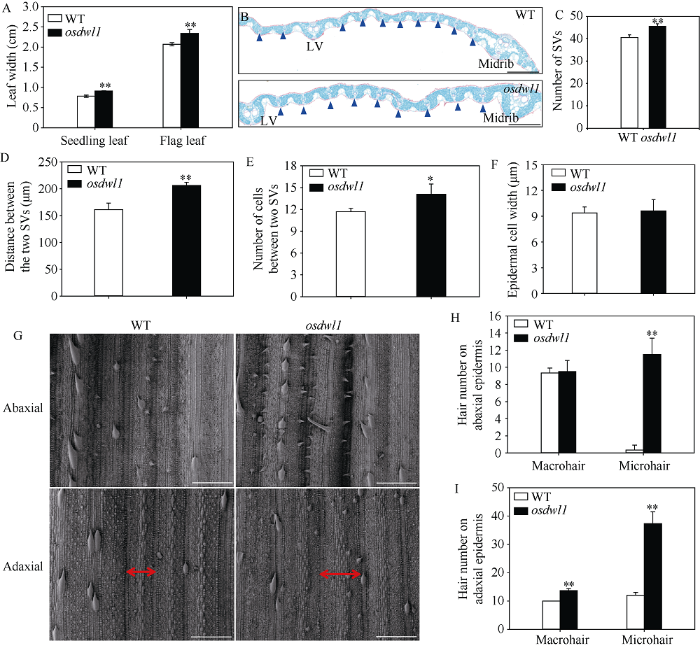
图2突变体osdwl1及其对照叶片的表型特征
A: 突变体及其对照幼叶及剑叶叶宽; B: 突变体及其对照剑叶石蜡切片, 蓝色三角形指示的是小维管束, LV代表大维管束; C: 突变体及其对照整个剑叶的小维管束数, SV代表小维管束; D: 突变体及其对照剑叶的小维管束之间的间距; E, F: 维管束之间的上表皮细胞数(E)及其宽度(F); G: 突变体及其对照剑叶上下表皮的扫描电镜观察, 双向箭头显示两个SV之间距; H, I: 突变体及其对照剑叶下表皮(H)和上表皮(I)的刺毛数。标尺为200 μm。* 表示在0.05水平上差异显著; ** 表示在0.01水平上差异显著(t-test)。
Fig. 2Phenotypic characteristics of leaf blade in osdwl1 and its wild-type plants
A: leaf blade width of the osdwl1 and its WT plants; B: transverse cross-sections of the flag leaf blade of the osdwl1 and its WT plants, blue triangles indicate small vascular bundles (SV), and LV means large vascular bundle; C: number of SVs in the whole flag leaves of osdwl1 and its WT plants; D: the distance between the two SVs in the osdwl1 and its WT plants; E: adaxial epidermal cell numbers between two SVs; F: adaxial epidermal cell width; G: SEM analysis of the abaxial and adaxial epidermis of the flag leaf blade in the osdwl1 and its WT plants, double arrow means the distance between two SVs; H, I: number of macrohairs and microhairs on the abaxial (H) and adaxial epidermis (I) of the flag leaf in the osdwl1 and its wild-type plants. Bar = 200 μm. Values marked with * and ** indicate significant differences by Student’s t-test at P < 0.05 and P < 0.01, respectively.
2.3 突变体osdwl1的生理分析
2.3.1 叶绿素含量及其光合作用效率 图3-A~D结果显示, 孕穗期osdwl1除叶绿素a/b比值(图3-D)及剑叶叶绿素含量(图3-A~C)外, 其倒二叶、倒三叶的叶绿素a (图3-A)、叶绿素b (图3-B)及叶绿素总含量(图3-C)依次极显著下降且极显著低于野生型对照。与野生型对照相比, osdwl1倒二叶和倒三叶的总叶绿素含量分别下降13.48%和26.59%。尽管osdwl1剑叶叶绿素含量无显著性变化, 但TEM结果(图3-E~H)显示突变体剑叶叶肉细胞的部分叶绿体类囊体结构松散(图3-H), 部分已开始降解(图3-G), 这势必影响突变体叶片的净光合速率(图3-I)及PSII光化学效率(Fv/Fm) (图3-J)。结果显示, 突变体剑叶、倒二叶和倒三叶的净光合速率和Fv/Fm明显低于野生型对照, 净光合速率分别下降27.22%、28.31%和22.25% (图3-I), 而其Fv/Fm的变化趋势与净光合速率基本一致, 分别下降13.34%、10.78%和20.40% (图3-J)。图3
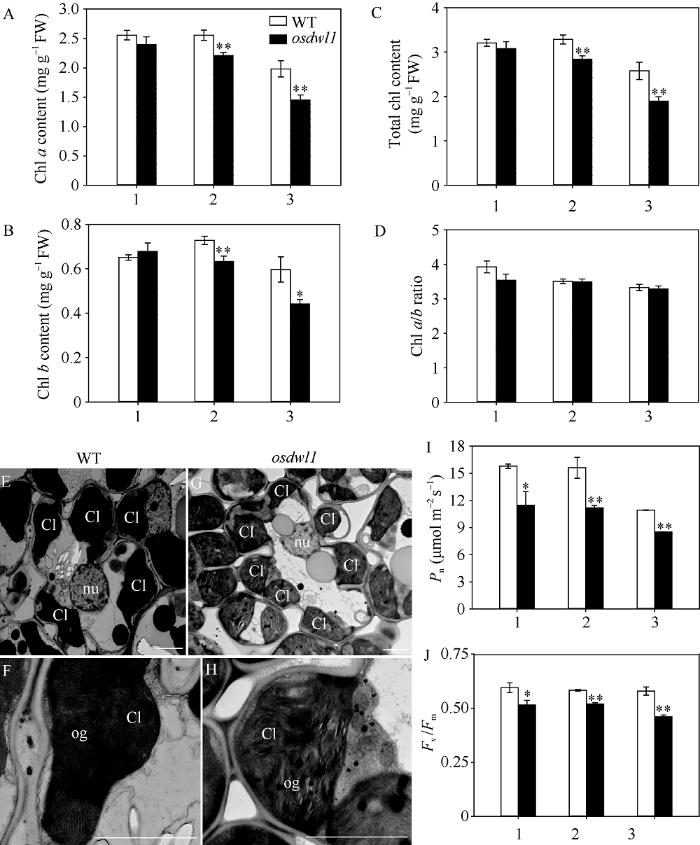
图3孕穗期突变体及其野生型叶片的光合特性及叶绿体超微结构
A~D: 突变体及其野生型叶片的叶绿素a (A)、叶绿素b (B)、总叶绿素含量(C)及叶绿素a/b比值(D); E~H: 突变体(G, H)及其野生型(E, F)剑叶叶肉细胞超微结构, nu代表细胞核; Cl代表叶绿体; og代表嗜锇颗粒; I~J: 突变体及其野生型叶片的净光合速率(I)及Fv/Fm值(J)。1: 剑叶; 2: 倒二叶; 3: 倒三叶。* 表示在0.05水平上差异显著; ** 表示在0.01水平上差异显著(t-test)。
Fig. 3Photosynthetic characteristics and chloroplast ultrastructure of leaves in the osdwl1 and wild-type plants at the booting stage
A-D: Chl a (A), Chl b (B) and total Chl contents (C), and Chl a/b ratio (D) of leaves in osdwl1 and wild-type plants; E-H: ultrastructure of chloroplast in osdwl1 (G, H) and wild-type plants (E, F), nu means nucleus, Cl means chloroplast, og means osmiophilic granule; I, J: Net photosynthesis rate (I) and Fv/Fm ratio (J) of leaves in osdwl1 and wild-type plants. 1: flag leaves; 2: 2nd leaves from top; 3: 3rd leaves from top. Values marked with * and ** indicate significant differences by Student’s t-test at P < 0.05 and P < 0.01, respectively.
2.3.2 ROS含量及其抗氧化酶活性分析 图4-A显示, 野生型对照和osdwl1的剑叶、倒二叶和倒三叶间的O2?含量均极显著增加, 但后者显著高于前者, 分别增加11.77%、21.16%和25.88%。图4-B显示, 野生型对照的剑叶、倒二叶和倒三叶间的H2O2含量无显著性差异, 而osdwl1则依次升高且倒二叶和倒三叶显著高于剑叶, 分别增加11.44%和24.72%。为了平衡植物细胞内的活性氧含量, 如H2O2和O2?, 防止其对细胞造成损伤, 植物细胞会表达多种保护酶, 如SOD和CAT等抗氧化酶。因此, 进一步测定了叶片的CAT (图4-C)和SOD (图4-D)酶活。结果显示, 野生型剑叶、倒二叶和倒三叶间的SOD和CAT酶活无显著性差异, 而突变体osdwl1则依次极显著下降。与osdwl1剑叶相比, 其倒二叶和倒三叶的SOD酶活分别下降8.00%和20.08%, CAT酶活则分别减少39.84%和51.32%。
图4
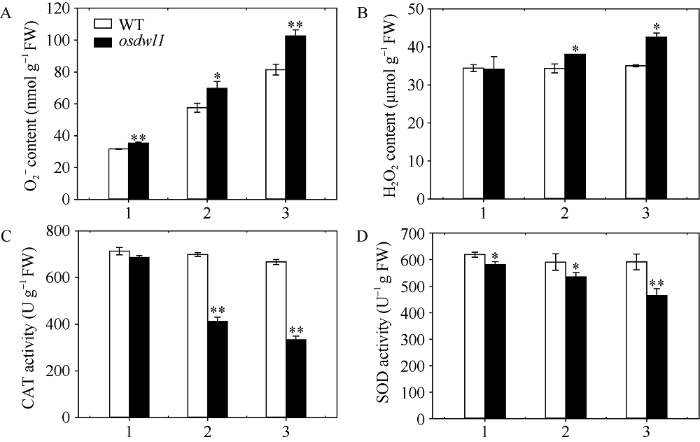
图4孕穗期突变体osdwl1及其野生型叶片的O2-和H2O2累积以及CAT和SOD的酶活
1: 剑叶; 2: 倒二叶; 3: 倒三叶。* 表示在0.05水平上差异显著; ** 表示在0.01水平上差异显著(t-test)。
Fig. 4Accumulation analysis of O2?and H2O2 contents, and CAT and SOD activities in the osdwl1 and its wild-type plants at booting stage
1: flag leaves; 2: 2nd leaves from top; 3: 3rd leaves from top. Values marked with * and ** indicate significant differences by Student’s t-test at P < 0.05 and P < 0.01, respectively.
2.3.3 MDA和可溶性蛋白含量 图5显示, 野生型对照的剑叶、倒二叶和倒三叶间的MDA含量(图5-A)和可溶性蛋白含量(图5-B)均无显著性差异。而osdwl1的剑叶、倒二叶和倒三叶间的MDA含量则依次显著升高, 除剑叶外, 倒二叶和倒三叶含量显著高于野生型对照(图5-A); 与突变体osdwl1剑叶相比,其倒二叶和倒三叶的MDA含量分别增加50.29%和79.87% (图5-A)。osdwl1的剑叶、倒二叶和倒三叶间的可溶性蛋白含量依次降低且均极显著低于野生型对照, 尤其是其倒三叶的含量极显著低于剑叶和倒二叶, 分别下降31.93%和28.53% (图5-B)。
图5
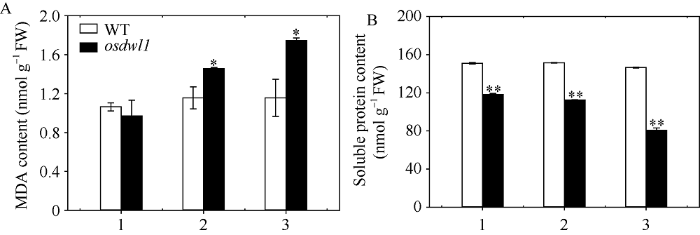
图5孕穗期突变体osdwl1及其野生型叶片的MDA和可溶性蛋白含量
1: 剑叶; 2: 倒二叶; 3: 倒三叶。* 表示在0.05水平上差异显著; ** 表示在0.01水平上差异显著(t-test)。
Fig. 5MDA and soluble protein contents of osdwl1 and the wild-type plants at booting stage
1: flag leaves; 2: 2nd leaves from top; 3: 3rd leaves from top. Values marked with * and ** indicate significant differences by Student’s t-test at P < 0.05 and P < 0.01, respectively.
2.4 遗传分析与基因定位
osdwl1/自选1号和osdwl1/93-11杂交F1植株形态均正常, 说明突变性状是由隐性位点控制。而osdwl1/自选1号的1105个F2群体中, χ2检验正常植株数(836)与具矮化及宽黄叶的植株数(269)的分离比符合3∶1 [χ2 = 0.25 < 3.84 (χ2(0.05,1))]; 而osdwl1/93-11的612个F2定位群体中, 正常单株数(455)与矮化及宽黄叶单株数(157)的分离比亦符合3∶1 [χ2 =0.46 < 3.84 (χ2(0.05,1))]的孟德尔遗传定律。这些结果表明osdwl1的矮化及宽黄叶性状由单隐性核基因控制。利用osdwl1/02428的F2群体中具有矮化及宽黄叶性状的673个单株作为基因定位群体。为了定位OsDWL1基因, 首先分别提取osdwl1/02428 F2群体中的10株野生型表型和10株具有矮化宽叶性状的单株基因组DNA, 分别等量混合成正常基因池和突变基因池。合成水稻12条染色体上的500对SSR标记及50对InDel标记, 逐条对02428和突变体osdwl1进行多态性分析, 而后选用均匀分布于12条染色体上的多态性分子标记, 利用BSA法分析其与OsDWL1基因间的连锁性关系。结果发现水稻6号染色体短臂上的SSR标记RM7399、RM19288、RM3805和RM19549与OsDWL1紧密连锁。利用这4个标记对osdwl1/02428的F2群体中的673个矮化宽叶单株进行基因型分析, 发现RM7399、RM19288、RM3805和RM19549的交换单株分别是80、23、19和121株, 初步将OsDWL1基因定位在RM19288和RM3805之间。为进一步精细定位OsDWL1基因, 继续合成位于RM19288和RM3805之间的10对SSR和InDel标记(附表1), 其中2对标记在02428和突变体osdwl1间存在多态性, 利用这2对标记将OsDWL1基因限定在RM19297与ID269-2之间, 物理距离约333 kb, 横跨AP001168、AP002838、AP000391和AP000559四个BAC (图6), 其间有EST支持的ORF为37个(
Table S1
附表1
附表1用于OsDWL1基因定位的分子标记
Table S1
标记 Marker | 正向引物 Forward primer (5'-3') | 反向引物 Reverse primer (5'-3') |
---|---|---|
RM7399 | CAGATATGATGTTCTTGCCCTTGC | GCTTGCCAGATCACCTACCTACC |
RM19288 | CGGAGCTGTTGCCGTTCTGC | CGATGTGCCATGTCAGGATGACC |
RM19297 | ATTTGCTCCGCTTCCAAATCACC | AGCGGCCAACAGAGACAACTGG |
ID269-2 | AGGGTGTGTTTAGTTCACGA | AAAATTTGTCATGGCTGTTG |
RM3805 | ACACCACCATCAACGTACCAACC | AAGTCGAGAGGAAGAAGCCAAGG |
RM19549 | CCTGGTACTAACCATGTGATTGAGC | AACGTCAGAGTCTCACCACAAGC |
新窗口打开|下载CSV
图6
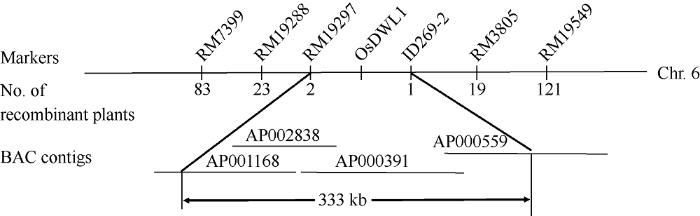
图6水稻矮化宽叶基因OsDWL1在6号染色体上的分子定位
Fig. 6Molecular mapping of OsDWL1 gene on the short arm of chromosome 6
Table 2
表2
表2定位区间内的基因及功能注释
Table 2
基因号 Locus identifier | 功能注释 Functional annotation |
---|---|
LOC_Os06g03390 | Expressed protein |
LOC_Os06g03486.1 | Expressed protein |
LOC_Os06g03514.1 | Expressed protein |
LOC_Os06g03520.1 | DUF581 domain containing protein, expressed |
LOC_Os06g03530.1 | Pentatricopeptide, putative, expressed |
LOC_Os06g03540.1 | Oligopeptide transporter, putative, expressed |
LOC_Os06g03560.1 | Oligopeptide transporter, putative, expressed |
LOC_Os06g03570.1 | Pentatricopeptide, putative, expressed |
LOC_Os06g03580.1 | Zinc RING finger protein, putative, expressed |
LOC_Os06g03600.1 | Transcriptional corepressor SEUSS, putative, expressed |
LOC_Os06g03610.1 | The CrRLK1L-1 subfamily has homology to the CrRLK1L homolog, expressed |
LOC_Os06g03640.1 | BAG domain containing protein, expressed |
LOC_Os06g03660.1 | Peroxisomal biogenesis factor 11, putative, expressed |
LOC_Os06g03670.1 | Dehydration-responsive element-binding protein, putative, expressed |
LOC_Os06g03676.1 | CAMK includes calcium/calmodulin dependent protein kinases, expressed |
LOC_Os06g03682.1 | Calcium-dependent protein kinase isoform AK1, putative, expressed |
LOC_Os06g03690.1 | RNA recognition motif containing protein, putative, expressed |
LOC_Os06g03700.1 | Oligopeptide transporter, putative, expressed |
LOC_Os06g03710.1 | DELLA protein SLR1, putative, expressed |
LOC_Os06g03720.1 | Ribonucleoside-diphosphate reductase small chain, putative, expressed |
LOC_Os06g03750.1 | Dehydration response related protein, putative, expressed |
LOC_Os06g03760.1 | LMBR1 integral membrane protein, putative, expressed |
LOC_Os06g03770.1 | ABC transporter, putative, expressed |
LOC_Os06g03780.1 | NUC153 domain containing protein, expressed |
LOC_Os06g03790.1 | 39S ribosomal protein L47, mitochondrial precursor, putative, expressed |
LOC_Os06g03800.1 | Pollen ankyrin, putative, expressed |
LOC_Os06g03810.1 | Expressed protein |
LOC_Os06g03820.1 | Expressed protein |
LOC_Os06g03830.1 | Retinol dehydrogenase, putative, expressed |
LOC_Os06g03840.1 | Bric-a-Brac, Tramtrack, Broad Complex BTB domain with H family conserved sequence, expressed |
LOC_Os06g03850.1 | Impaired sucrose induction 1, putative, expressed |
LOC_Os06g03860.4 | Uncharacterized membrane protein, putative, expressed |
LOC_Os06g03890.1 | Alpha-L-fucosidase 3 precursor, putative, expressed |
LOC_Os06g03910.1 | Hydrolase, NUDIX family, domain containing protein, expressed |
LOC_Os06g03920.1 | Expressed protein |
LOC_Os06g03930.1 | Cytochrome P450 86A1, putative, expressed |
LOC_Os06g03940.1 | Spastin, putative, expressed |
新窗口打开|下载CSV
3 讨论
株高是影响农作物株型、生物量、抗倒性及机械收割的重要因素[4,5]。研究表明, 植株过高是引起水稻倒伏的最主要原因[4,5]; 而植株过矮, 会造成水稻生长量不足, 故适当增加株高, 不仅可以降低叶面积密度(叶面积指数与株高的比值), 还有利于CO2扩散及中下部叶片吸收利用太阳光能, 增加光合速率, 进而提高植株生物量[26]。影响水稻株高的因素包括穗长、节间数和节间长度, 除少部分因穗长和节间数量发育缺陷的突变体外, 大多数矮秆水稻是由于节间长度变短造成的[4,5]。本研究中osdwl1的节间长度均极显著比野生型对照缩短, 导致osdwl1株高显著低于野生型对照(图1-E, F), 茎节的树脂半薄切片证实节间缩短的根本原因在于突变体细胞长度更小(图1-H)。植物株高既受诸如光温水气肥等外界环境的影响, 也受植物内源激素及基因的调控, 但归根结底受内源基因网络的精细调控[4,5,6]。大量研究结果证实, 水稻株高一般受1~3对主效基因控制, 但也受微效基因调控[4,5]。迄今已证实, 调控株高的基因包括GA代谢途径的DELLA蛋白基因、参与油菜素内酯和独脚金内酯合成代谢的细胞色素P450基因与钙调蛋白、RNA编辑相关的PPR (pentatricopeptide repeat)蛋白基因以及核糖体蛋白基因等[4,5]。如水稻编码PPR蛋白的OGR1基因的功能缺失突变体ogr1表现为矮化及不育等特性[27]; 与油菜素内酯合成代谢相关的水稻P450基因CYP90D2/D2的缺失突变[28]、拟南芥钙调蛋白基因DWL1的部分缺失过表达转基因后代均表现矮化症状[29]; 参与GA信号通路的水稻DELLA蛋白基因SLR1的功能缺失也导致水稻矮化[30]; 此外, 拟南芥编码核糖体蛋白L10基因SAC52的突变可以恢复acl5-1突变体的矮化症状[31]。在本研究的OsDWL1基因的定位区间内(图6), 已克隆了一个矮化基因, 即LOC_Os06g03710, 该基因DLT/OsG RAS-32编码一个GRAS蛋白(GAI-RGA-SCR), 其双碱基缺失突变体d62表现为矮化少蘖、宽叶浓绿等特征[32]。本研究中的osdwl1则表现为矮化少蘖、宽叶黄化(图1), 因此与d62性状不完全一样。进一步通过测序分析osdwl1中的DLT基因, 证实其序列与野生型对照一致。而与报道相关的导致水稻矮化相关的基因分别为PPR蛋白基因(LOC_Os06g03530和LOC_ Os06g03570)、细胞色素P450基因(LOC_ Os06g03930)、钙调蛋白基因(LOC_Os06g03676)、以及核糖体蛋白基因(LOC_Os06g03790) (表2)。当然, 候选基因的最终确定要依赖于这些基因的测序分析及遗传互补验证。
大量研究表明, 矮化基因一般具有“一因多效”的特点, 即矮化突变体除植株矮化外, 还伴随分蘖、叶片和籽粒等组织器官的变化[4,5]。本研究中osdwl1除表现矮化少蘖外, 叶片从苗期开始就表现为黄化宽叶特性(图1和图2)。研究表明, 叶片黄化是叶片衰老的重要外在表现, 而其内在表现则为程序性细胞死亡(programmed cell death, PCD), 并由此带来叶绿体降解、蛋白质降解、ROS (reactive oxygen species)累积、膜脂过氧化以及光合速率下降等生理生化变化[32]。因此, 叶绿素含量和叶片净光合速率是衡量叶片衰老的重要生理指标[33]。本研究结果显示, 突变体osdwl1的倒二叶和倒三叶的总叶绿素含量及其光合速率均极显著低于其野生型自选1号(图3), 预示其叶片已开始衰老(图1)。伴随叶片衰老, 突变体叶绿体也开始降解(图3), 进而造成叶绿体中的电子传递链受到抑制, 致使H2O2和O2?等ROS急剧增加[34-35] (图4-A, B)。同时, 过量ROS将作用于细胞膜脂质, 使其过氧化而产生大量MDA [34-35] (图5-A)。与此同时, 为了清除细胞内的过多ROS并保护细胞膜系统, 正常植物细胞会及时启动属于可溶性蛋白的抗氧化酶, 如SOD和CAT的表达[36,37]。其中, SOD是O2?的专一作用酶[36,37], 突变体osdwl1的剑叶、倒二叶和倒三叶的SOD酶活极显著低于野生型自选1号(图4-D), 从而导致突变体叶片中的O2?含量显著增加(图4-A)。而CAT是作用于H2O2的保护酶[36,37], 突变体osdwl1的倒二叶和倒三叶之间的CAT酶活显著低于野生型(图4-C), 致使其倒二叶和倒三叶中的H2O2显著增加(图4-B)。
4 结论
osdwl1是一个新鉴定到的矮化少蘖、宽叶黄化的突变体, 其宽叶黄化症状始于三至四叶期幼苗。与野生型对照相比, osdwl1的剑叶、倒二叶和倒三叶的叶绿素含量极显著降低, 从而导致其净光合速率极显著下降。osdwl1倒二叶和倒三叶CAT、SOD活性极显著下降, 降低其清除H2O2的能力, 导致ROS明显增加并促使细胞膜脂过氧化, 致使MDA含量极显著升高。遗传分析表明, osdwl1的矮化宽叶性状由一对隐性核基因控制, 进一步利用图位克隆技术将OsDWL1定位于6号染色体短臂的RM19297与ID269-2之间, 其间物理距离约333 kb, 这为进一步克隆该基因并揭示其矮化宽黄叶分子生理机理奠定基础。参考文献 原文顺序
文献年度倒序
文中引用次数倒序
被引期刊影响因子
[本文引用: 1]
[本文引用: 1]
[本文引用: 1]
[本文引用: 1]
[本文引用: 1]
[本文引用: 1]
DOI:10.1007/s00425-017-2798-1URLPMID:29110072 [本文引用: 11]

MAIN CONCLUSION: This review presents genetic and molecular basis of crop height using a rice crop model. Height is controlled by multiple genes with potential to be manipulated through breeding strategies to improve productivity. Height is an important factor affecting crop architecture, apical dominance, biomass, resistance to lodging, tolerance to crowding and mechanical harvesting. The impressive increase in wheat and rice yield during the 'green revolution' benefited from a combination of breeding for high-yielding dwarf varieties together with advances in agricultural mechanization, irrigation and agrochemical/fertilizer use. To maximize yield under irrigation and high fertilizer use, semi-dwarfing is optimal, whereas extreme dwarfing leads to decreased yield. Rice plant height is controlled by genes that lie in a complex regulatory network, mainly involved in the biosynthesis or signal transduction of phytohormones such as gibberellins, brassinosteroids and strigolactones. Additional dwarfing genes have been discovered that are involved in other pathways, some of which are uncharacterized. This review discusses our current understanding of the regulation of plant height using rice as a well-characterized model and highlights some of the most promising research that could lead to the development of new, high-yielding varieties. This knowledge underpins future work towards the genetic improvement of plant height in rice and other crops.
DOI:10.2183/pjab.93.014URLPMID:28413198 [本文引用: 11]

Traditional breeding for high-yielding rice has been dependent on the widespread cultivation of gibberellin (GA)-deficient semi-dwarf varieties. Dwarfism lowers the
DOI:10.1007/s11103-004-4038-xURLPMID:16217603 [本文引用: 2]

Plant architecture, a collection of the important agronomic traits that determine grain production in rice, is mainly affected by factors including tillering, plant height and panicle morphology. Recently, significant progress has been made in isolating and collecting of mutants that are defective in rice plant architecture. Although our understanding of the molecular mechanisms that control rice tillering, panicle development and plant height are still limited, new findings have begun to emerge. This review, therefore, summarizes the recent progress in exploring the mechanisms that control rice plant architecture.
URLPMID:15659436 [本文引用: 1]
URLPMID:17655651 [本文引用: 1]
URLPMID:19542179 [本文引用: 1]
DOI:10.1046/j.1365-313x.2003.01648.xURLPMID:12581309 [本文引用: 1]

Although the shoot apical meristem (SAM) is ultimately responsible for post-embryonic development in higher plants, lateral meristems also play an important role in determining the final morphology of the above-ground part. Axillary buds developing at the axils of leaves produce additional shoot systems, lateral branches. The rice TB1 gene (OsTB1) was first identified based on its sequence similarity with maize TEOSINTE BRANCHED 1 (TB1), which is involved in lateral branching in maize. Both genes encode putative transcription factors carrying a basic helix-loop-helix type of DNA-binding motif, named the TCP domain. The genetic locus of OsTB1 suggested that OsTB1 is a real counterpart of maize TB1. Transgenic rice plants overexpressing OsTB1 exhibited markedly reduced lateral branching without the propagation of axillary buds being affected. We also demonstrated that a rice strain carrying a classical morphological marker mutation, fine culm 1 (fc1), contain the loss-of-function mutation of OsTB1 and exhibits enhanced lateral branching. Expression of OsTB1, as examined with a putative promoter-glucuronidase (GUS) gene fusion, was observed throughout the axillary bud, as well as the basal part of the shoot apical meristem, vascular tissues in the pith and the lamina joint. Taking these data together, we concluded that OsTB1 functions as a negative regulator for lateral branching in rice, presumably through expression in axillary buds.
DOI:10.1111/j.1365-313X.2006.02916.xURLPMID:17092317 [本文引用: 1]

Rice tillering is an important agronomic trait for grain production. The HIGH-TILLERING DWARF1 (HTD1) gene encodes an ortholog of Arabidopsis MAX3. Complementation analyses for HTD1 confirm that the defect in HTD1 is responsible for both high-tillering and dwarf phenotypes in the htd1 mutant. The rescue of the Arabidopsis max3 mutant phenotype by the introduction of Pro(35S):HTD1 indicates HTD1 is a carotenoid cleavage dioxygenase that has the same function as MAX3 in synthesis of a carotenoid-derived signal molecule. The HTD1 gene is expressed in both shoot and root tissues. By evaluating Pro(HTD1):GUS expression, we found that the HTD1 gene is mainly expressed in vascular bundle tissues throughout the plant. Auxin induction of HTD1 expression suggests that auxin may regulate rice tillering partly through upregulation of HTD1 gene transcription. Restoration of dwarf phenotype after the removal of axillary buds indicates that the dwarfism of the htd1 mutant may be a consequence of excessive tiller production. In addition, the expression of HTD1, D3 and OsCCD8a in the htd1 and d3 mutants suggests a feedback mechanism may exist for the synthesis and perception of the carotenoid-derived signal in rice. Characterization of MAX genes in Arabidopsis, and identification of their orthologs in pea, petunia and rice indicates the existence of a conserved mechanism for shoot-branching regulation in both monocots and dicots.
URLPMID:31521223 [本文引用: 1]
DOI:10.1270/jsbbs.16198URLPMID:29085249 [本文引用: 1]

Grain size is a trait that is important for rice (Oryza sativa L.) yield potential. Many genes regulating grain size have been identified, deepening our understanding of molecular mechanisms of grain size determination in rice. Previously, we cloned SMALL AND ROUND SEED 5 (SRS5) gene (encoding alpha-tubulin) from a small and round seed mutant and revealed that this gene regulates grain length independently of the brassinosteroid (BR) signaling pathway, although BR-related mutants set small grain. In this study, we showed that overexpression of SRS5 can promote grain length and demonstrated that the overexpression of SRS5 in BR-related mutants rescued the shortened grain length, which is an unfavorable phenotype in the yield potential of BR-related mutants, while preserving the useful semi-dwarf and erect leaf phenotypes.
DOI:10.1016/j.bbrc.2014.12.034URLPMID:25522878 [本文引用: 1]

To better understand the genetic of rice agronomic traits, we selected two different rice germplasms in phenotypes, Xian80 and Suyunuo, to construct genetic population for QTL analysis. A total of 25 QTLs for six traits were found in a 175 F2 population. Major QTLs, qPH12,qLW12.2, qLL12 and qGW12.1, explaining 50.00%, 57.08%, 15.41% and 22.51% phenotypic variation for plant height, leaf width, leaf length and grain width, respectively, were located on the same interval of chromosome 12 flanking SSR markers RM519 and RM1103. In consideration of the great effects on plant height and leaf width, the locus was named DNL1 (Dwarf and Narrowed Leaf 1). Using a segregating population derived from F2 heterozygous individuals, a total of 1363 dwarfism and narrowed-leaf individuals was selected for screening recombinants. By high-resolution linkage analysis in 141 recombination events, DNL1 was narrowed to a 62.39kb region of InDel markers ID12M28 and HF43. The results of ORF analysis in target region and nucleotide sequence alignment indicated that DNL1 encodes cellulose synthase-like D4 protein, and a single nucleotide substitution (C2488T) in dnl1 result in decrease in plant height and leaf width. Bioinformatical analysis demonstrated that a conserved role for OsCSLD4 in the regulation of plant growth and development. Expression analysis for OsCSLDs showed OsCSLD4 highly expressed in roots, while other CSLD members had comparatively lower expression levels. However, no clear evidence about CSLD4/DNL1 expression was associated with its function.
URLPMID:19220793 [本文引用: 2]
DOI:10.3724/SP.J.1006.2013.00767URL [本文引用: 1]

叶片形态是水稻“理想株型”的重要组成部分,是当前水稻高产育种关注的重点。本文通过对已克隆多个叶形相关调控基因综述了水稻叶片形态(叶片卷曲度、倾角、披散程度以及叶片宽度)建成的分子遗传学研究进展。综合分析认为,水稻叶片的卷曲主要是通过卷叶基因调控叶片近轴/远轴间的发育、泡状细胞的发育及其膨胀和渗透压、厚壁组织的形成以及叶片角质层的发育等来实现。影响植株空间伸展姿态的叶倾角主要通过叶角基因调控油菜素内酯的信号传导来影响叶枕细胞的生长发育;唯一被克隆的影响叶片披垂度的披叶基因DL1是通过控制叶片中脉发育而改变叶片形态的;而窄叶基因则主要通过调控生长素的合成与极性运输、维管组织的发育和分布,影响叶片维管束数目及宽度。但到目前为止,所有已克隆的叶形调控基因间相互调控关系的研究还不够深入,还不能完整清晰地勾勒水稻叶形建成和发育的分子调控网络。因此,在已有的研究基础上更深入地探索水稻叶片形态建成的分子调控机制,对进一步构建相关的调控网络,塑造水稻理想株型具有重要意义。]]>
[本文引用: 1]
DOI:10.1016/j.pbi.2004.11.009URLPMID:15653401 [本文引用: 1]

Shoots are characterized by indeterminate growth resulting from divisions of undifferentiated cells in the central region of the shoot apical meristem. These cells give rise to peripheral derivatives from which lateral organ initials are recruited. During initial stages of cell recruitment, the three-dimensional form of lateral organs is specified. Lateral organs such as leaves develop and differentiate along proximodistal (base-to-tip), dorsoventral (top-to bottom) and mediolateral (middle-to-margin) planes. Current findings are refining our knowledge of the genes and genetic interactions that regulate these early processes and are providing a picture of how these pathways may contribute to variation in leaf form.
DOI:10.1104/pp.108.118778URLPMID:18562767 [本文引用: 1]

The size and shape of the plant leaf is an important agronomic trait. To understand the molecular mechanism governing plant leaf shape, we characterized a classic rice (Oryza sativa) dwarf mutant named narrow leaf1 (nal1), which exhibits a characteristic phenotype of narrow leaves. In accordance with reduced leaf blade width, leaves of nal1 contain a decreased number of longitudinal veins. Anatomical investigations revealed that the culms of nal1 also show a defective vascular system, in which the number and distribution pattern of vascular bundles are altered. Map-based cloning and genetic complementation analyses demonstrated that Nal1 encodes a plant-specific protein with unknown biochemical function. We provide evidence showing that Nal1 is richly expressed in vascular tissues and that mutation of this gene leads to significantly reduced polar auxin transport capacity. These results indicate that Nal1 affects polar auxin transport as well as the vascular patterns of rice plants and plays an important role in the control of lateral leaf growth.
[本文引用: 1]
[本文引用: 1]
DOI:10.1007/s11103-019-00848-4URLPMID:30843130 [本文引用: 1]

KEY MESSAGE: The OsPLS2 locus was isolated and cloned by map-based cloning that encodes a Upf1-like helicase. Disruption of OsPLS2 accelerated light-dependent leaf senescence in the rice mutant of ospls2. Leaf senescence is a very complex physiological process controlled by both genetic and environmental factors, however its underlying molecular mechanisms remain elusive. In this study, we report a novel Oryza sativa premature leaf senescence mutant (ospls2). Through map-based cloning, a G-to-A substitution was determined at the 1st nucleotide of the 13th intron in the OsPLS2 gene that encodes a Upf1-like helicase. This mutation prompts aberrant splicing of OsPLS2 messenger and consequent disruption of its full-length protein translation, suggesting a negative role of OsPLS2 in regulating leaf senescence. Wild-type rice accordingly displayed a progressive drop of OsPSL2 protein levels with age-dependent leaf senescence. Shading and light filtration studies showed that the ospls2 phenotype, which was characteristic of photo-oxidative stress and reactive oxygen species (ROS) accumulation, was an effect of irritation by light. When continuously exposed to far-red light, exogenous H2O2 and/or abscisic acid (ABA), the ospls2 mutant sustained hypersensitive leaf senescence. In consistence, light and ROS signal pathways in ospls2 were activated by down-regulation of phytochrome genes, and up-regulation of PHYTOCHROME-INTERACTING FACTORS (PIFs) and WRKY genes, all promoting leaf senescence. Together, these data indicated that OsPLS2 played an essential role in leaf senescence and its disruption triggered light-dependent leaf senescence in rice.
DOI:10.1071/CP12065URL [本文引用: 1]

Narrow-leafed lupin (Lupinus angustifolius L.) is an important grain legume crop in Australia. Metribuzin is an important herbicide used to control weeds in lupin crops. This study investigated metribuzin tolerance mechanism in narrow-leafed lupin by comparing two induced mutants (Tanjil-AZ-33 and Tanjil-AZ-55) of higher metribuzin tolerance with the susceptible wild type. Sequencing of the highly conserved region of the chloroplast psbA gene (target site) revealed that the sequences of the wild type and the mutants were identical and therefore metribuzin tolerance is not target site based. Photosynthetic activity was measured and the leaf photosynthesis of the two tolerant mutants was initially inhibited after metribuzin treatment, but recovered within 2.5 days whereas that of the susceptible plants remained inhibited. The photosynthetic measurements confirmed the target site chloroplast was susceptible and the tolerance mechanism is nontarget site based. Investigation with known cytochrome P450 monooxygenase inhibitors (omethoate, malathion and phorate) showed that tolerance could be reversed in both mutants, indicating the tolerance mechanism in two tolerant mutants may involve cytochrome P450 enzymes. Interestingly, the inhibitor tridiphane reversed metribuzin tolerance of only one of the two tolerant mutants, indicating diversity in metribuzin tolerance mechanisms in narrow-leafed lupin. These results signify that further investigation of metribuzin metabolism in these plants is warranted. In conclusion, metribuzin tolerance mechanism in lupin mutants is non-target site based, likely involving P450-mediated metribuzin metabolism.
DOI:10.1371/journal.pone.0161203URLPMID:27532299 [本文引用: 1]

D1 protein in the PSII reaction center is the major target of photodamage, and it exhibits the highest turnover rate among all the thylakoid proteins. In this paper, rice psf (premature senescence of flag leaves) mutant and its wild type were used to investigate the genotype-dependent alteration in PSII photo-damage and D1 protein turnover during leaf senescence and its relation to ABA accumulation in senescent leaves. The symptom and extent of leaf senescence of the psf mutant appeared to be sunlight-dependent under natural field condition. The psf also displayed significantly higher levels of ABA accumulation in senescent leaves than the wild type. However, the premature senescence lesion of psf leaves could be alleviated by shaded treatment, concomitantly with the strikingly suppressed ABA level in the shaded areas of flag leaves. The change in ABA concentration contributed to the regulation of shade-delayed leaf senescence. The participation of ABA in the timing of senescence initiation and in the subsequent rate of leaf senescence was closely associated with PSII photodamage and D1 protein turnover during leaf senescence, in which the transcriptional expression of several key genes (psbA, psbB, psbC and OsFtsH2) involved in D1 protein biosynthesis and PSII repair cycle was seriously suppressed by the significantly increased ABA level. This response resulted in the low rate of D1 protein synthesis and impaired repair recovery in the presence of ABA. The psf showed evidently decreased D1 protein amount in the senescent leaves. Both the inhibition of de novo synthesized D1 protein and the slow rate of proteolytic removal for the photodamaged D1 protein was among the most crucial steps for the linkage between light-dependent leaf senescence and the varying ABA concentration in psf mutant leaves. OsFtsH2 transcriptional expression possibly played an important role in the regulation of D1 protein turnover and PSII repair cycle in relation to ABA mediated leaf senescence.
URLPMID:26994476 [本文引用: 1]
URL [本文引用: 1]
URL [本文引用: 1]
[本文引用: 1]
[本文引用: 1]
URLPMID:19453459 [本文引用: 1]
URLPMID:23902579 [本文引用: 1]
DOI:10.1038/nature03973URLPMID:16193053 [本文引用: 1]

Brassinosteroids are plant-specific steroid hormones that have an important role in coupling environmental factors, especially light, with plant growth and development. How the endogenous brassinosteroids change in response to environmental stimuli is largely unknown. Ca2+/calmodulin has an essential role in sensing and transducing environmental stimuli. Arabidopsis DWARF1 (DWF1) is responsible for an early step in brassinosteroid biosynthesis that converts 24-methylenecholesterol to campesterol. Here we show that DWF1 is a Ca2+/calmodulin-binding protein and this binding is critical for its function. Molecular genetic analysis using site-directed and deletion mutants revealed that loss of calmodulin binding completely abolished the function of DWF1 in planta, whereas partial loss of calmodulin binding resulted in a partial dwarf phenotype in complementation studies. These results provide direct proof that Ca2+/calmodulin-mediated signalling has a critical role in controlling the function of DWF1. Furthermore, we observed that DWF1 orthologues from other plants have a similar Ca2+/calmodulin-binding domain, implying that Ca2+/calmodulin regulation of DWF1 and its homologues is common in plants. These results raise the possibility of producing size-engineered crops by altering the Ca2+/calmodulin-binding property of their DWF1 orthologues.
URLPMID:31227696 [本文引用: 1]
DOI:10.1111/j.1365-313X.2008.03647.xURLPMID:18694459 [本文引用: 1]

Disruption of the Arabidopsis thaliana ACAULIS5 (ACL5) gene, which has recently been shown to encode thermospermine synthase, results in a severe dwarf phenotype. A previous study showed that sac51-d, a dominant suppressor mutant of acl5-1, has a premature termination codon in an upstream open reading frame (ORF) of SAC51, which encodes a putative transcription factor, and suggested the involvement of upstream ORF-mediated translational control in ACL5-dependent stem elongation. Here we report the identification of a gene responsible for sac52-d, another semi-dominant suppressor mutant of acl5-1. SAC52 encodes ribosomal protein L10 (RPL10A), which is highly conserved among eukaryotes and implicated in translational regulation. Transformation of acl5-1 mutants with a genomic fragment containing the sac52-d allele rescued the dwarf phenotype of acl5-1. GUS reporter activity under the control of a SAC51 promoter with its upstream ORF was higher in acl5-1 sac52-d than in acl5-1, suggesting that suppression of the acl5-1 phenotype by sac52-d is attributable, in part, to enhanced translation of certain transcripts including SAC51. We also found that a T-DNA insertion allele of SAC52/RPL10A causes lethality in the female gametophyte.
DOI:10.1007/s00425-010-1263-1URLPMID:20830595 [本文引用: 2]

A dwarf mutant, dwarf 62 (d62), was isolated from rice cultivar 93-11 by mutagenesis with gamma-rays. Under normal growth conditions, the mutant had multiple abnormal phenotypes, such as dwarfism, wide and dark-green leaf blades, reduced tiller numbers, late and asynchronous heading, short roots, partial male sterility, etc. Genetic analysis indicated that the abnormal phenotypes were controlled by the recessive mutation of a single nuclear gene. Using molecular markers, the D62 gene was fine mapped in 131-kb region at the short arm of chromosome 6. Positional cloning of D62 gene revealed that it was the same locus as DLT/OsGRAS-32, which encodes a member of the GRAS family. In previous studies, the DLT/OsGRAS-32 is confirmed to play positive roles in brassinosteroid (BR) signaling. Sequence analysis showed that the d62 carried a 2-bp deletion in ORF region of D62 gene which led to a loss-of-function mutation. The function of D62 gene was confirmed by complementation experiment. RT-PCR analysis and promoter activity analysis showed that the D62 gene expressed in all tested tissues including roots, stems, leaves and panicles of rice plant. The d62 mutant exhibited decreased activity of alpha-amylase in endosperm and reduced content of endogenous GA(1). The expression levels of gibberellin (GA) biosynthetic genes including OsCPS1, OsKS1, OsKO1, OsKAO, OsGA20ox2/SD1 and OsGA2ox3 were significantly increased in d62 mutant. Briefly, these results demonstrated that the D62 (DLT/OsGRAS-32) not only participated in the regulation of BR signaling, but also influenced GA metabolism in rice.
DOI:10.1146/annurev.arplant.57.032905.105316URLPMID:17177638 [本文引用: 1]

Leaf senescence constitutes the final stage of leaf development and is critical for plants' fitness as nutrient relocation from leaves to reproducing seeds is achieved through this process. Leaf senescence involves a coordinated action at the cellular, tissue, organ, and organism levels under the control of a highly regulated genetic program. Major breakthroughs in the molecular understanding of leaf senescence were achieved through characterization of various senescence mutants and senescence-associated genes, which revealed the nature of regulatory factors and a highly complex molecular regulatory network underlying leaf senescence. The genetically identified regulatory factors include transcription regulators, receptors and signaling components for hormones and stress responses, and regulators of metabolism. Key issues still need to be elucidated, including cellular-level analysis of senescence-associated cell death, the mechanism of coordination among cellular-, organ-, and organism-level senescence, the integration mechanism of various senescence-affecting signals, and the nature and control of leaf age.
URLPMID:27135335 [本文引用: 2]
[本文引用: 2]
DOI:10.1093/aob/mcf118URLPMID:12509339 [本文引用: 3]

Oxidative stress is induced by a wide range of environmental factors including UV stress, pathogen invasion (hypersensitive reaction), herbicide action and oxygen shortage. Oxygen deprivation stress in plant cells is distinguished by three physiologically different states: transient hypoxia, anoxia and reoxygenation. Generation of reactive oxygen species (ROS) is characteristic for hypoxia and especially for reoxygenation. Of the ROS, hydrogen peroxide (H(2)O(2)) and superoxide (O(2)(.-)) are both produced in a number of cellular reactions, including the iron-catalysed Fenton reaction, and by various enzymes such as lipoxygenases, peroxidases, NADPH oxidase and xanthine oxidase. The main cellular components susceptible to damage by free radicals are lipids (peroxidation of unsaturated fatty acids in membranes), proteins (denaturation), carbohydrates and nucleic acids. Consequences of hypoxia-induced oxidative stress depend on tissue and/or species (i.e. their tolerance to anoxia), on membrane properties, on endogenous antioxidant content and on the ability to induce the response in the antioxidant system. Effective utilization of energy resources (starch, sugars) and the switch to anaerobic metabolism and the preservation of the redox status of the cell are vital for survival. The formation of ROS is prevented by an antioxidant system: low molecular mass antioxidants (ascorbic acid, glutathione, tocopherols), enzymes regenerating the reduced forms of antioxidants, and ROS-interacting enzymes such as SOD, peroxidases and catalases. In plant tissues many phenolic compounds (in addition to tocopherols) are potential antioxidants: flavonoids, tannins and lignin precursors may work as ROS-scavenging compounds. Antioxidants act as a cooperative network, employing a series of redox reactions. Interactions between ascorbic acid and glutathione, and ascorbic acid and phenolic compounds are well known. Under oxygen deprivation stress some contradictory results on the antioxidant status have been obtained. Experiments on overexpression of antioxidant production do not always result in the enhancement of the antioxidative defence, and hence increased antioxidative capacity does not always correlate positively with the degree of protection. Here we present a consideration of factors which possibly affect the effectiveness of antioxidant protection under oxygen deprivation as well as under other environmental stresses. Such aspects as compartmentalization of ROS formation and antioxidant localization, synthesis and transport of antioxidants, the ability to induce the antioxidant defense and cooperation (and/or compensation) between different antioxidant systems are the determinants of the competence of the antioxidant system.
DOI:10.3389/fpls.2015.01092URLPMID:26697045 [本文引用: 3]

Abiotic stresses such as drought, cold, salt and heat cause reduction of plant growth and loss of crop yield worldwide. Reactive oxygen species (ROS) including hydrogen peroxide (H2O2), superoxide anions (O2 (*-)), hydroxyl radical (OH*) and singlet oxygen ((1)O2) are by-products of physiological metabolisms, and are precisely controlled by enzymatic and non-enzymatic antioxidant defense systems. ROS are significantly accumulated under abiotic stress conditions, which cause oxidative damage and eventually resulting in cell death. Recently, ROS have been also recognized as key players in the complex signaling network of plants stress responses. The involvement of ROS in signal transduction implies that there must be coordinated function of regulation networks to maintain ROS at non-toxic levels in a delicate balancing act between ROS production, involving ROS generating enzymes and the unavoidable production of ROS during basic cellular metabolism, and ROS-scavenging pathways. Increasing evidence showed that ROS play crucial roles in abiotic stress responses of crop plants for the activation of stress-response and defense pathways. More importantly, manipulating ROS levels provides an opportunity to enhance stress tolerances of crop plants under a variety of unfavorable environmental conditions. This review presents an overview of current knowledge about homeostasis regulation of ROS in crop plants. In particular, we summarize the essential proteins that are involved in abiotic stress tolerance of crop plants through ROS regulation. Finally, the challenges toward the improvement of abiotic stress tolerance through ROS regulation in crops are discussed.