1.
Introduction
Diamond is a very excellent wide band gap semiconductor material, and it has much more superior physical properties when compared to other currently used wide band gap semiconductor materials such as SiC and GaN. For the high quality diamond, the electron and hole mobility reach as high as 4500 and 3800 cm2/(V·s)[1], respectively; its breakdown electric field strength reaches 10 MV/cm[2]; its thermal conductivity can be as high as 22 W/(cm·K)[3]; the dielectric constant can be as low as 5.5[4]; so it can be said to be the ultimate semiconductor[4]. The excellent electrical, thermal, and optical properties of diamond make it well suited for deep ultraviolet (DUV) detectors, LEDs[5], and high-energy particle detectors[6], as well as high-temperature, high-frequency, and high-power devices such as Schottky barrier diodes[7], field effect transistors[8, 9], and other devices.
High-performance diamond devices largely depend on the crystal quality of the diamond epitaxial layer[10, 11]. During the epitaxial growth of high-quality single crystal diamond layers, the impurity atoms may be incorporated into the diamond epitaxial layer, which leads to degradation of the diamond epitaxial layer quality. In order to improve the quality of the diamond epitaxial layers grown by means of microwave plasma chemical vapor deposition (MPCVD), Sakaguchi et al.[12–14] introduced a small amount of oxygen to the reaction chamber, and they found that the addition of oxygen can effectively inhibit impurity atoms (such as silicon atoms, boron atoms, etc.) from being incorporated into diamond epilayers with a thickness of 200 μm. The authors ascribed the reduction of the chemical impurities in the epitaxial layer to the possible chemical reactions between oxygen and impurity atoms to form oxides, which cannot be incorporated into the diamond epitaxial layer due to their relatively large volume per molecule[12–14]. In addition, the authors[12–14] also found that the addition of oxygen can effectively suppress cracks in the diamond epitaxial layer. In their epitaxial growth of diamond, Das et al.[15] found that when oxygen is added to the growth chamber, OH radical groups will appear in the plasma, which can etch the non-diamond phase, such as graphite etc., in the diamond epilayers. Tallaire et al.[16] also introduced oxygen into their growth chamber during the epitaxial growth of diamond, and they found that the OH radical groups in the plasma can effectively etch the defects on the surface of the diamond substrate, leading to improvement of the quality of the diamond epitaxial layer. Stehl et al.[17] grew heteroepitaxial diamond epilayers with different thicknesses on an iridium substrate under the oxygen-free condition, and performed the Raman spectroscopy measurement on their diamond samples; the Raman measurement showed that as the thickness of the diamond epitaxial layer increases, the full width at half maximum of the Raman characteristic peak narrows, indicating that the dislocation density in the diamond epitaxial layer decreases as the thickness of the epitaxial layer increases.
As mentioned above, the addition of oxygen to the epitaxial growth chamber and increasing the film thickness can both improve the quality of the diamond epilayers. It is necessary to investigate the interplay of the introduction of oxygen and the variation of the epilayers thickness in the epitaxial growth of diamond by means of MPCVD. In this work, we added oxygen to the growth chamber and, at the same time, to investigate the effect of the variation in the thickness of the diamond epilayers up to 1.7 mm on their optical properties and crystallinity.
2.
Experiment
In this work, considering that it is difficult to obtain a diamond epitaxial layer of high crystallinity on a substrate with lattice mismatch in heteroepitaxial growth[17], we choose homoepitaxial growth on the (100) diamond substrate. Diamond homoepitaxial growth was performed by means of MPCVD. The diamond substrate is obtained by the high pressure and high temperature method, and the crystallographic orientation of the diamond substrate is (100). The diamond substrates were firstly etched in the hydrogen plasma for 30 min just before growth. The epitaxial growth parameters were as follows: microwave power was 5 kW, growth pressure was 160 torr, growth temperature was 1150 °C, the hydrogen flow rate was 500 sccm, the methane flow rate was 30 sccm, the oxygen flow rate was 0.5 sccm. Four diamond samples were grown and these are labeled as D21, D22, D23, and D24 with the epilayer thicknesses of 1.027, 1.337, 1.768, and 1.784 mm, respectively. The crystallinity of the diamond epitaxial layers was characterized by X-ray diffraction (XRD). The optical properties of the diamond samples are studied by laser Raman spectroscopy and DUV photoluminescence spectroscopy. The experimental parameters used for the Raman measurement were as follows: laser wavelength was 514.5 nm, and laser power was 0.8 mW. The parameters of the diamond deep ultraviolet photoluminescence spectrum were as follows: the laser wavelength was 177 or 213 nm, and the sample temperature was 10 K.
3.
Results and discussion
Fig. 1 shows the XRD spectrum of the (004) plane of the diamond sample D21. The diffraction peak at the (004) plane of 119.4° from the sample D21 can be clearly observed without other peaks observed in Fig. 1, which indicates that the diamond epitaxial layer, as a single crystal, has good crystallinity with the growth direction of the diamond epitaxial layer being along the (100) direction. The growth direction of the D22, D23, and D24 diamond samples is along the (100) direction. Fig. 2(a) shows the rocking curve of sample D21, which has a typical shape for diamond. Fig. 2(b) shows the variation of the full width at half maximum (FWHM) with the sample thickness. It can be seen from the figure that the FWHM of the rocking curve increases as the sample thickness increases. Sample D21 has the thickness of 1.027 mm, and the FWHM of its rocking curve is 216.1 arcsec; sample D22 is of 1.337 mm in thickness and its FWHM in the rocking curve is and 250.5 arcsec; D24 has the thickness of 1.784 mm, and its FWHM remarkably increases up to 690.1 arcsec, three times that of sample D21.

class="figure_img" id="Figure1"/>
Download
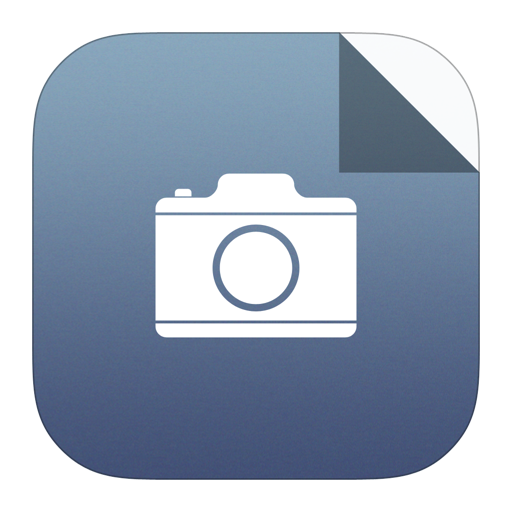
Larger image
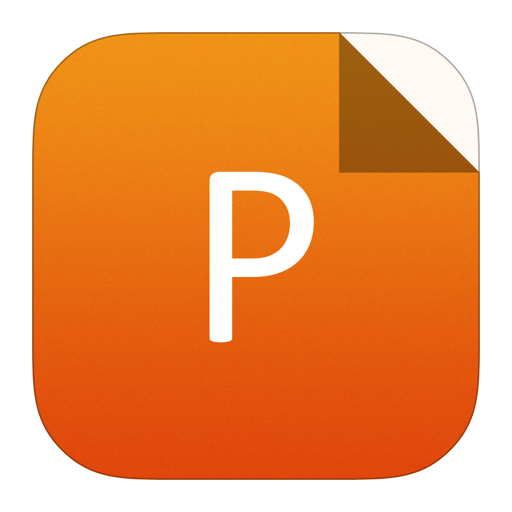
PowerPoint slide
Figure1.
XRD spectrum of diamond sample D21.
It is well known that the FWHM of the rocking curve of a crystal sample reflects its crystallinity: the narrower the FWHM of the rocking curve, the better the crystal quality and fewer defects within the crystal sample. Therefore, based on the experimental results presented in Fig. 2(b), it can be concluded that as the thickness of the epitaxial layer of the oxygen-doped diamond sample increases, the quality and crystallinity of diamond samples degrades.

class="figure_img" id="Figure2"/>
Download
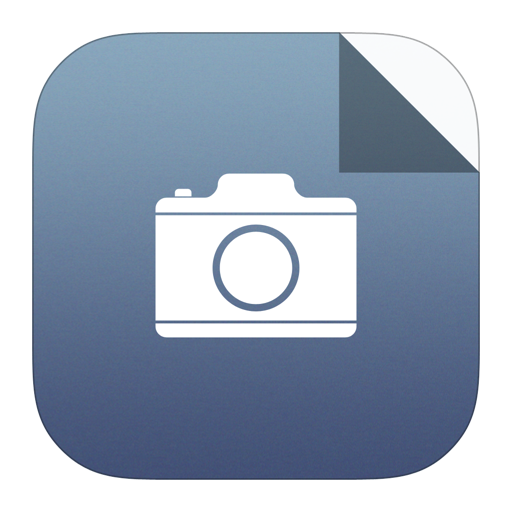
Larger image
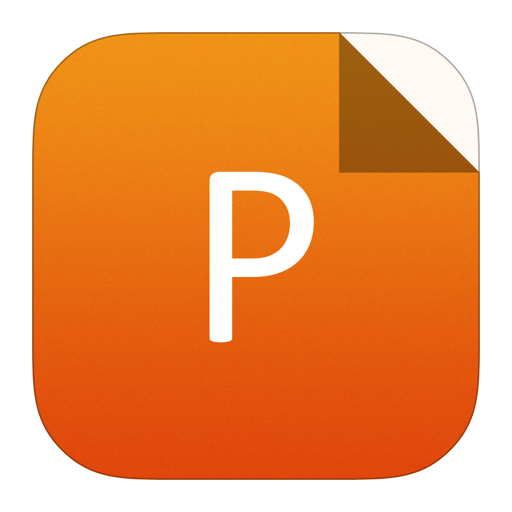
PowerPoint slide
Figure2.
(a) The rocking curve of the (004) plane of diamond sample D21. (b) The full width at half maximum of the rocking curve of the (004) plane of different thickness diamond samples D21, D22, and D24.
The conclusion that the quality of the diamond epilayers degrades with increasing thickness is further confirmed by the measurements on the optical property. The conclusion that the quality of the diamond epilayers degrades with increasing thickness is further confirmed by the measurements on the optical property. The Raman spectrum was used to study the diamond optical properties. Fig. 3(a) shows the laser Raman spectra from diamond samples D21, D22, and D23. The diamond Raman characteristic peak at 1332.5 cm?1[18] can be clearly observed from all the three samples, as observed from the figure. It should be noted that, except for the characteristic peak of diamond at 1332.5 cm?1, no graphite characteristic peak near 1580 cm?1, as well as other peaks, was observed in the Raman spectrum. This indicates that the diamond epitaxial layer has only the diamond phase and none of the non-diamond phase, such as graphite, etc. Fig. 3(b) shows the variation of the peak intensity in the Raman spectra of diamond epitaxial layers of different thickness samples. It is found that as the thickness of the diamond epitaxial layer increases, the intensity of its Raman peak decreases, which implies that the quality of the diamond epitaxial layer gets worse with increasing thickness.

class="figure_img" id="Figure3"/>
Download
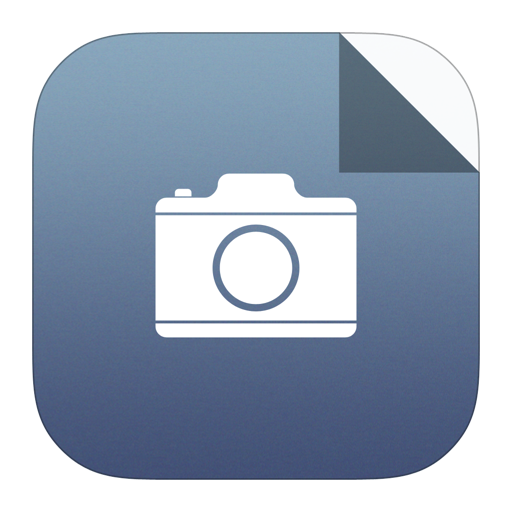
Larger image
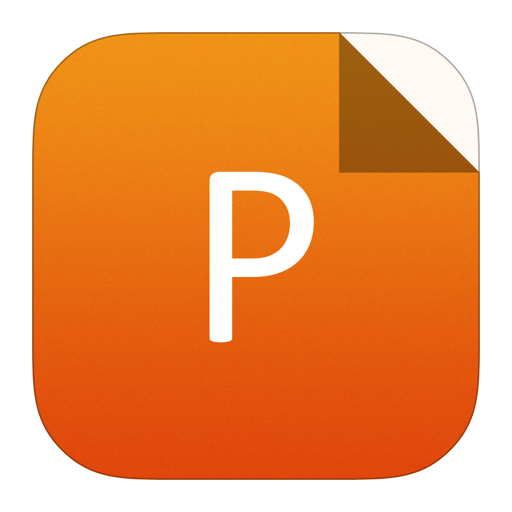
PowerPoint slide
Figure3.
(Color online) (a) Raman spectrum of different diamond samples. (b) Raman characteristic peak intensity varies with the thickness of the epitaxial layer.
Figs. 4(a) and 4(b) show the low-temperature (10 K) PL spectra near the bandgap of diamond samples D21, D22, and D23. From Fig. 4(a), the strong free exciton emission peaks A1 and B1 of the diamond sample can be clearly observed. At the same time, the phonon replica B2 and B3 of the B1 peak of the diamond single crystal can also be observed, which shows that the quality of diamond samples is relatively high. Fig. 4(b) shows that as the thickness of the diamond epitaxial layer increases, the intensity of the B1 peak of the diamond gradually decreases. The intensity of the free exciton emission peak B1 in the diamond PL spectrum can characterize the quality of the diamond sample. The stronger the intensity of the free exciton emission peak, the better the quality of the diamond.

class="figure_img" id="Figure4"/>
Download
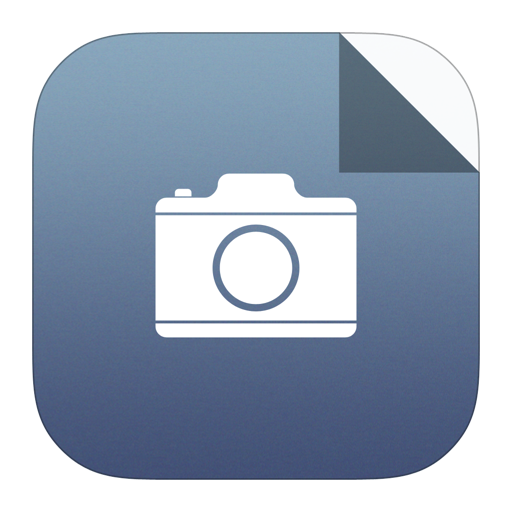
Larger image
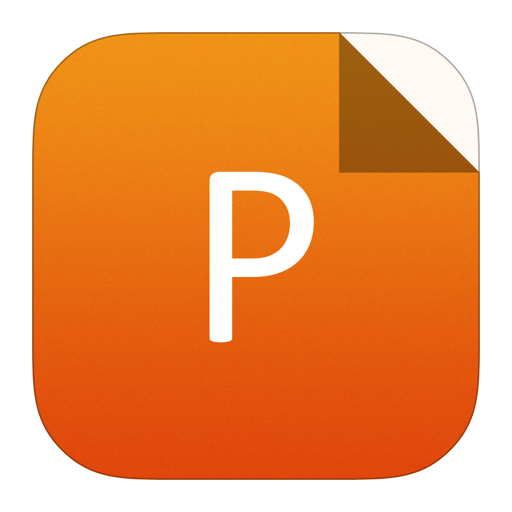
PowerPoint slide
Figure4.
(Color online) (a) Low-temperature (10 K) photoluminescence (PL) spectrum of different diamond samples. (b) Intensity of B1 peak changes with the thickness of the epitaxial layer.
From the characterization of the quality of our diamond samples by means of X-ray, Raman, and PL spectrum, it can be concluded that the optical quality as well as crystallinity of the diamond epilayer grown with the introduction of oxygen degrades to some extent with increasing layer thickness. This experimental result is in contrast with the results of the epitaxial growth under the oxygen-free condition performed by Stehl et al.[17], where the crystallinity of the diamond epilayers heteroepitaxially grown on the iridium substrate is improved as the thickness of the epilayers increases.
In order to grow high quality diamond epitaxial layers, it is necessary to prevent the formation of defects during the epitaxial growth[18]. It is well known that there are two main types of sources of defects in the growth process of diamond epitaxial layer. One type is from the substrate; for example, it is well known that the dislocations in the substrate can propagate into the epilayers as threading dislocations. The other type of defect source is due to growth conditions or the presence of impurities during diamond growth[19, 20]. In their heteroepitaxial growth of diamond, Stehl et al.[17] used iridium as the substrate, whose lattice is face-centered cubic, and the lattice mismatch between the substrate and diamond is about 7%. Therefore, a large amount of misfit dislocations would propagate, as threading dislocations, into the diamond epilayers from the substrate/epilayers interface. It is well known that the amount of threading dislocations in an epitaxial layer decreases as its thickness increases, which is due to the fact that threading dislocations that are close enough to each other will eventually merge together and the total density of threading dislocations[21, 22] is gradually reduced. Hence, the crystallinity of the diamond layer improves as its thickness increases, as observed by Stehl[17]. During the epitaxial growth of diamond, if the density of defects (such as threading dislocations) is very high, these defects will merge with each other, leading to a decrease in defect density and improving the quality of crystal, as the epilayer grows. In comparison with heteroepitaxial diamond layers, the defect density is much lower in a homoepitaxial diamond layer; therefore, the defect-merging effect may be insignificant.
In general, during the homoepitaxial growth of diamond by MPCVD, after the diamond substrate was etched by the H2/O2 plasma, many etch pits appeared on the diamond substrate surface, which indicates that the OH radical groups in the plasma have a strong etching ability[23, 24]. In our growth experiment, when oxygen molecules were added to the reaction chamber, these molecules are decomposed into oxygen atoms in the plasma; these oxygen atoms further combine with the hydrogen atoms to form OH radical groups. OH is the most stable structure on the oxygenation diamond surface[25, 26]. These OH radical groups have a much stronger etching effect on the diamond than the hydrogen atoms, which results in many more new defects on the diamond growth surface, as the epitaxial growth continues, degrading the crystallinity of the diamond epilayer continuously as its thickness increases.
4.
Conclusion
In conclusion, we have studied the interplay of the introduction of oxygen with increasing the thickness of the diamond layer in the homoepitaxial growth by MPCVD. We found that as the thickness of the diamond increases, the crystallinity of diamond degrades. This phenomenon can be ascribed to the enhanced etching effect of the OH radical group formed in the reaction chamber after oxygen was introduced into the reaction chamber.