1.
Introduction
Tin disulfide, first synthesized about 200 years ago[1], has been applied to various optoelectronic devices and solar collectors[2–11]. From the experimental perspective, it is a layered semiconductor with a CdI2-type structure in which the two close-packed sheets of sulfur atoms sandwich the sheets of tin atoms[12]. Including the synthesized layers, SnS2 thin films have attracted much attention since their discovered in 2014[13]. Thereafter, several stoichiometric compounds have been used as the reference for fabricating compounds with different tin-to-sulfur ratios[1, 14, 15] such as SnS (orthorhombic), Sn2S3 (rhombic), Sn3S4 (tetragonal), and SnS2 (trigonal)[16–22]. SnS contains more than 70 poly-type structures[2]. To use silicon as the converter of solar to electrical energy, several alternatives have been introduced, such as SnS, the band gap of which is estimated as 1.2 eV and it is comparable with that of silicon[20, 23]. As another alternative, SnS2, with a band gap of 2.2 eV, is suitable for photoconductive and photo-electrochemical cells[16, 20, 24]. SnS2 is a layered hexagonal CdI2-type structure (a = 0.3648 nm, c = 0.5899 nm, space group P3m1), containing tin atoms sandwiched between two layers of hexagonally disposed close-packed sulfur atoms[25, 26].
Most of the solar spectra are absorbed above the band gap by SnS layers of 1 mm thickness. Sensors, lithium micro-batteries, photovoltaics, photo-electrochemical (PEC), photoconductive cells, intercalation battery systems, and several other devices are only a few of the promising candidates for the application of this material in the different branches of science[4–9, 27]. Although these materials abundantly exist in nature[10], their optical and electrical properties can be controlled by doping with impurities[28, 29].
Several methods are used for the production of thin films, including electrodeposition[4], chemical vapour deposition[5], evaporation[30], the spin coating technique[31], spray pyrolysis[32], successive ionic layer adsorption and reaction (SILAR)[33], and chemical vapor transport[34]. The present study aims to investigate the effects of the substrate temperature on the physical properties of chemically sprayed SnS2:Cu thin films for photovoltaic applications. It is worth mentioning that the spray pyrolysis technique is used in this study for preparing large-area and low-temperature thin films at temperatures below 500 °C due to its versatility, viability, and low cost[35].
2.
Experimental procedure
2.1
Deposition of the Cu-doped SnS2 thin films by spray pyrolysis
First, the glass substrates and deposited films were coated by using the spray pyrolysis technique, as shown schematically in Ref. [36]. Then, tin chloride (SnCl4·5H2O, 7.012 g) was dissolved in deionized (DI) water (50 mL) to produce a 0.4 M Sn solvent. The mixture was added to thiourea (H2N CS NH2, 0.761 g) to obtain 0.2 M sulfur. After that, it was added to copper chloride (0.269 g) to produce 0.04 M copper chloride. All of the mixtures gave SnS2 thin films with 1 % Cu impurity. Finally, the precursor was sprayed onto a glass substrate at different temperatures (Ts = 400, 425, or 450 °C).
The glass substrates were cleaned and placed on a rotating hot plate under the conditions mentioned in Table 1. The fabricated thin films were finally deposited on the substrate. It should be said that the experiments were performed under the same conditions and with short time intervals to prevent the rapid reduction of the hot plate’s temperature.
Distance of nozzle from substrate (cm) | Pressure of carrier gas (atm) | Deposition rate | Volume of sprayed solvent (mL) |
35 | 3 | 10 | 50 |
Table1.
Conditions of deposition.
Table options
-->

Download as CSV
Distance of nozzle from substrate (cm) | Pressure of carrier gas (atm) | Deposition rate | Volume of sprayed solvent (mL) |
35 | 3 | 10 | 50 |
2.2
Characterization of deposited SnS2:Cu thin films
The structural, morphological, optical, and electrical properties of SnS2:Cu thin films have been studied here by using various techniques. First, the X-ray diffraction (XRD) patterns of the films were recorded to investigate their structural properties. The analysis was conducted with a D8 Advanced Bruker system using CuKα radiation (λ = 0.154056 nm) with 2θ ranging from 10° to 70°.
Then, the morphological analysis was performed by using scanning electron microscopy (SEM; KYKY EM 3200) with a LEO 1450 VP system.
Finally, the optical absorption (UV–vis) and films transparency were studied and measured at 300–1100 nm by using an Agilent 8453 UV–vis double beam spectrophotometer at room temperature. The thickness of the layers was measured by using a Taly step profilometer[37] (roughness detector with a stylus–Taylor Hobson Model).
2.3
Electrical measurements
Electrical resistivity (Fig. 1) was measured by using the ‘DC’ two-probe method. The two ends of the sample were coated on aluminium electrodes through thermal evaporation. A non-conducting glass slide was used for coating with the films. Both dark and light electrical conductivities were obtained for all the samples. S stands for the photosensitivity[9] and is obtained as:

class="figure_img" id="Figure1"/>
Download
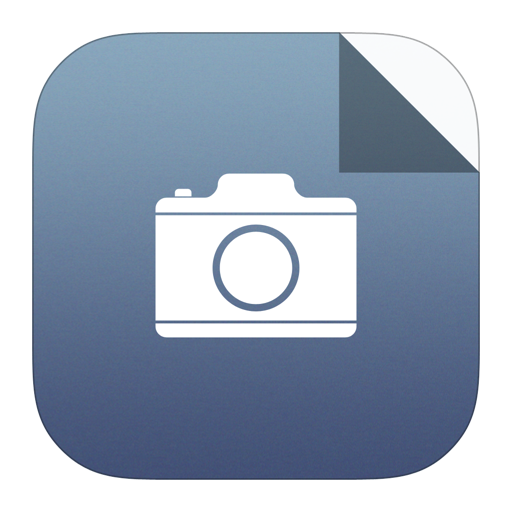
Larger image
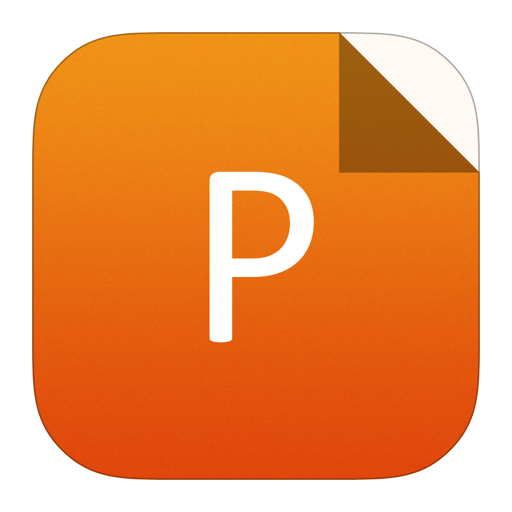
PowerPoint slide
Figure1.
Basic setup for direct-current two-probe method.
$S = frac{{{R_{ m{L}}} - {R_{ m{d}}}}}{{{R_{ m{d}}}}}, $ ![]() | (1) |
in which, Rd (RL) is the electrical resistance under dark conditions (under illumination). Before recording the RL, all the samples were placed under 8000 Lux illumination for 120 s by using a normal light source (150 W) at the distance of 35 cm. The light intensity and electrical resistance were estimated by using a TES-1339 light meter and UNI-T multi-meter, respectively.
3.
Results and discussion
3.1
Structural characterization
Fig. 2 depicts the XRD patterns of the SnS2:Cu thin films produced at different substrate temperatures (Ts = 400, 425, or 450 °C). As can be observed, the structure of the thin films is polycrystalline and the corresponding peak is related to the (001) plane of the SnS2 phase. The crystalline structure of the films was compared with the standard JCPDS data file No.83-1705 corresponding to the SnS2 phase. The other phases, such as SnS and its combination with Cu, were not identified in the pattern. Comparing the three curves in the XRD pattern indicates that the maximum value (the preferred peak) appears at 400 °C. The peaks intensity decreases as the substrate temperature increases from 400 to 450 °C. The broad hump observed in this figure is due to the amorphous glass substrate, which has been also observed in the results reported by previous studies[2, 38, 39].

class="figure_img" id="Figure2"/>
Download
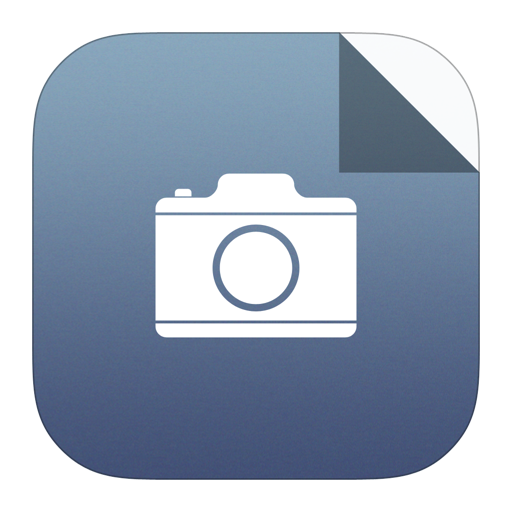
Larger image
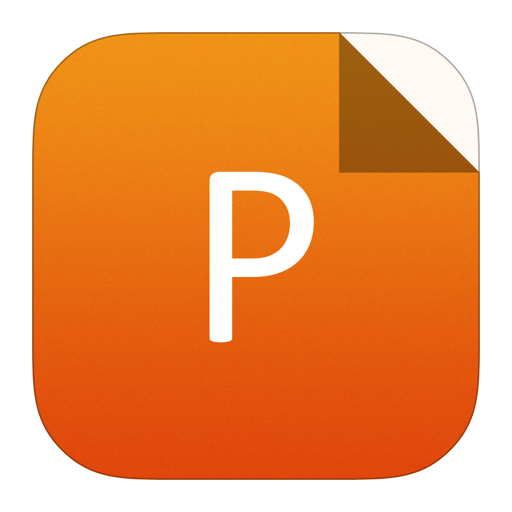
PowerPoint slide
Figure2.
XRD patterns of thin films with various substrate temperatures.
The lattice distance (d), average crystalline grain size (D), and strain (ε) of the (001) plane are given by the following equations[40]:
$d = frac{lambda }{{2sin theta }}, $ ![]() | (2) |
$D = frac{{klambda }}{{beta cos theta }}, $ ![]() | (3) |
$varepsilon = frac{{beta cos theta }}{4}, $ ![]() | (4) |
where λ defines the wavelength of the employed X-ray, θ is the Bragg’s angle, K is a constant (~1), and β stands for the full width at half maximum (FWHM) of the XRD peak. Table 2 lists the values of the aforementioned structural parameters. It can be seen that the distance between the crystal planes decreases with the increment in the substrate temperature. However, the trend is reversed for D and ε (see Fig. 3).
Ts (°C) | (hkl) | 2θ (°) | FWHM (°) | D (nm) | ε | d (observe) (?) | d (standard) (?) |
400 | 001 | 14.68 | 1.382 | 5.80 | 5.98 | 6.03 | 5.81 |
425 | 001 | 14.76 | 1.131 | 7.1 | 4.89 | 6.00 | 5.81 |
450 | 001 | 14.84 | 1.403 | 5.7 | 6.07 | 5.96 | 5.81 |
Table2.
Results of XRD patterns.
Table options
-->

Download as CSV
Ts (°C) | (hkl) | 2θ (°) | FWHM (°) | D (nm) | ε | d (observe) (?) | d (standard) (?) |
400 | 001 | 14.68 | 1.382 | 5.80 | 5.98 | 6.03 | 5.81 |
425 | 001 | 14.76 | 1.131 | 7.1 | 4.89 | 6.00 | 5.81 |
450 | 001 | 14.84 | 1.403 | 5.7 | 6.07 | 5.96 | 5.81 |

class="figure_img" id="Figure3"/>
Download
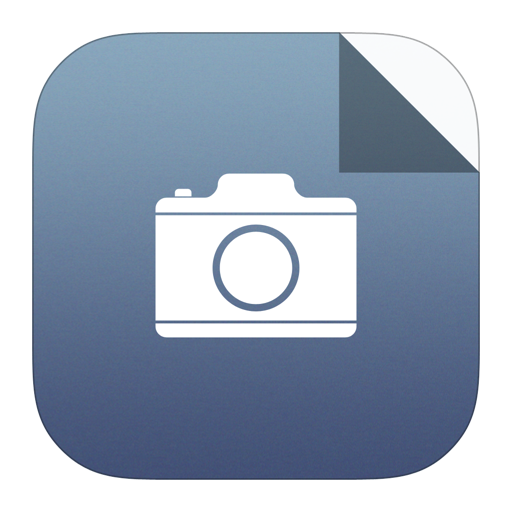
Larger image
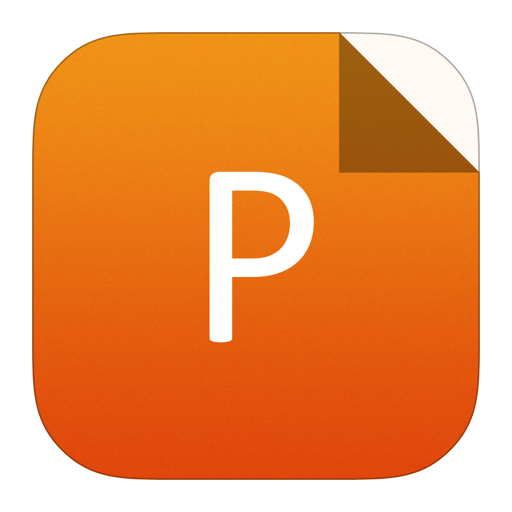
PowerPoint slide
Figure3.
(Color online) The variations of average crystalline grain size (D) and strain (ε) as a function of substrate temperatures.
3.2
Morphological studies
Fig. 4 shows the SEM images of SnS2 thin films with Cu impurities and with various substrate temperatures. The films exhibit a uniform surface and granular morphology. Further to this, the SEM images illustrate the film surface lacks cracks or pinholes. The average grain sizes at different substrate temperatures of 400, 425, and 450 °C are 53, 57, and 114 nm, respectively. The grain size increases as the substrate temperature increases. The grains are tightly bonded together and form a dense structure leading to the formation of larger grains.

class="figure_img" id="Figure4"/>
Download
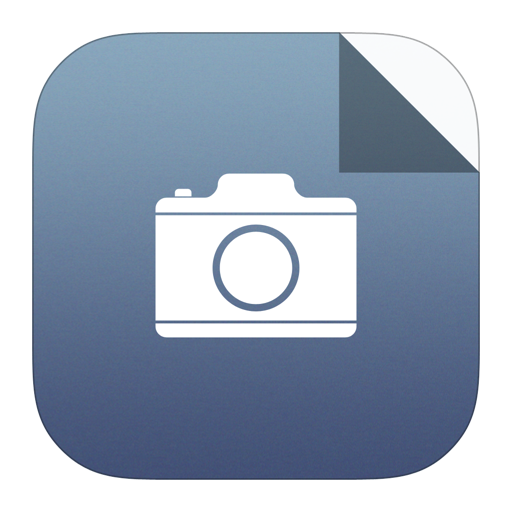
Larger image
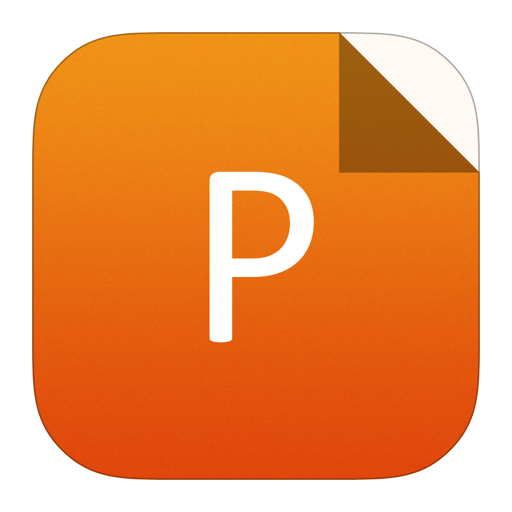
PowerPoint slide
Figure4.
(Color online) SEM images of thin films at (a) 400, (b) 425, and (c) 450°C.
3.3
Optical studies
The absorption coefficient has been calculated by using the absorbance data according to Lambert’s law[41]:
$alpha = frac{{2.303A}}{t}, $ ![]() | (5) |
where A is the optical absorbance and t is the film thickness. Fig. 5 plots the absorption coefficient variation against the photon energy (hv) corresponding to the films made at different substrate temperatures. The absorption coefficient increases as the photon energy and substrate temperature increases. The average transmittance of the layers in the range of visible light for the fabricated samples at different substrate temperatures are summarized in Table 3. Fig. 6 also depicts the corresponding graphical illustration. The transmittance values meet the maximum value for the samples prepared at Ts = 425 °C. The porosity of the samples can effectively reduce the transmittance.

class="figure_img" id="Figure5"/>
Download
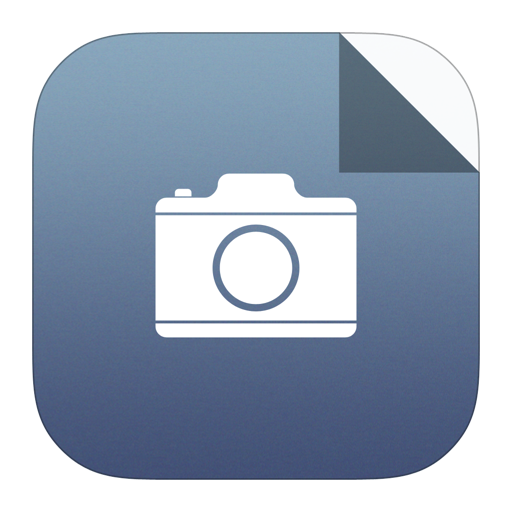
Larger image
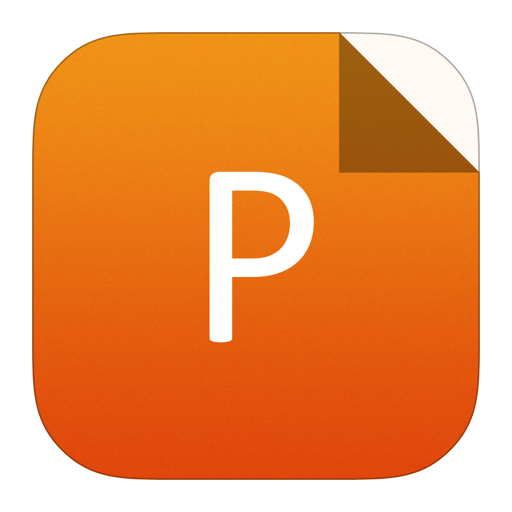
PowerPoint slide
Figure5.
(Color online) The variation of absorption coefficient versus photon energy (hv) for different substrate temperatures.

class="figure_img" id="Figure6"/>
Download
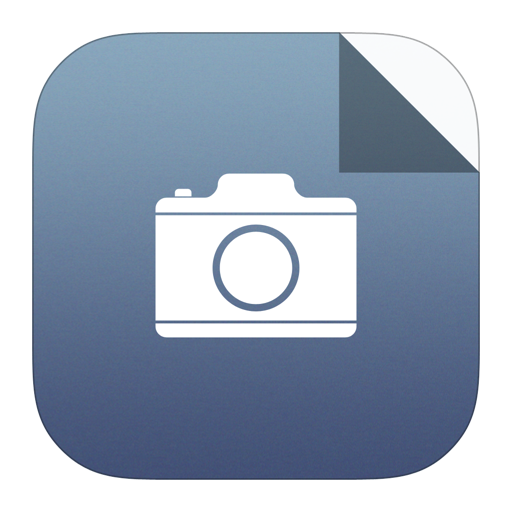
Larger image
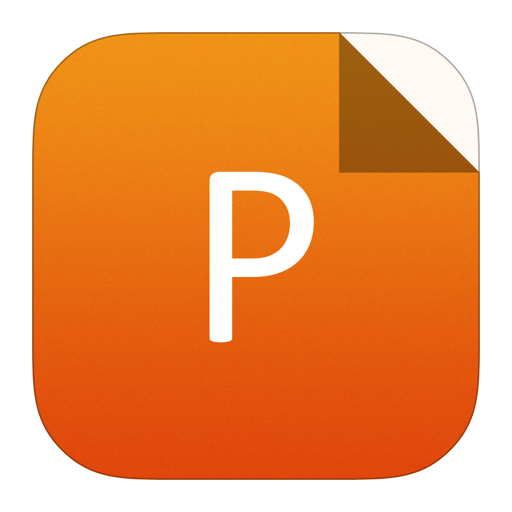
PowerPoint slide
Figure6.
(Color online) The variation of transmittance versus wavelength for different substrate temperatures.
In this study, the direct optical band gap of the samples fabricated at three different substrate temperatures is determined by using Tauc’s equation[5] through UV–vis measurements and by plotting (α


${left( {alpha hv } ight)^2} = Aleft( {hv - {E_{ m{g}}}} ight).$ ![]() | (6) |
Here α, β, and Eg are the absorption coefficient, constant value, and direct band gap, respectively. The latter is concluded from Fig. 7 for all three substrate temperatures. In general, an increment in the substrate temperature yields an increasing – decreasing trend in the band gap. According to the XRD patterns, the SnS2 phase is preferred over the other phases, such as SnO2, at low substrate temperatures. SnO2 is amorphous and cannot be detected in the patterns. Sulfur, due to its volatility, evaporates as the substrate temperature increases. Therefore, SnO2 accounts for the major portion of the product. Hence, the band gap is near that of SnS2 (about 2.4 eV) at low temperatures[36] and that of SnO2 (about 3.6 eV) at high temperatures[42].

class="figure_img" id="Figure7"/>
Download
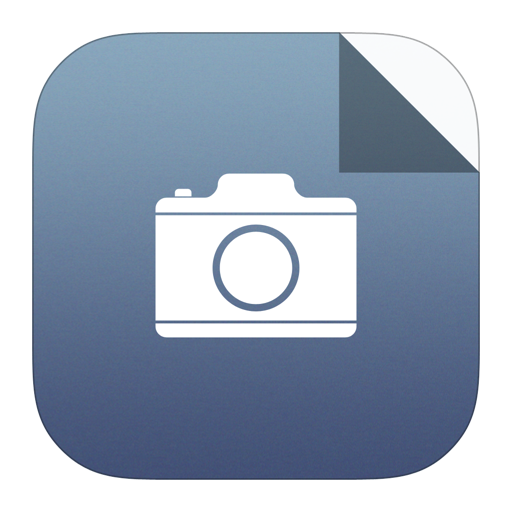
Larger image
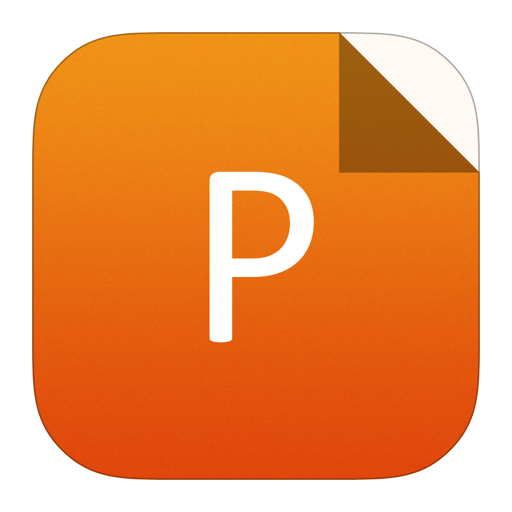
PowerPoint slide
Figure7.
(Color online) Plots of (αhv)2 versus hv for different substrate temperatures.
3.4
Electrical studies
The van der Pauw configuration has been applied for analyzing the Hall effect, which is capable of measuring the mobility, density, and type of the carriers. The influence of the substrate temperature has been investigated based on the electrical properties of the thin films. Table 3 gives the summary of the electrical measurement results. The obtained results reveal that the films exhibit n-type conductivity. Previous studies reported p-type treatment for SnS2 thin films[43, 44]. Similar to previous reports, the Hall effect has been used in the n-type procedure for the present thin films[35, 45–47]. On increasing the substrate temperature, the density of the carriers and Hall mobility experience an increasing trend, resulting in reduced special resistivity, increased grain size, reduced grain boundaries, and electron scattering. Fig. 8 proves this claim.
Substrate temperature (°C) | 400 | 425 | 450 |
Average transmittance in the range of visible light | 34 | 53 | 35 |
Thickness (nm) | 480 | 420 | 350 |
Eg (eV) | 2.81 | 3.23 | 3.06 |
ρ (Ω·cm) | 9.25 | 0.172 | 0.174 |
Conductivity type | n | n | n |
n (1016 cm?3) | 8.06 | 77.86 | 86.22 |
μ (cm?2/V.s) | 8.38 | 46.67 | 41.66 |
Table3.
Results of optical and electrical characterization.
Table options
-->

Download as CSV
Substrate temperature (°C) | 400 | 425 | 450 |
Average transmittance in the range of visible light | 34 | 53 | 35 |
Thickness (nm) | 480 | 420 | 350 |
Eg (eV) | 2.81 | 3.23 | 3.06 |
ρ (Ω·cm) | 9.25 | 0.172 | 0.174 |
Conductivity type | n | n | n |
n (1016 cm?3) | 8.06 | 77.86 | 86.22 |
μ (cm?2/V.s) | 8.38 | 46.67 | 41.66 |

class="figure_img" id="Figure8"/>
Download
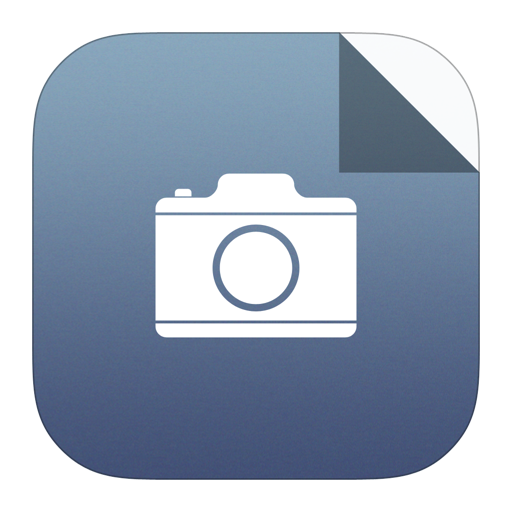
Larger image
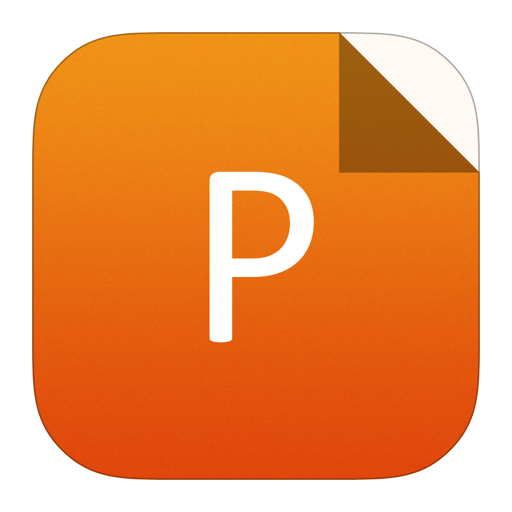
PowerPoint slide
Figure8.
(Color online) The variations of resistivity (ρ), carrier concentration (n) and electron mobility (μ) as a function of substrate temperature.
Samples were placed in radiating 8000 LUX light to investigate the effects of the light on the layers’ resistivity. The resistivity was recorded for specified periods of time. The relative resistivity is calculated by using Eq. (1). The variation of the resistivity with the light radiation duration is plotted in Fig. 9. The light responses with time show a varying trend among all the layers. The resistivity decreases with the increment in the radiation duration and reaches the saturation point after a while. The saturation conditions are affected by the equilibrium process of the light carrier production, which is time independent. As can be seen from Fig. 9, the layer sensitivity decreases as the substrate temperature increases. The decrease in the layer sensitivity is the result of several conditions, including the high substrate temperature and low sulfur level in the layers. Sulfur evaporates and escapes from the samples in the case of higher temperatures.

class="figure_img" id="Figure9"/>
Download
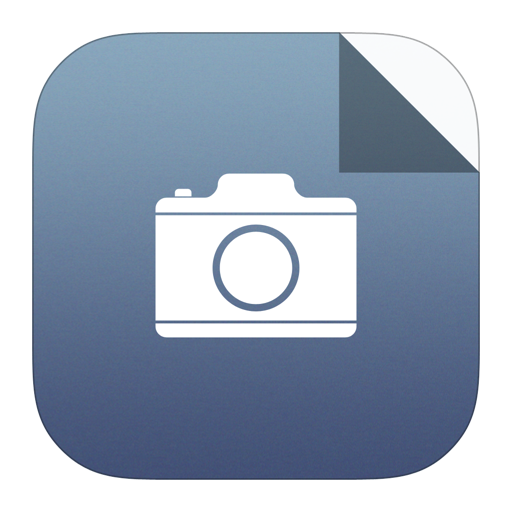
Larger image
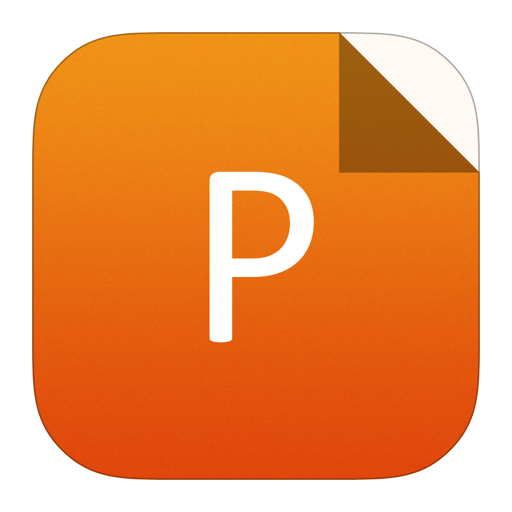
PowerPoint slide
Figure9.
(Color online) The variations of
m{L}}} - {R_{
m{d}}}}}{{{R_{
m{d}}}}}$
4.
Conclusions
The present study investigates the effects of the substrate temperature on the physical properties of the copper-doped tin disulphide films prepared by spray pyrolysis. The structural, morphological, optical, and electrical properties have been determined by XRD, SEM, UV–Vis spectroscopy, Hall effect, and photosensitivity analyses. The XRD patterns indicate that the samples are polycrystalline. Also, the SEM images indicate that the grain size increases with the increment of the substrate temperature and the grains are not spherical. Growing in size, the grains are tightly bonded and form a dense structure with increasing substrate temperature and this results in an increasing–decreasing trend in the band gap of the thin films.
In general, the resistance of the thin films decreases as the substrate temperature increases. The optical energy band gap of the films exhibits an increase – decrease trend. The mobility and density of the carriers increase with the increment in substrate temperature. The analyses of the Hall Effect clarify that the films exhibit n-type conductivity. It can thus be concluded that the physical properties of SnS2:Cu can be well modified by varying the substrate temperature.