1.
Application and design problems
77 GHz silicon–germanium (SiGe) power amplifiers have shown good performance in automotive radars, and the cheaper cost of SiGe technology compared to III–V compound?semiconductor and advanced CMOS technology makes mass production possible. In a 77 GHz automotive radar system, the PA may need to give more than 10 dBm output power to satisfy detecting function requirements[1]. If the antenna loss is taken into account, the required output power of the PA may be even higher. However, the collector-emitter breakdown voltage for the open base (BVceo) of the heterojunction bipolar transistor (HBT) limits the maximum output voltage[2, 3]. Simply increasing bias current is not a good method because bias current density is related to characteristic frequency (fT) and such a high frequency power amplifier needs sufficiently high fT to satisfy a W-band design. So, the bias current density is limited to a certain range. Large transistors also cannot solve the power problem effectively for too large transistors that have large parasitic capacitances and small output impedance, which is very difficult to match. Besides the power problem, the bias circuit should also be designed to be insensitive to temperature and process, and be controlled in a smooth way if the power amplifier needs to be controllable off the chip.
An on-chip bias circuit for the W-band SiGe HBT power amplifier is proposed in this work. This bias solution has the advantages of improving the output power of the power amplifier, temperature insensitivity, and smooth controllability.
2.
General analysis
Traditional bias circuits by using a two current mirror source and a beta helper current source lack the precise control over temperature and process variations. An insensitive and controllable bias circuit was proposed for the GaAs HBT power amplifier[4]. It is a suitable solution for the GaAs HBT power amplifier on mobile phones. The bias circuit in this paper is designed to make a new bias solution suitable for a W-band SiGe HBT power amplifier used for an automotive radar system or other communication systems.
Characteristic frequency is the most important factor which needs to be considered firstly in microwave amplifier design. The characteristic frequency of SiGe HBT is a function of current density. Taking IBM 8HP as an example of 0.13 μm SiGe BiCMOS technology, as shown in Fig. 1[5], the HBT fT first increases gradually to a typical peak value of about 200 GHz with the increasing current density and then drops sharply once passing the peak current density. If we set the DC current density of the power amplifier at the peak current density, a small increase of Vbe would sharply increase collector current (Ic), and then may result in a steep drop in cut-off frequency. As a result, we prefer to set the value of the current density to be slightly lower than the peak current density.

class="figure_img" id="Figure1"/>
Download
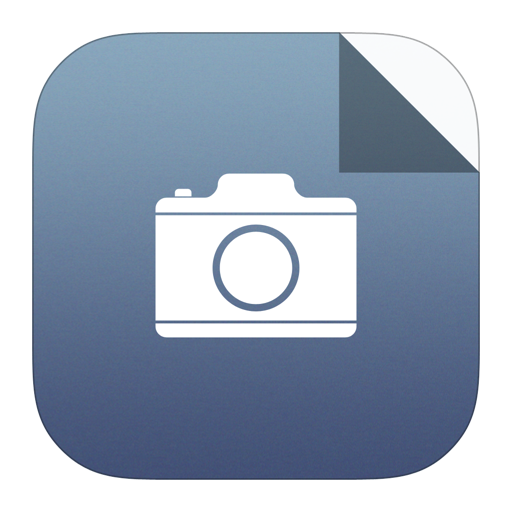
Larger image
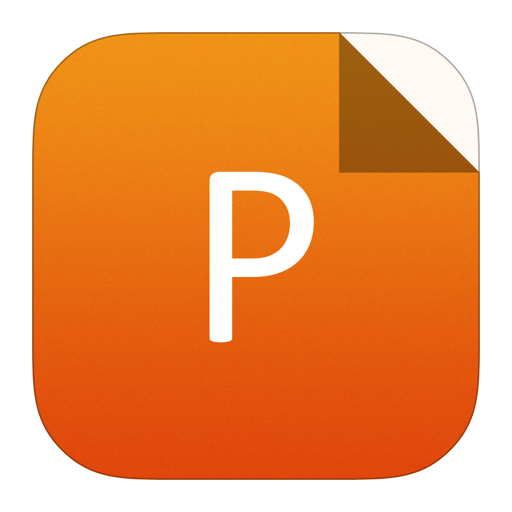
PowerPoint slide
Figure1.
Relationship between characteristic frequency and current density of silicon-germanium HBT in IBM 8HP technology.
The bias circuit schematic is shown in Fig. 2, where the part enclosed in the red dashed box represents our bias circuit and the part in the blue dashed box represents a sketch of a SiGe HBT transistor in a power amplifier with choke inductor, input capacitor, and output capacitor. The bias circuit contains two voltage sources, one is Vcon and the other is Vdd. Q1 represents a power amplifier transistor with its base and collector connected to the input and output terminals, respectively. Vcon is used as a controller to control the base voltage of transistor Q1. Another transistor Q2 plays a detecting role in detecting the current of Q1. Q4, Q5, and Q3 form a negative feedback loop, not only for controlling the current of Q1 but also for controlling smoothly when Vcon changes. On one hand, Q2, Rf1, Q4, Rf2, Q5, Rf3, and Q3 form a negative feedback loop when Ic of Q1 changes. On the other hand, when Vcon is applied artificially to control the current of Q1, Rf2 makes a negative feedback to Q5 to smoothly control the Q1 base voltage against steep variations in it, and suitable values of R3 and R4 could also contribute to smooth controlling. A smooth control is necessary because the current density of the power amplifier should be set to an appropriate fT range to ensure normal operation of the PA.

class="figure_img" id="Figure2"/>
Download
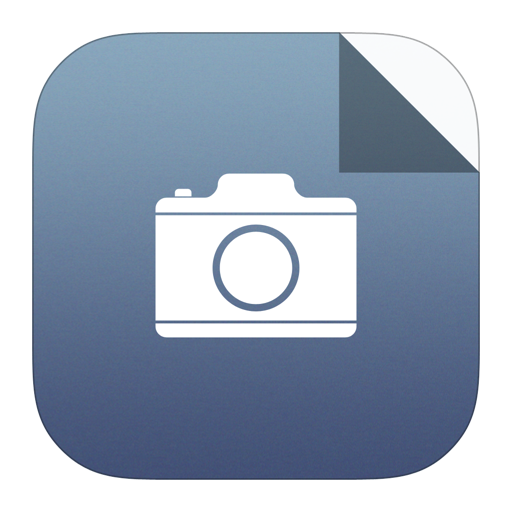
Larger image
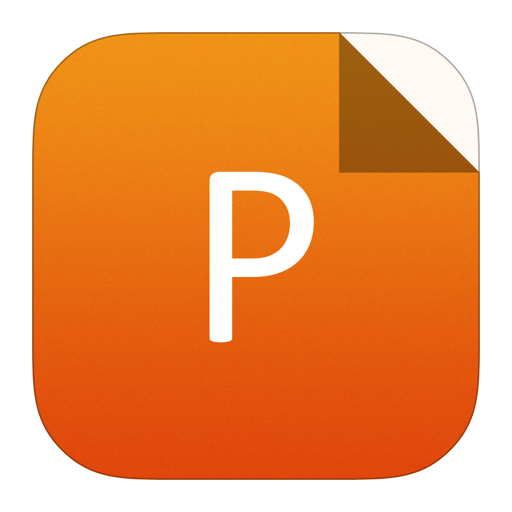
PowerPoint slide
Figure2.
(Color online) Bias circuit structure.
3.
HBT breakdown voltage consideration
With the evolution of the SiGe HBT technologies, the speed of the transistors is normally improved at the cost of its breakdown voltage to some extent. As the famous Johnson limit predicts, there is always a tradeoff between breakdown voltage BVceo and fT[6]. In IBM 8HP 0.13 μm SiGe BiCMOS technology, BVceo of a normal high-frequency SiGe HBT is about 1.8 V corresponding to the peak fT of 200 GHz, which means the supply voltage of a class A power amplifier using such HBTs is around only 0.9 V[7]. However, taking the 77 GHz automotive radar power amplifier as an example, if a 77 GHz automotive radar’s detectable distance is as long as 200 m, the power amplifier needs to generate about 10 dBm output power at least according to the famous radar equation[8]. If the supply voltage of our SiGe HBT power amplifier is 0.9 V, the last stage of the power amplifier has to be a large size one in order to meet the requirement of current density and the requirement of output power simultaneously. On the other hand, the driving stages also need to be biased to heavy current to drive the large output transistor to provide sufficiently high output power.
SiGe HBTs show a robust tolerance for base resistance in supporting a high value of collector-emitter breakdown voltage for a given base resistance (BVcer) because low values of intrinsic and extrinsic base resistance enable the devices to shunt large amounts of hole current[9]. Several W-band SiGe HBT power amplifier designs[1–3] have proved that a relatively low base resistance can make the HBTs work under high power supply voltage. As a result, the power amplifier can provide much more output power.
Fig. 3 shows how we get the output resistance of our bias circuit. We assume that the bias circuit is a voltage source with an output resistor r, enclosed in the red gridlines in Fig. 3. First, let the bias circuit drive a load r1 and get the voltage and current of r1 as U1 and I1, respectively. Then let the bias circuit drive another load r2, and get the voltage and current of r2 as U2 and I2, respectively. We get the value of r by the equations below.
${{I_1}} ; left( {{{r_1}} + {{r}}} ight){ m{ }} = { m{ }}{{U}},$ ![]() | (1) |
${{I_1}} ; {{r_1}}{ m{ }} = { m{ }}{{U_1}},$ ![]() | (2) |
${{I_2}} ; left( {{{r_2}} + {{r}}} ight){ m{ }} = { m{ }}{{U}},$ ![]() | (3) |
${{I_2}} ; {{r_2}}{ m{ }} = { m{ }}{{U_2}}.$ ![]() | (4) |
From Eqs. (1) and (2), we get
${{U_1}}{ m{ }} + { m{ }}{{I_1}} ; {{r}}{ m{ }} = { m{ }}{{U}}.$ ![]() | (5) |
From Eqs. (3) and (4), we get
${{U_2}} + {{I_2}} ; {{r}}{ m{ }} = { m{ }}{{U}}.$ ![]() | (6) |
Let Eqs. (6) – (5), finally we get the output resistance:
${{r}} = left( {{{U_2}} - {{U_1}}} ight)/left( {{{I_1}} - {{I_2}}} ight).$ ![]() |
We used the method in Fig. 3 and found that simulation results showed that output resistance approximately equaled to R1 in our bias circuit structure. Because as for our bias circuit, detector Q2 is much smaller than power amplifier HBT Q1 to save power and the resistor R2 has a large resistance value. When the circuit works, we can see a very low resistance from the emitter of Q3. Therefore, the resistance R1 is approximately equal to the output resistance of the bias circuit, and the output resistance of our bias circuit is designed around 200 Ω[3, 10].

class="figure_img" id="Figure3"/>
Download
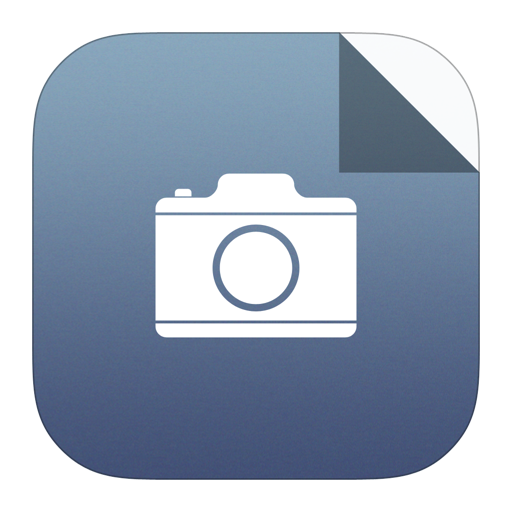
Larger image
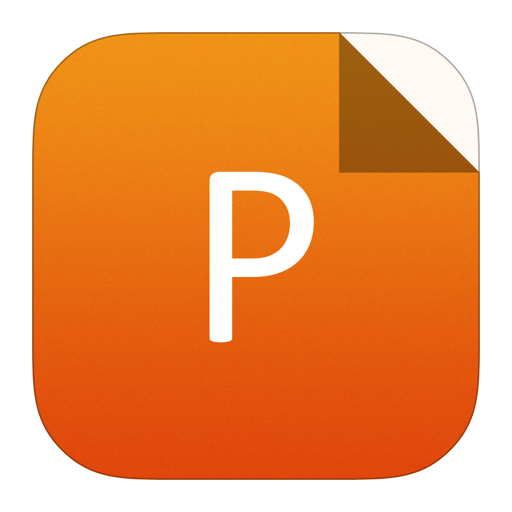
PowerPoint slide
Figure3.
(Color online) A schematic diagram that we used to calculate output resistance.
4.
Insensitivity and smooth control
As mentioned before, current density of SiGe HBTs determines the cut-off frequency. So, it is important to ensure that the current density of the power amplifier is stable. With the help of negative feedback, the circuit could improve the immunity to the fluctuation caused by temperature and process. When the current Ic of Q1 rises or falls, Ic of detector Q2 also rises or falls, then the negative feedback loop composed of Rf1, Q4, Rf2, Q5, Rf3, and Q3 works in turn to lower or raise the collector current of Q1, respectively. So, this negative feedback loop could help to stabilize the bias current of the power amplifier. Fig. 4 shows a simulation result of the relationship between the Ic of Q1 (3 × 18 μm × 0.12 μm SiGe HBT in IBM 8HP) and the temperature, where the solid line represents that case Q1 is biased with our bias circuit solution, and the dashed line corresponds to the condition where the base of Q1 is biased with an ideal voltage source connected with a resistor of 200 Ω.

class="figure_img" id="Figure4"/>
Download
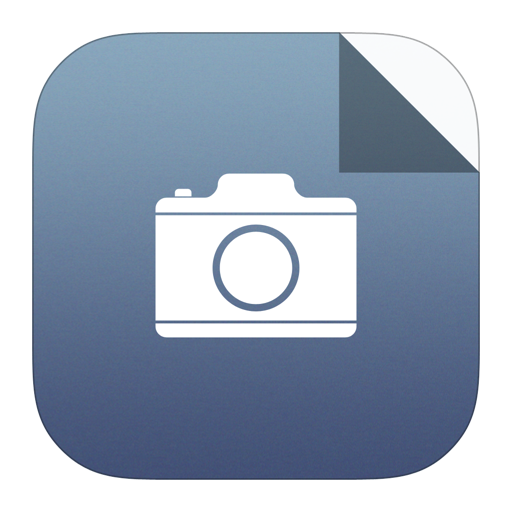
Larger image
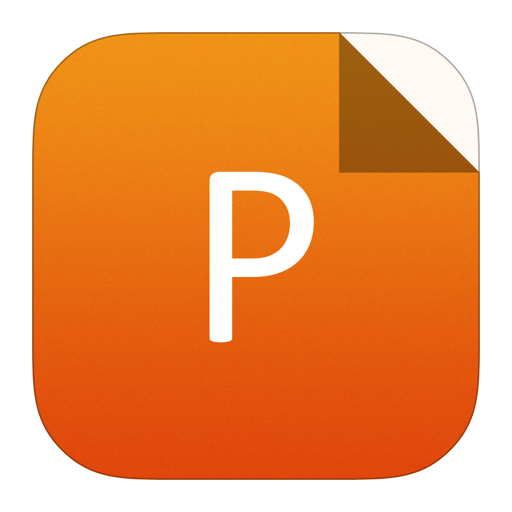
PowerPoint slide
Figure4.
Simulation result of comparison between this bias solution and an ideal voltage source with 200 Ω output resistor.
Sometimes we want the gain and current of the power amplifier to be controllable. In our bias solution, Ic of Q1 changes negatively with the controller Vcon. As discussed in the first part of this paper, the feedback mechanism of this circuit is effective in smooth controlling. On one hand, the negative feed loop composed of Q2, Q3, Q4, Q5, Rf1, Rf2, and Rf3 is good for smooth controlling. On the other hand, the addition of Rf2 as an emitter degeneration resistor also contributes to smooth controlling compared to direct controlling. In addition to these feedback factors, choosing suitable R3 and R4 could also help since the base voltage of Q5 is Vcon[R3/(R3 + R4)]. If the ratio of R3/(R3 + R4) is smaller, the change in the base of Q5 gets smaller, then the Ic of Q1 is not changed so fast with controller Vcon.
Fig. 5 shows the simulation result of Ic of Q1 as a function of temperature when R3 equals to 1400 Ω, R4 equals to 300 Ω, Vcon varies from 1.4 to 1.5 V, and Fig. 6 shows the simulation result of Ic of Q1 as a function of temperature when R3 equals to 1150 Ω, R4 equals to 500 Ω, and Vcon varies from 1.75 to 1.85 V. We can see that the power amplifier biased with the bias circuit can be controlled in a smooth way and different configurations like the values of R3 and R4 of the circuit can also change this smoothness.

class="figure_img" id="Figure5"/>
Download
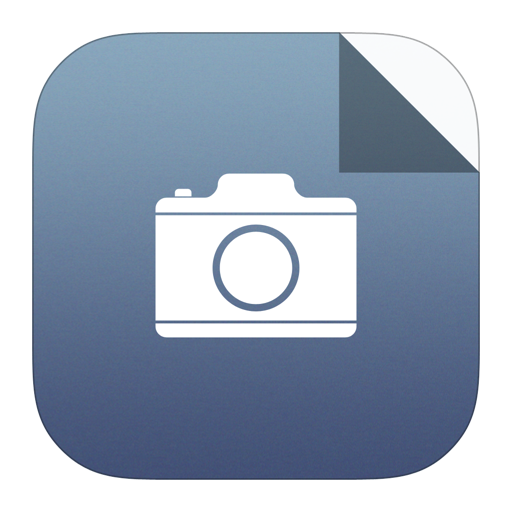
Larger image
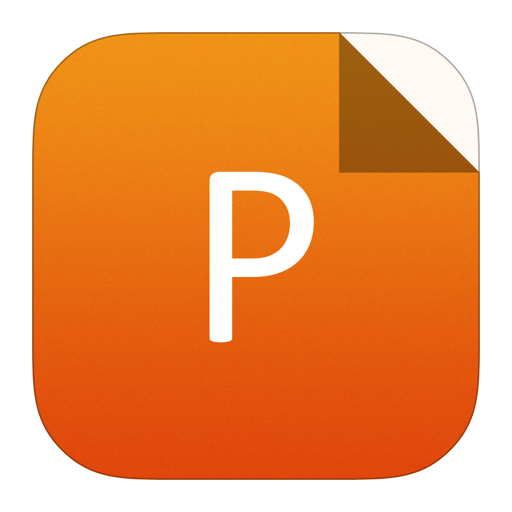
PowerPoint slide
Figure5.
Simulation results when R3/(R3 + R4) equals about 0.82.

class="figure_img" id="Figure6"/>
Download
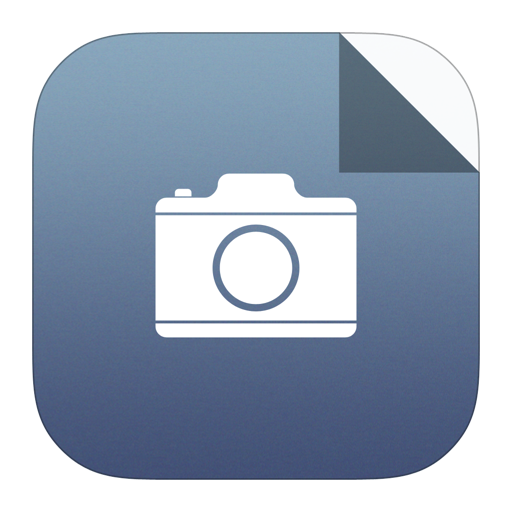
Larger image
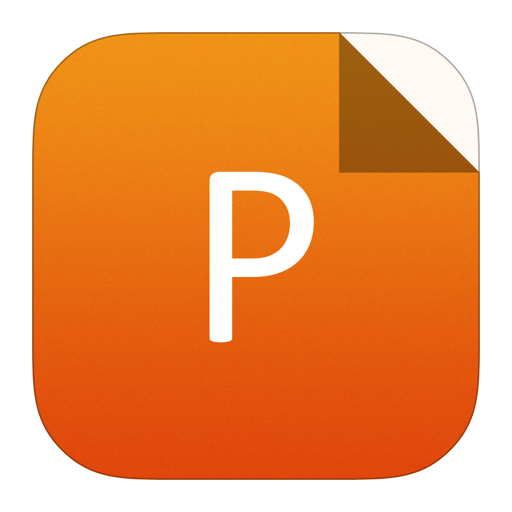
PowerPoint slide
Figure6.
Simulation results when R3/(R3 + R4) equals about 0.7.
5.
Analysis of bias circuit working in a 4-stage power amplifier
The gain that HBT transistors can provide decreases with the increase of working frequency. 77 GHz millimeter wave automotive radar power amplifiers usually require a multi-stage design to meet the gain requirement of more than 10 dB[1,2].
Fig. 7 shows a schematic of a 4-stage 77 GHz power amplifier biased by four ideal voltage sources with 200 Ω output resistors, and the simulated S-parameters versus temperature are shown in Fig. 8(a). We used our bias circuit to bias the same 4-stage power amplifier as shown in Fig. 7(b), and the simulated S-parameters versus temperature are shown in Fig. 8(b). Comparing the results of these two cases, both the insertion gain and input/output matching at low temperature and the gain flatness across the whole temperature range are found to be significantly improved by modifying the bias circuit.

class="figure_img" id="Figure7"/>
Download
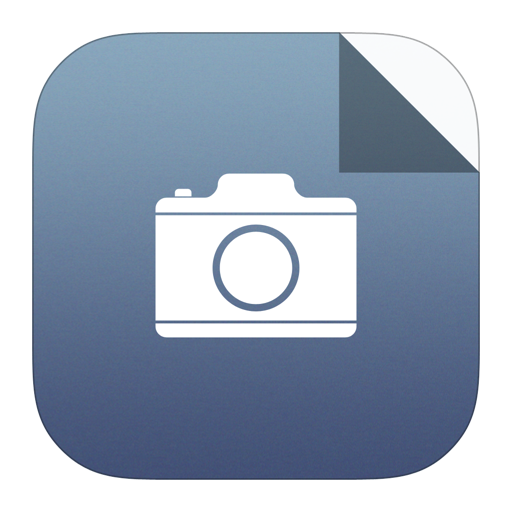
Larger image
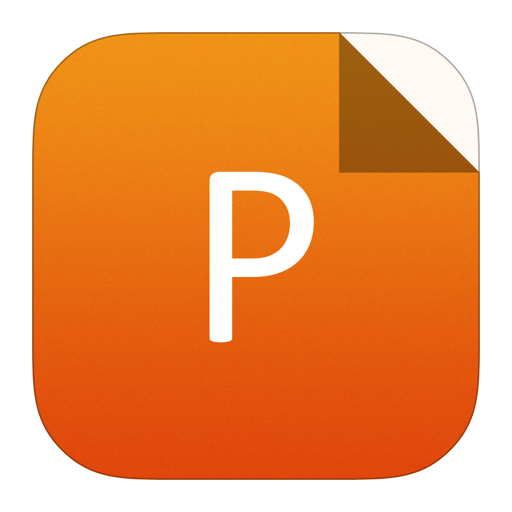
PowerPoint slide
Figure7.
Schematic of a 4-stage 77 GHz power amplifier biased by (a) ideal voltage sources with 200 Ω output resistors and (b) our bias circuits.

class="figure_img" id="Figure8"/>
Download
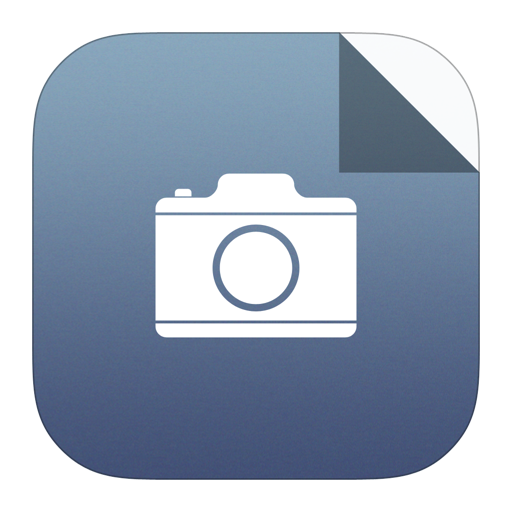
Larger image
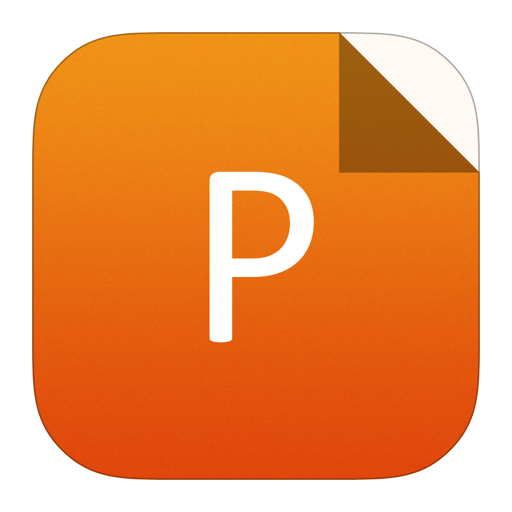
PowerPoint slide
Figure8.
The simulated S-parameters versus temperature for the 4-stage power amplifier biased by (a) ideal voltage sources with 200 Ω output resistors and (b) our bias circuits, respectively.
The output impedance of the bias circuit we designed is about 200 Ω at 77 GHz, and the input and output impedance of an HBT transistor in IBM 8HP technology is generally less than 10 Ω in the real part and a very small imaginary part at 77 GHz. In millimeter frequency operation around 77 GHz, seen from the base of the SiGe HBT, our bias circuit can be regarded as a fairly large impedance connected in parallel with much smaller impedance. Therefore, both input and output impedances of the power amplifier can be expected to be hardly influenced by the application of our bias circuit.
6.
Conclusion
This paper proposed a new on-chip bias solution for W-band SiGe HBT power amplifier. In order to adapt to W-band power amplifier applications, for example 77 GHz automotive radar, the circuit structure is designed with some key considerations including breakdown voltage and current density related to fT. Insensitivity and controllability are also considered in this bias solution. As verified by the simulation results, the addition of the negative feedback structure can give good performance in temperature insensitivity and smooth controllability.