1.
Introduction
Amorphous silicon dioxide (a-SiO2) attracts much attention due to its exceptional combination of properties and particular technology applications in optical materials such as windows, lenses, and optical fibers[1]. The amorphous form (a-SiO2), often known as fused quartz, vitreous silica or silica glass, is present in many electronic and optoelectronic devices, for example a gate dielectric in metal–oxide–semiconductor (MOS) transistors[2]. Moreover, amorphous silica has been one of the key materials in optic industries because of high optical transparency and resistance to laser damage. However, defects and impurities constitute an intrinsic part of the materials in applications. Indeed, atomic species that are introduced in the optical materials during the manufacturing processes degrade performance of the devices. Furthermore, optical absorption (OA) of point defects is often the main factor for high-purity silica, limiting the practically achievable UV transmittance[3]. Otherwise, silica glass is highly susceptible to react under irradiation and plays a significant role as precursors in the laser-induced defect formation process[4]. What is more, hydrogen defects are one of the most harmful defects in silica glass.
As is well-known, hydrogen can be trapped in the bulk materials in four forms: interstitial molecular H2, interstitial atom H, O–H+(2Si=O–H)+, and Si–H?(
m{4O}}bar equiv {
m{Si?H}}$

Since the publication of glass model by Zachariasen[10] and the early X-ray studies of Warren and coworkers[11], the structure of amorphous silicon dioxide (a-SiO2) is known to form a network of randomly interconnected tetrahedra. These tetrahedra are composed of one silicon atom at the center and four oxygen atoms at the corners where each oxygen atom is shared by two tetrahedral units such that the total number of O atoms is twice that of Si atoms. Therefore, structural models can be constructed on the basis of these findings[10, 11]. To further understand hydrogen defect impurity-induced damage under ultrashort intense laser, it is necessary to study the optical properties of amorphous silica containing hydrogen defects within the atomic level density functional theory.
In this work, after testing, the 216 atoms (72 units) model is used to investigate electronic and optical properties of amorphous silica whose neutral and charged states of hydrogen are illuminated by means of the density functional theory (DFT)[12]. At first, these SiO2 models of defect-free and hydrogen defects were presented. After that, extensive analyses of structural properties were performed in order to assess the qualities of models. Finally the proper models were used to analyze electronic and optical properties of defect-free and hydrogen defects a-SiO2.
2.
Computational methods
The defect-free amorphous silica cluster structure was manually constructed, which is known to form a randomly interconnected tetrahedral network. These tetrahedra are composed of one silicon atom at the center and four oxygen atoms at the corners where each oxygen atom is shared by two tetrahedral units such that the total number of O atoms is twice that of Si atoms. Then, 216 atoms (72 units) model was constructed and then put into a cubic box (30.00 × 30.00 × 30.00 ?3). The structure relaxations of defect-free a-SiO2 model and hydrogen defect a-SiO2 model were carried out. After geometry optimization, the parameter of the cubic box was changed to 24.99 × 25.32 × 25.42 ?3. Subsequently, the electronic and optical properties of defect-free and hydrogen-defect a-SiO2 were performed with density functional theory (DFT)[12] and Perdew–Burke–Enzerhof (PBE)[13] exchange and correlation interaction functional under the generalized gradient approximation (GGA)[14, 15]. The valence electrons were labeled as Si 3s23p2, O 2s22p4. The cut-off energy of 500 eV, a relatively high accuracy for ground state electronic convergence (10?5 eV) and maximum atom force (0.05 eV/?) were used. The K-point was only gamma for Brillouin zone integration under Monkorst–Pack method[16]. All the calculations of electronic and optical properties were obtained at the gamma point in the vacuum conditions.
3.
Results and discussion
3.1
Structural properties
According to the theoretical[4, 17, 18] and experimental[19] findings, the bond lengths of Si–O (1.61 ?) Si–H (1.54 ?), O–H (1.11 ?) and bond angles of O–Si–O (109.47°), Si–O–Si (145°–150°) are employed in the models. The outermost shell electron distribution Si 3s2p2 forms sp3 hybridized orbits and Si–O covalent bonds[20]. As SiO2 is a tetrahedral network structure, silicon locates in the center, while four oxygen ions array around a central silicon. The ratio of Si atom and O atom keeps at 1 : 2. Then the model of SiO2 network was shown in Fig. 1(a). Subsequently, the positive (H+) (0.46% atom percent) and negative (H?) (0.46% atom percent) charged states were emerged by adding a hydrogen atom bound to silica forming five coordinates Si(V) and a hydrogen atom bound to oxygen forming three coordinates O(III), respectively. Moreover, a single neutral hydrogen atom or hydrogen molecule was randomly located in the void of the network under the constraint which was not further than 2.0 ? away from its nearest neighbor.

class="figure_img" id="Figure1"/>
Download
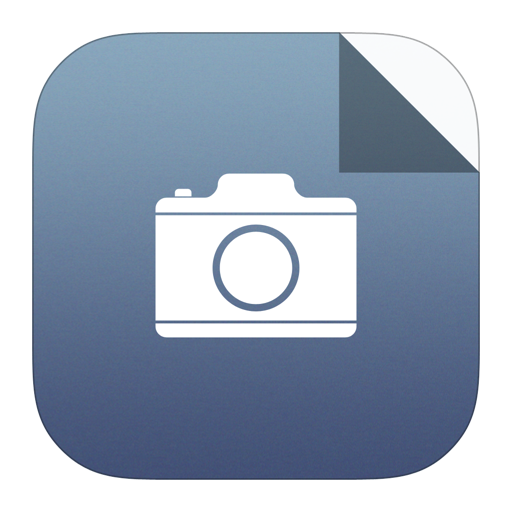
Larger image
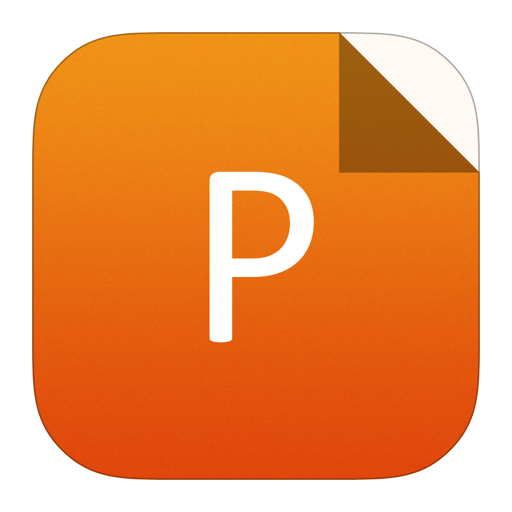
PowerPoint slide
Figure1.
(Color online) The structure of defect-free and charged states of a-SiO2: white, red and yellow balls represent hydrogen, oxygen and silica atoms, respectively.
After geometry optimization, the distribution of bond length of Si–O and bond angles of Si–O–Si, O–Si–O for perfect amorphous silica were given in Figs. 2(a)–2(c). The distribution curves of Si–O bond length roughly presents Gaussian and the average distance between Si and O is 1.62 ? which is consistent with the experimental value of 1.62 ? shown in Table 1[19]. The bonds of Si–O range from 1.496 to 1.648 ?. The altering distance between silica ion and oxygen ion is reasonable compared with the experimental value of 1.62 ? and theoretical values of 1.63 ?[17–19]. The angles of Si–O–Si change from 128.0° to 177.5°, simultaneously angles of O–Si–O range from 105.0° to 118.5°. However, the averages of Si–O–Si and O–Si–O bond angles are 148.5° and 109.8°, respectively, which are in good agreement with the experimental and theoretical values seen in Table 1[17–19]. It is indicated that the calculated amorphous silica model is reasonable and can be used to further calculate electronic and optical properties of a-SiO2 with DFT.
Parameter | This work | Theory | Experiment |
Si–O (?) | 1.62 | 1.63a | 1.62b |
O–Si–O (°) | 109.8 | 109.4a | 109.5b |
Si–O–Si (°) | 148.5 | 148.8a | 147.9–151b |
a Refs. [17, 18], b Ref. [19]. |
Table1.
The average bond lengths and angles of defect-free amorphous silica.
Table options
-->

Download as CSV
Parameter | This work | Theory | Experiment |
Si–O (?) | 1.62 | 1.63a | 1.62b |
O–Si–O (°) | 109.8 | 109.4a | 109.5b |
Si–O–Si (°) | 148.5 | 148.8a | 147.9–151b |
a Refs. [17, 18], b Ref. [19]. |
Subsequently, hydrogen defects have been investigated in the neutral, positive and negative charge states. For the neutral state, the hydrogen atom H and hydrogen molecular H2 are randomly localized in the void of network. As was shown in Fig. 2(b) for the negative charged state, the H atom is bound to Si forming a Si–H bond to give a fivefold coordinated Si(V). After geometry optimization, around fivefold coordinated Si(V), the distances between Si and O are 1.67, 1.68, 1.69, 1.68 ?, corresponding to SOA standing for ≡SiO–[20–22], meanwhile Si–H bond length is 1.45 ? smaller than experimental values of 1.54 ?[19]. Therefore, an electrostatic force attraction can be formed between the hydrogen atomic nucleus and electron cloud of neighboring elect ronegative atoms[20]. Fig. 2(c) indicated the positive charged state, where hydrogen atom gets bound to an oxygen atom to form a threefold coordinated O(III) atom. After optimization, around threefold coordinated O(III), the distances between Si and O are 1.61, 1.62 ?, corresponding to SOR standing for ≡SiO+[20–22], meanwhile O–H bond length is 1.16 ? larger than experimental values of 1.11 ?[19]. Therefore, a weak electrostatic force attraction may be formed between the hydrogen atomic nucleus and electron cloud of neighboring electronegative atoms[20]. During the calculation, all the dangling bonds were saturated by hydrogen in order to eliminate the effects of dangling bonds as defects, which can significantly influence both the electronic and optical properties.

class="figure_img" id="Figure2"/>
Download
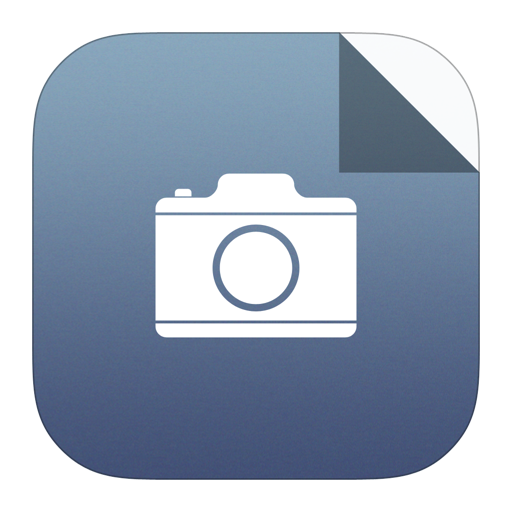
Larger image
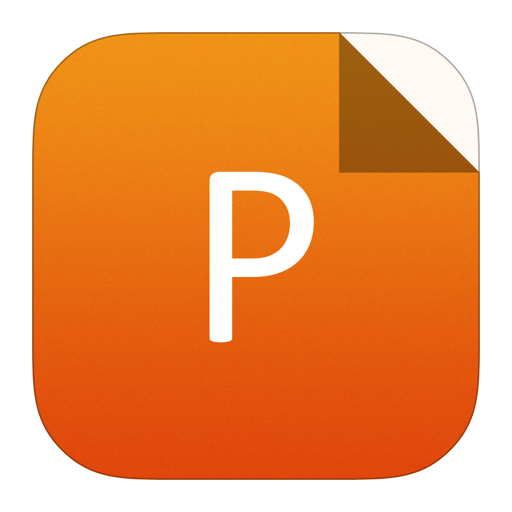
PowerPoint slide
Figure2.
(Color online) The distribution of the bond lengths and bond angles for relaxed defect-free amorphous silica structure and the black line is fitted by Gaussian functions.
3.2
Electronic properties
The electronic densities of states (DOS) were computed for each of five samples as shown in Figs. 3(a) and 3(b). The DOS of defect-free a-SiO2 exhibited similar behavior to that of pure amorphous silica[4]. The lowest lying group was identified as O 2s because a strong oxygen s-character was generated together with a very small amount of Si s- and p-states. The states between 0 and –10.0 eV were attributed to bonding O 2p-Si sp3 states which were mainly composed of O p- and some Si s- and p-orbitals, namely sp3 hybrid states. The remaining group from 3.5 and 6.0 eV was mostly contributed to by oxygen p-states and corresponds to O 2p non-bonding orbitals. As point defects or impurities did, the hydrogen defect introduced new defect energy levels in the electronic structure of a-SiO2.
For the neutral charged state in Fig. 3(a), the distributions of DOS for hydrogen atom and hydrogen molecule defect entirely moved towards the lower energy for the reason that the hydrogen atom introduced one occupied level in the gap. The shapes of DOS curves of hydrogen atom and hydrogen molecule defects of a-SiO2 were similar to that of the defect-free a-SiO2.
Fig. 3(b) depicted the DOS of positive charged states. From this, the DOS of the positive charge was almost overlapped with that of the defect-free. Note that a level was lowered in the region at –18, –6 and –2 eV due to the bond formed between H atom and O atom. There was no occupied state in the gap but an unoccupied level appeared between the valence band maximum and the conduction band minimum. Obviously, a new defect level appeared at 1.4 eV for which the hybrid of the H 1s and O 2p forms an unoccupied level. The new generated defect level can easily absorb photons to accumulate enough energy to initiate optical transition and finally induce damage.

class="figure_img" id="Figure3"/>
Download
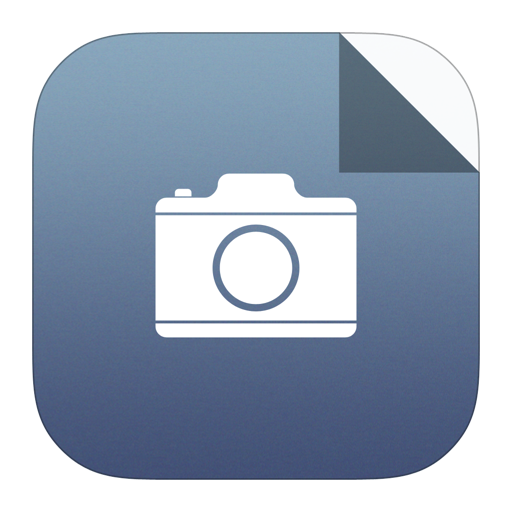
Larger image
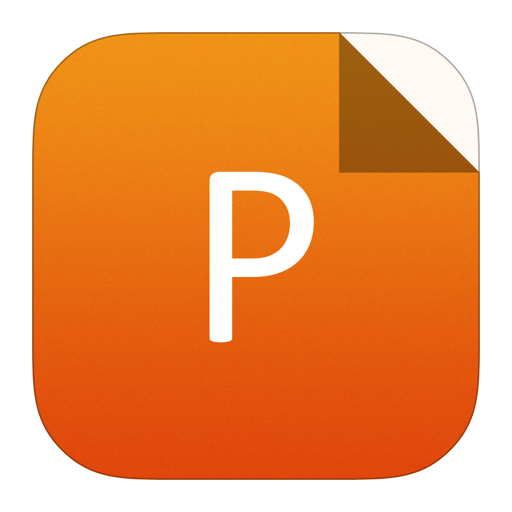
PowerPoint slide
Figure3.
(Color online) The DOS of defect-free and hydrogen defect of a-SiO2.
Meanwhile in the negative charged state, an unoccupied state was introduced at fermi level. The distribution of DOS for Si–H–(
m{4O}}bar equiv {
m{Si - H}}$

3.3
Optical properties
The optical properties of a-SiO2 can be well described by the complex dielectric function ε(ω) = ε1(ω) + iε2(ω) linked with the electronic structure. ε1(ω) which is associated with the dispersion is the real part. ε2(ω) is the imaginary part which is proportional to the absorption spectrum[23, 24]:
$${varepsilon _2}(omega ) = left( {frac{{4{pi ^2}{e^2}}}{{{m^2}{omega ^2}}}} ight){sumlimits_{i, j} {intlimits_k {leftlangle {ileft| M ight|j} ight angle } } ^2}{f_i}(1 - {f_i})delta ({E_{j, k}} - {E_{i, k}} - omega ){{ m d}^3}k.$$ ![]() | (1) |
The real part ε1(ω) was obtained from the imaginary ε2(ω) by the Kramers-Kronig relationship[23, 24]:
$${varepsilon _1} = 1 + frac{2}{pi }pint_0^infty {frac{{omega '{varepsilon _2}(omega ')}}{{{{omega '}^2} - {omega ^2}}}} { m d}omega '.$$ ![]() | (2) |
In which p denoted the principal value.
According to the calculated ε(ω), absorption coefficient I(ω) can be obtained which represented how far the light of a particular wavelength can penetrate into a material. When exposed to a beam of known and narrow range of kinetic energies of electrons, loss function L(ω) showed that some electrons lost energy and deflected. Optical conductivity σ(ω) which includes the absorptive σ1(ω) and reactive σ2(ω) describes current response to a time-varying external electrical field of frequency of two compounds[23, 24]:
$$I(omega ) = sqrt 2 omega {left[{sqrt {{varepsilon _1}{{(omega )}^2} + {varepsilon _2}{{(omega )}^2}}-{varepsilon _1}(omega )} ight]^{1/2}}, $$ ![]() | (3) |
$$L(omega ) = frac{{{varepsilon _2}(omega )}}{{{varepsilon _1}{{(omega )}^2} + {varepsilon _2}{{(omega )}^2}}}, $$ ![]() | (4) |
$$sigma (omega ) = sigma (omega ) + isigma (omega ) = - ifrac{omega }{{4pi }}left[{varepsilon (omega )-1} ight].$$ ![]() | (5) |
The absorption coefficients for defect-free and hydrogen defect a-SiO2 were described in Fig. 4. Seen from Fig. 4, the defect-free a-SiO2 occupied two absorbed peaks at 7.5 eV according well with Tamura's result at 7.4[25] and 10.4 eV in good agreement with the absorption peak at 10.4 eV from 10 to 300 K reported by Vella et al.[26]. Two absorbed peaks for hydrogen atom and hydrogen molecule defects of a-SiO2 were also observed at 7.5 and 10.4 eV. Only the difference was the absorbed strength between neutral states and the defect-free. Easily seen, the interstitial hydrogen atom defect of amorphous silica embraced the highest absorption peaks among five samples. Furthermore, no extra absorption peaks were introduced for the interstitial hydrogen and hydrogen molecule defects. If exposed under laser irradiation, these defects would be bonded to oxygen atom to form O–H bond which can enhance light absorbance and finally induce irreversible modification. In the negative state, one more defect absorption peak occurs at 0.9 eV in the visible region besides two absorption bands arose around 7.5 and 10.4 eV in the deep ultra-violet (DUV) region.

class="figure_img" id="Figure4"/>
Download
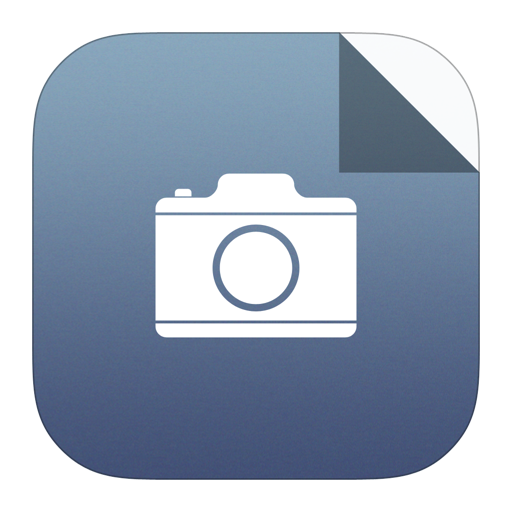
Larger image
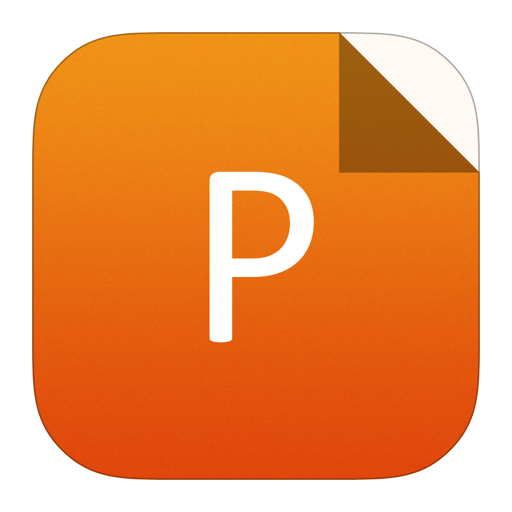
PowerPoint slide
Figure4.
(Color online) The schemes of absorption coefficients for defect-free and hydrogen defect of a-SiO2.
Moreover, the negative hydrogen defect began to become absorptive from 0 to 2.0 eV in the visible light region and then became transparent from 2.0 to 4.5 eV in Vis–UV. As the incident light energy was larger than 4.5 eV and less than 12.0 eV, the negative charged hydrogen defect of amorphous silica absorbed photons and introduced two strong absorption peaks.
As for the positive states, the entire absorption spectrum caught red-shift and two strong absorption peaks arose at 6.9 and 9.0 eV meanwhile two extra absorption peaks occurred at 12.2 and 15.2 eV because the H 1s orbital made deviation towards O 2p. Fortunately, the absorption peaks at 9.0, 12.2 and 15.2 eV accorded with that of the silanol group Si–OH at > 7.3 eV [27], which is the dominant network-bound form of hydrogen.
Fig. 5 showed the conductivity of defect-free and hydrogen defect amorphous silica, (a) real parts and (b) imaginary parts. The conductivity was composed of the absorptive σ1(ω) and reactive σ2(ω) indicating current response to a time-alternating external electrical field of frequency. The real part represented the in-phase current, which produced the resistive joule heating, while the imaginary part represented the π/2 out-of-phase inductive current. All of them caught the same fluctuations except for the negative charged states at 4.0 eV and positive charged states at 13.5 and 17.3 eV, respectively. Additionally, four peaks arose at 7.2, 8.2, 10.4, and 10.8 eV except that the negative charged states caught three peaks at 7.2, 8.2, and 10.4 eV. Furthermore, the imaginary parts of defect-free and hydrogen defect amorphous silica are less than zero from 0 to 8.2 eV and 9.0 to 10.0 eV. The minimum values of imaginary conductivity presented at 6.0 eV and the hydrogen atom defect of a-SiO2 achieved the smallest value. In the high-frequency region, however, which corresponded to the visible and ultraviolet regimes, Re σ
m {Im}}$


class="figure_img" id="Figure5"/>
Download
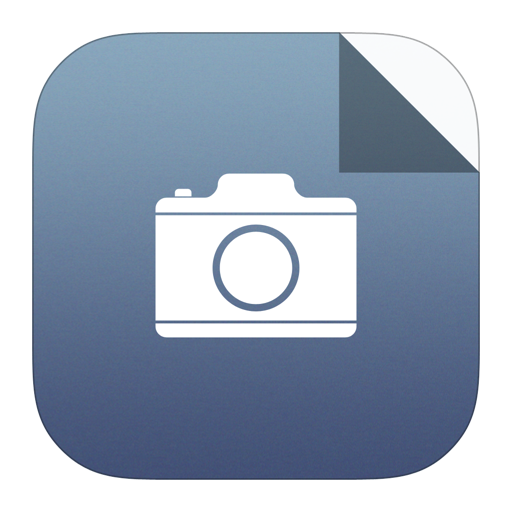
Larger image
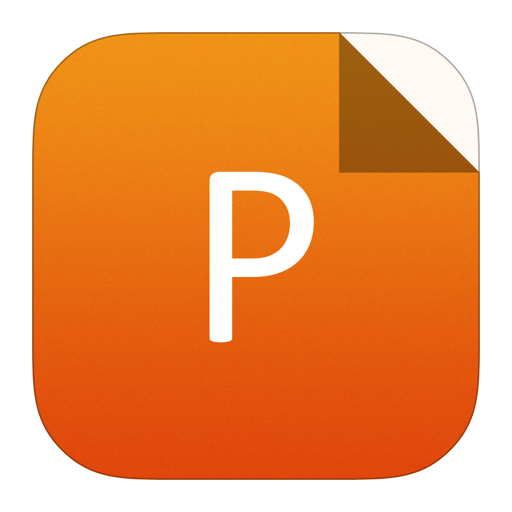
PowerPoint slide
Figure5.
(Color online) The conductivity of defect-free and hydrogen defects of amorphous silica. (a) Real parts. (b) Imaginary parts.
The energy loss spectra of defect-free and defected structures were shown in Fig. 6. The energy loss spectrum L(ω) as an important factor described the energy loss of a fast photon traversing in materials. The prominent peak of the L(ω) spectrum represented the characteristics associated with the plasma oscillations. The main and strong loss peaks were at around 7.5 and 10.4 eV for the defect-free of a-SiO2. All the loss spectra were almost overlapped except for the charged states. There existed a platform ranging from 3.9 to 5.0 eV for negative charged state of a-SiO2 while the interstitial atom H defect of a-SiO2 embraced the largest value of energy loss among all the structures at 7.0, 8.0, 10.0, and 10.8 eV. Only the positive charged state of a-SiO2 leaded to one energy loss peak but the other had two energy loss peaks from 10.0 to 11.0 eV. Moreover, the intensity of energy loss spectrum for the positive defected a-SiO2 had larger values from 11.5 to 18.0 eV especially at 13.7 and 17.3 eV compared with other structures due to the new bond formation.

class="figure_img" id="Figure6"/>
Download
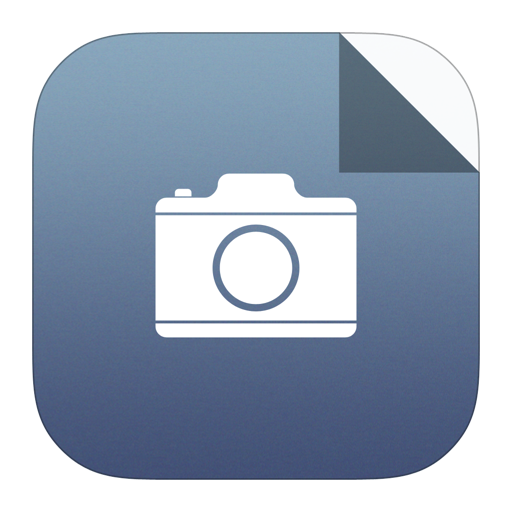
Larger image
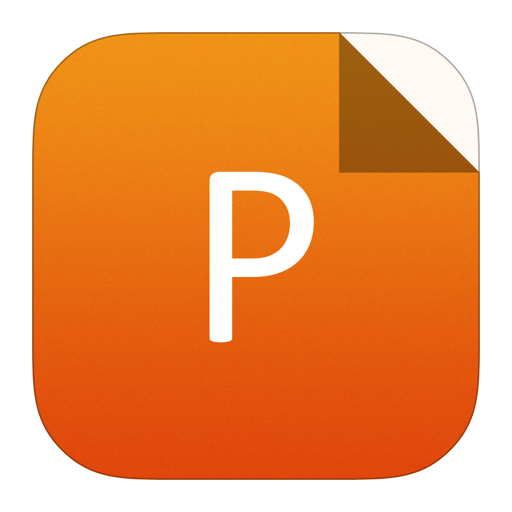
PowerPoint slide
Figure6.
(Color online) The loss function of defect-free and hydrogen defects of amorphous silica.
4.
Conclusion
The electronic and optical properties of defect-free and hydrogen defect a-SiO2 were investigated by DFT in the frame of GGA/PBE method. It is found that hydrogen defects influenced the DOS distribution. Firstly, the negative charged state defect introduced new defect level between the valence band top and conduction band bottom. However, the neutral and positive charged state defects made both the valence band and conduction band transfer to the lower energy. Additionally, the interstitial hydrogen atom defect a-SiO2 caught the strongest intensity of absorption at 7.4 and 10.5 eV. As for the positive states, the whole absorption spectrum caught red-shift and two strong absorption peaks arose at 6.9 and 9.0 eV meanwhile two extra absorption peaks appeared at 12.4 and 15.2 eV because the H 1s orbital made deviation towards O 2p. Otherwise, the negative hydrogen defect a-SiO2 lead to absorption peak from 0 to 2.0 eV. It was concluded that the neutral hydrogen defect a-SiO2 improved absorption intensity, and the negative hydrogen defect a-SiO2 became absorptive in visible light region, as well as the positive hydrogen defect produced absorption at lower energy. The conductivity and loss function were also discussed. This may give insights into understanding the mechanisms of laser-induced damage for optical materials such as amorphous silica.