1.
Introduction
Semiconductor laser sources for blue-green and ultraviolet (UV) spectral ranges are very useful in many fields of science and technology due to the wide range of available wavelengths, compactness, efficiency, reliability, low cost and ease of use.
Alloys AlxGa1–xN with a band gap in the range of 3.4–6.2 eV have emerged as prospective semiconductor materials with applications to laser sources for a blue-green spectral range. Nowadays, the shortest wavelength of UV laser diodes based on GaN is 336 nm[1]. For the generation of shorter wave radiation, optical pumping is typically used[2–5]. The most shortwave stimulated emission with a wavelength λ = 214 nm has been achieved under the optical excitation of an AlN layer on a c-Al2O3 substrate with a threshold power density of 9 MW/cm2[2]. The excitation of simulated emission in the range of 235–250 nm in Al0.7Ga0.3N/AlN heterostructures with multiple quantum wells under femtosecond optical pumping has been reported[3].
In the paper[6] the luminescence characteristics of heavily silicon-doped AlxGa1–xN films with a different Al content (x), which were grown by molecular beam epitaxy on sapphire substrates, were studied under the excitation by a low-energy (< 20 keV) electron beam. The luminescence spectra of the AlxGa1–xN films with x > 0.42 exhibited a broadband emission covering the whole visible and near-infrared spectral range from 1.72 to 2.7 eV (460–720 nm), while the band-edge luminescence is negligibly weak. Super-radiance within the broad band was obtained by pumping the samples by an electron beam with a power higher than 200 kW. A similar broadband emission was previously observed in the cathodoluminescence spectra of Al 0.37Ga0.63N films at 77 K[7] and Al0.98Ga0.02N films at 12 K[8], with intensity being much weaker than that of the band-edge luminescence. It is deemed that under the condition of the paper[6] the luminescence is related to optical transitions via levels of the complex comprising an impurity atom and a lattice point defect[9, 10]. However, the luminescent properties and stimulated emission via defect levels in wide band gap AlGaN alloys have been scarcely studied. Nonetheless, some tentative conclusion from the experimental results[6] can be drawn. First, based on light-emitting structures of coherent and non-coherent natures varying from blue-green to near-infrared parts of the spectrum, including the structures with a broad emission band in a single emitting element, and tunable lasers in a large wavelength range can be created. Second, the wideband emission spectrum of heavy doped AlGaN samples gives premises for developing lasers with ultra-short pulses.
Considering all the above arguments, the aim of this work was to study the spectral, temporal and polarization characteristics of spontaneous and stimulated luminescence of Al0.5Ga0.5N alloys and to determine the active medium gain under optical pulsed pumping by laser radiation with λ = 266 nm. This method provides a means for investigations in a wider range of conditions than under the electron beam pumping.
2.
Experimental setup
The Al0.5Ga0.5N films studied were grown by molecular beam epitaxy in a modified Riber CBE–32 machine. Ammonia was used as a source of active nitrogen, the mixture of silane (SiH4) with nitrogen (N2) was used as a source of impurity atoms (Si). The gas mixture flow was 3 sccm; the silane content was 0.7%. The Al0.5Ga0.5N films with a thickness h0 = 1.2 μm were grown at a temperature of 860 °C and an ammonia flow of 130 sccm on (0001) oriented 400-μm-thick nitridated sapphire substrates with an AlN buffer layer with a thickness hp = 320 nm. The synthesis technology and the parameter measurement for AlGaN films are described in more detail in Refs. [6, 11]. The chemical composition of the layers obtained by the SIMS method (Secondary Ion Mass Spectrometry) is shown in Fig. 1. In AlGaN films the free electron concentration was ~ 1.4 × 1018 cm?3. The geometrical dimensions of the samples were 15 × 10 mm2. Cleaved edges of samples for the luminescence output were prepared by cutting without polishing.

class="figure_img" id="Figure1"/>
Download
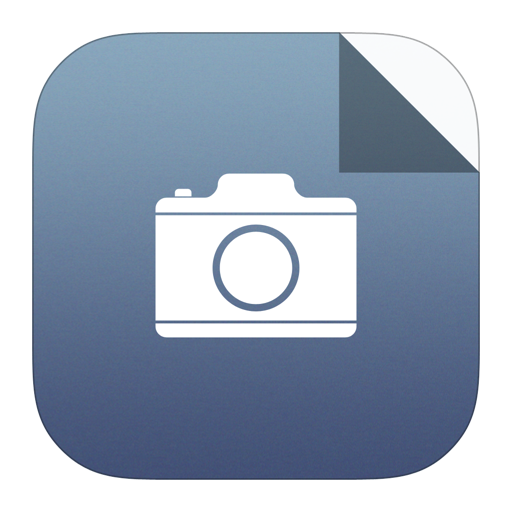
Larger image
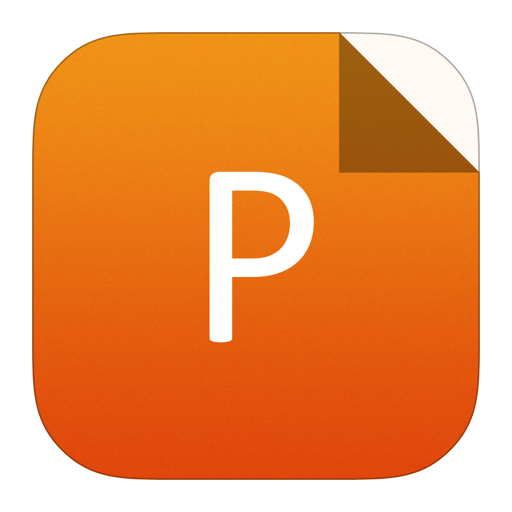
PowerPoint slide
Figure1.
(Color online) The Al, Ga, Si atomic concentrations in Al0.5Ga0.5N versus the film depth.
Fig. 2 shows the experimental scheme. Samples were excited from the side of the Al0.5Ga0.5N film. The excitation of photoluminescence (PL) was conducted using the fourth harmonic of pulsed Nd:YAG laser with λ = 266 nm; the FWHM pulse radiation was 8 ns and the repetition rate was 10 Hz. The UV pumping beam was directed perpendicular to the sample surface and it was focused on the film surface into a uniform strip with a length of about 1 cm and a width controlled by a system of two cylindrical lenses. The maximum pulse power density on the surface of the sample was W ≈ 8 MW/cm2 with the non-uniformity of its intensity along the strip less than 10%.

class="figure_img" id="Figure2"/>
Download
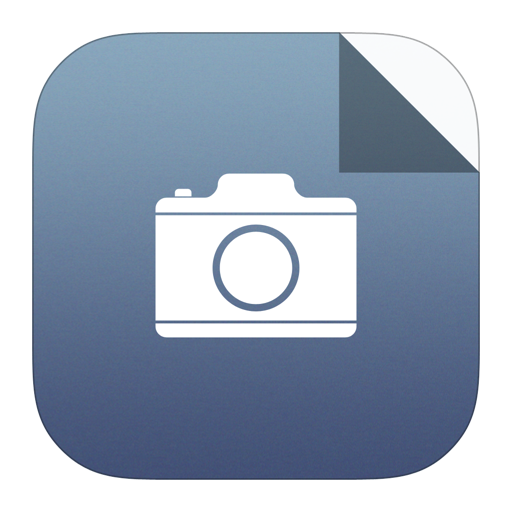
Larger image
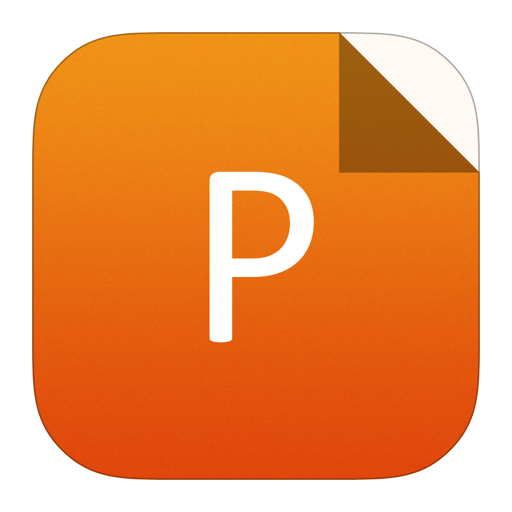
PowerPoint slide
Figure2.
(Color online) Experimental setup: 1 – sample, 2 – Glan prism polarizer, 3 – lens, 4 – spectrometer, 5 – blind, 6 – pump radiation with λ = 266 nm, 7 – recorder radiation.
The absorption depth of the pumping light and the diffusion length of charge carriers determine the depth of the excited region. The photon energy (4.66 eV) of the pumping light lies in the region of band-to-band transitions in AlGaN, where the absorption coefficient is about (1.0–1.5) × 105 cm?1. This corresponds to the absorption depth less than 100 nm[12]. The expansion of the excited region in the transverse direction takes place also due to the diffusion of electron-hole pairs. The diffusion length in AlGaN film is no more than 150 nm[13]. Thus, it can be accepted that the excited region in the transverse direction is less than 0.25 μm. The length of the excited region (excitation length) L is determined by the position of a shutter and can be changed using microscrews in the range from 0 to 1 cm with increments of 10 μm.
The PL measurement was conducted both in direction almost perpendicular to the film plane and from the cleaved edges of the film. All optical measurements were performed at room temperature using a spectrometer with calibrated wavelength and spectral sensitivity. Luminescence spectra were recorded in the spectral range of 200–750 nm using a spectrometer equipped with a CCD camera with a high linearity in a large dynamic range[14]. A spectrometer equipped with a photomultiplier was used for precise measurements; an electrical signal from the photomultiplier was recorded and averaged over more than 100 laser pulses with a Tektronix 2024 oscilloscope. Radiation was transmitted to the spectrometers using a multi-mode quartz optical fiber. A Glan prism placed in front of the spectrometer was used for measuring the linear luminescence polarization. A single– pass amplification method was used to measure the optical gain of light excited along the strip[15, 16]. The appearance of stimulated luminescence was identified through the superlinear increase in the luminescence intensity with increasing strip length.
The time-resolved PL technique was used to measure the time evolution of the radiation emission from the sample. In these experiments PL from the cleaved edges of the film was dispersed by a prism monochromator and detected by the photomultiplier and the Tektronix oscilloscope with a time resolution of 1 ns.
3.
Experimental results
Fig. 3 shows typical luminescence spectra in the perpendicular direction (1) and from the cleaved edge (2) for a 1 cm long strip. For comparison we also demonstrate a cathodoluminescence spectrum (3) emitted from the cleaved edge from the paper[6]. The PL spectrum measured in the backscattering geometry comprises a single broad band centered at λ ≈ 600 nm. At the same time, the PL spectra taken from the cleaved edge of the sample split into several almost equidistant peaks with the maxima at λ ≈ 435, 477, 528, 595 and 680 nm. The interval between these PL bands is ~ 2100 cm?1.

class="figure_img" id="Figure3"/>
Download
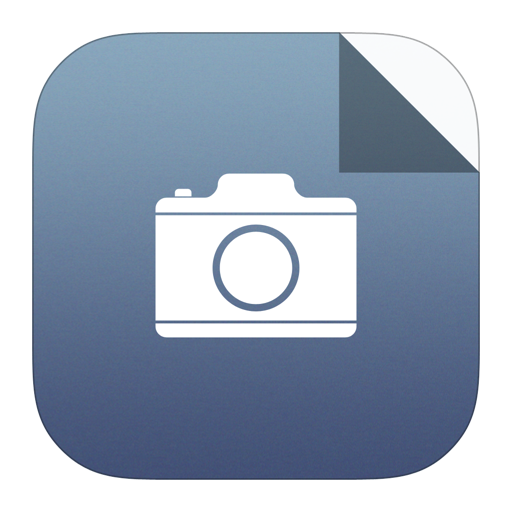
Larger image
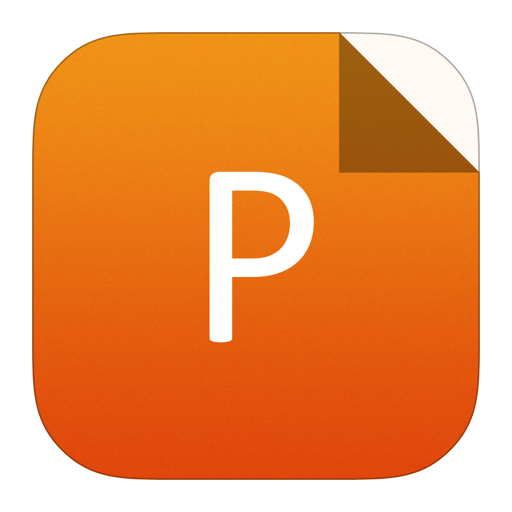
PowerPoint slide
Figure3.
(Color online) PL spectra of the Al0.5Ga0.5N film under (1, 2) the electron beam excitation[6] and (3) the optical excitation at λ = 266 nm: (1) emission in the direction perpendicular to the film; (2, 3) emission from the cleaved edge.
Fig. 4 shows the PL spectra under different excitation pumping power densities PPD being W ≈ 0.006, 0.2, 4, 40, 100, and 1000 kW/cm2.

class="figure_img" id="Figure4"/>
Download
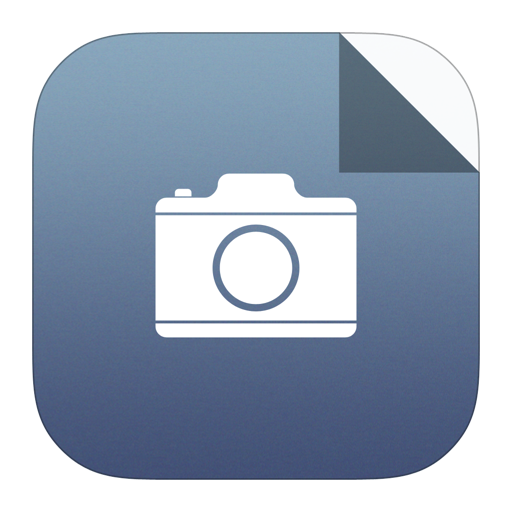
Larger image
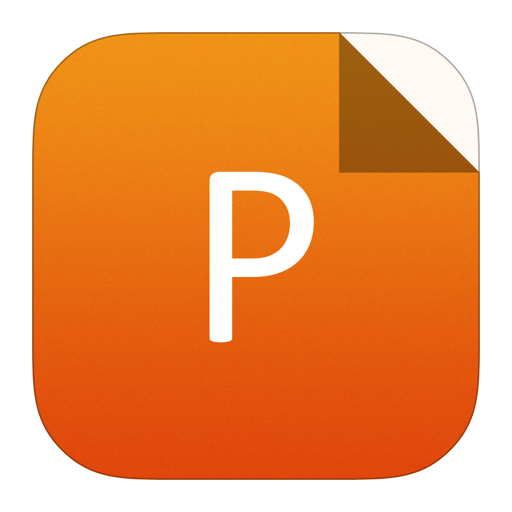
PowerPoint slide
Figure4.
(Color online) The edge luminescence spectra of the Al0.5Ga0.5N film with stripe length L = 1.2 mm for various values of the pulsed pumping power densities.
Fig. 5 shows the PL intensity in the perpendicular direction and from the cleaved edge versus the pump power lengths L = 1.2 mm. The PL intensity measured from the cleaved edge increases steeply at an excitation power density of approximately 20 kW/cm2, then up to 100 kW/cm2 and after that it varies almost directly with PPD. In contrast, the luminescence in the perpendicular direction is close to linear (see curve 1 in Fig. 5).

class="figure_img" id="Figure5"/>
Download
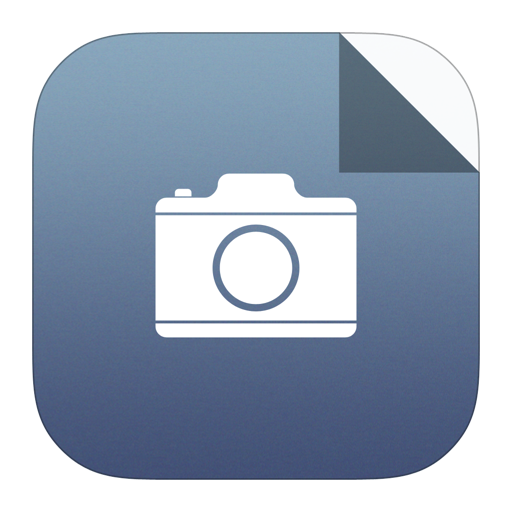
Larger image
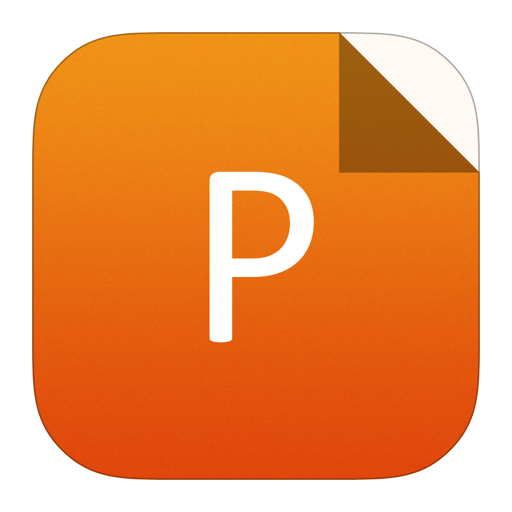
PowerPoint slide
Figure5.
(Color online) PL intensity of the Al0.5Ga0.5N film: (1) emission in the direction perpendicular to the film, (2) emission from the cleaved edge, λ = 560(1) and λ = 528(2) nm, L = 1.2 mm.
Fig. 6 shows a part of spectra from the PL edge taken near λ ≈ 528 nm without a Glan prism (2) and at different ζ angles of the polarizer. These PL spectra demonstrate the presence of two differently polarized peaks with different spectral positions.

class="figure_img" id="Figure6"/>
Download
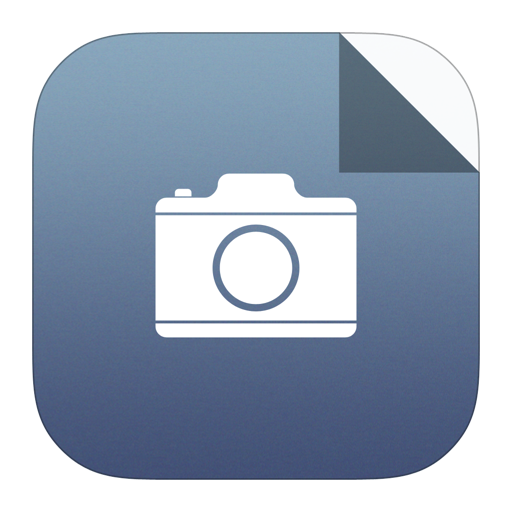
Larger image
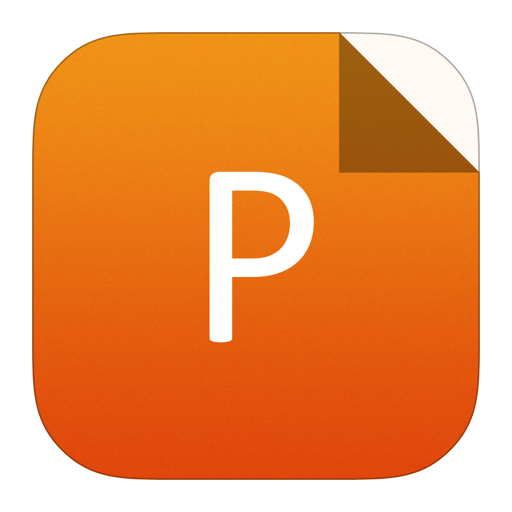
PowerPoint slide
Figure6.
(Color online) Fragments of the luminescence spectrum (1) without the polarizer and the polarizer at different rotation (2) ζ = 0°, (3) ζ = 45° , (4) ζ = 90°.
Fig. 7 shows the PL intensity IS from the edge at a wavelength of λ ≈ 528 nm versus the strip length. It is seen that, first, the intensity rapidly increases, then saturates with increasing strip length. The experimental data in the strip length range L = 0–0.13 cm is also shown by Eq. (2).

class="figure_img" id="Figure7"/>
Download
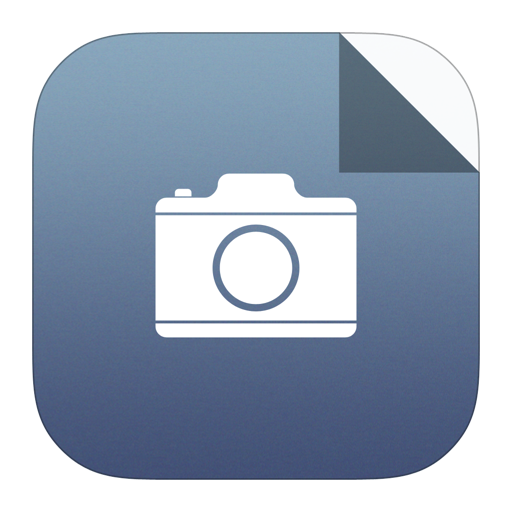
Larger image
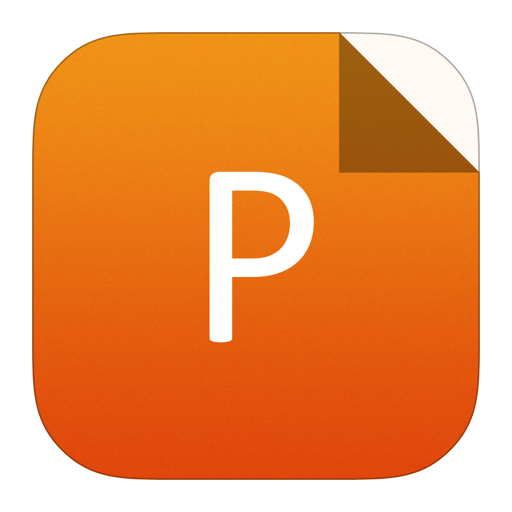
PowerPoint slide
Figure7.
(Color online) The gain of the Al0.5Ga0.5N/AlN structure. 1 – experimental data; 2 – exponential approximation of the initial section of the experimental data according to Eq. (2).
Fig. 8 demonstrates the emission pulse at 595 nm from the edge versus PPD. It is evident that it is characterized by a sum of two exponents with different power designated as τf (the fast decay time) and τs (the slow decay time). The same figure shows the fast decay time τf versus PPD.

class="figure_img" id="Figure8"/>
Download
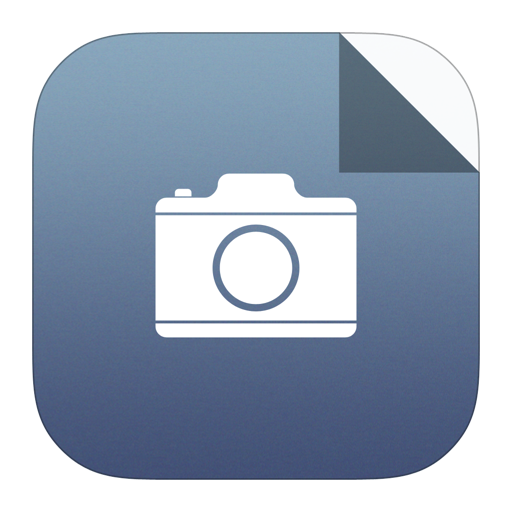
Larger image
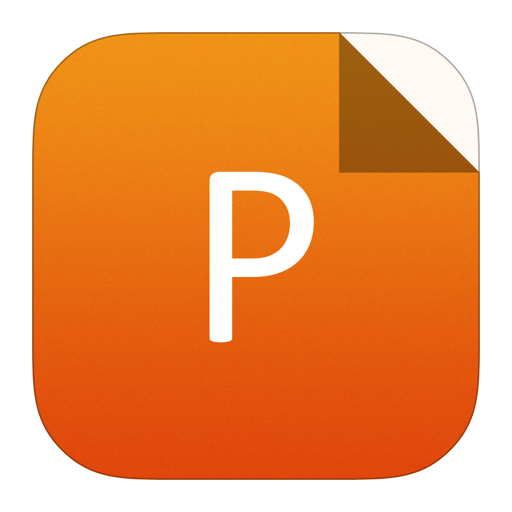
PowerPoint slide
Figure8.
(Color online) The decay time τf of the PL emission at λ = 595 nm from the Al0.5Ga0.5N film as a function of the power density W of the pump radiation (λ = 266 nm). The inset shows the typical oscillogram of the PL radiation.
4.
Discussion
The threshold character of the radiation intensity increasing at PDD by more than 15 kW/cm2 (see Fig. 5) and an exponential growth with an increase in the length of the radiation area (see Fig. 7) are indicative of the development of induced PL from the side of the structure under study. Since this radiation is spread in the waveguide structure, its spectral and polarization characteristics should be determined by the waveguide parameters. It consists of the AlGaN film with a refractive index n1 ≈ 2.244[17] of the AlN buffer layer with n2 ≈ 2.085[18] and the sapphire substrate with n3 ≈ 1.77[18]. The refractive indices were taken for λ = 530 nm (see Fig. 9).

class="figure_img" id="Figure9"/>
Download
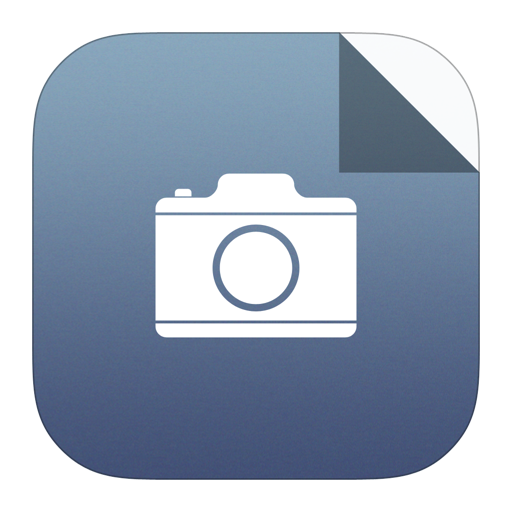
Larger image
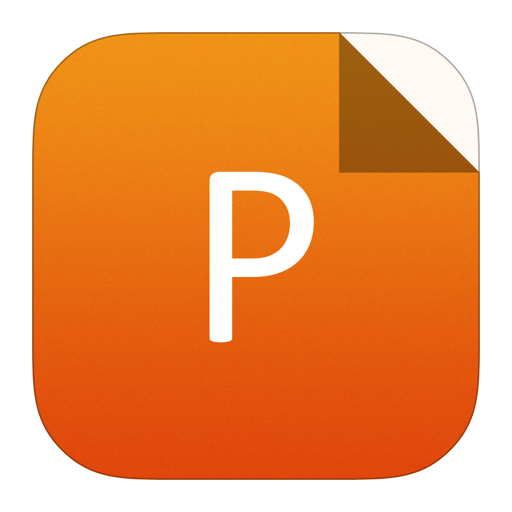
PowerPoint slide
Figure9.
The AlGaN/AlN/Al2O3 waveguide structure.
The outer surfaces of the waveguide structure contact with air with n0 ≈ 1. In this case, n1 > n2 > n3 > n0, and therefore there are several critical angles: arcsin Θ01 = n0 / n1 ≈ 26.46° at the Al0.5Ga0.5N–air interface, arcsin Θ12 = n1/n2 ≈ 68° at the Al0.5Ga0.5N–AlN interface, and arcsin Θ23 = n2 / n3 ≈ 58.1° at the AlN–Al2O3 interface. The beams coming from any point inside the AlGaN film undergo total internal reflection at angles of incidence greater than these angles[19]. Calculations based on the Fresnel formulas[19] indicate that the reflection coefficient at the Al0.5Ga0.5N film–AlN buffer layer interface is small (~ 0.14%) because of a small difference between the refractive indices of the contacting media. As a result, radiation from Al0.5Ga0.5N penetrates effectively in AlN and total internal reflection of this radiation occurs at angles of incidence more than Θ23 ≈ 58.1°. Thus, for angles 68° > Θ > 58.1° the structure comprising the Al 0.5Ga0.5N film and the AlN buffer film can be regarded as a uniform waveguide.
The light field in a waveguide can be represented as the sum of two planar TE and TM waves. These waves (modes) have mutually perpendicular polarizations and propagate in zigzag fashion owing to total internal reflection at the boundaries of the structure. For the self-consistent field distribution pattern, it is required that the total phase shifts in the waves moving from the film bottom to the top and down should be an integer multiple to 2π[19]:
$$2k{n_1}h{ m {cos}}{varTheta _{ m{m}}} + {{ m{delta }}_1} + {{ m{delta }}_2} = 2{ m{pi }}m, $$ ![]() | (1) |
where k = 2π / λ is the wave number, h = h0 + hp is the thickness of the waveguide, Θm is the angle of incidence, δ1 and δ2 are the phase shifts at total internal reflection at the boundaries of the waveguide, m is an integer number. This equation allows us to calculate the mode spacing and the output wavelength based on the known parameters of the waveguide.
The uncontrollable cleavage edge of the sample was used for the light extraction for the mode propagating at an angle Θm ≈ 52.1° (arcsinΘm = n1 / n3 provided that hp is less than the emission wavelength) of total internal reflection. Otherwise, in the case of an ideal surface of the waveguide edge cleaved perpendicular to the axis of the strip an extraction from waveguide radiation is prohibited. In this case, the extraction output is allowed at high angles of incidence greater than Θm. However, it might be that at high angles of incidence the gain decreases due to a small length of the zigzag path within the active medium and the competition modes at the homogeneous nature of the gain. At smaller angles of incidence (52.1° > Θm > 26.46°) the extraction of radiation is possible due to the leaky modes.
The obtained experimental data are in good agreement with the waveguide model, described by formula (1) with m = 8, Θm ≈ 52.1° and h = h0 + hp ≈ 1.52 μm for radiation with λ ≈ 528 nm. Phase shifts δ1 and δ2 at total internal reflection have been determined from the Fresnel formulas[19]. The spectral position of the neighboring peaks in the emission spectrum λ = 435, 477, 528, 595 and 680 nm in Fig. 4 is consistent with the model for m = 10, 9, 8, 7 and 6 respectively. Consequently, the considered waveguide model described by Eq. (1) adequately describes the experimental results (435, 477, 528, 595 and 680 nm).
It is expected that the degree of polarization of spontaneous luminescence of the Al0.5Ga0.5N film is small P ≈ 0.15[20]. Polarization measurements reveal two groups of PL bands with different positions of the maxima. This polarization appears from the co-existence in the waveguide of two TE and TM modes, with perpendicular polarization and different phase shifts δ1 in total internal reflection at the interface with the sapphire substrate. Consequently, the resonant wavelengths of these modes are different according to Eq. (1). Due to the almost homogeneous nature of the active medium gain two modes in the waveguide can compete. Despite the use of an approximate model of the waveguide the experimental value shown in Fig. 6 Δλexp = 8 nm is in satisfactory agreement with the calculation Δλcal = 11 nm.
The direct evidence of the presence of light amplification in the medium is the exponential growth of the radiation intensity with increasing length of the excitation region. The intensity of the output radiation from the cleaved edge of the sample with increasing length of the excitation region for a weak signal is given by:
$${I_{ m{s}}}left( L ight){ m{ }} = { m{ }}left( {{I_{ m{s}}}S/g} ight)left[{exp left( {gL} ight)-1} ight], $$ ![]() | (2) |
here IS is the power of spontaneous emission per unit volume, S is the cross sectional area of the excited region, g = g1 – a is the experimentally observed gain, g1 is the amplification by the stimulated emission process, a is optical loss.
The gain value per unit length for the test structure calculated by Eq. (2) is g ≈ 14.5 cm?1 at the pump power density ~ 2.2 × 105 W/cm2. Taking into account the zigzag path of light in a waveguide, which increases the actual length of the light path, the gain value should be reduced g* = g sinΘm ≈ 11.4 cm?1. Taking into account the penetration of pumping radiation less than 0.25 μm, the real gain can be as high as ~ 70 cm?1.
Eq. (2) for the light amplification under these conditions predicts an unlimited increase in the radiation intensity that does not occur in the experiment. An elongation of the active region of the medium does not lead to a proportional increase in the radiation intensity (at a given pump power density). It can be explained by the energy loss through the leaking modes and the generation of absorbing centers in the medium.
The most likely physical mechanism leading to the occurrence of broadband emission in the doped AlGaN films is a donor–acceptor pair recombination[10]. As a result of this process, photons are emitted whose energy depends on the donor–acceptor distance. Consequently, the total emission band is unhomogeneously broadened. In a laser with an inhomogeneous gain line all modes whose frequencies are within the gain band of the laser form a set of longitudinal modes that can exist simultaneously, without the mode competition. The nature of luminescence and the identification of emission bands are a subject of a separate study.
5.
Conclusion
The luminescence of heavily silicon-doped Al0.5Ga0.5N layers grown by MBE excited by a pulsed laser with λ = 266 nm was studied. A broad band peaked at about λ = 600 nm dominates in the PL spectrum measured. The same spectrum is observed from the film side at small pumping power values. From the threshold density of the pumping power PPD ~ 15 kW/cm2, the radiation intensity sharply increases and, beginning from ~ 100 kW/cm2, it grows practically linearly along with the PPD growth. The gradual reduction of the duration τf, which is faster at small pumping power values, is observed in the whole investigated PPD range. At the PPD value of 1 MW/cm2, the duration of the fast part is close to the pumping pulse duration. It is reasonable to assume that, at PPD values, the duration of the fast part characterizes the lifetime of upper working levels at the radiating transition. Based on this, it is possible to evaluate the radiation center density ΔN. Using the following formula:
$$g = Delta N times sigma, sigma = sqrt {frac{{{ m {ln}}2}}{pi }} frac{A}{{4pi c{n^2}}}frac{{lambda _0^4}}{{Delta lambda }},$$ ![]() | (3) |
where σ is the amplification cross-section[21], λ0 is peak emission wavelength, Δλ is the full width at half maximum, n is the refractive index, A = 1/τ = 3.3 × 106 s?1 (from Fig. 8 at W ≈ 0) is the Einstein coefficient. Thus, we have ΔN ≈ 9 × 1019 cm?3, which correlates with the concentration of dopant silicon atoms NSi ≈ 3 × 1020 cm?3 (see Fig. 1).
The PL spectrum taken from the cleaved edge of the sample splits into several narrow, almost equidistant, components with different polarizations. Such a transformation of the PL spectrum is due to the light propagation in a planar waveguide comprising the Al0.5Ga0.5N layer and the AlN buffer layer. The light is amplified within the waveguide. The calculated gain of the active medium is g ≈ 70 cm?1 at λ ≈ 528 nm. All results obtained indicate the stimulated nature of radiation. The width of the emission band exceeds 300 THz from 415 to 750 nm.
Acknowledgments
1. The reported study was funded by RFBR according to the research project No 16-02-00018.
2. Characterization of heterostructure were performed using SIMS facility CAMECA IMS-7f at the Federal Joint Research Center "Material science and characterization in advanced technology" with financial support by Ministry of Education and Science of the Russian Federation (id RFMEFI62117X0018).