
Cartilage on the furculae of living birds and the extinct bird Confuciusornis: a preliminary analysis and implications for flight style inferences in Mesozoic birds
WU Qian1,2,3, Jingmai K. O’CONNOR4, LI Zhi-Heng1,2, Alida M. BAILLEUL
通讯作者: *alida.bailleul@ivpp.ac.cn
收稿日期:2020-11-20网络出版日期:2021-04-20
基金资助: |
Corresponding authors: *alida.bailleul@ivpp.ac.cn
Received:2020-11-20Online:2021-04-20

摘要
飞行的早期演化是古生物研究的热点问题之一。现生鸟类演化出多种不同飞行方式,然而对中生代鸟类飞行方式的研究亟需寻找具有指示意义的指标。骨骼与关节组织尤其是乌喙骨-叉骨关节是实现鸟类飞行功能的重要组成,因此其关节的组织形态可能有助于反映现生鸟类的飞行方式。鸟类膜质骨中的次级软骨受到表观遗传的重要影响,只能在关节受到肌肉运动刺激的情况下形成,因此能够反映鸟类关节的形成力学环境;对三种不同飞行方式的现生鸟类(珠颈斑鸠、树麻雀和普通楼燕), 以及热河生物群中最为常见的古鸟类之一孔子鸟(Confuciusornis)的叉骨乌喙骨关节的组织学特征进行了分析,显示在所有三种现生鸟类中,叉骨与乌喙骨之间均存在可动关节,并且在叉骨上存在次级软骨。而孔子鸟的叉骨上也存在次级软骨,这是次级软骨组织在中生代鸟类叉骨化石中的首次报道。进一步分析发现,不同现生鸟类物种的次级软骨组织形态存在差异,还需更多数据建立相关形态功能关系以便用于帮助推断中生代鸟类的飞行方式。
关键词:
Abstract
The early evolution of flight is one of the most studied topics in vertebrate paleontology. Living birds have evolved to utilize a variety of flight styles, but studies focused on inferring flight strategies in Mesozoic birds are often contradictory and without a clear consensus, making it necessary to find additional informative characteristics that can be useful for inferences in fossils. Virtually nothing is known about the histology of the avian pectoral girdle, even though skeletal and joint tissues are key candidates to solve form-function relationships. Avian secondary cartilage found on the dermal bones of the avian skeleton is influenced by epigenetics and only forms when joints are stimulated by muscle contractions. As the only dermal bone in the avian postcranium, the furcula is a potential site for the formation of furcular secondary cartilage and merits further attention. It is still unknown whether adult living birds and fossil birds have furcular secondary cartilage. Here we present histological analyses conducted on the furcula-coracoid articulation in three living birds (Spilopelia chinensis, the Spotted dove; Passer montanus, the Eurasian tree sparrow; and Apus apus, the Common swift), taxa that utilize different flight styles, and one of the most common fossil birds of the Jehol Biota, Confuciusornis. Secondary cartilage was identified on the furculae of the living birds and of Confuciusornis, representing the first report of furcular secondary cartilage in the fossil record. Clear differences in secondary cartilage morphologies were observed in the living species, but additional data is required to establish a strong form-function relationship that could be useful for making inferences in Mesozoic birds.
Keywords:
PDF (2620KB)元数据多维度评价相关文章导出EndNote|Ris|Bibtex收藏本文
本文引用格式
吴倩, 邹晶梅, 李志恒, 艾莉达. 现生鸟类和孔子鸟(Confuciusornis)叉骨软骨的研究:初步分析以及对中生代鸟类飞行方式的启示. 古脊椎动物学报[J], 2021, 59(2): 106-124 DOI:10.19615/j.cnki.1000-3118.201222
WU Qian, Jingmai K. O’CONNOR, LI Zhi-Heng, Alida M. BAILLEUL.
1 Introduction
The evolution of flight at the dinosaur-bird transition and its refinement during the early radiation of Mesozoic birds is one of the most studied macroevolutionary innovations in vertebrate paleontology (e.g., Jenkins, 1993; Dial et al., 2008; Dececchi and Larsson, 2011; Brusatte et al., 2014). The energy demands associated with powered flight are extremely high (Bishop and Butler, 2015), and a variety of flight strategies exist in living birds (Neornithes) to optimize the energy costs generated during wing flapping. For example, instead of continuously flapping their wings, some small birds intermittently flap and glide with spread wings to save energy (Usherwood, 2016). Others employ a bounding flight which involves an intermittent ballistic phase with wings folded against the body, which was apparently utilized by many Cretaceous enantiornithines (Usherwood, 2016; Serrano et al., 2018). Larger birds with elongated wingspans or broad wings evolved the ability to soar using continental or marine air currents. These birds can fly for long distances with very few flapping motions (Butler, 2016; Serrano and Chiappe, 2017).Complex form-function relationships exist between flight styles and the morphology of the flight apparatus in extant birds (e.g., Shatkovska and Ghazali, 2017). These differences are expressed through variation in the shape and size of the bones forming the pectoral girdle (i.e., furcula, scapula, and coracoid), the forelimb (e.g., humerus), proportions of the flight muscles, and/or in the shape and size of flight feathers. For example, morphometric analyses have demonstrated that the shape of the furcula and the interclavicular angle vary depending on flight strategy (e.g., soaring birds have a U-shaped furcula, a morphology rarely seen in flapping taxa or wing propelled divers; see Hui, 2002; Close and Rayfield, 2012). The furcula consists of two medially fused clavicles, colloquially known as the ‘wishbone’, and was hypothesized by Vickaryous and Hall (2010) to be homologous with the interclavicle of crocodylians (even though a more recent study suggests that the homology of the furcula is still not fully resolved; see Hall and Vickaryous, 2015).
Since the morphology of the avian skeleton can be utilized to discriminate between some flight styles, attempts have been made to infer the flight strategies of Early Mesozoic birds using skeletal remains. The considerable morphological disparity observed in the flight apparatus of various lineages of Mesozoic birds suggests that a variety of flight styles similar to that observed in modern birds most likely already existed in the Early Cretaceous (e.g., Close and Rayfield, 2012; Serrano and Chiappe, 2017; Liu et al., 2017; Serrano et al., 2018).
Different methods have been used to infer flight styles in extinct birds. The most widely applied method involves morphofunctional analyses based on parameters of aerodynamic efficiency (e.g., wing span and body mass estimates) developed by Serrano et al. (2017) which have been applied to reconstruct the flight modes in several enantiornithines and the basal pygostylian Sapeornis(Liu et al., 2017; Serrano and Chiappe, 2017; Serrano et al., 2018; Chiappe et al., 2019a, b). Close and Rayfield (2012) used morphometric analysis coupled with phylogenetic comparative methods on furculae to infer flight styles in numerous fossil birds. Other authors noted that non-skeletal soft tissues of the wing also hold potential clues to reconstruct flight behavior, such as the shape of the pro- and postpatagium and thickness of the primary feather rachises, which have been used to make inferences regarding flight in the early pygostylian Confuciusornis (Falk et al., 2016). Preserved barb angles of flight feathers have also been used to inform on flight strategies (e.g., Feo et al., 2015), although this relationship has subsequently been questioned (Wang et al., 2019a).
Despite the diversity of available methods, the early evolution of flight and flight styles in Mesozoic birds remain controversial (e.g., Wang et al., 2019a). Studies often show contradicting results for the same species and discussions are ongoing regarding whether stem birds (like the two key species Archaeopteryx and Confuciusornis) exhibited active (i.e., powered by flapping) or passive (i.e., gliding) flight (see Vazquez, 1992; Senter, 2006; Nudds and Dyke, 2010; Feo et al., 2015; Wang et al., 2019a). It is therefore crucial to find additional markers of flight style or informative characteristics in extant birds that can be useful for flight inferences in fossils. Such markers may complement previous morphometrics and soft tissue analyses. Histological markers within the skeleton are ideal candidates to resolve questions relating to form and function since skeletal tissues like bone and calcified cartilage are most prone to fossilization (because they are mineralized) and because they react to- and reflect the biomechanical environment in which they were formed and/or maintained (Murray and Drachman, 1969; Carter, 1987; Mu?ller, 2003).
Ponton et al. (2007) and Mitchell et al. (2017) compared the bone histology of furculae (cross-sections of the ramus) in a variety of birds and showed that soaring birds generally have higher haversian bone densities (i.e., more secondary osteons resulting from bone remodeling) than flapping birds due to the different biomechanical regimes experienced during flight. In the present study, we propose that new histological markers of flight styles may also be found on the articular surface of the epicleideal process (epicleideum) of the furcula, also known as the processus acromialis, which articulates with the processus acrocoracoideus of the coracoid bone (sensu Baumel and Raikow, 1993). This joint, formally called the acrocoracoclavicular joint (Baumel and Raikow, 1993), is here referred to as the furcula-coracoid joint for readability.
From a developmental perspective the furcula is very interesting because it is the only dermal bone (synonymous with membrane bone) in the postcranial skeleton (Hall, 2001). All other postcranial bones are part of the endoskeleton and therefore form very differently from dermal bones (Kardong, 2019). Similarly, it is the only bone in the pectoral girdle to have an embryonic origin from the neural crest cells. All other elements of the pectoral girdle (and those located more caudally as well) are derived from the mesoderm (Ponomartsev et al., 2017).
The two clavicles originally formed part of the dermatocranium (in the opercular bone series) in early aquatic vertebrates. During the early evolution of tetrapods these bones ‘detached’ and got incorporated into the pectoral girdle (McGonnell, 2001; Kardong, 2019). Like all other bones forming the dermatocranium, the furcula forms through direct intramembranous ossification during embryonic development. In contrast, all other skeletal element involved in flight (e.g., coracoid, scapula, humerus) form through indirect endochondral ossification involving bone replacement of a primary cartilage model (Hall, 2001). This unique type of bone formation (and the embryonic origin from neural crest cells) within the flight apparatus also reflects unique tissues. Hall (1986) found that cartilage arises on the furcula of developing chick embryos. This cartilage, called ‘secondary cartilage’ (sometimes also referred to as ‘adventitious cartilage’), originates from periosteal stem cells after ossification and is distinct from the primary cartilage that precedes ossification (endochondral ossification) in the bones of the endoskeleton (Murray and Smiles, 1965; Hall, 1967, 2000). Secondary cartilage is solely found on dermal bones and is highly sensitive to mechanical stimuli such as muscle contractions; its formation and maintenance in birds is apparently governed by epigenetics, more so than primary cartilage (Murray and Drachman, 1969; Hall, 1972; Persson, 1983). Among all extant sauropsids this tissue is only found in Neornithes (which is why it was formerly referred to as ‘avian secondary cartilage’; Hall, 2000). Crocodiles, turtles and lepidosaurs do not form secondary cartilage on their dermal bones (Hall, 2000). However, it was also recently found on the dermal bones of the skulls of some ornithischian dinosaurs (Hypacrosaurus, Bailleul et al., 2012, 2013) and the fossil ornithurine bird Yanornis (Bailleul et al., 2019a), suggesting this avian tissue evolved millions of years ago, and most likely has a dinosaurian origin (Bailleul et al., 2012).
This cartilaginous tissue was also proposed to be a useful indicator of cranial joint movement in fossil birds and other dinosaurs (Bailleul et al., 2019a, b). Using the same principle, we propose that the presence and/or the type and amount of secondary cartilage on the furcula has the potential to inform on flight-related movements, or at least the biomechanical environment in the furcula-coracoid joint created by flight-induced stimuli. Investigation into the furcula-coracoid joint in living birds with different flight capabilities has the potential to reveal histological characteristics that pertain to particular flight styles, which in turn may be used to make more robust inferences of joint structure and function in Mesozoic birds and complement existing morphometric analyses.
To our knowledge published histological studies on the furcula-coracoid joint of adult birds are non-existent (only works on embryos exist; e.g., Russell and Joffe, 1985; Hall, 1986; Vickaryous and Hall, 2010). According to Baumel and Raikow (1993), the furcula-coracoid joint can be a ‘syndesmosis’, a ‘symphysis’ or a ‘typical synovial joint’ depending on the avian species but clear histological images are not presented. In avian embryos, no secondary cartilage was reported on the developing furcula in quails (Russell and Joffe, 1985), but secondary cartilage does arise on the furcula in the chick after the 9th day of incubation, only to disappear rapidly during the next day (it is simply a ‘transitory secondary cartilage’; Hall, 1986, 2001). It is still unknown if adult birds have furcular secondary cartilage, but it is logical to hypothesize that it would be present. Secondary cartilage, if present on the epicleideum would function as a cushion between the furcula and the coracoid, acting as an articular cartilage, and accommodate flight induced stress.
In the present study we test two primary hypotheses: 1) secondary cartilage is present on the furcula in adult birds across the phylogeny of Neornithes - we preliminarily test this hypothesis by investigating three species of living birds (the Spotted dove, the Eurasian tree sparrow and the Common swift); 2) the presence of secondary cartilage on the furcula is not unique to Neornithes but was also present in stem birds. For this, we sampled a disarticulated furcula of Confuciusornis (IVPP V 11521) one of the most common Mesozoic birds from the Early Cretaceous Jehol Biota (Wang et al., 2019b). We used paraffin histology to study the extant specimens, and ground sections and demineralization for the fossil sample to test our two main hypotheses. Additionally, the scapula-coracoid of this same specimen (V 11521) was also histologically sampled to analyze the other side of the joint (where the furcula articulates).
Lastly, because the three living species have different flight styles (i.e., continuous flapping for the Spotted dove; flapping-gliding flight for the Common swift; and bounding flight mixed with flapping-gliding for the Eurasian tree sparrow; Bruderer et al., 2010; Shatkovska and Ghazali, 2017) we preliminarily investigate a third question of whether any obvious histological differences can be used to discriminate between flight categories (e.g., presence/absence of secondary cartilage; type/amount of secondary cartilage; or joint type), keeping in mind that a much larger sample size of birds must be investigated in the future in order to fully resolve this question. We report three main findings: the histology of the furcula-coracoid joint in living birds; the presence of secondary articular cartilage on the furcula of adult birds; and the first evidence of fossilized secondary cartilage on the furcula of a fossil bird. We discuss new potential avenues to infer flight styles in Mesozoic birds, pending a more in-depth analysis of a larger sample of extant birds.
2 Materials and methods
Specimens We used three frozen specimens of adult living birds (or relatively close to skeletal maturity based on size and plumage) from China: Spilopelia chinensis (Spotted dove); Passer montanus (Eurasian tree sparrow) and Apus apus (Common swift) (Fig. 1). These specimens (cadavers) were donated to Institute of Vertebrate Paleontology and Paleoanthropology, Chinese Academy of Sciences (IVPP) by the Beijing Wildlife Rescue Center a few years ago.Fig. 1
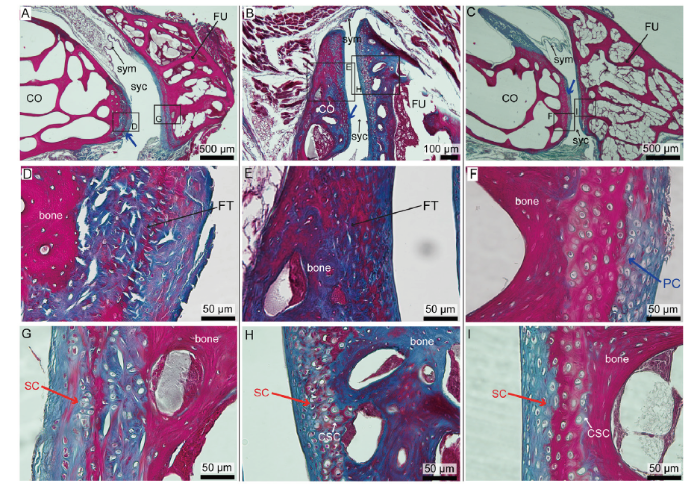
Fig. 1Histology of the furcula-coracoid joint in the Spotted dove, the Eurasian tree sparrow and the Common swift with paraffin slides stained with a modified Masson’s trichrome
A, D, G. images of the joint in the Spotted dove; B, E, H. images of the joint in the Eurasian tree sparrow; C, F, I. images of the joint in the Common swift. A-C. show the synovial joints at low resolution; D-F. close-ups on the primary cartilage on the coracoid; G-I. close-ups on the secondary cartilage on the furcula Abbreviations: CO. coracoid; CSC. calcified secondary cartilage; FT. fibrous tissue; FU. furcula; PC. primary cartilage; SC. secondary cartilage; syc. synovial cavity; sym. synovial membrane
Confuciusornis (IVPP V 11521) is a disarticulated partial skeleton consisting of a sternum, ribs, vertebrae, furcula (Fig. 2), scapulocoracoid (Fig. 3), humeri, pelvis, femora and a partial tail (Hou et al., 1999). All elements were previously prepared free from the matrix and the furcula appears as an isolated skeletal element that is laying on top of the midshaft of a partial humerus (Fig. 2A). Although this specimen was originally described as the paratype of Confuciusornis dui (Hou et al., 1999), this referral is unsupported since V 11521 preserved none of the elements on which the diagnosis of this species is based nor is it small in size compared to other specimens of Confuciusornis (notably, size is not a reliable indicator in this clade due to observed developmental plasticity; Chinsamy et al., 2020). We consider this specimen to be most likely C. sanctus but to be conservative we refer the specimen to Confuciusornis indet. The other humerus (midshaft) of this specimen is being analyzed for another study to determine its age (data not shown).
Fig. 2
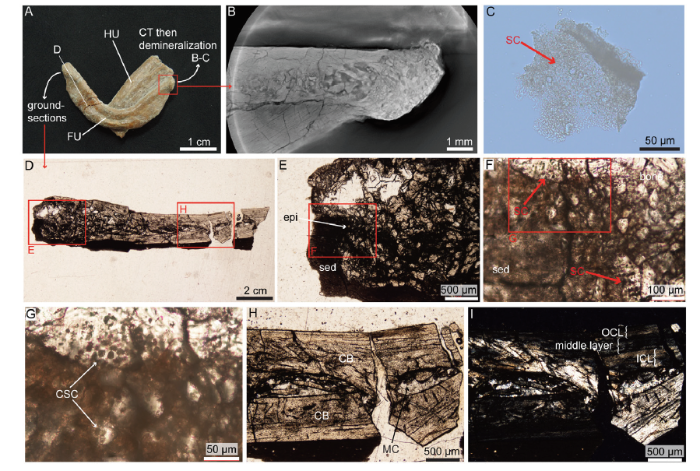
Fig. 2Morphology, CT-scans and histology of the furcula of Confuciusornis (IVPP V 11521)
A. photograph of the furcula; B. CT-scan of the left epicleideal process of the furcula; C. demineralized piece of cartilage from the left epicleideal process after 48 hours in EDTA; D. longitudinal ground-section in the right epicleideal process of the furcula; E-G. close-ups of that epicleideal process showing calcified secondary cartilage; H-I. close-ups on the rami seen in D, shown under natural transmitted light (H) and cross-polarized light (I)
Abbreviations: CB. cortical bone; CSC. calcified secondary cartilage; epi. epicleideum; FU. furcula; HU. humerus; ICL. inner circumferential layer; MC. medullary cavity; OCL. outer circumferential layer; SC. secondary cartilage; sed. sediment
Fig. 3
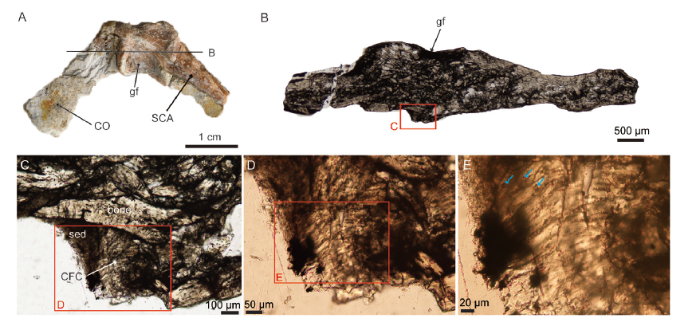
Fig. 3Morphology and histology of the scapulocoracoid of Confuciusornis (IVPP V 11521)
A. photograph of the fused scapulocoracoid showing the coracoid on one side (CO), the scapula (SCA) on the other side, and the glenoid fossa (gf) for the articulation of the humerus in between; B. a ground-section (cross section) through the glenoid fossa and on the opposite side, remnants of calcified fibrocartilage (CFC) can be seen with some sediment (sed) in a concave area; C-E. close-ups on the fibrocartilage, a few chondrocyte lacunae can be seen as well (blue arrows)
CT-scanning of extant species The frozen extant specimens were thawed and the right and left shoulder joints were dissected (the furculae were cut in the midline). The joints were put in 10% neutral buffered formalin (NBF) and each right shoulder joint was CT-scanned on a microCT scanner at IVPP (voxel size 25.093 μm for the dove and sparrow; voxel size 32.935 μm for the swift). CT data is stored and accessible online at OSF (https://osf.io/wtgck/ and https://osf.io/qntgp/).
Paraffin histology and staining of extant specimens After the dissected shoulder joints were left into NBF for at least 48 hours, the left joints were prepared for cross-sections of the furcula-coracoid joint. The fixed joints were demineralized in HCl and EDTA (JYBL-II, Cat DD0017, Leagene) for 24 to 48 hours. After demineralization, the samples were processed following a standardized protocol for paraffin sections (e.g., see Bailleul et al., 2017): they were dehydrated with a graded series of ethanol (in 70%, 80%, 90%, 95%, and 100% EtOH for 1 hour each, and two additional 100% EtOH of 1 hour), cleared in xylene (three change of 30 min each), infiltrated in melted paraffin wax (3 changes of 30 min) and embedded manually (Paraplast Plus EMS Cat#19216). Embedded samples were sectioned at 5 μm on a rotary microtome (Leica Biosystems RM2265) and mounted on charged slides (Superfrost Plus, Fisher Scientific).
Dried slides were stained using a modified Masson’s trichrome (Witten and Hall, 2003) as follows: deparaffinized in xylene (2 changes for 5 min each), dehydrated with graded series of ethanol (100%, 95%, 90%, 80%, 70% and 50% for 1 min each), rinsed in deionized water for 2 min, stained for 10 min with Mayer’s acid hematoxylin (Ruitaibio), rinsed in deionized water for 1 min, stained with Xylidine Ponceau/Acid Fuschin for 2 min (equal volumes of 0.5% xylidine ponceau 2R CI no. 16150 in 1% acetic acid and 0.5% Acid Fuchsin CI no. 42685 in 1% acetic acid), rinsed for 1 min in deionized water, stained for 4 min with 1% phosphomolybdic acid, rinsed for 1 min in deionized water, stained with light green for 90 seconds (2% light green in 2% citric acid, diluted 1 :10 with deionized water prior to use) and rinsed in deionized water for 1 min. Sections were then dipped twice in 100% ethanol for 10 seconds, cleared in xylene for 4 min (1 min×4), and coverslipped with Permount. Stained slides were observed under natural light using a Nikon eclipse LV100NPOL and photographed with a DS-Fi3 camera and the software NIS-Element v4.60.
CT-scanning of Confuciusornis The Confuciusornis furcula was first scanned whole at the IVPP on a flat scanner (160 KV Micro-computed Laminography system; voxel size 45.27 μm) and then a second time with a focus on the left epicleideal process (Fig. 2B) (voxel size of 5.28 μm; same scanner and parameters; stored online at OSF: https://osf.io/qe9r6/). CT-scans of high resolution of the left epicleideal process were analyzed to investigate the presence of secondary cartilage, but since the resolution was not enough to confidently identify potential remnants of cartilage, additional histological analyses were necessary.
Demineralization of Confuciusornis The left epicleideal process was cut with a dremel equipped with a rotating diamond blade and demineralized in a solution of EDTA (disodium ethylenediaminetetraacetic acid) (0.5 M; pH 8.0) for three weeks. The original plan was to make 5 μm paraffin slides of demineralized Confuciusornis furcula; however, most of the tissues were lost during microtome sectioning and apparently did not adhere to the glass slides (which only show sediment grains; data not shown). However, we were still able to photograph some ‘floating’ tissues after 48 hours in the demineralizing solution without making paraffin sections (Fig. 2B). For this, a few drops of solution were pipetted on top of a glass slide which was coverslipped and rapidly photographed.
Ground-sections - Histology of Confuciusornis Concomitantly, the right process of the furcula was broken off (Fig. 2A) and three longitudinal ground sections were made for a complete and robust histological analysis. The scapulocoracoid was also prepared for histology. The broken fragment of the furcula and the scapulocoracoid were embedded in EXAKT Technovit 7200 one-component resin and allowed to dry for 12 hours, cut into slices and polished until the desired optical contrast was reached (slice thickness ~50-70 μm). Ground sections were made and observed under natural and polarized light using a Nikon eclipse LV100NPOL and photographed with a DS-Fi3 camera and the software NIS-Element v4.60. The scapulocoracoid was analyzed to look for any trace of primary cartilage on the surface opposite to the glenoid fossa, where the furcula is expected to articulate (Fig. 3).
3 Results
Furcula-coracoid joint histology in extant birds Cross-sections between the processus acromialis of the furcula and the processus acrocoracoideus of the coracoid show that the joint is synovial in all three species (Fig. 1A-C). In each specimen, a synovial cavity can be observed between the two bones and is delimited by a thin undulating synovial membrane (Fig. 1A-C). Articular cartilage can be seen covering the ends of the furcula and coracoid within the synovial cavity: given the developmental origins of the bones (the coracoid is endochondral, the furcula intramembranous), the articular cartilage constitutes primary cartilage on the coracoids (blue arrows; Fig. 1A-C and close-ups in Fig. 1D-F), and secondary cartilage on the furcula (Fig. 1G-I).In each species, the furcula is slightly convex and the coracoidal facet is concave although in the Eurasian tree sparrow this concavity is not very pronounced (Fig. 1B). In all three species, the furcula is filled with red bone marrow (Fig. 1A-C). This result is not surprising given that red bone marrow in the furcula was previously reported by Schepelmann (1990) in adult pigeons, which belong to the same avian order as the Spotted dove (Columbiformes). However, in many other extant birds the furcula is pneumatized and without red bone marrow (O’Connor et al., 2004; Canoville et al., 2019). The coracoid of the Spotted dove and the Common swift are pneumatic (filled with air-sacs and air pockets; Fig. 1A, C) but in the Eurasian tree sparrow it is filled with red bone marrow (Fig. 1B).
High magnification of the articular cartilage reveals clear differences between each species. First, the primary cartilage of the Common swift shows the typical aspect of articular cartilage with round chondrocytes embedded in a hyaline extracellular matrix (Fig. 1F). However, in the Spotted dove and the Eurasian tree sparrow the primary cartilage does not show round chondrocytes (Figs. 1D, E) and the observed tissues are more reminiscent of a ligament or tendon being transformed into cartilage with potential metaplasia of fibroblasts cells into cartilage-like cells. Metaplasia is a third way of cartilage formation and it is curious to observe this type of tissue within the coracoidal articular cartilage of the dove and sparrow.
The primary (Fig. 1F) and the secondary cartilage of the swift (Fig. 1I) are quite similar and show the same zonal arrangement as that observed in the articular cartilages (primary and secondary) of adult duck heads (stained with the same modified Masson’s trichrome; Bailleul et al., 2017). In the swift, the primary and secondary cartilage shows four main zones stained in different colors: the two most external zones made of hyaline cartilage stain blue (called the superficial tangential zone and the middle zone); the deep zone stains pink; and the calcified cartilage zone adjacent to the subchondral bone stains light purple (Fig. 1F, I). The calcified zone of the secondary cartilage (which would be expected to fossilize better than the rest of the cartilage since it is mineralized) is however extremely thin in this species (with a maximum thickness of about 20 μm in the studied section; Fig. 1I).
The secondary cartilage in the Spotted dove and Eurasian tree sparrow show clear round chondrocytes (unlike their primary cartilage) but the clear zonal arrangement like that observed in the swift is lacking (Fig. 1G, H). In the Eurasian tree sparrow, the deep pink zone and the light purple calcified layer are hard to distinguish. There is an apparent irregular calcification surrounding the deep chondrocytes (see the very small purple rings in the territorial matrix around the cells), embedded into a pink intraterritorial matrix (Fig. 1H). In the Spotted dove, the secondary cartilage is thick and highly fibrous (Fig. 1G) but it is unclear if any of this secondary cartilage is calcified (no clear light purple layer typical of calcified cartilage can be seen). Determining whether the secondary cartilage of the dove furcula is a calcified fibrocartilage or not will necessitate further work and sampling.
Furcula histology of Confuciusornis The furcula of Confuciusornis has previously been described as boomerang-shaped and lacking a hypocleidium (Chiappe et al., 1999; Hou et al., 1999) (Fig. 2A). Even our high resolution CT scans were not powerful enough to clearly determine the presence or absence of cartilage (Fig. 2B), therefore, histological analyses were necessary. After demineralizing the left epicleideal process for 48 hours, some tissue was detached from the bone and showed clear round chondrocyte lacunae embedded in a light extracellular matrix, which constitutes fossilized secondary cartilage (Fig. 2C). A longitudinal ground section through the right epicleideum and ramus of the same furcula (Fig. 2D) shows a relatively thick layer of calcified cartilage with numerous round chondrocyte lacunae (Fig. 2E-G). In some areas, these cells appear to be organized in rows (Fig. 2F, lower arrow).
The right ramus is made of compact parallel-fibered bone with a laminar vascularization (Fig. 2H-G). The bone close to the epicleideal process is crushed and difficult to analyze, but in the more distal ramus no secondary reconstructions can be seen. The medullary cavity can still be identified between the two crushed cortical layers. The cortex is composed of three layers: an inner circumferential layer (ICL) with flat osteocyte lacunae, a middle layer significantly more vascularized with dense, plump osteocytes and an outer circumferential layer (OCL) which is almost avascular and has flat osteocytes (Fig. 2H, I). No line of arrested growth (LAG) is observed.
Scapulocoracoid histology of Confuciusornis Because secondary cartilage is found on the epicleideal process of the furcula in Confuciusornis (Fig. 2), it is logical to assume that a layer of primary cartilage exists on the other side of the joint on the scapulocoracoid (Fig. 1). The scapulocoracoid of this same specimen (IVPP V 11521; Fig. 3) shows evidence of a calcified fibrocartilage with a few chondrocyte lacunae visible on the surface opposite to the glenoid fossa. This fibrocartilage (Fig. 3C, D) most likely was part of the joint linking the furcula to the scapulocoracoid in Confuciusornis.
4 Discussion
The early evolution of the avian flight is complex and much remains unknown. Flight style inferences relying on morphometric methods have produced contradicting results. For example, Serrano et al. (2018) inferred that some Early Cretaceous Spanish enantiornithines (Concornis lacustris and Eoalulavis hoyasi) had a mixed type of flight involving continuous flapping and bounding, whereas Close and Rayfield (2012) inferred that these same species had a flap-gliding type of flight. The most controversial topic in the evolution of the avian flight concerns the passive versus active flight debate for stem birds like Archaeopteryx and the key species studied here, Confuciusornis (Vazquez, 1992; Senter, 2006; Nudds and Dyke, 2010; Feo et al., 2015; Falk et al., 2016; Wang et al., 2019a). Inferring flight styles in Mesozoic birds is no easy task because the form-function relationships between flight apparatus and flight strategies in living birds are not so clear-cut (e.g., Close and Rayfield, 2012) and the morphology of the flight apparatus of stem avians is unlike that observed in extant analogues (e.g., absence of an ossified sternum in Archaeopteryx and Sapeornis).Although largely overlooked, the furcula plays an important mechanical role during flight in extant birds by stabilizing the shoulder joint and acting as a mechanical spring (Jenkins et al., 1988). It is also sometimes associated with the interclavicular air sac and therefore also plays a role in respiration in some species. Its unique developmental origin (as a dermal bone) when compared to the rest of the pectoral bones suggests it merits further investigation, especially regarding the surface where it articulates with the rest of the pectoral girdle and may form secondary cartilage. The formation and maintenance of secondary cartilage within cranial joints of developing chicks is dependent upon embryonic motility and active joint movement. Early experimental studies have shown that secondary cartilage arises mostly under compression (e.g., Hall, 1967, 1972, 1986), and that it does not form during paralysis (Murray and Drachman, 1969; Hall, 1972; Persson, 1983). This is unlike primary cartilage, which forms both under normal and paralyzed conditions (Murray and Drachman, 1969; Hall, 1972; Persson, 1983). The authors of these early studies concluded that mechanical stimuli and physical movement directly within the joint (i.e., active movement sensu Müller, 2003) are responsible for the formation of secondary cartilage (Murray and Drachman, 1969). This led Bailleul et al. (2019a, b) to similarly conclude that if fossilized secondary cartilage is found within a joint in an extinct bird (or other dinosaur), it could be used as an indicator of movement and give cues as to the biomechanical environment of the joint.
Similarly, if the furcula-coracoid joint experiences drastically different degrees of mobility related to differences in flight strategies utilized by various taxa (which is associated with different types of forces, or amounts of force), we may expect clear differences in the histology of the furcular secondary cartilage (e.g., in the amount, the type of secondary cartilage, the degree of calcification of secondary cartilage, or more generally in the joint structure). This study serves as a preliminary investigation into this issue. The data presented here provide a starting point for a more robust investigation involving a greater number of taxa and a more quantitative analysis in the future. The preliminary data gathered from the three living species (Spotted dove, Eurasian tree sparrow, and Common swift) and one fossil taxon (Confuciusornis) are discussed below.
Furcula-coracoid joint structure All three extant taxa sampled in this study, the Spotted dove (Spilopelia chinensis), the Eurasian tree sparrow (Passer montanus) and the Common swift (Apus apus), have a synovial joint between their furcula and coracoid (Fig. 1). Although no histology is shown, Baumel and Raikow (1993) report that this joint type is typical of many other groups (penguins, razorbills, pelicans, storks, sea eagles and hornbills: Spheniscus, Alca, Pelecanus, Ciconia, Haliae?tus and Buceros; Baumel and Raikow, 1993:161-162). We found clear evidence of secondary cartilage in each of the three species, which unequivocally confirms that adult birds do have secondary cartilage on their furculae, confirming our first hypothesis. We anticipate that this is widespread across the avian phylogeny, although variations will exist. For example, Fürbringer (1888) reported that the furcula-coracoid joint is completely ossified as a synostosis in frigatebirds and thus furcular secondary cartilage is most likely absent in this group in adults (also see Baumel and Raikow, 1993:162).
We also show the presence of secondary cartilage in Confuciusornis, representing the first evidence of this tissue on the furcula of any fossil bird, confirming our second hypothesis (Fig. 2). The region opposite to the glenoid fossa on the fused scapulocoracoid of this same specimen (IVPP V 11521) shows evidence of a small patch of calcified fibrocartilage, which undoubtedly represents part of the connective tissue that linked it to the furcula (Fig. 3). This histological data allows us to completely rule out the option that the furcula-scapulocoracoid joint of Confuciusornis was a syndesmosis (i.e., a suture composed essentially of collagen fibers between the two bones). The joint may have been synovial, as observed in the three extant species studied here, or a synchondrosis, with cartilage on each side linked together by fibrous, collagenous tissue, somewhat synonymous or similar to the term ‘symphysis’ used by Baumel and Raikow (1993:61). Currently, no histological characteristics have been identified that can unequivocally differentiate between these two types of joints in fossil specimens (see discussion in Bailleul et al., 2019a).
Potential form-function relationship at the furcula-coracoid joint All three species analyzed here utilize different flight styles; the Spotted dove employs continuous flapping, whereas the Common swift is a flap-glider and the Eurasian tree sparrow has a combination of bounding flight mixed with flapping-gliding (Bruderer et al., 2010; Shatkovska and Ghazali, 2017). Despite these differences, all three taxa possess a synovial joint morphology. The other species Baumel and Raikow (1993) reported as also having a synovial furcula-coracoid joint further encompass a wide variety of flight styles, including species that cannot fly but use their wings in wing-propelled swimming (i.e., penguins) or those that are poor flyers (i.e., razorbills). This preliminary data suggest that joint type will not be as informative as other histological features to discriminate flight styles, but it would be useful to collect histological data on the species listed by Baumel and Raikow (1993) to both confirm their report and gather additional histological details.
In contrast, clear differences were observed in the secondary cartilage histology in each extant species (Fig. 1G-I): in the dove fibrocartilage is observed, which may or may not be partially calcified; in the sparrow it is irregularly calcified; and in the swift it resembles typical articular cartilage with globular chondrocytes showing a zonal arrangement (already described in another avian species; Bailleul et al., 2017). Unfortunately, whether these differences are due to any particularity of their flight styles and/or other factors (like phylogeny or ontogeny) will necessitate an increase of our sample size; thus, we cannot confirm our third hypothesis at this time.
Flight style of Confuciusornis and brief discussion about furcular secondary cartilage in non-avian dinosaurs The flight style of Confuciusornis is highly debated. For example, Falk et al. (2016) hypothesized that this species had a unique flight style that is not present in modern species, while others have suggested that it was not even capable of powered flight (e.g., Nudds and Dyke, 2010). Because we cannot establish a strong form-function relationship in extant species with the limited histological data gathered thus far, we also cannot yet make any inferences regarding flight style in this key taxon using secondary cartilage at this time. However, we notice that the secondary cartilage of Confuciusornis more closely resembles that of the sparrow and the swift than that of the dove. Moreover, based on the data of Ponton et al. (2007) and Mitchell et al. (2017), the absence of secondary osteons in the ramus of the furcula would suggest that Confuciusornis was not a soaring bird. This is consistent with morphological data (Hou et al., 1999) which does not suggest that this stem bird was capable of soaring over long distances.
We provide here the first report of furcular secondary cartilage in a fossil bird, but it is highly probable that secondary cartilage was present on the furculae of many other stem avians and more derived ornithuromorphs because the ability to form secondary cartilage within joints on dermal bones was already present in non-avian dinosaurs before the origin of birds (Bailleul et al., 2012). We may even expect that non-volant non-avian dinosaurs also had some secondary cartilage on their furcula. Indeed, the formation of secondary cartilage may simply require compressive forces including those not generated by flight (e.g., in non-volant theropod dinosaurs); however, we may expect drastic differences in the amount of secondary cartilage, the type of secondary cartilage, or the joint type between volant and non-volant taxa. Only additional data will shed light on this possibility.
5 Conclusions and perspectives
Establishing clear form-function relationships in living animals that shed light on the paleobiology of extinct organisms is an extremely difficult task. Further complicating inferences, the flight apparatus of each stem avian lineage is morphologically distinct from each other and that of extant birds. This explains why the early evolution of avian flight is still so controversial and poorly understood. Both general histology and joint histology are particularly powerful tools for making functional inferences because skeletal tissues detect and are reactive to biomechanical stimuli.Our preliminary data demonstrates histological differences in both the furcular secondary cartilage and in the primary cartilage on the coracoid in the three studied living birds, which utilize different flight styles. However, additional data must be collected to investigate if a clear form-function relationship exists. In the future, the calcified parts of these cartilages should be specifically targeted and measured because they have the greatest potential to enter the fossil record and thus be useful for comparison with extinct taxa. However, we cannot forget the common mechanism of post-mortem soft-tissue mineralization seen in the Jehol Biota, which may be full of yet undiscovered fossilized joint tissues like originally unmineralized hyaline cartilage (e.g., see Jiang et al., 2017).
The possibility of inferring quantitative joint movement based on secondary cartilage morphology (or quantity) will be a difficult endeavor from a methodological perspective. Recent data involving the paralysis of chick embryos showed that embryonic muscle contraction is required for the development of normal furcula morphology and suggested that there may be a threshold of embryonic motility above which additional movement does not promote increased growth, but below which growth is impaired (Pollard et al., 2017).
Similarly, we should keep in mind the possibility that secondary cartilage (postnatal secondary cartilage on adult birds furculae, not embryonic secondary cartilage) may simply require a threshold of joint movement (or mechanical stimuli) to be maintained, and therefore it may not show drastic differences between flight styles if the stimulus threshold is low. Hall (1986) showed that furcular secondary cartilage did arise in paralyzed chick embryos due to small contractions of the amnion, and that the threshold of movement for the initiation and maintenance of secondary cartilage in embryos is lower for the clavicle than for the craniofacial membrane bones. The thresholds may even be different between embryos and adults, and much more research is necessary to fully understand this interesting issue and a greater understanding of the histology of the avian flight apparatus is required, not simply at the furcula-coracoid joint but also in all other pectoral joints and in non-volant non-avian dinosaurs.
In the future, there is a good chance that even if distinct flight categories cannot be discriminated by histology, we may at least be able to histologically discriminate ‘active flight’ from ‘passive flight’ (or volant versus flightless). By combining existing data from morphometric analyses, aerodynamic models, and new histological data, there is hope that considerable light will soon be shed on the evolution of flight in early birds, including in the iconic basal pygostylian Confuciusornis.
Acknowledgements
We thank Zhou Zhonghe for fruitful discussions and encouragements. We thank Zhang Shukang for sectioning the furcula of Confuciusornis; Zhang Limin for facilitating lab access; and Ying Pengfei for CT scanning at IVPP. We also thank the Beijing Wildlife Rescue Center for providing specimens and two reviewers for their useful comments. This research was supported by the National Natural Science Foundation of China Grant 41688103. AMB also thanks CAS-PIFI for support (Chinese Academy of Sciences-President’s International Fellowship Initiative).参考文献 原文顺序
文献年度倒序
文中引用次数倒序
被引期刊影响因子
DOIURL [本文引用: 3]
DOIURL [本文引用: 1]
DOIURL [本文引用: 3]
DOIURL [本文引用: 4]
[本文引用: 2]
[本文引用: 9]
[本文引用: 1]
DOIURL [本文引用: 2]
DOIURL [本文引用: 1]

The evolution of birds from theropod dinosaurs was one of the great evolutionary transitions in the history of life [122]. The macroevolutionary tempo and mode of this transition is poorly studied, which is surprising because it may offer key insight into major questions in evolutionary biology, particularly whether the origins of evolutionary novelties or new ecological opportunities are associated with unusually elevated "bursts" of evolution [23, 24]. We present a comprehensive phylogeny placing birds within the context of theropod evolution and quantify rates of morphological evolution and changes in overall morphological disparity across the dinosaur-bird transition. Birds evolved significantly faster than other theropods, but they are indistinguishable from their closest relatives in morphospace. Our results demonstrate that the rise of birds was a complex process: birds are a continuum of millions of years of theropod evolution, and there was no great jump between nonbirds and birds in morphospace, but once the avian body plan was gradually assembled, birds experienced an early burst of rapid anatomical evolution. This suggests that high rates of morphological evolution after the development of a novel body plan may be a common feature of macroevolution, as first hypothesized by G.G. Simpson more than 60 years ago [25].
DOIURL [本文引用: 1]
DOIPMID [本文引用: 1]

Medullary bone (MB) is an estrogen-dependent, sex-specific tissue produced by female birds during lay and inferred to be present in extinct avemetatarsalians (bird-line archosaurs). Although preliminary studies suggest that MB can be deposited within most skeletal elements, these are restricted to commercial layers or hormonally treated male pigeons, which are poor analogues for wild birds. By contrast, studies in wild bird species noted the presence of MB almost exclusively within limb bones, spurring the misconception that MB deposition is largely restricted to these regions. These disparate claims have cast doubt on the nature of MB-like tissues observed in some extinct avemetatarsalians because of their "unusual" anatomical locations. Furthermore, previous work reported that MB deposition is related to blood supply and pneumatization patterns, yet these hypotheses have not been tested widely in birds. To document the skeletal distribution of MB across Neornithes, reassess previous hypotheses pertaining to its deposition/distribution patterns, and refine the set of criteria by which to evaluate the nature of purported MB tissue in extinct avemetatarsalians, we CT-scanned skeletons of 40 female birds (38 species) that died during the egg-laying cycle, recorded presence or absence of MB in 19 skeletal regions, and assessed pneumatization of stylopods. Selected elements were destructively analyzed to ascertain the chemical and histological nature of observed endosteal bone tissues in contentious skeletal regions.Although its skeletal distribution varies interspecifically, we find MB to be a systemic tissue that can be deposited within virtually all skeletal regions, including cranial elements. We also provide evidence that the deposition of MB is dictated by skeletal distribution patterns of both pneumaticity and bone marrow; two factors linked to ecology (body size, foraging). Hence, skeletal distribution of MB can be extensive in small-bodied and diving birds, but more restricted in large-bodied species or efficient flyers.Previously outlined anatomical locations of purported MB in extinct taxa are invalid criticisms against their potential reproductive nature. Moreover, the proposed homology of lung tissues between birds and some extinct avemetatarsalians permit us to derive a series of location-based predictions that can be used to critically evaluate MB-like tissues in fossil specimens.
[本文引用: 1]
[本文引用: 1]
DOIURL [本文引用: 1]
DOIURL [本文引用: 1]
DOIURL [本文引用: 1]
DOIURL [本文引用: 5]
DOIURL [本文引用: 1]
DOIURL [本文引用: 1]
DOIURL [本文引用: 3]
[本文引用: 3]
[本文引用: 1]
DOIURL [本文引用: 2]
PMID [本文引用: 4]
DOIURL [本文引用: 4]
[本文引用: 3]
PMID [本文引用: 3]

Clavicles (collar bones) are variably present in mammals. Furculae (wishbones)--which may or may not be homologous with clavicles--are variably present and/or fused in birds and present in theropod dinosaurs. In this overview the development of clavicles and furculae is discussed with special attention to modes of skeletogenesis (whether intramembranous or endochondral), numbers of centres of ossification (one in chick furculae; two in murine clavicles), presence of cartilage (primary in clavicles, secondary in furculae), evidence from experimental analysis and from mutations for dependence of both clavicular and furcular growth on mechanical stimulation, and syndromes and mutations affecting clavicular development leading to both under and over development. J. Exp. Zool. 289:153-161, 2001.Copyright 2001 Wiley-Liss, Inc.
[本文引用: 1]
DOIURL [本文引用: 4]
DOIURL [本文引用: 1]
DOIURL [本文引用: 1]
DOIURL [本文引用: 1]
DOIURL [本文引用: 1]
[本文引用: 2]
DOIURL [本文引用: 2]
PMID [本文引用: 1]

The pectoral girdle articulates the forelimb with the axial skeleton in all vertebrates with paired anterior appendages. The structure of the pectoral girdle and its position along the axial skeleton has changed significantly during vertebrate evolution. These morphological changes have been well described, but there is little comparative embryology to indicate how these changes may have occurred. It is equally obscure how the muscles that connect the head with the pectoral girdle have maintained appropriate attachments even though these 2 structures have become separated. Here I review the changes in the pectoral girdle across different vertebrate taxa, indicating, where known, the developmental mechanisms underlying these changes. I also suggest how the muscular connections between the head and pectoral girdle have been maintained between these once adjacent bones, displaced during vertebrate evolution.
DOIURL [本文引用: 2]
PMID [本文引用: 2]
DOIURL [本文引用: 5]
DOIURL [本文引用: 1]
DOIURL [本文引用: 3]
DOIURL [本文引用: 1]
[本文引用: 3]
DOIURL [本文引用: 1]
DOIURL [本文引用: 1]
DOIURL [本文引用: 2]
DOIURL [本文引用: 2]
PMID [本文引用: 1]

During postnatal development of the pigeon, a large portion of the skeleton becomes pneumatized, displacing the hemopoietic bone marrow. The consequences of pneumatization on distribution and quantity of bone marrow as well as the availability of other sites for hemopoiesis have been investigated. Hemopoietic marrow of differently aged pigeons divided into five groups from 1 week posthatching (p.h.) up to 6 months p.h. was labeled with Fe-59 and examined by serial whole-body sections. Autoradiography and morphometry as well as scintillation counts of single bones and organs were also carried out. No sign of a reactivation of embryonic sites of erythropoiesis was found. Bone marrow weight and its proportion of whole-body weight increased during the first 4 weeks p.h. from 0.54% to 2.44% and decreased in the following months to about 1.0%. The developing bone marrow showed a progressive distribution during the first months of life, eventually being distributed proportionally over the entire skeleton, except for the skull. At the age of 6 months p.h. bone marrow had been displaced, its volume decreasing in correlation to increasing pneumaticity and conversion to fatty marrow. This generates the characteristic pattern of bone marrow distribution in adult pigeons, which shows hemopoietic bone marrow in ulna, radius, femur, tibiotarsus, scapula, furcula, and the caudal vertebrae.
[本文引用: 2]
DOIURL [本文引用: 3]
DOIURL [本文引用: 1]
DOIURL [本文引用: 4]
DOIURL [本文引用: 3]
DOIURL [本文引用: 2]
DOIURL [本文引用: 2]
[本文引用: 2]
[本文引用: 4]
[本文引用: 1]
DOIURL [本文引用: 1]