全文HTML
--> --> -->随着微加工工艺的发展, 依靠一些纳米结构可以实现亚波长尺寸下的波长选择功能, 例如: 金属纳米结构通过等离激元共振可以实现亚波长滤光[2], 半导体纳米线依靠能带工程依靠禁带渐变可以实现波长分析[3,4]; 与此同时, 纳米线作为光检测器具有广阔的应用前景, 具有以形貌和几何尺寸调节吸收的出色能力[5-9]. 与等离激元相比, 硅纳米线(silicon nanowires, SiNW)具有的主要优势在于, 由于金属和掺杂半导体中的自由电子密度大, 等离子激元通常与高损耗相关, 在SiNW中未观察到这种高损耗[10,11]. 目前使用较多的方法如利用径向结方式制备光电探测器, 多采用掺杂、涂覆、生长等方式在SiNW外再增加一层以形成径向PN结[12-14], 或采用纳米线阵列[6,12]及其他结构, 如谐振器、异质结等[15-19], 大多需要较复杂的工艺. 而Sumetsky[20]发现, 小角度介电锥可以用作完全限制光的光学微谐振器. 通常沿圆锥形表面传播的射线在圆锥体的较窄侧上是有界的, 而在其较宽的侧面上是无界的, 因此任何不平行于圆锥体轴发射的射线最终都会移动到无穷远处. 但是, 对于小角度的介电锥, 由于回音壁模式(whispering gallery modes, WGM)效应, 可以约束光束在锥内的位置. 根据介电锥的这种特性, 利用锥形SiNW制备光电探测器, 由于其自身结构能够将不同波长的光约束在不同位置, 已经具备了分光能力, 而SiNW自身也具有良好的光吸收能力[21-23]. 因此我们认为利用锥形SiNW自身的结构和性质就能够直接制备无滤波片(filter-free)的色彩分辨探测器件, 并进行了仿真验证, 展示了纳米线自身腔模式色彩分辨能力以及线上集成宽光谱探测的潜力.
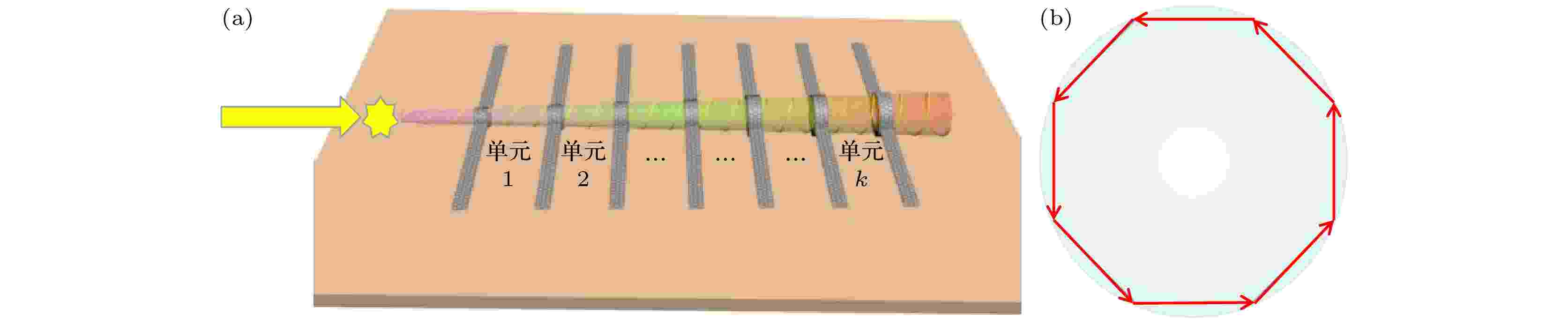
Figure1. (a) Schematic diagram of the Si nano-cone device; (b) the whispering gallery mode.


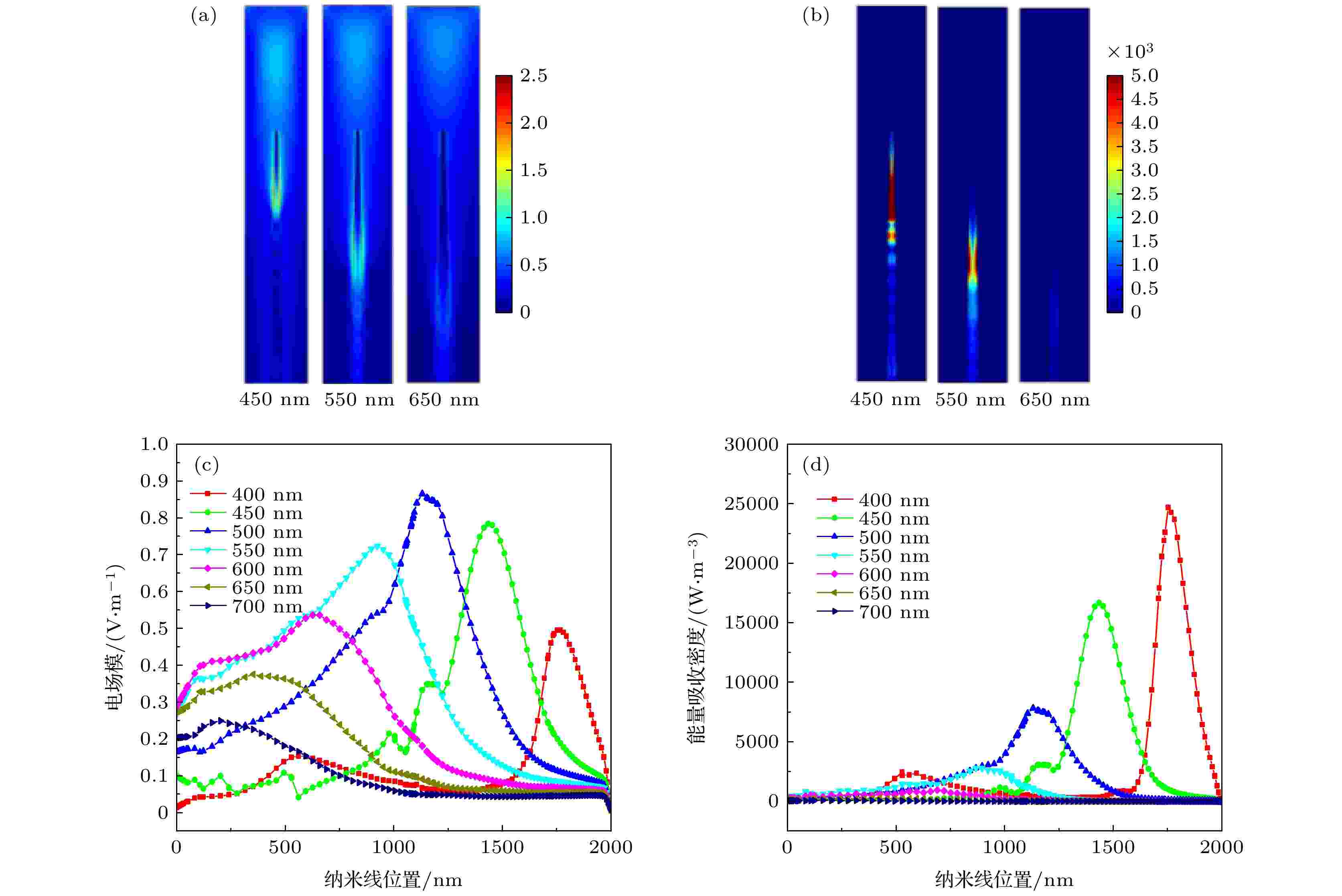
Figure2. The simulation results of the light field distribution (a) and the absorption (b) with the wavelength of 450, 550 and 650 nm, respectively. The light field distribution (c) and absorption (d) of the typical incident wavelength along the axial of Si nano-cone.
器件1/nm | 器件2/nm | 器件3/nm | |||||||||
r1 = 20 | r2 = 80 | L = 2000 | r1 = 20 | r2 = 100 | L = 2000 | r1 = 20 | r2 = 80 | L = 2500 | |||
500 nm入射光吸收峰值直径/nm | 92 | 94 | 90 | ||||||||
550 nm入射光吸收峰值直径/nm | 106 | 107 | 107 | ||||||||
两峰值距离/nm | 234 | 172 | 325 |
表1在具有不同几何结构的器件中, 不同波长的入射光吸收最大值位置比较
Table1.Comparisons of the max absorption position in different size devices with different geometry.
考虑到实际器件制备时需要制备在衬底上. 因此也考虑了增加衬底情况, 加入二氧化硅衬底后, 首先验证了入射光条件不变的情况并与无衬底器件进行比较. 在加入衬底后, 对于相同入射波长(550 nm), 两个器件的吸收峰值位置并没有发生改变, 仍处于相同位置, 峰值处截面直径约为107 nm. 但是衬底器件的吸收要好于无衬底器件, 如表2所列, 衬底器件的光场吸收约是无衬底器件的1.08倍, 能量吸收约是1.16倍, 证明加上衬底后, 纳米线对光的吸收和转化效率都更高了, 这可能是由于锥形纳米线与衬底之间的间隙反射了部分光入射纳米线, 这一部分超过了从衬底泄露的能量. 之后改变了入射光的偏振角度, 使入射光的偏振方向平行于衬底并与纳米线中心轴线垂直. 我们发现, 带有衬底的器件, 当入射光偏振方向为水平方向时, 光场和能量吸收约是无衬底器件的1.14和1.30倍, 提高量相比垂直偏振增加了1倍, 这可能是由于偏振方向水平时, 衬底的光泄露减弱了, 从而使得其增量增加.
入射光波长550 nm | 无衬底器件 | 衬底器件(入 射光偏振垂 直衬底) | 衬底器件(入 射光偏振平 行于衬底) |
与无衬底器件 比值 (光场) | 1 | 1.08 | 1.14 |
与无衬底器件 比值 (吸收) | 1 | 1.16 | 1.30 |
表2无衬底器件与有衬底器件对入射光能量吸收积分对比
Table2.The total absorption of the nano-cone devices with or without substrate.
同时也考虑了器件是否能应对不同角度的入射光, 因此通过旋转纳米锥器件来模拟入射光从不同角度入射的情况. 如图3以500 nm入射光为例, 当入射角度较小时, 无论对光场分布还是吸收分布, 影响都相对较小. 当角度逐渐增大时, 其对于光场分布的影响逐渐明显, 但是对于吸收来说, 纳米线的吸收中心区域并没有发生太大的变化, 对应波长的吸收位置在轴线上的相对位置几乎没有发生改变, 因此对于纳米锥器件的分辨率和精度影响较小. 但当入射角度过大如达到30o的入射角度时, 可以明显地发现, 器件会几乎失去波长选择的能力. 因此当实际制备及使用器件时, 要控制入射光以一个相对较小的角度耦合入射以保证器件的正常工作.
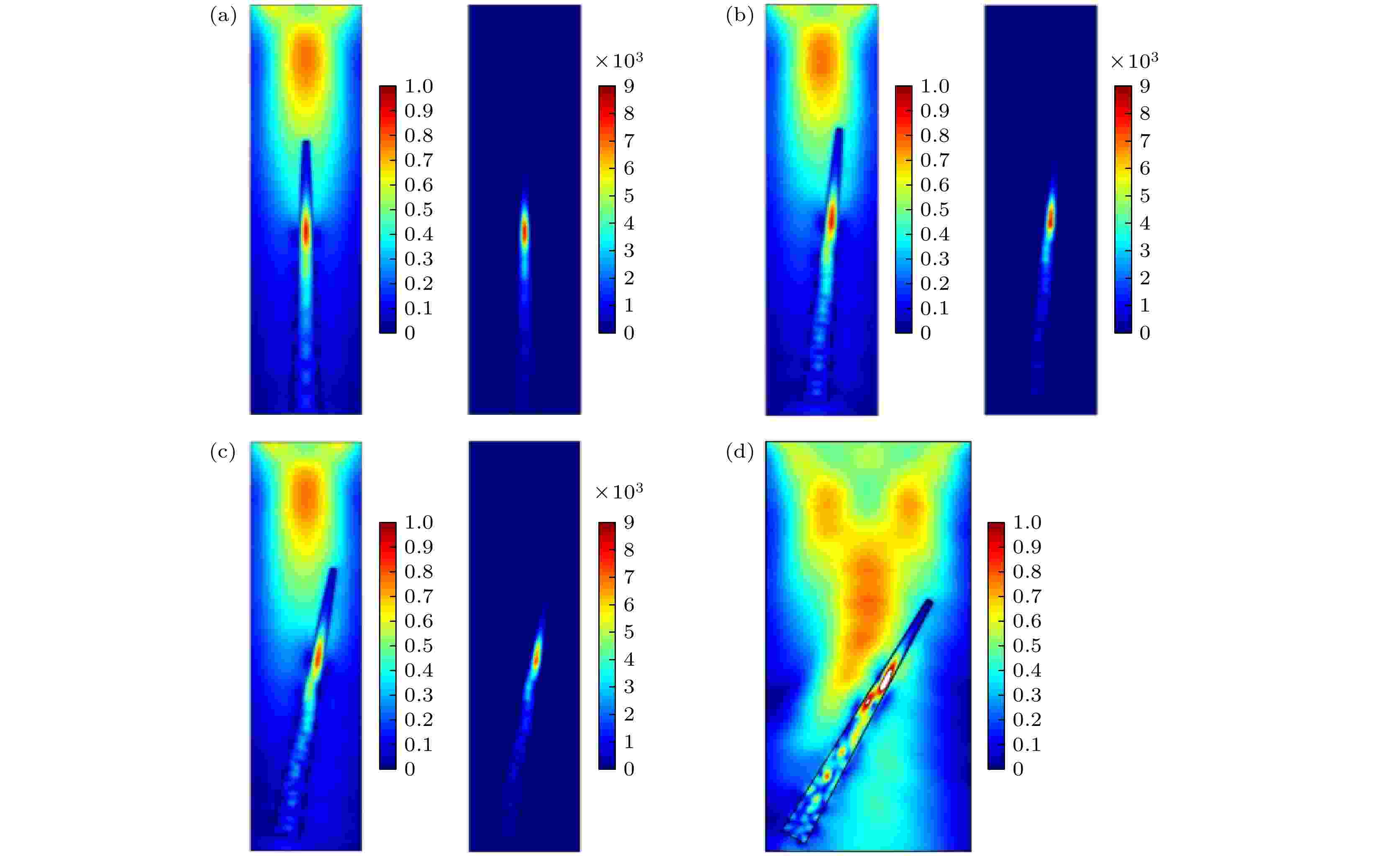
Figure3. The simulation results of the light field distributions (left) and the absorption (right) with wavelength of 500 nm when the incident angle is (a) 0o, (b) 5o (c) 10o, and (d) 30o.
改变入射光角度除了会改变入射光分布以外, 也会影响锥形硅器件对光的吸收. 图4探究了不同波长的入射光在不同入射角度的情况下其吸收强度密度的变化. 从图4可以观察到, 不同波长的入射光在不同角度下入射时, 其吸收强度的变化趋势基本完全相同. 以500 nm入射光为例, 当入射角度在10o以下时, 不同的入射角度对吸收强度的影响很小, 而一旦超过10o, 其吸收强度会发生较大的变化. 尽管当入射角度增加时, 其吸收强度会增加, 但是同时其光场分布也会发生改变, 锥形硅也失去了波长选择的能力. 而锥形硅器件作为具有色彩分辨探测能力的器件, 最核心和基本的能力就是其波长选择能力, 因此仍要保证入射光以10o以下小角度入射纳米锥器件.
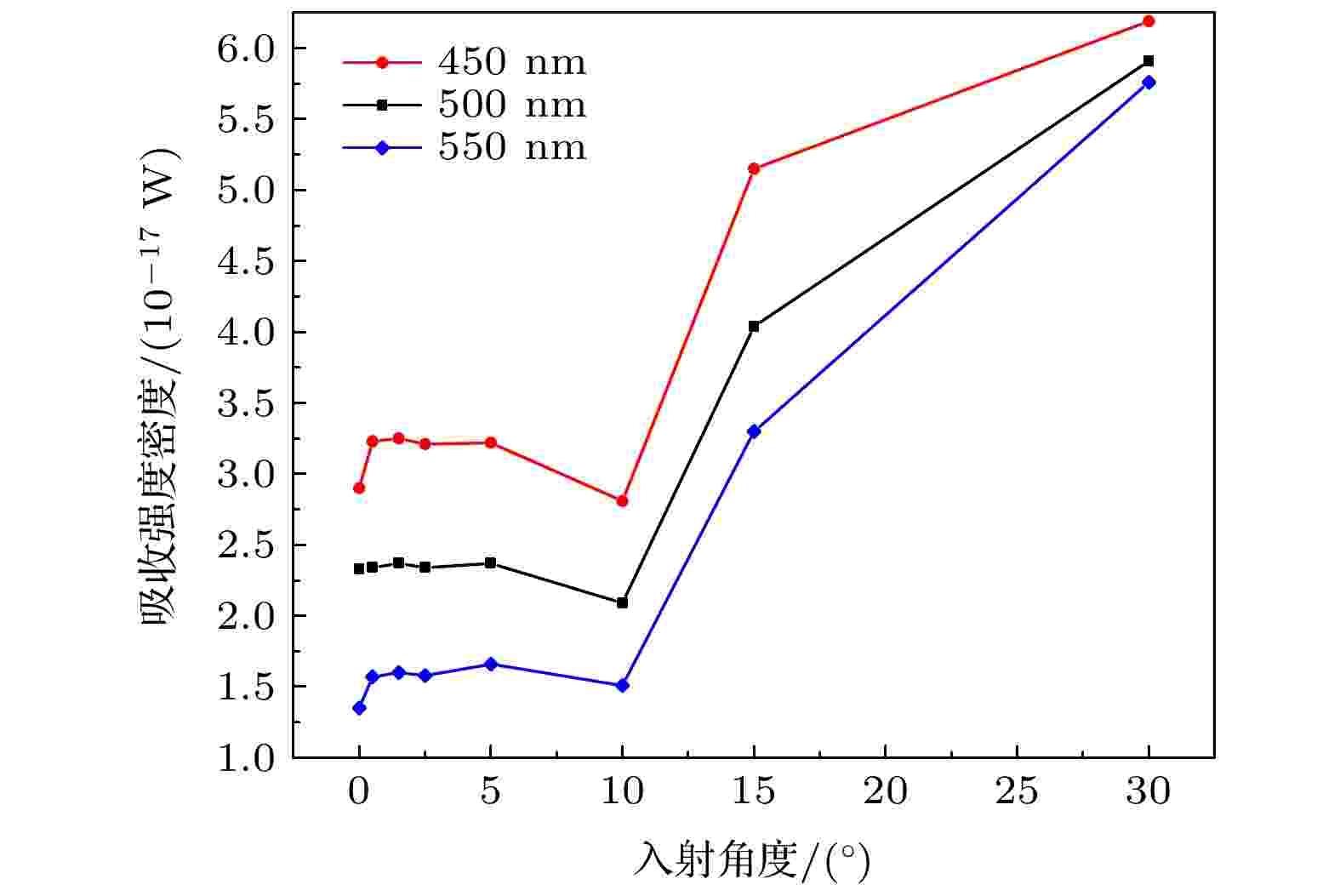
Figure4. The energy absorption density of typical incident light with different incident angles.
单根纳米线做光电器件时, 使用金属电极往往会引起比较大的损耗, 因此模拟了石墨烯做电极的情况, 在尺寸为r1 = 20 nm/r2 = 80 nm/L = 2000 nm的锥形硅纳米线器件上, 距顶端分别为1600和200 nm处添加20 nm宽的石墨烯电极. 首先在光吸收的位置上, 增加石墨烯电极后位置并没有发生改变. 在能量吸收方面, 计算了所有波长的入射光在纳米线内部的能量吸收. 发现当且仅当吸收位置在石墨烯电极附近时, 能量吸收会略小于无石墨烯电极的能量吸收, 约0.05%(如图5). 那么即使制作较密集的石墨烯电极, 总吸收损耗影响也较小. 并且石墨烯电极对光吸收位置即器件分辨率等也没有影响, 可以认为石墨烯是合适的电极材料.
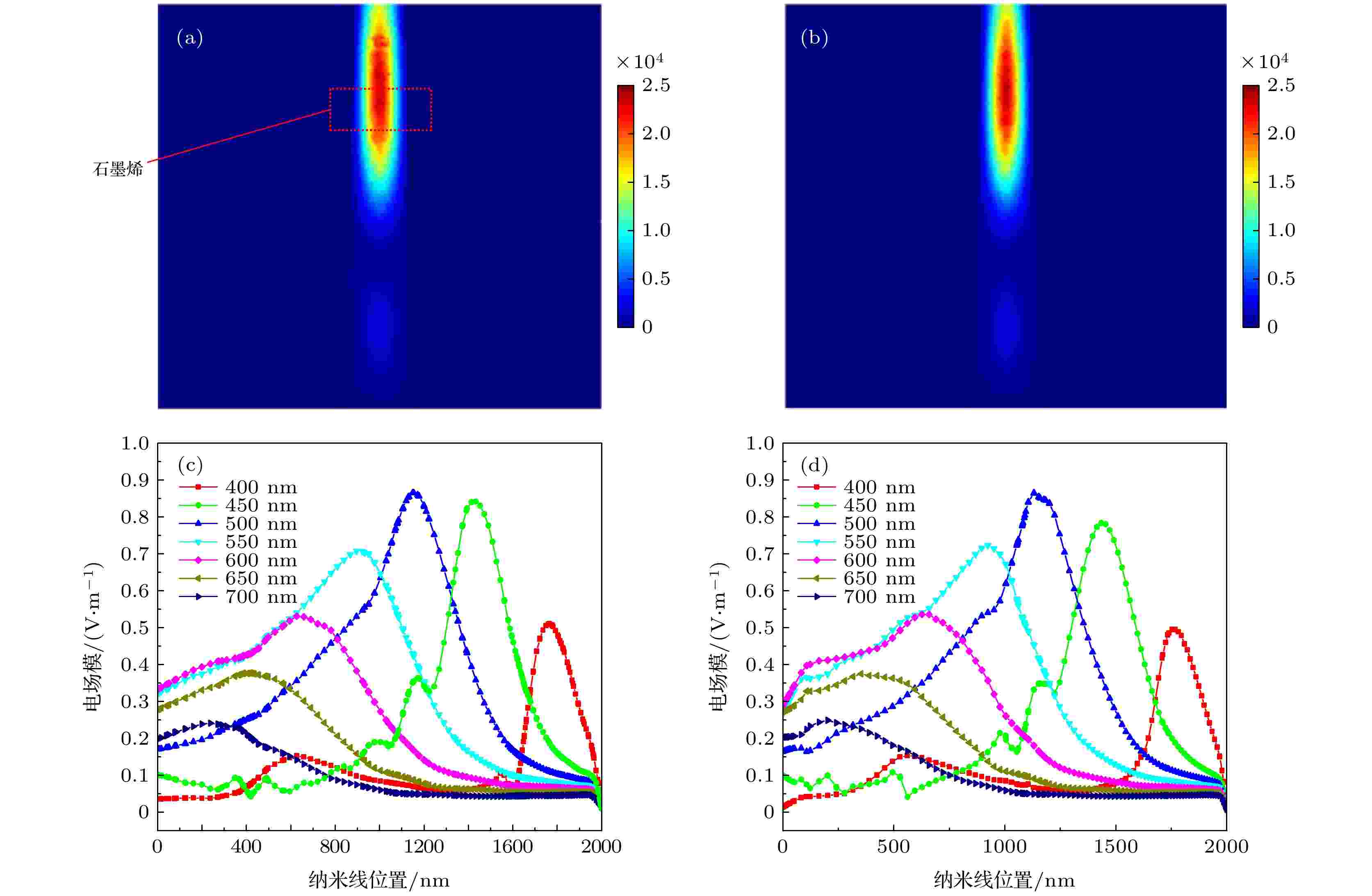
Figure5. (a) Comparisons of light field distribution between devices with (a) and without (b) graphene electrodes under incident light of with 420 nm. Light field distribution with typical incident light along the axis of nano-cone devices with (c) and without (d) graphene electrodes.
当制备获得实际器件后, 该锥形纳米线器件可以利用归一化吸收谱与实际测量得到的电流值Ii (Ii表示在不同位置实际测量光电流), 计算重构入射光谱[24,25]. 归一化吸收谱






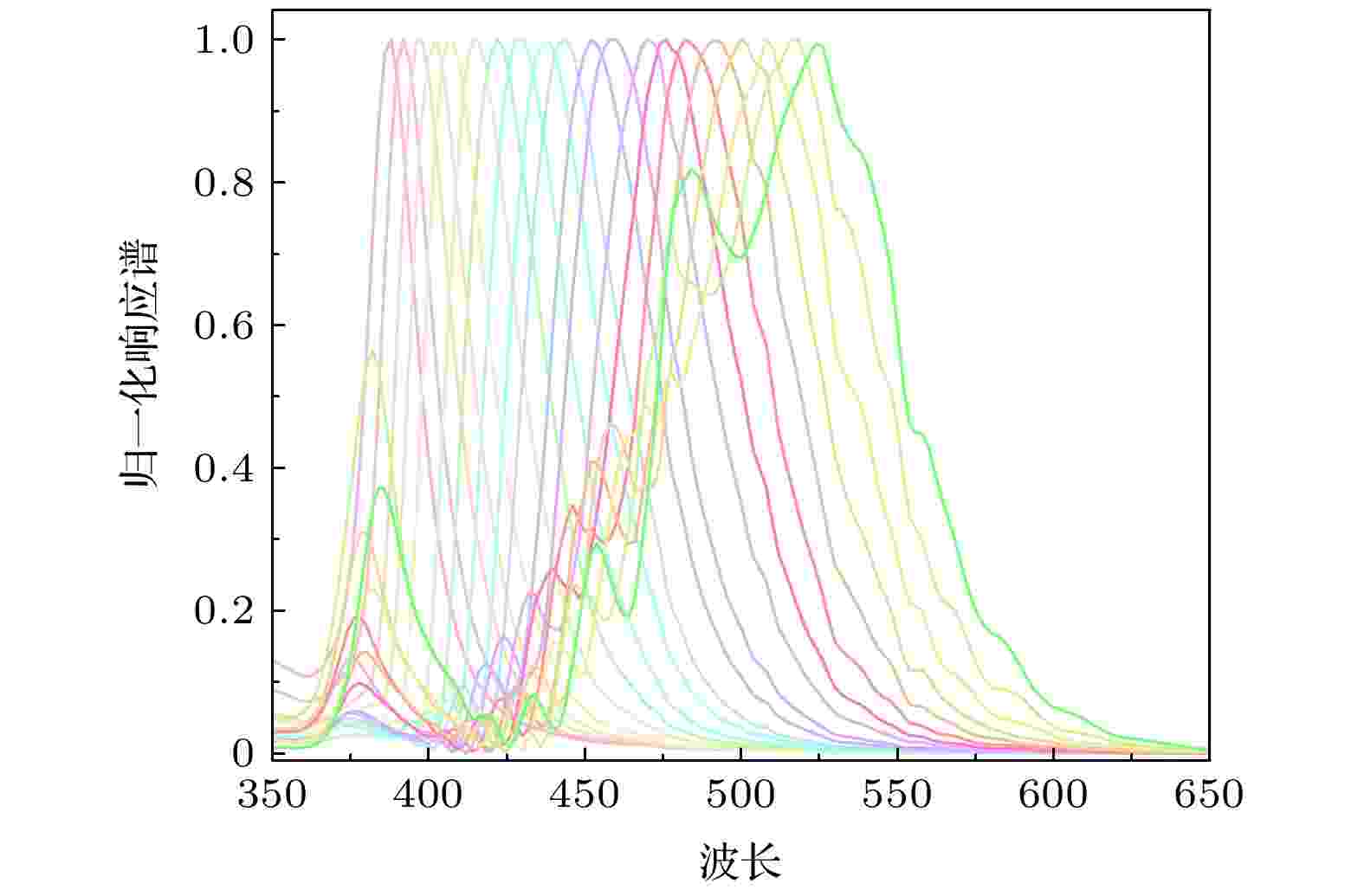
Figure6. The simulation results of normalized response spectrum of a nano-cone device.
不仅是硅, 事实上其他材料也可以通过锥形结构实现自结构的光电探测器. 为了探究不同材料带来的影响, 仿真计算了锥形锗纳米线的情况. 首先, 由于Ge的n/k值与Si相差较大且禁带宽度更窄, 因此我们猜想Ge能够吸收的光波长更长, 并依此确定模型的基本尺寸(r1 = 30 nm/r2 = 130 nm/L = 2000 nm). 仿真结果证明了我们的猜想, 该尺寸的Ge纳米锥在靠近尖端的位置即可吸收800 nm的入射光, 并且能够吸收波长更长的红外光, 其光场分布曲线与吸收曲线与Si纳米线相似, 主要区别在于吸收波段不同. 这证明除了改变器件尺寸以外, 还可以通过改变材料来覆盖不同波段的光, 以更贴近我们的实际需求.