全文HTML
--> --> -->采用外腔方式制备的面发射激光器结构在实现高功率输出的同时, 还能保证激光具有高的光束质量与良好的光斑形貌, 解决上述边发射半导体激光器及VCSEL激光器在实际应用中遇到的问题[6]. 同时, 由于这种结构拥有一个灵活的外腔, 可以在腔内放置频率转换、波长调谐或锁模功能的各种光学元件[7-9], 结合半导体材料宽的激光光谱范围, 这种外腔式的面发射激光器结构具有极宽的光谱覆盖范围, 并可实现诸如腔内锁模、脉冲调制等功能. 因此, 垂直外腔面发射半导体激光器(vertical external cavity surface emitting laser, VECSEL)技术一经出现, 即获得了人们的广泛关注[10]. 采用外部光注入的方式使VECSEL产生激光激射被称为光抽运VECSEL激光器, 这种结构所用的增益芯片内部各个材料层无需掺杂, 避免了半导体材料掺杂带来的光吸收等问题[11], 但是VECSEL的关键在于要有高增益的内部发光区结构.
Kuznetsov等[6]在1997年首次系统介绍了光抽运VECSEL的发光区量子阱设计方法, 实现了980 nm VECSEL激光输出功率超过0.5 W. 随后, Yoo等[12]通过在多量子阱势垒之间插入高势垒非吸收层的方法, 有效减小饱和载流子寿命, 将光抽运VECSEL功率提升到5 W. 2008年, Rudin等[13]通过InGaAs两侧增加GaAsP势垒的方法, 采用7个量子阱发光区, 实现–20 ℃下VECSEL激光功率达23 W. 近几年随着金刚石热沉散热技术[14]、双面散热[15]等技术的提出, 以及腔内多增益芯片抽运方案[16]的出现, VECSEL性能水平不断得到提升. 目前, 国内的科研机构也在VECSEL方面取得了一些成果, 例如, 重庆师范大学利用后端抽运方式, 获得了调谐范围为4 nm的65 mW的绿光输出[17], 以及搭建共振Y形腔, 实现最大功率达到293 mW的双波长输出[18]. 虽然如此, 关于如何系统设计VECSEL增益区量子阱结构及势垒结构的研究报道仍然很少, 而实现一个高增益的发光区是实现VECSEL高性能工作的前提.
本文从VECSEL增益芯片的增益区设计出发, 对增益区量子阱的材料组分、厚度变化引起的增益光谱差异进行对比分析, 并对不同载流子浓度下量子阱的增益系数以及其温漂特性进行深入分析, 确定合适的量子阱材料参数. 在此基础上, 对不同构型的势垒限制结构引起的量子阱增益谱差异进行对比, 得到合适的增益区势垒结构. 对MOCVD外延生长的VECSEL晶圆进行了腔模位置的验证, 所制备的VECSEL实现了激光输出功率达到9.82 W, 光光功率曲线斜率效率达到35.5%, 并且在功率最高点功率曲线并未饱和. 结果发现, 采用不同反射率的外腔镜, VECSEL系统的激光波长随抽运功率的漂移速度有明显差异, 说明采用不同反射镜会导致VECSEL增益芯片内部热效应有差异, 这也是不同反射镜组成的VECSEL系统激光输出功率有较大差异的原因.
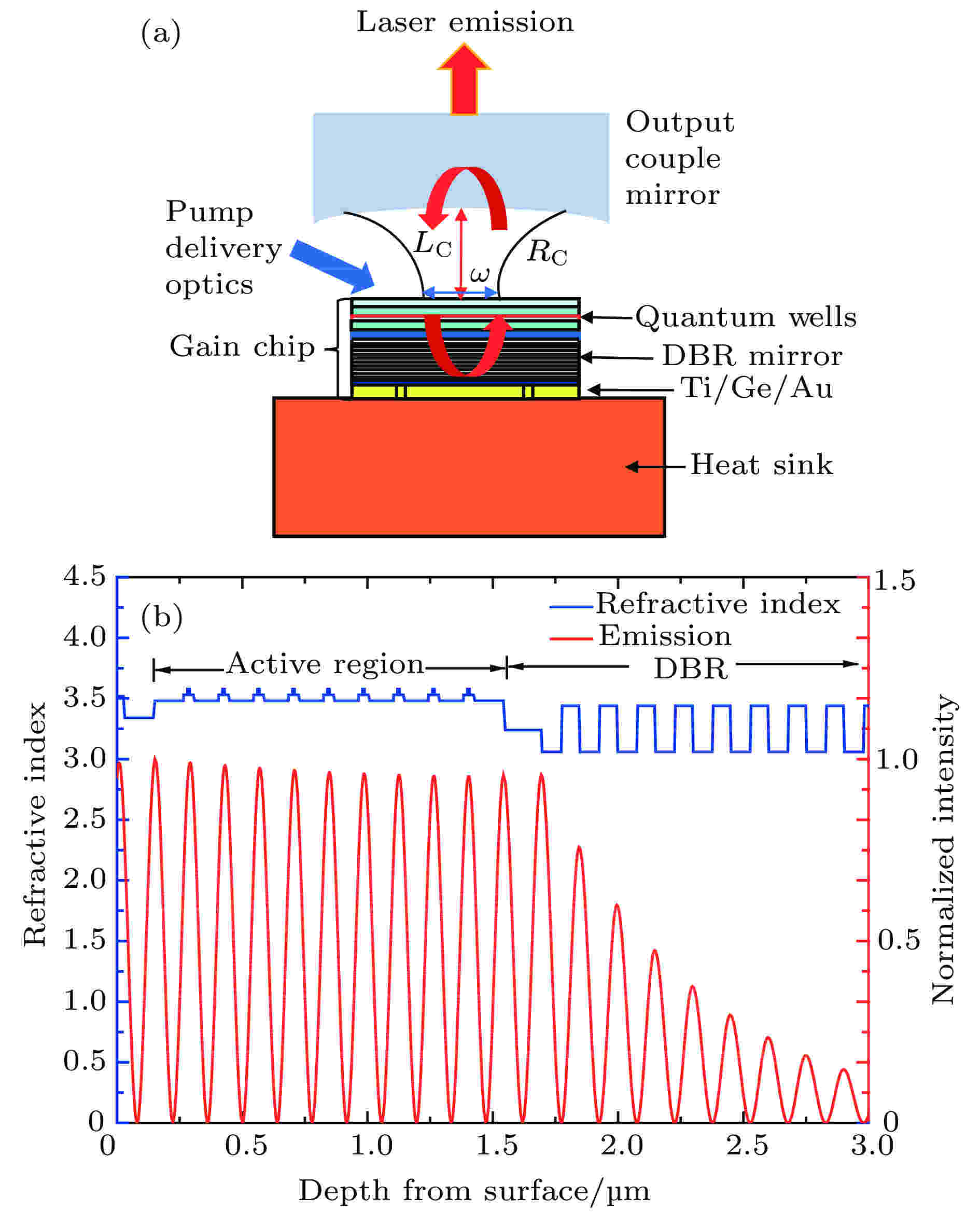
Figure1. (a) Schematic diagram of the VECSEL system; (b) distributions of the refractive index of each layer and the optical field within the gain chip.
InGaAs量子阱是980 nm波段最常使用的量子阱结构, 这是因为InGaAs量子阱带来的压应变效应可以使得价带的轻重空穴带有效分离, 实现低的透明载流子密度和高的材料增益. 因此, 本研究VECSEL增益区采用InGaAs量子阱, 并首先开展量子阱的组分及厚度设计. 在模拟中采用Al0.06Ga0.94As作为势垒层材料. 使用PICS3 D软件中基于上述增益计算方法的理论模型, 对不同组分及厚度InGaAs量子阱的能带波长进行筛选.
图2(a)为计算的InGaAs量子阱能级分立后发光波长分别在970, 975以及980 nm的量子阱In组分、量子阱厚度关系. 可以看出, 这3个出光波长所对应的3条量子阱组分与厚度关系的变化趋势相一致. 对于同一种发光波长, 当量子阱厚度增加时, 需要降低In组分的含量以保持发光波长不变. 当量子阱的厚度小于6 nm时, In组分的含量随量子阱厚度的增加快速降低; 而当量子阱厚度大于6 nm后, 该趋势较为平缓. 这是因为量子阱厚度较大时, 材料的量子效应弱化, 能级分立效应不明显, 导致能带的变化主要依靠In组分来调节. 对比3种不同波长所对应的量子阱In组分和厚度曲线, 可以看出, 对于相同厚度的量子阱, 增加量子阱In组分可以得到更长的出光波长.
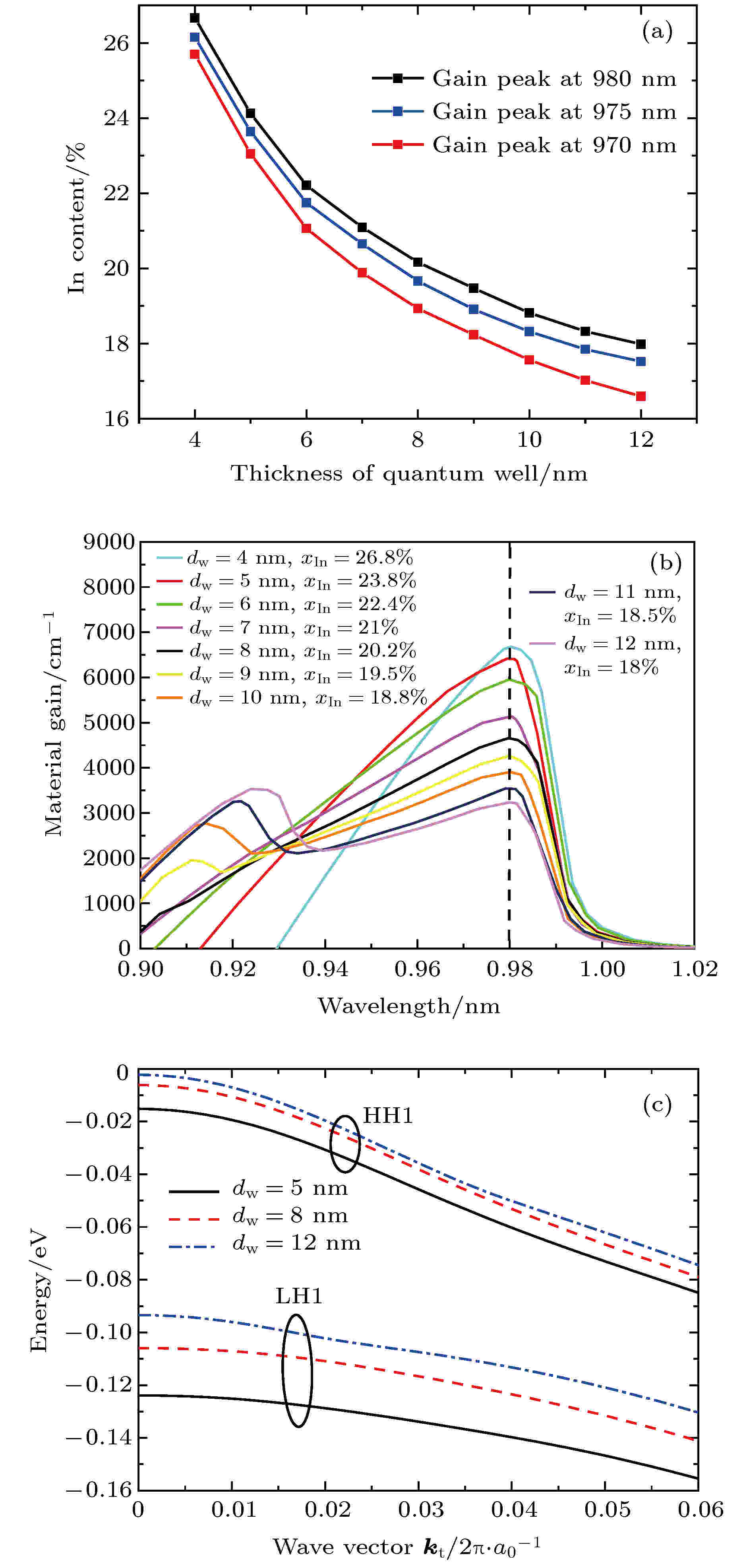
Figure2. (a) Relationships between the In content and thickness of quantum wells when its emitting wavelength is 970, 975, 980 nm; (b) the gain spectra of different quantum wells with the same gain peak wavelength of 980 nm; (c) the valence subband structures of InGaAs QWs corresponding to a wavelength of 980 nm (HH1, the first heavy hole subband; LH1, the first light hole subband.).
图2(b)为出光波长在980 nm的InGaAs量子阱增益谱曲线. 可以看出, 不同组分及厚度的InGaAs量子阱增益谱差异非常明显. 随着量子阱厚度的增加, 量子阱的增益谱峰值不断降低. 同时, 对于厚度超过9 nm的量子阱结构, 其增益谱左侧出现一个次级的增益峰. 这是因为厚度增加, 量子阱能级分立效应变弱, 第一子能级和第二子能级分立不足, 导致两个子能级同时发光. 而第二子能级对应的能带宽度要大于第一子能级, 因此, 出现的次级增益峰所对应的波长比主增益峰要短. 总体看来, 使用窄的量子阱厚度, 可以实现高的增益峰值, 然而增益谱的宽度也在不断缩小. 并且, 量子阱厚度由4 nm增加至5 nm时, 增益峰值相差不大, 但是量子阱的增益谱宽度明显增加. 增益谱宽度的增加, 伴随着纵模限制因子的增加, 将进一步地限制光场和载流子, 防止其泄露[21]. 此外, 当量子阱厚度从5 nm继续增加至6 nm时, 增益谱宽度没有特别明显的变化, 但是增益峰强度却在降低. 因此, 从增益谱看来, 采用5 nm的量子阱厚度是比较理想的选择.
量子阱的组成和厚度在保持增益峰值为980 nm的情况下被进行了调整, 以优化增益特性. 在图2(c)中, 自上往下依次是第一重空穴带(HH1)、第一轻空穴带(LH1). 选取5, 8, 12 nm厚的不同的In组成的InGaAs量子阱系列的计算价带子带被绘成平面内波长kt的函数. 随着QWs中In含量的增加, 压缩应变增大, 导致价带的态密度降低. 同时, 第一重空穴HH1的曲率随着In组成的增加而增大, 使得价带和导带的态密度更加匹配. 这些效应有望导致更高的差分增益和更低的透明载流子密度. 这更加确定了量子阱的厚度为5 nm较为理想的推论.
量子阱的增益谱可以看出量子阱工作时可提供的各波长增益情况, 而量子阱的峰值增益随载流子浓度的变化可以看出量子阱实际工作时的增益输出能力. 图3(a)为模拟的InGaAs量子阱峰值增益随量子阱内部载流子浓度的变化关系, 增益峰值波长在980 nm. 可以看出, 不同厚度的量子阱结构随载流子浓度的变化曲线具有明显差异. 在图3(a)中, 当量子阱的厚度从4 nm增加到12 nm时, 载流子密度明显降低. 这是因为为了保持设计的波长不变, 量子阱中的In成分相应地降低, 此时量子阱材料带隙的增加导致价带顶能态密度的减小, 进而导致载流子流密度减小[22]. 此外, 量子阱厚度大于9 nm时, 很低的载流子浓度就可以产生光增益, 然而随着载流子浓度的增加, 量子阱的增益曲线快速饱和. 随着量子阱厚度不断减小, 增益曲线的饱和值不断增加, 同时, 量子阱的微分增益dg/dn不断增加. 这说明随着量子阱厚度的降低, 量子阱在高的抽运功率下可以提供的增益更高, 并且单位载流子产生的光增益也更高. 从图3(a)可以看出, 5—6 nm的量子阱厚度可以在很大的载流子注入浓度范围下获取高的增益.
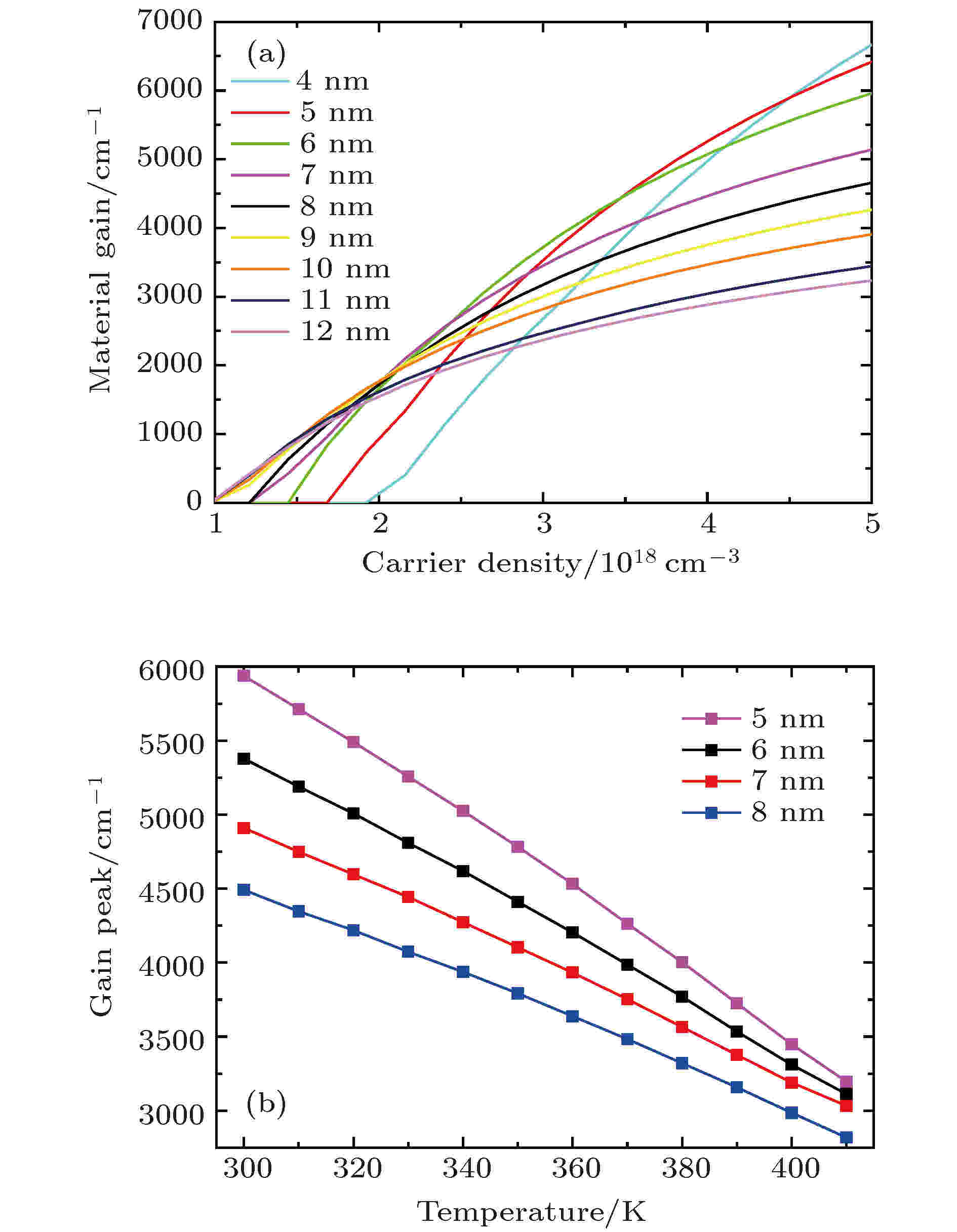
Figure3. (a) The change of gain peak with the carrier density within quantum wells when the gain peak wavelength is 980 nm; (b) the change of material gain with the operating temperature.
图3(b)为不同量子阱的峰值增益随工作温度的变化曲线. 随着工作温度的增加, 量子阱的峰值增益不断降低. 可以看出, 量子阱的厚度越小, 其峰值增益随工作温度的变化越明显, 也就是说, 在高温下量子阱的增益衰减就越明显. 这是因为随着工作温度的增加, 薄的量子阱厚度更容易产生注入载流子的泄露, 从而降低载流子利用效率. 然而从图3(b)的曲线可以看出, 即使薄的量子阱厚度具有更快的增益衰减速度, 在相同的工作温度下, 薄的量子阱仍然能提供更高的光增益.
根据上述对量子阱增益谱及增益-载流子浓度关系的分析, 可以确定量子阱的最佳厚度在5 nm左右. 上述设计是量子阱增益峰值位于980 nm的设计结果, 在实际器件工作时, 由于自产热效应, VECSEL的增益区往往工作在较高的工作温度. 为了保证工作时增益峰值波长与激光器的实际出光波长实现较好的匹配, 对量子阱的增益谱及增益峰值波长随工作温度的漂移情况进行了模拟, 如图4所示.
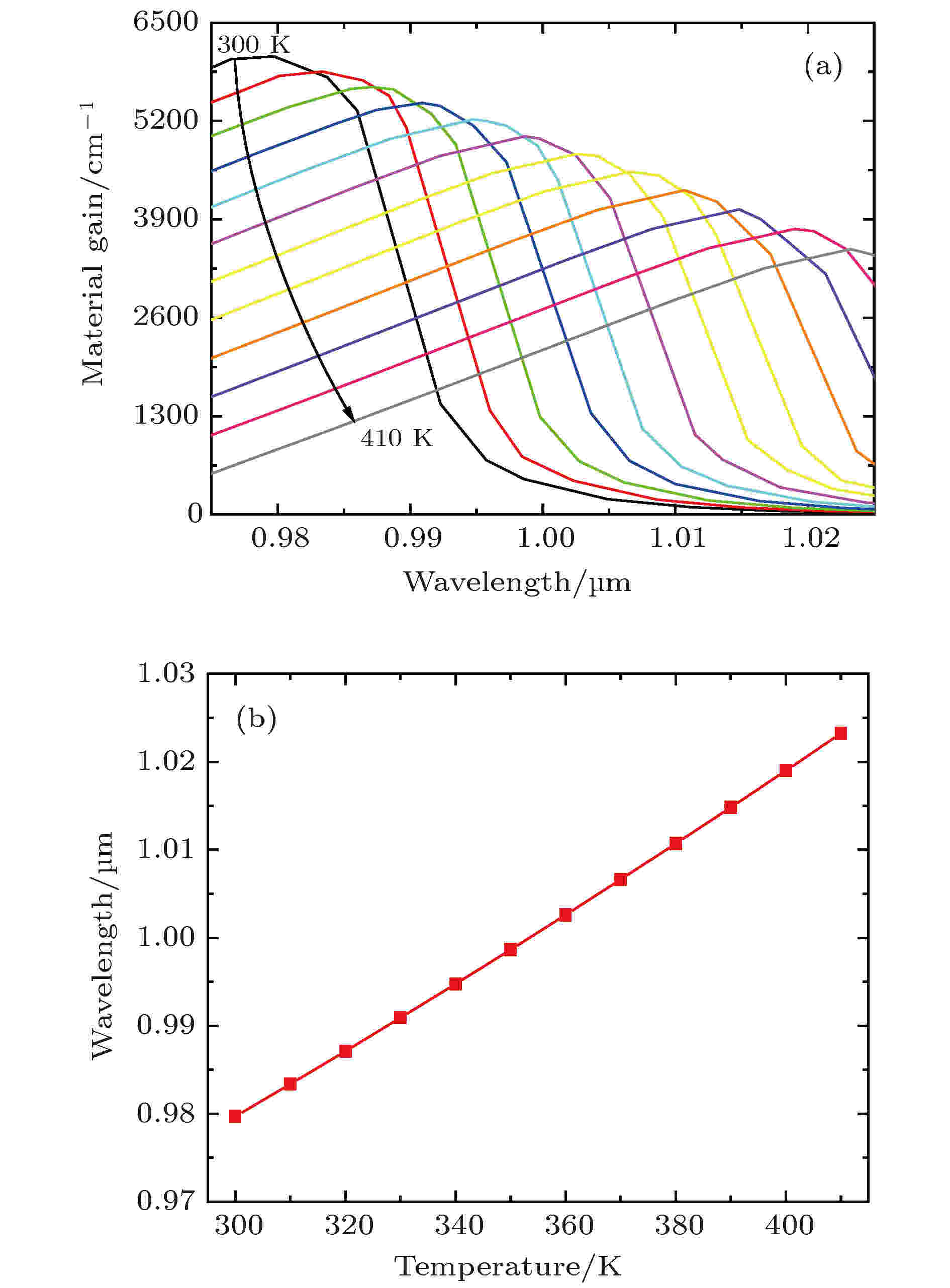
Figure4. (a) The gain spectra and (b) the gain peak wavelength of 5 nm InGaAs quantum well at different opera-ting temperatures.
图4(a)为模拟的量子阱增益谱随工作温度的变化. 随着工作温度的增加, 量子阱的整个增益谱向着长波长漂移, 并且增益峰值也随之快速降低. 图4(b)为统计的量子阱增益峰值波长随工作温度的变化. 图4(b)中增益谱峰值波长随工作温度的增加呈现线性关系, 温漂系数为0.36 nm/K. 根据该系数及芯片内部的实际工作温度后, 可以根据所需要的VECSEL激光出光波长, 确定出量子阱在室温下的增益谱峰值波长位置.
量子阱的势垒层可以限制载流子在量子阱内复合, 提高量子阱发光效率. 为保证量子阱结构具有良好的限制特性, 实现高的增益, 对量子阱两侧的势垒结构进行优化. 表1列出了5种较为常见的980 nm波段所用的量子阱/势垒发光区结构.
1 | Al0.06Ga0.94As barrier/InGaAs QW/ Al0.06Ga0.94As barrier |
2 | GaAs barrier/InGaAs QW/GaAs barrier |
3 | GaAsP barrier/InGaAs QW/GaAsP barrier |
4 | GaAsP/GaAs barrier/InGaAs QW/GaAs barrier/GaAsP |
5 | GaAs barrier/InGaAs QW/GaAsP barrier |
表1模拟的5种发光区材料结构
Table1.Simulated material structures of 5 kinds of luminous zone.
在上述结构中, 前4种为对称势垒结构的发光区, 其中第2种和第4种的区别在于第4种结构的GaAs外部又增加了GaAsP的应变补偿层. 第3, 4, 5种中结构带有GaAsP应变补偿层, GaAsP势垒的组分和厚度设计可以保证完全补偿InGaAs量子阱带来的应变. 第5种为由GaAs和GaAsP组成的非对称势垒结构. 图5为模拟的上述5种发光区结构的增益谱以及增益系数随载流子浓度的变化关系. 从图5(a)中可以看出, 这5种发光区的材料结构的增益峰值波长都小于980 nm. 如图4(b)所示, 在高温操作下, 输出波长会以0.36 nm/K的速度进行红移. 而芯片内的温度在高功率操作下约为340 K[23], 经计算需要对设计的波长进行蓝移15 nm左右. 此外, 第5种非对称的势垒结构的增益峰值波长明显短于其他结构, 而其增益峰值与第4种结构相差不大, 这是由于GaAs和GaAsP的非对称势垒造成量子阱内部波函数的非对称分布, 从而使得量子阱的整体量子效应增强, 能级宽度增加. 在图5(a)中, 第1种至第4种结构的增益谱形状相差不大, 增益峰值有明显区别; 第3种结构的发光区增益峰值明显高于其他几种结构, 这一方面是因为GaAsP与InGaAs具有较大的价带带阶, 更好地限制了载流子. 另外, 由于GaAsP与InGaAs的应变方向相反. 因此, 紧邻InGaAs的GaAsP在一定程度上增强了InGaAs的应变效应, 使得其增益水平得到提升.
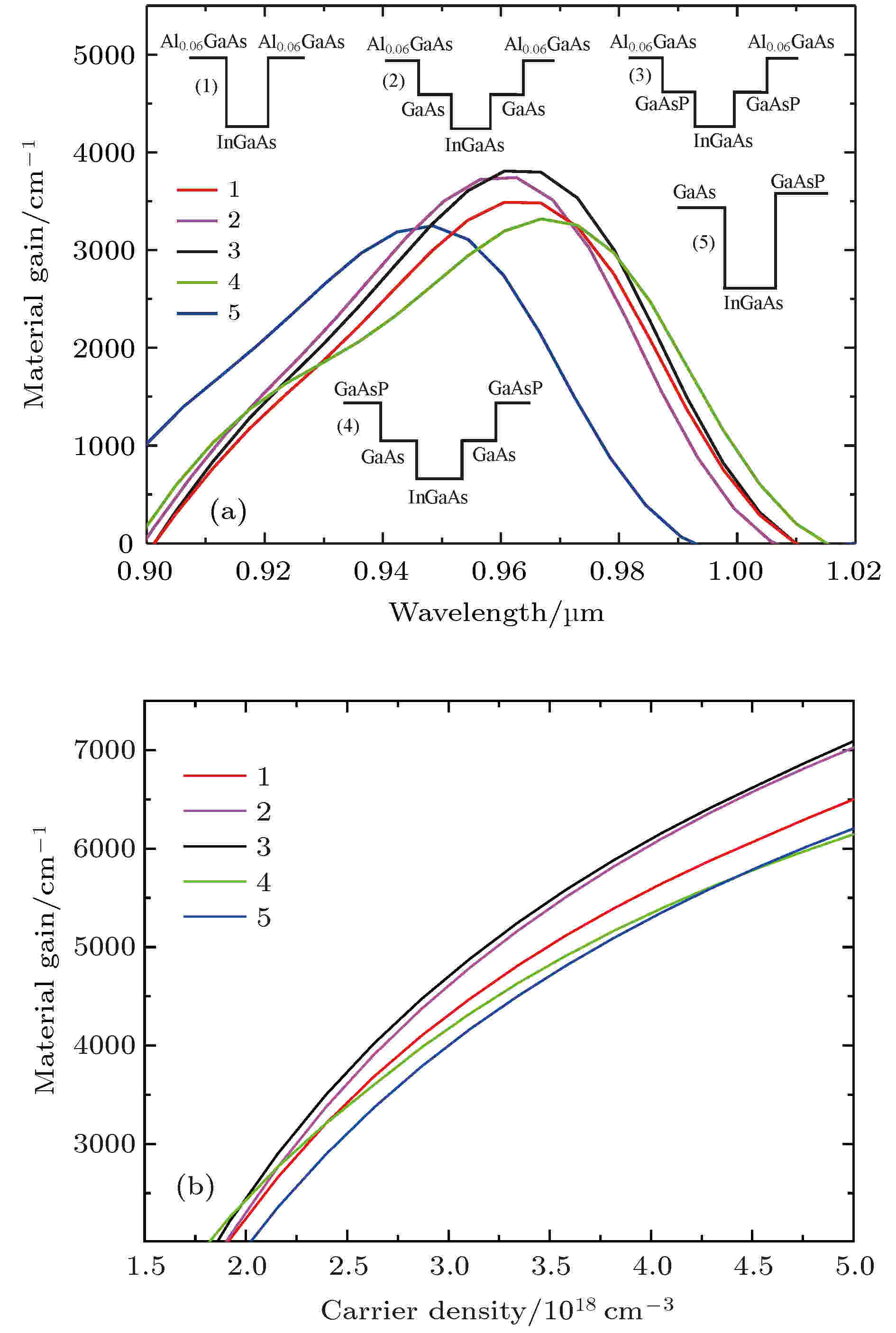
Figure5. (a) The gain spectra of InGaAs quantum well with different barrier layers; (b) the gain peak changing with the carrier density for different structures.
图5(b)中为5种不同量子阱结构的峰值增益随载流子浓度的变化. 可以看出, 这5种结构的峰值增益随载流子浓度的变化关系曲线的趋势是一致的, 在功率较高时有一定的饱和效应出现. 但是相比其他4种结构, 第3种结构的发光区一直可以实现更高的饱和增益, 并且在较低的载流子浓度下就可以产生光增益. 因此, 采用第3种结构的发光区, 即GaAsP barrier/InGaAs QW/GaAsP barrier结构, 可以为激光振荡提供较好的光增益特性. 除此之外, GaAsP结构带来了反向应变补偿, 可以有效补偿多个量子阱带来的应变效应, 使得量子阱及两侧吸收区的材料质量更好.
首先对制备的VECSEL增益芯片表面腔模特性进行验证, 采用椭偏仪测试的增益芯片反射谱如图6所示. 图6中测试了3个不同角度入射光的反射光谱, 0°表示垂直入射. 可以看出, 反射带带宽超过100 nm, 并且随着入射光角度的减小, VECSEL腔模波长增加; 垂直入射时, 腔模位置约在970 nm. 当入射角度较大时, 中心反射带的反射率降低, 并且腔模位置蓝移. 对于直型腔来说, 垂直入射的反射光谱腔模位置就是其出光波长位置. 在本实验中拟采用直行腔结构, 这就说明所制备的增益芯片出光波长在970 nm附近. 为了达到最佳的操作, 量子阱的发射光谱相对于微腔谐振波长要轻微地蓝移, 以解决积累在高抽运功率下量子阱(0.3 nm/K)和微腔谐振(0.1 nm/K)的不同发射热漂移[10]. 因此, 970 nm的腔模位置确认了增益芯片的准确生长. 特别需要注意的是, 对于808 nm的抽运光来说, 其入射角度必须在合适的位置. 入射角度过大, 虽然能够相对降低VECSEL芯片表面对808 nm抽运光的反射率, 但是在高功率输出的情况下, 聚焦在芯片表面的抽运光斑直径较大, 因而抽运功率密度分布不均匀, 进而影响VECSEL的激射[24]; 入射角度过小时, 抽运系统可能会阻挡激光振荡. 因此, 抽运光的入射度在0°—30°范围内最合适. 由图6可以看出, 所制备的增益芯片对抽运光的反射率较高, 达到20%左右, 这将在一定程度上影响整个系统的抽运效率.
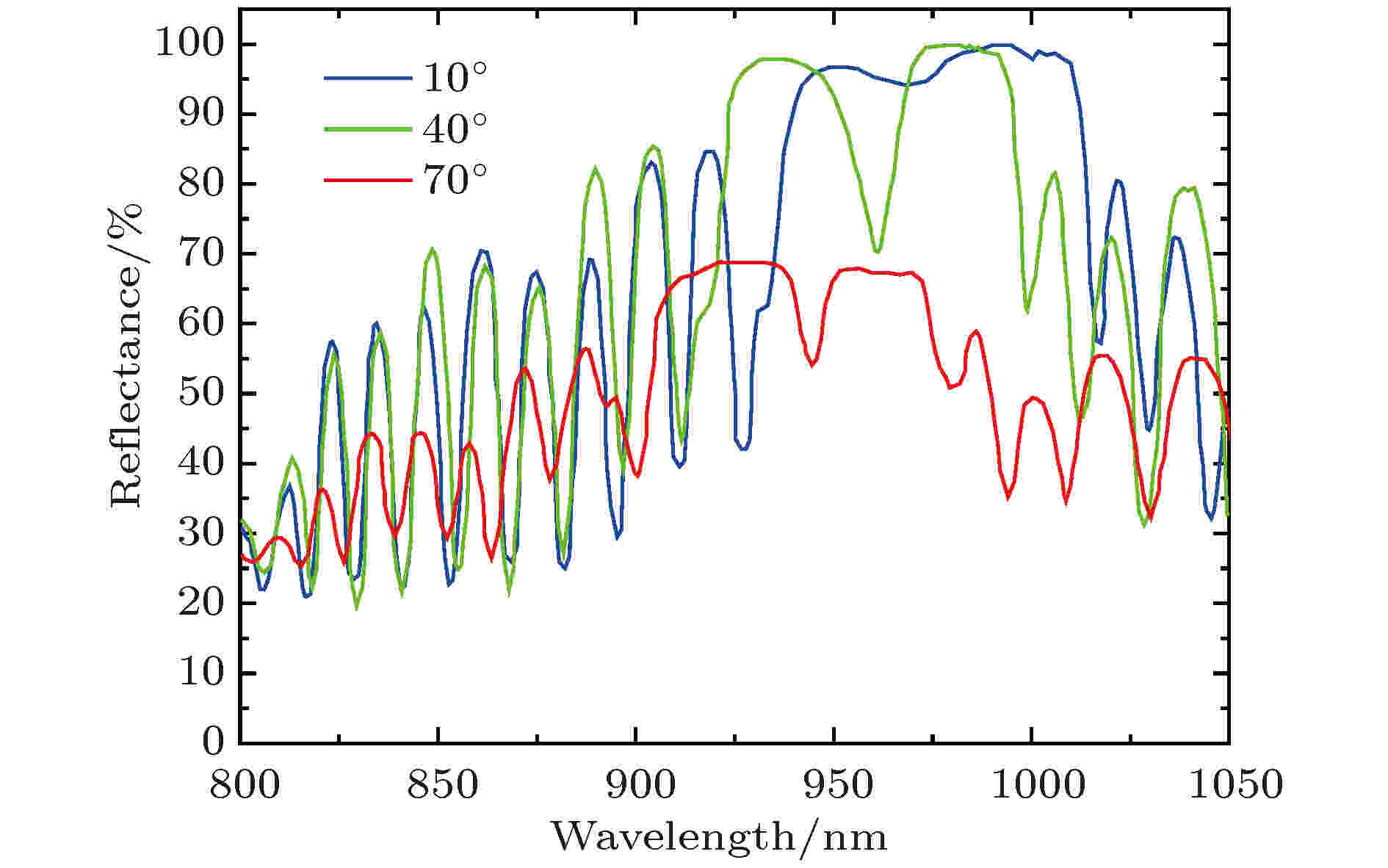
Figure6. The measured reflection spectra of the gain chip when the optical incident angle is 0°, 40°, and 70°.
搭建如图1(a) 所示的直型腔VECSEL系统, 铜热沉底部采用高功率TEC控温, 控温温度为–10 ℃. 抽运光源采用高功率的808 nm光纤输出激光模块, 光纤纤芯为300 μm. VECSEL系统采用3种不同反射率的外腔镜, 外腔镜反射率分别为96.3%, 97.7%和99.1%, 采用3种不同外腔镜搭建的VECSEL系统输出功率曲线如图7(a) 所示. 可以看出, 外腔镜反射率为97.7%时, VECSEL系统的功率输出水平明显优于其他两种反射镜系统. 当抽运光功率在35 W时, VECSEL系统的输出功率达到了9.82 W, 光光斜率效率达到35.5%, 并且VECSEL系统的功率曲线并没有出现饱和现象, 而只是受限于抽运光功率水平. 这3种外腔镜的反射率对VECSEL系统的阈值光功率也有影响, 采用99.1%反射率的外腔镜可以得到更低的阈值光功率, 约为5.5 W; 而采用96.3%和97.7%反射率的外腔镜, VECSEL系统的阈值光功率非常接近, 约为8 W.
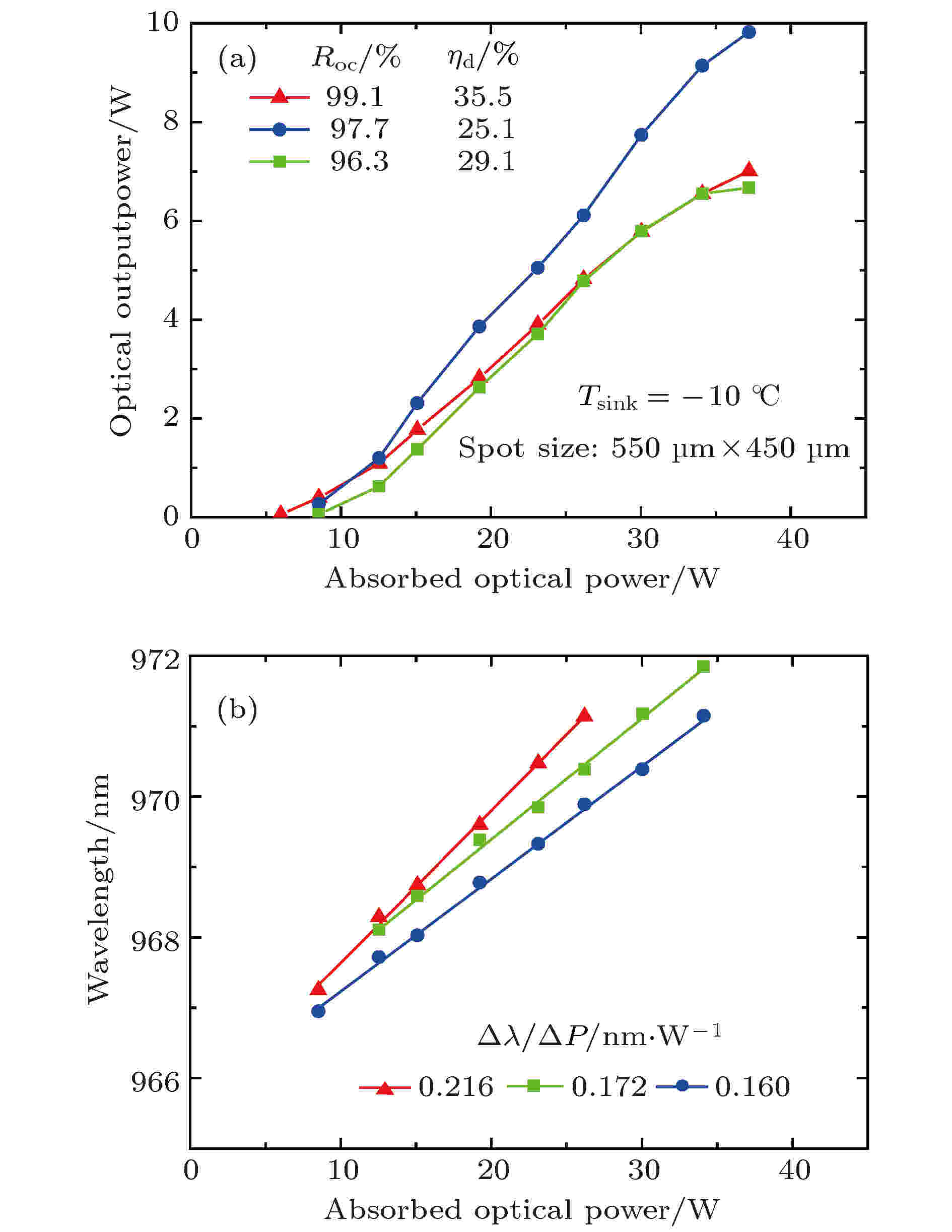
Figure7. (a) The output power of VECSEL and (b) the lasing wavelength changing with the pump power, with the output mirror reflectivity of 99.1%, 97.7%, and 96.3%.
为评估3种不同反射率的反射镜对VECSEL系统内部热效应的影响, 测量了3种反射镜输出的激光波长随抽运功率的变化关系, 如图7(b)所示. 图中激光波长随抽运光功率的变化基本呈现线性关系, 并且不同反射率的外腔镜所输出的激光波长漂移速度不一样. 很明显, 采用反射率为97.7%的外腔镜, VECSEL系统的激光波长随抽运功率的变化系数最小, 为0.16 nm/W; 而采用另外两种反射率的外腔镜, VECSEL系统的激光波长随抽运功率的变化系数为0.172 nm/W和0.216 nm/W. 这说明采用反射率为97.7%的外腔镜, 增益芯片的热积累更小, 这也是图7(a) 中该外腔镜输出的激光功率与光光斜率效率更高的原因. 我们还发现, 虽然图7(a) 中反射率为96.3%与反射率为99.1%的两种外腔镜输出功率曲线类似, 然而在图7(b) 中, 这两种反射率的外腔镜组成的VECSEL系统的激光波长随抽运光的漂移速度却不一样, 采用反射率为99.1%的外腔镜会有更大的波长漂移速度(0.216 nm/W), 也就是其增益芯片的热效应更明显. 这或许是因为虽然输出的功率类似, 但是更高的反射率意味着有更多的光在腔内振荡而无法输出, 因此增益芯片内部的光能量更高, 由此产生的光子再吸收更明显, 相应的自产热效应更强.
采用CCD相机观察外腔镜为97.7%反射率的VECSEL系统的输出光斑情况, 激光功率为9.82 W时的光场发散角测试结果见图8所示, 插图为所捕获的VECSEL系统输出光斑形貌. 由图8可以看出, VECSEL系统输出的光斑在正交方向上的发散角全角分别为9.2°和9.0° (1/e2全角), 具有良好的对称性, 并且激光光斑呈现良好的高斯分布. 由插图中捕获的激光光斑可以看出, 光斑呈现良好的圆形对称性, 并且分布非常均匀.
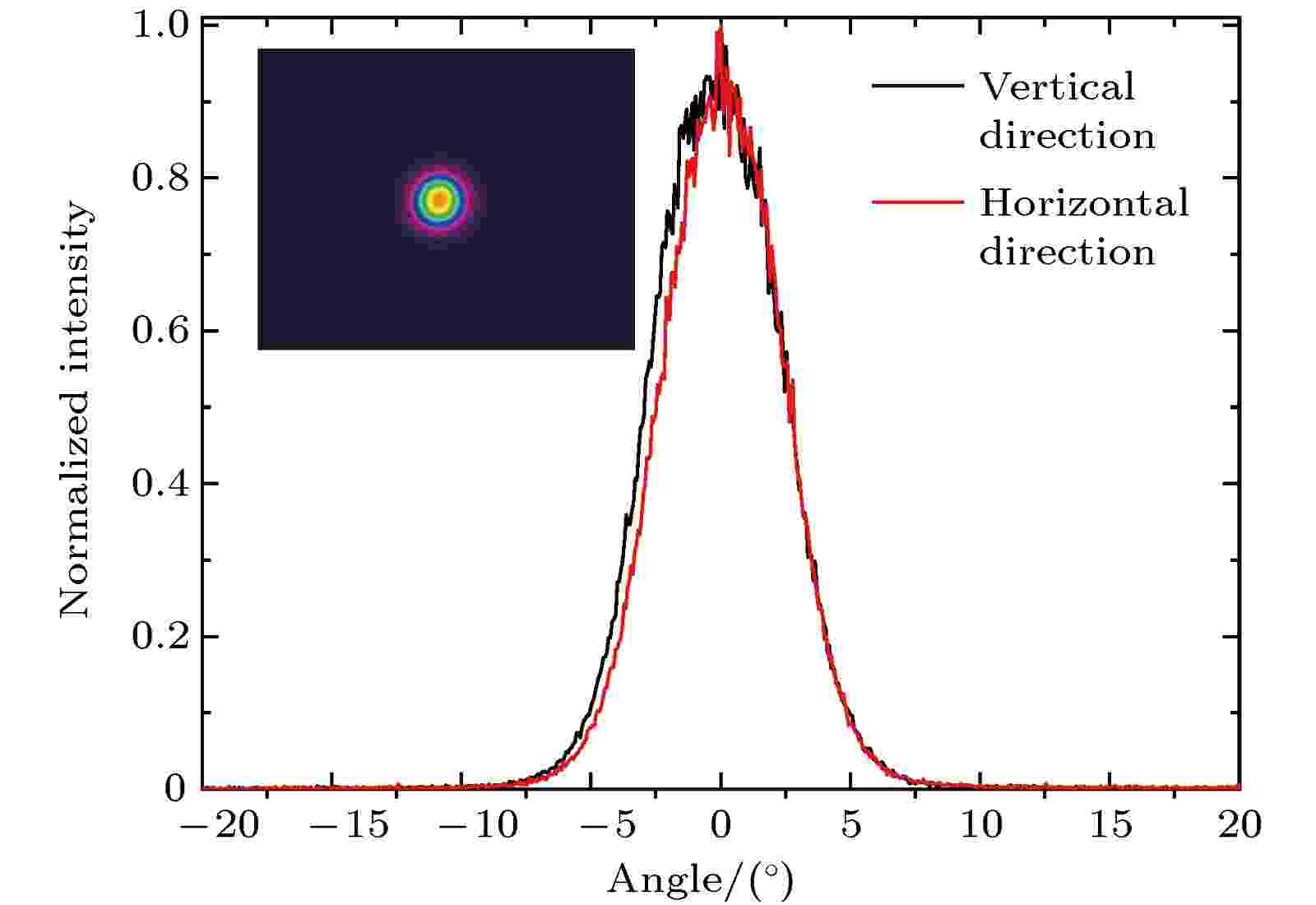
Figure8. The divergence angles of VECSEL along the orthogonal direction, inserted is the measured 2D optical spot pattern.