全文HTML
--> --> -->本文提出利用单晶硅制备具有法诺共振效应的超构表面(metasurface)结构[13], 利用其共振模场的场增强效应实现高效率的三次谐波激发(THG), 如图1(a)所示. 近年来, 基于高折射率纳米结构中共振效应的超构表面已被证明可以实现对光波传输以及光与物质相互作用过程的有效调控[14-18]. 相较基于贵金属的超构表面, 硅基超构表面具有在可见光波段吸收损耗低且可有效避免热效应[19-21]等优点. 已有多种基于硅基超构表面的线性光学器件被报道, 如宽带超构表面透镜[22,23]、偏振无关的光束波前调制器[24]等. 若在硅基超构表面中实现高效率的三阶非线性效应, 对进一步拓展这些光学器件的非线性功能至关重要. 本文通过设计与制备一种由L形米氏共振单元所组成的超构表面结构, 实现了硅介质THG信号的220倍增强, 其转换效率提升至3 × 10–7.
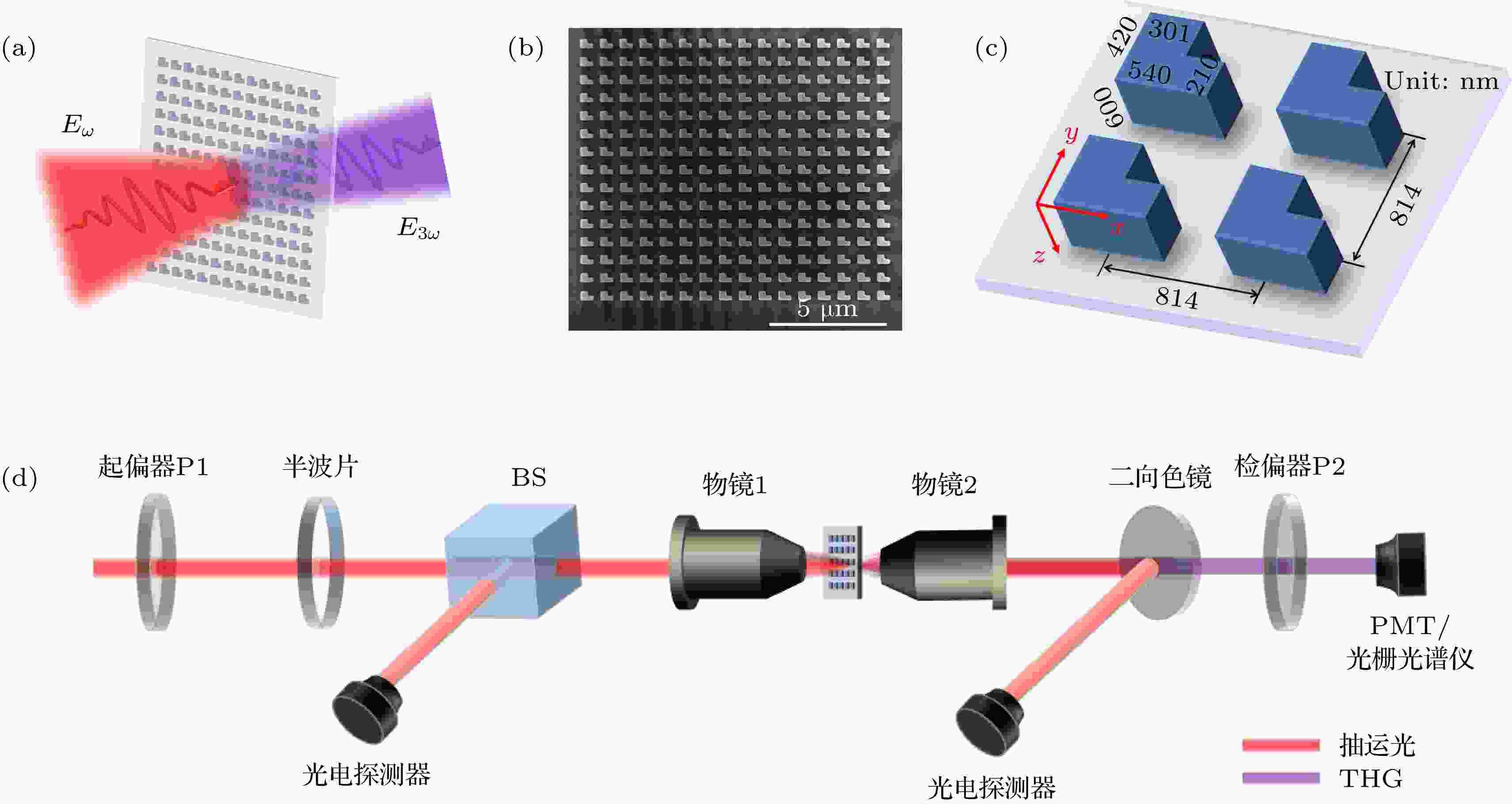
Figure1. (a) Schematic of THG from the silica-based metasurface; (b) a top-view scan electron microscope image of the metasuface; (c) the schematic diagram of the L-shaped resonators; (d) illustration of experimental set-up.
该超构表面的单个共振单元具有非对称结构, 使其面内的“亮模式”电偶极共振与面外的“暗模式”磁偶极共振发生模式耦合, 形成法诺共振. 且在阵列情况下, 单个共振单元共振模式的辐射衰减由共振单元间叠加的局域场进行补偿形成高Q的法诺共振和电磁场增强[13]. 为实现最大的场增强效应, 选用的结构为由16 × 15阵列排布的共振单元构成, 如图1(b)所示. 图1(c)给出了每个共振单元的具体结构参数.
图1(d)给出了测量硅基超构表面THG的实验光路系统, 其中物镜1 (50 × Mitutoyo Plan Apo NIR, NA = 0.42)用于聚焦抽运光并收集反射信号, 物镜2 (20 × Mitutoyo Plan Apo NIR, NA = 0.4)用于收集透射以及THG信号. 抽运光及THG信号光的偏振方向分别通过起偏器P1和半波片以及检偏器P2进行控制和检测.
实验中, 为测量所制备器件的法诺共振光谱, 使用波长范围在1530—1560 nm可调谐窄带连续激光器作为抽运光源, 系统的反射以及透射信号利用近红外光电探测器进行实时采集记录. 为观察和有效测量所制备器件激发的THG信号, 将抽运光源更换为可调谐皮秒脉冲光纤激光器, 其波长范围为1540—1560 nm, 脉冲宽度为8.8 ps, 重复频率为18.5 MHz. 硅超构表面结构内激发的THG信号穿过蓝宝石基底, 被物镜2收集并经二向色镜滤波后输入到光电倍增管(PMT)或光栅光谱仪中, 以测量其功率或波长信息.
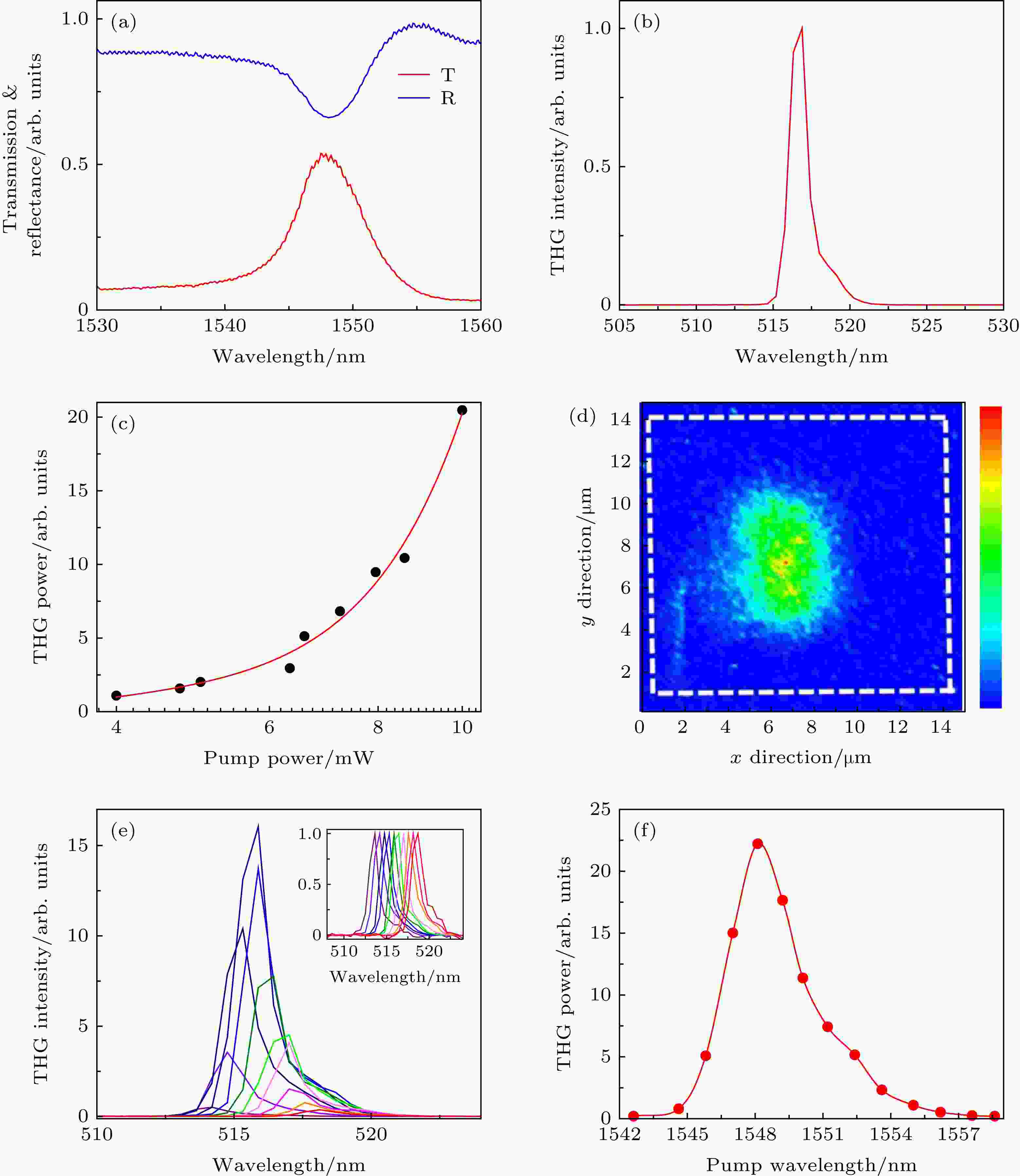
Figure2. (a) Reflection and transmission spectra of the metasurface in the wavelength range of 1530?1560 nm (T, transmission spectrum; R, reflection spectrum); (b) THG signal peak when the pump wavelength coincides with the resonant wavelength at 1548 nm; (c) power dependence of THG intensity; (d) spatial scanning of THG intensity distribution; (e) spectra of THG signals pumped with different wavelengths, and the inset shows the result of normalizing each line; (f) integral results for all THG spectra shown in panel (e).
在1548 nm波长处, 透射谱和反射谱分别出现极大值和极小值的情况, 意味着前向散射光在该点出现增强. 由此可以确定当抽运波长为1548 nm时, 可在共振单元内部激发共振效应, 共振峰半峰值宽度(FWHM) Δλ约为5.5 nm. 另外, 由于米氏共振单元具有L形, 可保证其横向电偶极子和纵向磁偶极子的米氏共振模式发生重叠, 使得共振单元间通过模式耦合形成法诺共振. 因此, 所获得共振线型呈现非对称的法诺线型, 且相较单个L形共振单元, 品质因子得到提高[13].
为了研究该共振效应对硅介质内三阶非线性效应的增强作用, 实验上采用脉冲激光激发超构表面结构的THG信号. 首先将激光器输出波长固定在位于共振波长中心的1548 nm, 产生了如图2(b)所示中心波长约为516 nm的THG信号. 保持抽运波长恒定, 连续调节激光器的输出功率, 得到如图2(c)所示的THG信号强度随抽运光功率的响应趋势, 图中红色曲线为三次方拟合函数曲线, 可以看出THG信号功率与抽运光功率间存在明显的三次方依赖关系. 当形成二维阵列时, 在单个共振单元法诺共振以及单元间局域场叠加补偿的共同作用下, 阵列中心区域结构单元支持明确的法诺共振模式且电磁场增强显著. 逐渐靠近阵列边缘时, 结构单元支持的共振模式存在较大辐射损耗, 使得电磁场增强较弱[13]. 因此, 由超构表面增强的三次谐波将呈现与超构表面共振模式分布相同的中间强、边缘弱的空间分布特性, 如图2(d)所示. 为了进一步提高THG的增强效果, 一方面, 可以通过改变共振单元的非对称系数改进共振单元模场的辐射衰减, 或者通过设计共振单元阵列的周期来扩大共振单元间模场的有效叠加区域等以增强超构表面在激发光波长处的电磁场分布. 另一方面, 可以构建在抽运激光和THG波长处均具有共振特性的超构表面, 通过双共振模式进一步提高THG的增强效果.
保持抽运光功率不变, 通过测量THG信号强度随抽运波长的依赖性, 进一步明确超构表面结构内所发生的共振效应对硅介质中所产生THG的增强效果. 图2(e)给出了当抽运波长从1540 nm逐渐移动到1560 nm的过程中, 所激发的THG光谱, 测量过程中, 所有入射波长对应的抽运激光功率均恒定为5 mW, 经透镜聚焦后的光斑直径约为3 μm. 由图2(e)光谱图可以看出, 随着抽运波长的改变, THG信号在中心波长位置发生移动的同时, 其强度表现出先增强后减弱的现象. 为了更为直观地说明这种强度变化, 对图2(e)中的每条THG谱线分别进行能量积分, 结果如图2(f)所示. 可以清楚地看到, 当抽运波长位于共振中心位置(1548 nm)时, 超构表面结构中的共振模式被激发, 并通过其强烈局域的模场对硅中的THG过程进行增强, 因此实现了最大的THG信号强度. 随着抽运波长逐渐偏离共振波长中心, 入射激光与共振模式不发生耦合, 仅在单次通过硅超构表面过程中对THG进行激发, 因此所产生的THG信号逐渐减弱并稳定到很低的强度. 因此, 通过对比波长位于1548 nm与远离1548 nm的抽运激光在相同功率下所激发的THG信号, 可以提取出超构表面结构的共振模式对THG的增强效果. 计算得到的增强因子约为220. 这种提取增强因子的方法, 由于保持抽运激光聚焦在超构表面相同位置处, 可以避免由于有效硅材料不同而引起的误差. 该结果证明了所设计的超构表面内发生法诺共振效应时, 结构内部的局域电磁场强度获得了显著增强, 且利用这种增强效应可以有效提高硅介质内所激发的THG信号强度.
为了阐释和验证上述实验结果, 根据所设计的超构表面结构参数利用有限元数值模拟软件(COMSOL)计算该结构发生共振时局域电磁场的分布特性, 数值模拟模型为位于500 μm厚蓝宝石衬底上单晶硅(n = 3.48)的16 × 15共振单元阵列, 且x, y和z方向均使用完美匹配层. 在入射端口设置沿y方向偏振的1530—1560 nm的宽谱平面偏振激发光. 在波长扫描后, 由所采用的COMSOL软件中的S参数得到超构表面的透射和反射谱线. 图3(a)左侧展示了16 × 15阵列超构表面的模场分布, 表明共振模场的分布主要集中在超构表面结构的中心区域. 图3(a)右侧为对单个共振单元的电磁场分布的模拟结果, 呈现出横向的电场分量(右上)以涡旋态的形式围绕在中心部位, 而纵向的磁场分量(右下)则集中在电场分量的中央. 这种强烈局域的电磁场模式与硅结构的重合可有效保证三阶非线性的增强.
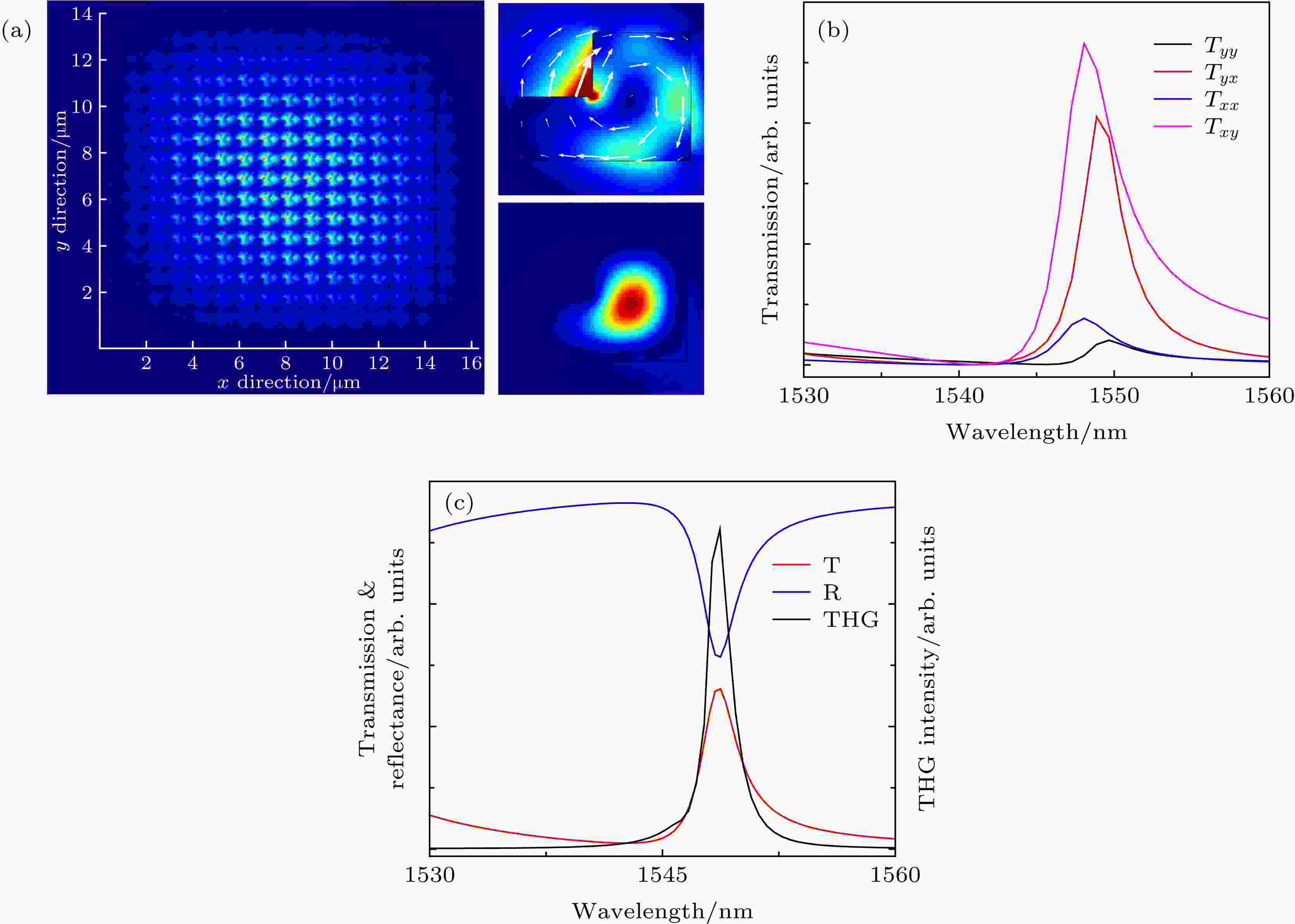
Figure3. (a) Numerical simulation of the distribution of electric field of the metasurface in the x-y plane; (b) numerical simulation of the anisotropic transmission spectra of the sample; (c) numerical simulation of reflection (R), transmission (T) spectra and intensity of THG signals.
考虑到由L形结构的非对称性引起的各向异性, 通过数值模拟的方式分析了样品透射光谱对入射光偏振方向以及透射信号偏振方向的依赖关系. 数值计算了Txx (入射光具有x方向偏振, 透射信号沿x方向检偏, 余下同理), Txy, Tyx, Tyy四种透射谱线, 结果如图3(b)所示. 数值模拟结果表明, 入射光的偏振方向沿x或y方向, 均可在结构内激发出共振模式, 但对应的共振波长相较约有4 nm的偏移. 此外, 无论是入射光的偏振沿x方向还是y方向, 在结构中激发的共振模式所辐射出的透射信号能量在y方向偏振分量均更强. 对比四组传输谱线, 可以看出Tyy具有最大的传输率, 因此实验上采用y方向偏振的入射光激发超构表面的共振模式, 如图2(a)所示. 进一步模拟计算了不同波长情况下超构表面结构内的局域电场分布, 并分别计算该结构在y偏振入射情况下共振波长处反射和透射信号的远场辐射强度(无检偏情况下), 结果如图3(c)所示. 数值模拟所获得共振线型与实验结果相符, 均为非对称的法诺线型, 且具有接近的共振线宽. 在此基础上, 根据模拟得到的局域电场分布(Ex, Ey, Ez), 结合硅材料的三阶非线性极化系数可以获得超构表面结构中激发的三阶非线性极化强度












考虑到共振单元两臂(分别沿图1(c)中的x和y方向)具有不同的尺寸, 当入射光的偏振方向发生改变时, 每个共振单元可能产生不同的共振响应. 因此, 测量了该超构表面结构对入射光偏振方向的敏感特性以及所激发THG信号的偏振依赖性, 结果如图4所示. 实验中选择y方向为基准方向, 入射光偏振方向相对于y方向的旋转角度由?表示, 如图4(a)所示.
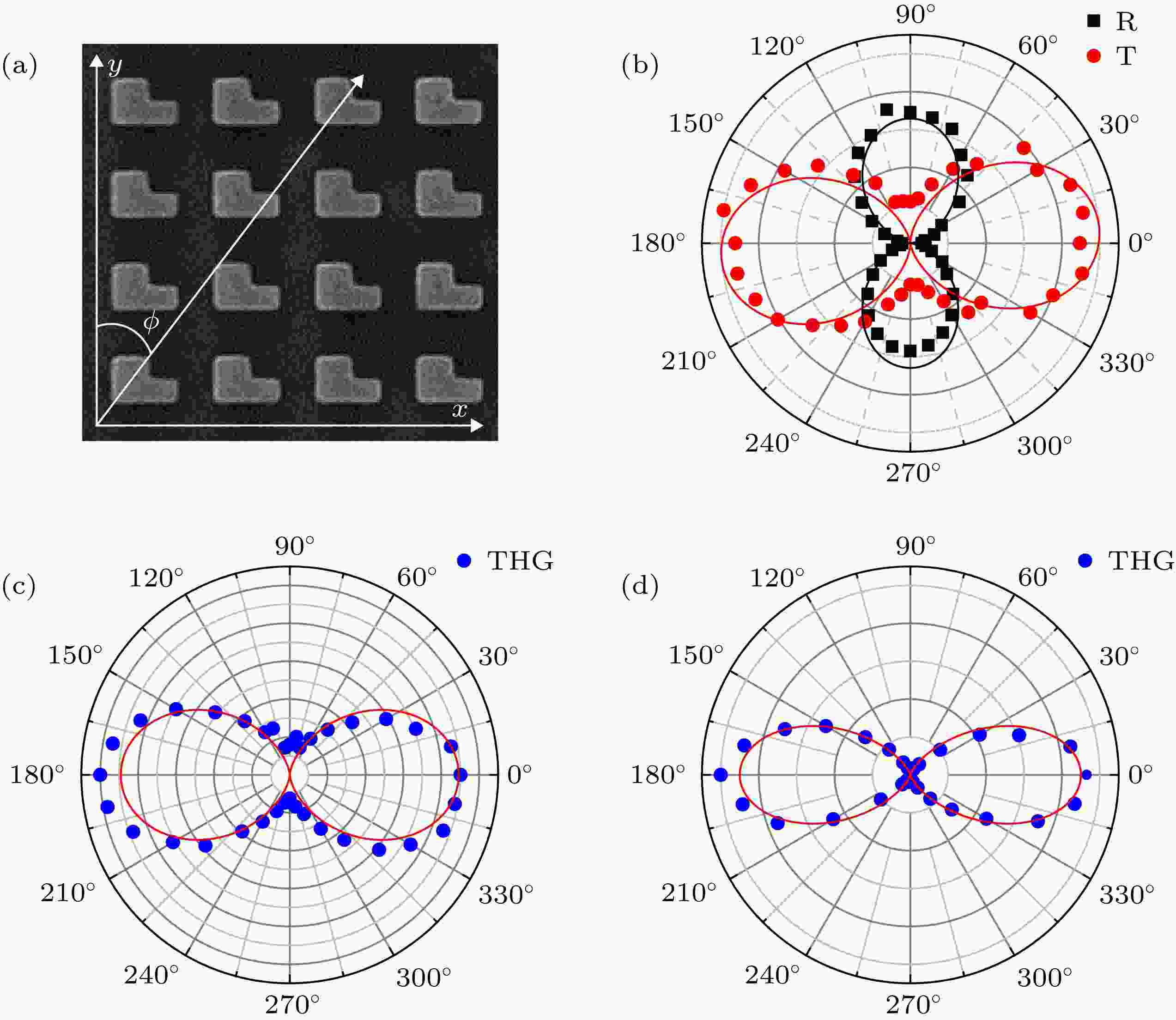
Figure4. (a) Orientation illustrated in the metasurface; (b) experimental measurement of polarization dependence of transmitted and reflected signals; (c) experimental measurement of the polarization dependence of THG signal intensity on pump light; (d) polarization detection of THG signals.
实验测量中, 激光器输出波长为1548 nm的抽运光经过起偏器和半波片调制后可形成沿任意方向偏振的线偏振抽运光. 透射/反射信号以及THG信号收集光路均无检偏器, 即系统的透射/反射光信号和THG信号均直接由光电探测器收集. 图4(b)展示了随着抽运光偏振态的改变, 收集到的透射和反射信号的强度变化. 可以看出, 当抽运光偏振方向与共振单元宽臂(沿y方向)平行时, 透射信号最强且反射信号最弱, 消光比超过了10 dB; 而当抽运光偏振光偏振方向与窄臂(沿x方向)平行时, 反射信号达到最强, 同时透射信号降到最低. 该结果表明, 当抽运光沿y方向偏振时能够最有效地激发该超构表面的共振效应, 使得入射光向前向散射光的转化效率达到最高[28]. 同时, 随着抽运光偏振方向由x方向至y方向转变的过程中, 结构内的共振效应以及局域电磁场强度逐渐增强, 并进一步表现为THG信号强度的提高, 如图4(c)所示, THG信号的消光比约为7 dB. 值得注意的是, 图4(b)和图4(c)中结果在90°情况下(入射光具有x方向偏振)均未能实现完全消光. 该结果可以借助图3(b)中的模拟结果进行解释. 虽然x方向偏振入射光激发出的共振模式与y方向偏振入射光激发的共振模式之间存在4 nm的中心波长差, 但波长为1548 nm的x方向偏振入射光仍然可以激发出部分共振模式, 使得透射信号和THG信号在x方向偏振入射光激发下仍可以测到.
基于以上结果, 将抽运光的偏振方向固定在y方向, 实现结构内的最强共振激发效果, 进而在THG信号收集光路上添加检偏器P2并对THG信号的偏振方向进行检测. 如图4(f)所示, 结果表明, 当抽运光为沿y方向偏振的线偏振光时, THG信号光同样为沿y方向偏振的线偏振光, 且其消光比达到15 dB. 这些偏振相关特性意味着仅需要改变抽运光的偏振态或者共振单元的排列方式, 即可有效地控制超构表面结构中所产生THG的强度或偏振方向, 这将进一步为提高硅基超构表面的功能性提供新的思路.