

Water sources of the typical desert vegetation in Ebinur Lake basin
HAO Shuai

通讯作者:
收稿日期:2020-12-2修回日期:2021-06-3网络出版日期:2021-07-25
基金资助: |
Received:2020-12-2Revised:2021-06-3Online:2021-07-25
Fund supported: |
作者简介 About authors
郝帅(1982-), 男, 博士, 主要从事干旱区水文过程研究。E-mail:

摘要
关键词:
Abstract
Keywords:
PDF (2825KB)元数据多维度评价相关文章导出EndNote|Ris|Bibtex收藏本文
本文引用格式
郝帅, 李发东. 艾比湖流域典型荒漠植被水分利用来源研究. 地理学报[J], 2021, 76(7): 1649-1661 doi:10.11821/dlxb202107006
HAO Shuai, LI Fadong.
1 引言
干旱半干旱区,降雨量稀少,水分是限制植被分布和生长最重要的因子[1]。干旱区自然环境中,荒漠植被可利用水源包括降水、河水、土壤水和地下水。干旱区盐生植被在长期进化过程中,会选择相适应的水分来源,以提高适应干旱环境的能力[2]。植被水源利用可由许多方法来确定,比如根系挖掘法[3]、液流测定法[4]、放射性示踪迹氚法[5]等,这些方法的不足之处在于成本高,不能详细区分不同水源,且存在放射性危险等。稳定同位素技术作为一种安全有效,没有破坏性的方法,可以用来确定和区分植被水分利用来源。在植物体内,根系吸收水分沿木质部运输,水分过程以液流形式存在,在到达未栓化幼嫩枝条和叶片之前,与外界环境不发生水分的交换,同位素不存在分馏现象[6,7,8]。分析木质部水和潜在水源的同位素值,可判断植物水分利用方式及量化不同水源在植物体内所占比例。运用稳定同位素方法研究植被水分利用在陆地生态系统中大量应用,比如森林生态系统[9,10]、农田生态系统[11,12]、草地生态系统[13,14]等,但干旱区植被生态系统研究较少,不同生境盐生植被吸水策略问题仍不十分明确,是干旱区植被生态系统研究的一个短板。艾比湖是新疆维吾尔自治区第一大咸水湖,处于准噶尔盆地西南低洼处,具有典型的干旱区生态环境特征,是流域的水盐汇集中心,它维护着整个流域生态系统的稳定,是新疆乃至整个西北地区的一道生态屏障[15]。在气候变化和人类活动影响下,艾比湖流域出现植被退化,水分的过度开发利用、土壤盐碱化加重等现象。将植被和水体作为一个系统综合考虑,研究荒漠植被和潜在水源相互作用关系,确定其不同生长期水分利用策略,为当地植被生态系统恢复提供理论支持。
本文选取艾比湖流域4种生境(河岸、沙丘、荒漠、盐沼)对应的典型荒漠植被胡杨(Populus euphratica)、梭梭(Haloxylon ammodendron)、白刺(Nitraria sibirica)和盐穗木(Halostachys caspica)为研究对象,运用氢氧稳定同位素方法,研究其在整个生长季(3—10月)水源以及用水策略。本文的目的是:① 阐明研究区降水、土壤水、植被茎水稳定同位素组成特征;② 量化典型荒漠植被生长期内水源利用比例;③ 探求荒漠植被水源利用影响机制。以期为干旱区植被生态系统保护和恢复提供科学依据。
2 研究区概况与研究方法
2.1 研究区概况
艾比湖流域(44°02'N~45°23'N, 79°53'E~83°53'E)属于典型的温带干旱大陆性气候,年平均气温8.0 ºC,年均降水量89.9~169.7 mm,年均蒸发量1569~3421 mm。艾比湖是准噶尔盆地西部最低洼地,是区域水盐汇集的中心,湖水主要来源于地表河流及地下水补给,目前以地表径流的形式补给的河流主要是精河和博尔塔拉河。流域地下水主要来自积雪融水和河水补给,艾比湖湿地周边地下水埋深约5 m[17]。该流域地貌景观多样,集湿地和荒漠化过程于一体。流域内植物区系受中亚和蒙古植物区系影响,植被过渡性明显,植物种类以杨柳科、藜科、柽柳科、禾本科等植物为主,典型植被主要有胡杨(Populus euphratica)、梭梭(Haloxylon ammodendron)、多枝柽柳(Tamarix ramosissima)、盐穗木(Halostachys caspica)、西伯利亚白刺(Nitraria sibirica Pall)、盐爪爪(Kalidium foliatum)、盐节木(Halocnemum strobilaceum)、碱蓬(Suaeda pterantha)、甘草(Glycyrrhiza uralensis)等[16]。
2.2 试验设计和野外采样
选取艾比湖流域不同生境建群种,分别为盐穗木(盐沼地)、胡杨(河岸)、白刺(荒漠)和梭梭(沙丘),在不同生境设置20 m×20 m样地(图1),在样地内选取3株在高度和冠幅均反映样地平均状况的植被作为采样对象,对植株栓质枝条进行采样,同时对土壤、河水和地下水进行采样,样地内荒漠植被形态特征见表1。图1
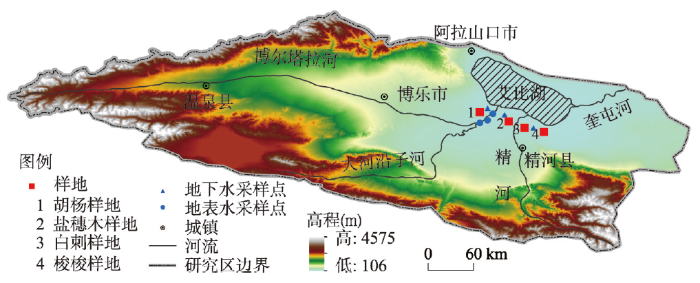
图1研究区样地设置及采样点示意图
Fig. 1Schematic diagram of sample setting and sampling points in the Ebinur Lake basin
Tab. 1
表1
表1典型荒漠植被形态特征
Tab. 1
植株 | 树高(cm) | 冠幅(南北(cm)×东西(cm)) | 生长型 | 根分布类型 | 群落类型 | 群落盖度(%) | 生境 | 采集地 |
---|---|---|---|---|---|---|---|---|
盐穗木 | 52.3±12.3 | (21.3±9.2)×(12.1±4.6) | 小灌木 | 浅根系 | 盐穗木—盐爪爪 | 15 | 盐沼地 | 北地站 |
胡杨 | 312.2±24.3 | (214.2±45.6)×(245.4±56.4) | 乔木 | 中深根系 | 胡杨—芦苇 | 50 | 河岸 | 博河站 |
梭梭 | 125.3±14.4 | (56.4±10.3)×(64.5±23.5) | 小乔木 | 中深根系 | 梭梭 | 10 | 沙丘 | 鸵鸟站 |
白刺 | 23.2±9.4 | (104.6±18.3)×(96.3±10.5) | 小灌木 | 浅根系 | 柽柳—白刺 | 30 | 荒漠 | 鸵鸟站 |
新窗口打开|下载CSV
样品采集时间覆盖植被整个生长季(3—10月)。河水采集主要在河岸生境,采集博尔塔拉河水;地下水在样地附近3个水井采集,3个井为饮用水井,井水编号从左到右依次为1#、2#和3#(图1),采集时必须保证是新鲜水,避免抽取水管中滞水;土壤采集靠近选取的植株,用土钻钻取土壤,采集深度为200 cm,其中0~40 cm深度每隔10 cm采集一层,40~200 cm深度每隔20 cm采集一层,每个植株周边钻取3个土壤剖面;植株采集要尽量减少光照强度等外界条件对同位素组成分析结果的影响,植株样品采集尽量在上午9:00—11:00。采集时选取栓质枝条,4个方向各采集1个3~5 cm小段,去除外皮。所采集样品均装入带有螺旋瓶盖的玻璃瓶中,用Parafilm膜密封瓶口,然后冷藏保存。
2.3 样品水分抽提及氢氧同位素组成测试
样品水分抽提及氢氧同位素组成测试在新疆师范大学新疆干旱区湖泊环境与资源实验室完成,采集水样均需先经过0.45 μm醋酸纤维素滤膜过滤。植株和土壤样品水分及河水提取采用全自动真空冷凝抽提系统(LI-2100),该仪器在样品水分抽提过程中不会发生同位素分馏,水分提取率在98%以上。氢氧稳定同位素组成(δ2H和δ18O)利用液态水稳定同位素分析仪(Model DLT-100)分析。每个样品分析6次,为了减小记忆效应,舍弃前2次分析结果,最终结果取后4次分析结果平均值。利用低温真空抽提技术获得的植株水有可能掺杂甲醇和乙醇类有机物质,醇类有机物与水的吸收峰非常接近,引起利用光谱方法测定的同位素组成产生误差。为了提高植株水δ2H和δ18O值测试的精度,本文将测定的植株水同位素组成经Schultz等[18]拟合的有机物校正曲线进行校正,尽量降低有机物对测定结果的影响。测得的氢氧稳定同位素组成为维也纳标准平均大洋水(V-SMOW)的千分偏差,可用以下公式表示:式中:Rsample和Rstandard分别为采集水样和标准样品的O或H的同位素比率(18O/16O或 2H/1H),其中δ2H和δ18O的测定精度分别为0.5‰和0.15‰。
2.4 数据处理和统计分析
本文采用IsoSource1.3.1软件计算植物对各水源的利用比例。计算时,增幅设为1%,质量平衡公差设置为0.1,IsoSource程序会利用线性混合模型计算所有可能的解,具体计算过程见Phillips等[19]。在分析比较各土壤层间土壤含水量和氢氧同位素值差异时,采用单因素方差分析(P < 0.05)。运用独立样本T-test来检验不同植被间茎水同位素值间差异性(P < 0.05)。统计分析在SPSS17.0中实现,图形在Origin 8.0中制作。
2.5 植被潜在水源划分
本文将植被潜在水源划分为5类:① 浅层土壤水(0~60 cm),同位素值变化有明显的季节及层间变化,主要是本层土壤容易受降水、温度、蒸发等气候因素影响;② 中层土壤水(60~140 cm),同位素值仍有较大季节性及层间变化,但与表层相比趋势已经趋缓;③ 深层土壤水(140~200 cm),较小的季节及层间变化,具有相对一致的同位素值;④ 地下水,同位素值季节变化很小或几乎没有变化;⑤ 河水,同位素值具有季节性变化特点[20,21,22]。土壤分层合并加权处理同位素值的公式如下所示:式中:δWT是土壤层合并后加权处理的土壤水δ2H和δ18O值;swcx和δx分别是土壤深度x处的土壤含水量与土壤水δ2H和δ18O值。
3 结果分析
3.1 降水稳定同位素组成变化特征
根据艾比湖流域事件尺度降水δ2H和δ18O值,统计分析得到该区域大气水线为δ2H=6.93(±0.18)δ18O-5.43(±1.37)(R2 = 0.99, n = 87)。与全球大气水线(δ2H = 8δ18O+10, GMWL)相比,研究区大气水线的斜率偏小。这主要因为研究区:① 地处内陆干旱区,次降水量小,空气湿度低,降水在降落过程中经历较强的蒸发分馏;② 远离海洋,降水水汽有相当一部分来自局地水循环[23],局地水分蒸发导致降水的不平衡分馏和重同位素的富集,使得大气水线的斜率偏小。艾比湖流域降水的
Tab. 2
表2
表2艾比湖流域降水δ2H和δ18O值季节变化(‰)
Tab. 2
春季 | 夏季 | 秋季 | 冬季 | ||
---|---|---|---|---|---|
均值 | -96.6 | -45.5 | -103.0 | -129.7 | |
范围 | -98.4~-78.4 | -63.1~-34.5 | -132.7~-62.8 | -148.2~-98.3 | |
均值 | -12.33 | -2.50 | -11.31 | -18.63 | |
范围 | -12.89~-9.32 | -5.94~1.20 | -17.74~-5.64 | -20.16~-14.38 |
新窗口打开|下载CSV
3.2 土壤水及地下水稳定同位素组成变化特征
植被生长季,不同生境土壤含水量较低,剖面变化表现为随着深度增加逐渐增加,由0~60 cm层的2.9±1.2%增加到140~200 cm层的18.4±2.3%(表3)。土壤水剖面Tab. 3
表3
表3不同生境土壤剖面土壤水含量及δ2H和δ18O值
Tab. 3
生境 | 0~60 cm | 60~140 cm | 140~200 cm | ||||||||
---|---|---|---|---|---|---|---|---|---|---|---|
含水量(%) | 含水量(%) | 含水量(%) | |||||||||
沙丘 | 2.9±1.2 | -41.5±15.2 | -2.48±1.45 | 5.2±1.0 | -60.5±8.1 | -7.19±0.93 | 8.9±1.1 | -66.3±5.2 | -8.50±0.49 | ||
荒漠 | 3.2±1.3 | -51.4±13.7 | -3.40±1.25 | 7.5±1.2 | -67.5±7.3 | -7.88±1.08 | 11.2±1.6 | -73.8±4.7 | -10.21±0.84 | ||
河岸 | 5.2±1.5 | -55.6±11.4 | -4.31±1.95 | 10.2±1.6 | -73.1±6.7 | -8.82±0.83 | 18.4±2.3 | -79.3±5.7 | -11.20±0.43 | ||
盐沼地 | 3.5±1.4 | -48.7±17.1 | -2.37±1.86 | 7.9±2.3 | -63.5±8.2 | -6.90±1.21 | 11.3±3.4 | -68.4±3.8 | -9.02±0.35 |
新窗口打开|下载CSV
土壤水中稳定同位素组成变化受大气降水、地表蒸发、水分在土壤中的水平迁移和垂直运动等多种因素的影响。在植被生长季,土壤水稳定同位素组成剖面总体变化表现为随着深度增加同位素值逐渐减小,到深层,土壤水同位素组成变幅最小,160 cm以下趋于稳定(图2),这同Sprenger[25]的研究结论是一致的。这是因为,深层土壤水在长期的混合和累积作用下,包含了降水和土壤剖面水同位素信息;再者,土壤深层受到气象因素影响较小,蒸发作用较弱,导致土壤水同位素组成的剖面变化特征。但是,本文中春季和秋季土壤表层的变化趋势并不完全符合这个规律,春季不同生境δ2H和δ18O值均在20~30 cm深度达最大值,说明积雪对表层20 cm以上土壤水稳定同位素组成有直接的影响。秋季不同生境土壤水稳定同位素值均在10~20 cm出现增加现象,这与夏季不断减小的趋势不同。主要是秋季温度降低而降雨量与夏季基本持平,降雨多是小强度降雨,入渗土壤较浅,导致表层10 cm土壤水同位素值不是最高,而土壤20 cm处由于得不到降雨的补充且蒸发影响明显,导致同位素值最高。
图2
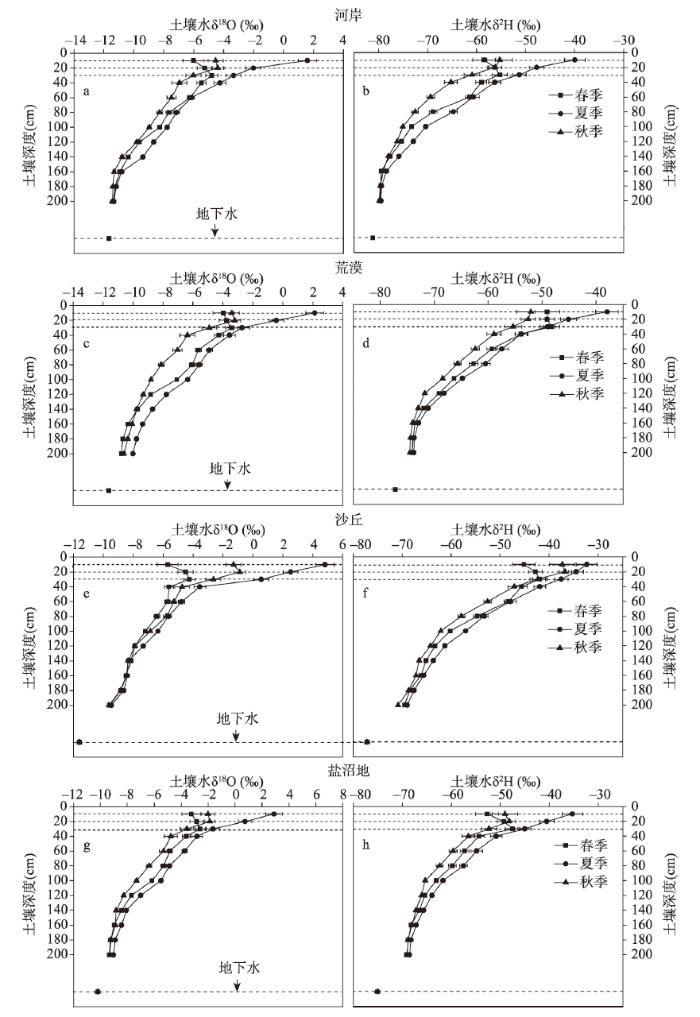
图2不同生境土壤水δ2H和δ18O值剖面(0~200 cm)变化特征
注:各图上部3条虚线自上而下依次表示土壤10 cm、20 cm和30 cm剖面。
Fig. 2The soil moisture δ2H and δ18O variation at different depths in different habitats
艾比湖流域地下水同位素值表现了一定的季节性差异,但差异不明显(表4)。不同季节间氢氧同位素值总体表现为春季最高,秋季次之,夏季最小。可以看出,地下水同位素值的季节变化同土壤水不同。通过分析可知,土壤水同位素组成主要受气象因素影响,而地下含水层分布在地表以下,井深在80 m左右,受外界气象因子变化影响较小,主要受补给源特征和水—岩作用影响。夏季山区降雨多,温度高,加上冰雪融化补给地下水,导致地下水表现出了较低的同位素值。
Tab. 4
表4
表4地下水δ2H和δ18O值季节变化特征(‰)
Tab. 4
井号 | 春季 | 夏季 | 秋季 | ||||||
---|---|---|---|---|---|---|---|---|---|
井深(m) | |||||||||
1# | -80.1±2.4 | -12.03±0.92 | -80.5±1.8 | -12.32±0.74 | -80.3±3.1 | -12.25±1.26 | 75 | ||
2# | -74.6±2.5 | -10.44±0.63 | -75.0±2.6 | -10.71±1.12 | -74.8±2.4 | -10.63±0.83 | 80 | ||
3# | -76.8±2.6 | -11.35±1.21 | -77.2±1.6 | -11.63±0.45 | -77.0±2.3 | -11.46±0.71 | 80 |
新窗口打开|下载CSV
3.3 植株水稳定同位素组成特征
不同生境植被植株水δ2H和δ18O值时间变化见图3。不同植株水δ2H和δ18O值时间变化趋势基本一致,表现为春季最高,夏季最低,秋季又逐渐增加,近似于“U”型。不同植被生长季内植株水δ2H和δ18O值不同,梭梭平均为-69.6‰和-9.36‰,分别在-71.4‰~ -67.8‰和-9.84‰~-8.81‰之间;盐穗木平均为-53.7‰和-4.47‰,分别在-57.6‰~ -47.8‰和-5.04‰~-3.47‰之间;白刺平均为-64.4‰和-7.49‰,分别在-70.8‰~-53.7‰和-8.91‰~-4.92‰之间;胡杨平均为-75.1‰和-10.18‰,分别在-80.4‰~-69.6‰和 -11.52‰~-7.51‰之间。从平均值来看,盐穗木植株水稳定同位素值最大,其次为白刺和梭梭,胡杨最小。从同位素值变化幅度来看,梭梭、盐穗木较小,白刺和胡杨较大。不同植被植株水同位素值季节间差异不同,经过方差分析可知,梭梭和胡杨植被春季、夏季和秋季间同位素值差异显著(P < 0.05),盐穗木和白刺夏季和秋季间同位素值差异不显著(P > 0.05),而春季与夏季和秋季同位素值则差异性显著(P < 0.05)。植株水同位素值的季节变化与土壤水含量关系密切,夏季土壤含水量浅层低,深层相对较高,同位素组成随深度增加逐渐贫化,地下水同位素值最低,与植株水同位素低值一致。但与降水量季节变化似乎无直接关系,夏季降水量大,同位素值偏高,与植株水同位素的低值不一致。植株水同位素信息反映了不同水源混合后的同位素组成特征,植株水同位素的季节性变化反映了植被不同生长季利用水源的不同。图3
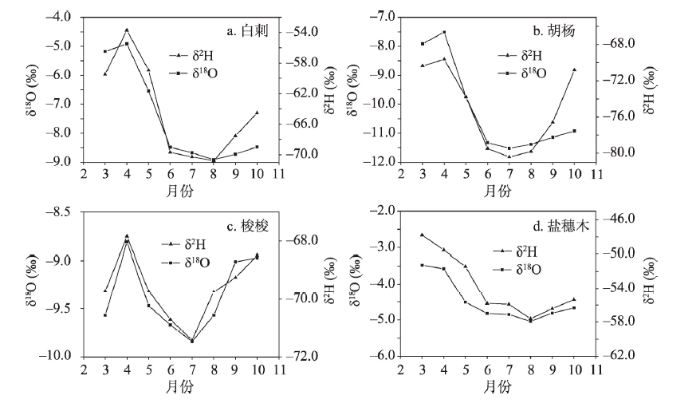
图3不同植被植株水δ2H和δ18O值时间变异特征
Fig. 3The monthly variation characteristics of xylem water δ2H and δ18O in the four plants
3.4 荒漠植被吸收潜在水源比例变化及影响机制
根据植被生境不同,吸水来源包括土壤水、地下水和河水,不同植被对潜在水源利用比例见图4。梭梭植被在整个生长期主要利用地下水,其次利用深层土壤水,几乎不用中间层和浅层土壤水。在夏季月份利用地下水比例最高,6月、7月和8月平均值分别达到了83%、88%和79%,用水范围分别在66%~96%、76%~97%和60%~94%之间。春季和秋季月份利用地下水的比例相比夏季略低,而深层土壤水比例略高,4月利用地下水的比例降低到48%,利用深层土壤水的比例升高到28%,9月利用地下水的比例为59%,利用深层土壤水的比例为26%,可见梭梭植被在夏季主要利用地下水,而春秋季在利用地下水的同时也倾向利用深层土壤水。白刺植被利用水源比例在整个生长季内变化较大,3月和4月主要利用浅层土壤水,贡献率分别为94%和80%,5月在利用表层土壤水的同时逐渐利用中间层土壤水,利用比例分别为28%和41%,6月、7月和8月主要利用水源转移到深层土壤水,且利用地下水的比例逐渐加大,这三个月份利用深层土壤水和地下水的比例分别为33%和29%、36%和34%、31%和27%。到了秋季,利用浅层和中间层土壤水的比例又逐渐升高,9月和10月利用比例分别为23%和33%、27%和36%。可见,白刺植被在不同生长季主要利用水源在浅层、中间层、深层土壤水和地下水之间变换。盐穗木植被在整个生长季利用水源主要集中在浅层和中间层土壤水。3月主要利用20~40 cm层次土壤水,比例为94%,4月利用40~60 cm层次土壤水比例为60%,中间层土壤水利用比例有所升高,比例为20%。到了夏季,浅层土壤水利用比例开始下降,中间层土壤水比例开始升高,6月、7月和8月利用浅层土壤水比例分别为46%、29%和51%,中间层土壤水比例分别为24%、36%和20%。秋季,利用水源以浅层土壤水为主,9月和10月利用比例分别为74%和79%。胡杨植被因为生长在博尔塔拉河岸边,水源利用在土壤水和地下水的基础上可能还直接利用博尔塔拉河水。3月和4月,胡杨主要利用中间层土壤水,利用比例分别为53%和54%,其他层次土壤水、地下水和河水利用比例不太高,其中河水利用比例为11%和11%。5月向其他水源转移,中间层土壤水、深层土壤水、地下水和河水利用比例分别为22%、22%、21%和24%。夏季月份主要利用水源为地下水,6月、7月和8月利用比例分别达到73%、88%和72%,其他水源利用比例很低,河水利用比例为5%、2%和5%。秋季月份,深层次土壤水和地下水利用比例相当,9月和10月利用比例分别为35%、46%和31%、32%,利用河水比例逐渐升高,分别为11%和21%。图4
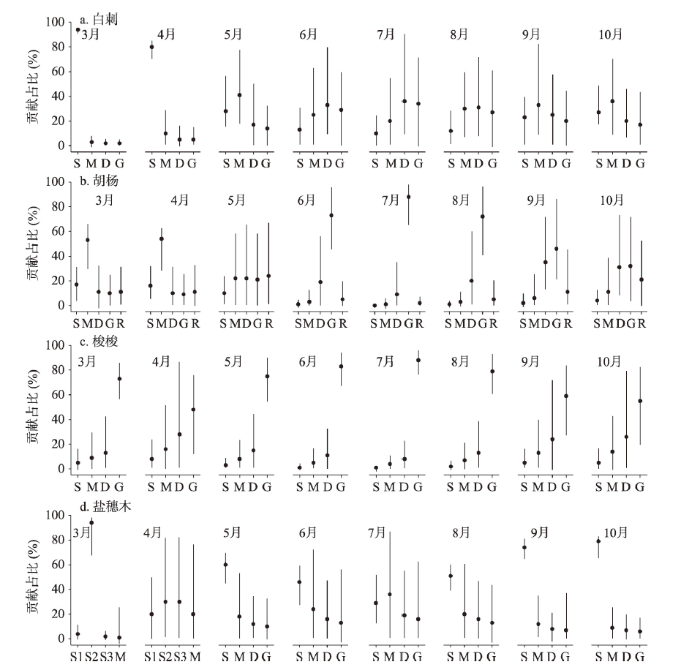
图4植被利用潜在水源占比
注:S1表示0~20 cm土壤水,S2表示20~40 cm土壤水,S3表示40~60 cm土壤水,S表示0~60 cm土壤水,M表示60~140 cm土壤水,D表示140~200 cm土壤水,G表示地下水,R表示河水;圆圈表示平均值,竖线表示水源贡献的最小值到最大值的范围。
Fig. 4Contribution rates of water consumption from different water sources
4 讨论
4.1 环境对荒漠植被水分利用影响
荒漠植被吸收不同水源的比例随生长期发生变化,这说明了植被在不同生长期对水分的需求是不同的,是植被适应干旱区环境变化,特别是可利用水源变化的体现,这是植被与环境协同进化的结果。尽管不同植被在不同生长期利用水源比例不同,但都呈现出相似的变化趋势,即春季由于积雪覆盖及融化,土壤表层含水量较高,荒漠植被会优先利用浅层土壤水,到了夏季,土壤含水量降低,植被(尤其是深根系植被)开始利用深层水源,比如深层次土壤水和地下水,到了秋季,土壤含水量较夏季有所增加,植被利用中间层土壤水比例增加。研究结果同古尔班通古特沙漠南缘的沙漠灌木研究结果是一致的[25]。荒漠植被不同生长季利用不同水源表明植被根系在不同季节对不同水源的吸收上具有选择性,可以通过不同深度根系的快速生长、激活或者休眠的方式来适应可利用水源的季节性转换[21]。不同生长季,土壤温度和含水量不同,在夏季,土壤温度较高(沙丘和盐沼地表层温度达40.5~45.6℃,表5),土壤含水量低(浅层土壤含水量为2.9%~5.2%,表3),在温度和干旱胁迫下可能会导致表层根系休眠或者细根脱水死亡。这种情况下,可能会激发深层土壤根系的生长以吸收利用充足的深层水源。本文中,沙丘生境的梭梭夏季主要利用地下水,盐沼地生境的盐穗木夏季利用中深层次土壤水逐渐增多,荒漠生境的白刺夏季利用水源也转入深层土壤水。因此,在干旱区荒漠植被—水系统中,荒漠植被具有重新分配不同深度根系功能的能力,进而可选择性吸收利用不同的水源供植被生长需要。土壤表层盐渍化程度也能诱导植物可利用水源发生变化[11]。由于强烈的蒸发作用,干旱区土壤表层表现出较高的盐渍化现象,尤其是盐沼地和荒漠生境(表层土壤全盐量分别为38.3 g/kg和26.5 g/kg,表5),为了减小盐胁迫的伤害,盐穗木和白刺通常放弃表层土壤水而选择吸收含盐量较小的深层土壤水,胡杨甚至会选择吸收含盐量更小的河水和地下水。而在低盐渍化区域,比如河岸生境,在春季胡杨较多的吸收利用表层和中层土壤水。Tab. 5
表5
表5不同生境土壤剖面温度、盐度和pH值特征
Tab. 5
生境 | 0~20 cm | 20~40 cm | 40~60 cm | ||||||||
---|---|---|---|---|---|---|---|---|---|---|---|
温度(℃) | pH | 全盐(g/kg) | 温度(℃) | pH | 全盐(g/kg) | 温度(℃) | pH | 全盐(g/kg) | |||
沙丘 | 45.6±3.4 | — | — | 40.3±3.5 | — | — | 32.2±2.9 | — | — | ||
盐沼地 | 40.5±3.6 | 9.33±0.34 | 38.3±5.6 | 36.2±2.9 | 8.92±0.25 | 24.5±3.5 | 29.6±2.1 | 8.85±0.14 | 13.5±2.4 | ||
荒漠 | 33.4±4.4 | 8.82±0.21 | 26.5±4.8 | 27.5±3.2 | 8.73±0.15 | 15.6±4.1 | 23.4±2.6 | 8.75±0.13 | 9.7±1.9 | ||
河岸 | 28.3±2.7 | 8.75±0.23 | 12.2±3.6 | 24.6±2.3 | 8.68±0.17 | 9.3±2.6 | 18.3±1.8 | 8.63±0.15 | 6.4±1.8 |
新窗口打开|下载CSV
植物生长于土壤之中,土壤水是植物水的直接来源,植物根系吸收土壤水过程控制着植物生长和水分状态。地下水是多数荒漠植被重要的水分来源,地下水埋深的变化可以直接影响土壤水分和养分的分布,引起植被对水资源的竞争,从而影响荒漠植被分布、生长及种群演替等群落格局[20],本文区地下水埋深较深,可达到5 m[17],因此深根系植被较容易利用。在本文中,梭梭在整个生长季对地下水利用比例最高,但是在土壤相对湿润的春季,也会利用土壤水。盐穗木主要利用浅层土壤水,特别是在春季,利用比例达94%,夏季利用中间层土壤水的比例增加。白刺同盐穗木相似,春秋季利用浅层土壤水比例最高,但到了夏季主要利用水源转向了深层土壤水。胡杨在整个生长季表现出对地下水的依赖性,尤其在夏季,值得注意的是,胡杨虽然生长在博尔塔拉河岸边,但通过同位素值观测,并没有表现出对河水利用的依赖,对河水利用的比例最大值为24%。
4.2 植被根系分布特征对水分利用影响
植物根系的分布特点决定植物的水分利用策略,植物对水分匮缺的生理响应与植物水分利用策略密切相关,并且和根系功能型一起决定着植物对限制其生存的环境因子的响应与适应特征(图5)。梭梭是深根系植被,拥有庞大的根系,可深达地下水层[21],而且地下水是一种稳定性水源,可满足植被在整个生长季的需求。另外梭梭植被根冠比大,相应的吸收根面积也大,有更多的光合产物分配于根系,这可能是深根系植物利用深层土壤水和地下水的一种必要投资。据研究,白刺直根能达2 m,水平根延伸4~6 m,2/3的总根面积限制在土壤剖面上层40 cm[26]。根据白刺根系分布判断,它能够吸收本文中整个土壤剖面的水分,所以在不同生长季,白刺根据土壤剖面含水量的大小采用了春季优先利用表层土壤水,夏秋季可以利用深层土壤水和地下水的生存策略。盐穗木根系没有找到可参考的资料,但可以参考同一生境且同为小灌木的琵琶柴植被根系,琵琶柴根系分布范围为0~80 cm,总吸收根面积的93%分布在0~60 cm深度[27]。可见,类似盐穗木这类半灌木植被根系分布较浅,利用的水分主要集中在浅层和中间层土壤。所以,在干旱荒漠区,盐穗木植被对盐碱和干旱胁迫的抵抗力也是较强的。据研究,胡杨植被在地下水埋深不低于4 m的河岸生长良好,且具有水分再分配的功能[28]。本文中胡杨在春季主要利用土壤水,可能是因为春季地下水埋深较低,而土壤水分含量较高的原因。夏季随着河水径流增加,对地下水补给增加,地下水上升,胡杨主要利用地下水,河水则利用率始终不太高,说明胡杨采取了多头下注的水分利用策略[29],虽然生境为河岸,因为河水的季节性变化较大,胡杨还是将河水作为一种辅助水源来利用。图5
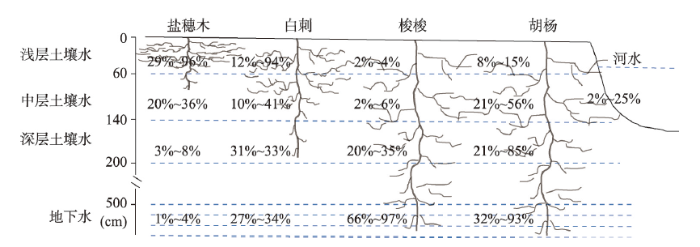
图5典型荒漠植被水分利用来源及比例
Fig. 5Water utilization sources and their proportions of the four desert vegetation species
5 结论
(1)艾比湖流域降水的氢氧稳定同位素组成表现出了显著的季节变化,夏季富集,冬季贫化,春秋季居中的态势。不同生境土壤水氢氧稳定同位素组成均表现为表层富集,随着土壤深度增加而逐渐贫化。(2)植株水氢氧稳定同位素值比较,不同季节均表现出盐穗木最大,其次为白刺和梭梭,胡杨最小。不同植株水氢氧稳定同位素值季节变化基本一致,表现为春季最高,夏季最低,秋季又逐渐增加。
(3)荒漠植被在不同生长期潜在水源及利用比例不同。梭梭植被在整个生长期主要利用地下水。白刺植被利用水源比例在整个生长季内变化较大,春季主要利用表层土壤水,夏季和秋季利用深层土壤水和地下水的比例有所升高。盐穗木植被春季主要利用20~60 cm层次土壤水,夏季利用中间层土壤水比例略有提升,而秋季则又转移利用浅层土壤水。胡杨植被春季主要利用中间层土壤水,夏季主要利用地下水,河水利用比例较低。秋季深层土壤水和地下水利用比例开始上升,河水利用比例也随之升高。荒漠植被根系的分布及水源可利用性决定荒漠植被的水分利用策略。
参考文献 原文顺序
文献年度倒序
文中引用次数倒序
被引期刊影响因子
DOI:10.11821/dlxb201701002 [本文引用: 1]

Changes in hydrological processes and water resources under climate change in the Tianshan Mountains of Central Asia have been investigated based on data analysis and paper review. Under the context of global warming hiatus, temperature of the Tianshan Mountains in Central Asia has been in a state of high variability, which has accelerated the melting of glacier and snow. Warming has resulted in a series of changes in water resources. Snowfall fraction decreased from 11%-24% in 1960-1998 to 9%-21% in 2000-2014, 97.25% of the glaciers retreated in the last 50 years; and water storage decreased at a rate of -3.72 mm/a during 2003-2014. Warming has led to changes in mountainous hydrological processes and water resources. The runoff in the Tianshan Mountains has increased due to the accelerated glacier/snow melt, however, the runoff will decrease in the long term under continuous warming and current precipitation conditions.
[本文引用: 1]
DOI:10.1111/gcb.12785PMID:25359123 [本文引用: 1]

Vegetation in water-limited ecosystems relies strongly on access to deep water reserves to withstand dry periods. Most of these ecosystems have shallow soils over deep groundwater reserves. Understanding the functioning and functional plasticity of species-specific root systems and the patterns of or differences in the use of water sources under more frequent or intense droughts is therefore necessary to properly predict the responses of seasonally dry ecosystems to future climate. We used stable isotopes to investigate the seasonal patterns of water uptake by a sclerophyll forest on sloped terrain with shallow soils. We assessed the effect of a long-term experimental drought (12 years) and the added impact of an extreme natural drought that produced widespread tree mortality and crown defoliation. The dominant species, Quercus ilex, Arbutus unedo and Phillyrea latifolia, all have dimorphic root systems enabling them to access different water sources in space and time. The plants extracted water mainly from the soil in the cold and wet seasons but increased their use of groundwater during the summer drought. Interestingly, the plants subjected to the long-term experimental drought shifted water uptake toward deeper (10-35 cm) soil layers during the wet season and reduced groundwater uptake in summer, indicating plasticity in the functional distribution of fine roots that dampened the effect of our experimental drought over the long term. An extreme drought in 2011, however, further reduced the contribution of deep soil layers and groundwater to transpiration, which resulted in greater crown defoliation in the drought-affected plants. This study suggests that extreme droughts aggravate moderate but persistent drier conditions (simulated by our manipulation) and may lead to the depletion of water from groundwater reservoirs and weathered bedrock, threatening the preservation of these Mediterranean ecosystems in their current structures and compositions. © 2014 John Wiley & Sons Ltd.
DOI:10.1002/hyp.9739URL [本文引用: 1]
DOI:10.1093/treephys/tpn019URL [本文引用: 1]
DOI:10.1002/eco.v8.1URL [本文引用: 1]
DOI:10.1007/s11104-006-9177-1URL [本文引用: 1]
DOI:10.13287/j.1001-9332.201604.005PMID:29732759 [本文引用: 1]

Water is a major limiting factor for plant growth in arid and semi-arid regions. To find out the main sources of water for two artificial sand-fixation plants (Caragana korshinskii and Artemisia ordosica), we analyzed the characteristics of hydrogen and oxygen stable isotopes in water molecules of rainfall, soil water and xylem water. To analyze water sources of these two plants, we used a direct comparison method and a multi-variate mixed linear model. The results showed that an equation of local meteoric water line in Shapotou was δD=7.83δO+5.64 (R=0.91). The value for rainfall δO varied during plant-growing season, which was higher in the beginning and end of growing season, and lower in the peak of growing season. The value for soil water δO in the upper layers changed dramatically. The change range became smaller in the deeper soil layer. C. korshinskii had a greater efficiency (56.1%) in utilizing soil water in 40-80 cm soil layer. A. ordosica had a utilizing efficiency of 56.4% in 20-60 cm soil layer. A week after rain event, C. korshinskii and A. ordosica showed a higher efficiency in upper soil water. C. korshinskii showed an increase of 12.5% in utilizing soil water in 0-40 cm soil layer and A. ordosicas showed an increase of 10% in utilizing soil water in 0-20 cm layer. These results suggested that C. korshinskii and A. ordosica changed their water use strategy after large rainfall events, which might enable them to more easily adapt to arid environment.
PMID:29732759 [本文引用: 1]
[本文引用: 1]
[本文引用: 1]
DOI:10.17521/cjpe.2017.0219 [本文引用: 1]

<i><b>Aims</b></i> The determination of the source of plant water is an important research on the plant-water relationship in arid and semiarid regions and helps to understand the adaptation strategy of desert species to the dry environment. Plant water use pattern affects plant community composition and ecosystem water budget. This study aims to investigate the water use patterns of <i>Caragana microphylla</i> and <i>Artemisia halodendron,</i> two typical shrub species, under altered rainfall conditions in Horqin Sandy Land. <i><b>Methods</b></i> Water treatments include ambient rainfall (natural rainfall), 50% increase in rainfall (enhanced rainfall) and 50% decrease in rainfall (reduced rainfall) by artificially intercepting and redistributing natural rainfall. Stable hydrogen and oxygen isotope ratios (<i>δ</i>D and <i>δ</i><sup>18</sup>O) were measured for xylem water, rainfall, and soil water in different soil layers (0-120 cm depth). The possible ranges of potential water sources used by <i>C. microphylla</i> and <i>A. halodendron </i>were calculated using the IsoSource model. <i><b>Important findings</b></i> 1) Alteration of ambient rainfall mainly affected the soil water condition in the shallow soil (0-30 cm). Increase in rainfall significantly increased the above- and below-ground biomass, and <i>δ</i><sup>18</sup>O values of soil water declined with soil depth. 2) Under the enhanced rainfall treatment, <i>A. halodendron </i>mainly used the soil water in the shallow soil (0-40 cm) and <i>C. microphylla </i>was able to extract water from multiple soil layers. Under the reduced rainfall treatment, both species increasingly relied on extracting water from deeper soil layers, 60-80 cm for <i>A. halodendron</i> and 60-120 cm for <i>C. microphylla</i>. 3) For the natural rainfall treatment, in the wet season, the upper soil water was recharged by rainfall, <i>C. microphylla </i>and <i>A. halodendron </i>extract the shallow soil water (0-60 cm). However, in the dry season, soil water content was dramatically reduced, and main water sources for <i>C. microphylla </i>shifted from topsoil to deeper soil, and <i>A. halodendron </i>can use multiple layers of soil water. In summary, <i>A. halodendron </i>is more capable of exploring deeper soil moisture under reduced rainfall in comparison with <i>C. microphylla,</i> and is likely to be more adaptive to this water-limiting desert environment.
[本文引用: 1]
[本文引用: 1]
[本文引用: 1]
DOI:10.1016/j.scitotenv.2017.10.315URL [本文引用: 2]
DOI:10.1007/s11104-013-1970-zURL [本文引用: 1]
DOI:10.1016/j.agee.2018.06.028URL [本文引用: 1]
DOI:10.13287/j.1001-9332.201707.021PMID:29741036 [本文引用: 1]

Nitraria sibirica usually exists in a form of nebkhas, and has strong ecological adaptability. The plant species has distinctive function for wind prevention and sand fixation, and resistance drought and salt. However, the water condition is still a limiting factor for the plant survival and development. In order to understand the water use strategy of the plant in different desert habitats, we selected the N. sibirica growing in sandy desert habitat and gravel desert habitat to study the seaso-nal variation of plant water sources and response to precipitation at the edge of the oasis of Linze in the Hexi Corridor. We measured the oxygen stable isotope of the plant stem water and the different potential water sources (precipitation, soil water and ground water), and used the IsoSource model to calculate the proportion of water sources from the potential water. The results showed that there were significant seasonal variation characteristics of δO value and water source of stem water for the plant in the two habitats. In the sandy habitat, the plant used more ground water in the less precipitation seasons including spring and fall, and more than 50% of the water sources absorbed from ground water. However, under the condition of gravel habitat, the plant could not achieve the ground water level depth of 11.5 m, and its water source was controlled by precipitation, which had large seasonal variability. The water sources of N. sibirica had significant responses to the change of precipitation in the two desert habitats. Following the rapid decrease of soil water content after the precipitation events, the plant in the sandy habitat turned to use the abundant ground water as the main sources of water, while the plant in the gravel habitat only used the less water from precipita-tion infiltration to the deep soil. Therefore, different water use strategies of the plant in the two habitats were the main reason for the difference in growth characteristics, and it had a strong ability of self-adjustment and adaptation.
PMID:29741036 [本文引用: 1]
[本文引用: 1]
[本文引用: 1]
[本文引用: 1]
[本文引用: 2]
[本文引用: 2]
[D].
[本文引用: 1]
[D].
[本文引用: 1]
DOI:10.1002/rcm.5236URL [本文引用: 1]
PMID:12759813 [本文引用: 2]

Stable isotopes are increasingly being used as tracers in environmental studies. One application is to use isotopic ratios to quantitatively determine the proportional contribution of several sources to a mixture, such as the proportion of various pollution sources in a waste stream. In general, the proportional contributions of n+1 different sources can be uniquely determined by the use of n different isotope system tracers (e.g., delta13C, delta15N, delta18O) with linear mixing models based on mass balance equations. Often, however, the number of potential sources exceeds n+1, which prevents finding a unique solution of source proportions. What can be done in these situations? While no definitive solution exists, we propose a method that is informative in determining bounds for the contributions of each source. In this method, all possible combinations of each source contribution (0-100%) are examined in small increments (e.g., 1%). Combinations that sum to the observed mixture isotopic signatures within a small tolerance (e.g., +/-0.1 per thousand ) are considered to be feasible solutions, from which the frequency and range of potential source contributions can be determined. To avoid misrepresenting the results, users of this procedure should report the distribution of feasible solutions rather than focusing on a single value such as the mean. We applied this method to a variety of environmental studies in which stable isotope tracers were used to quantify the relative magnitude of multiple sources, including (1) plant water use, (2) geochemistry, (3) air pollution, and (4) dietary analysis. This method gives the range of isotopically determined source contributions; additional non-isotopic constraints specific to each study may be used to further restrict this range. The breadth of the isotopically determined ranges depends on the geometry of the mixing space and the similarity of source and mixture isotopic signatures. A sensitivity analysis indicated that the estimated ranges vary only modestly with different choices of source increment and mass balance tolerance parameter values. A computer program (IsoSource) to perform these calculations for user-specified data is available at http://www.epa.gov/wed/pages/models.htm.
DOI:10.1016/j.scitotenv.2018.03.307URL [本文引用: 3]
DOI:10.1007/s11104-014-2342-zURL [本文引用: 1]
DOI:10.3390/w12092414URL [本文引用: 2]
[本文引用: 1]
[本文引用: 1]
[本文引用: 2]
[本文引用: 2]
DOI:10.1002/2015RG000515URL [本文引用: 1]
DOI:10.1007/s11104-017-3332-8URL [本文引用: 1]
DOI:10.1016/j.chnaes.2009.05.009URL [本文引用: 1]
[本文引用: 1]
DOI:10.3724/SP.J.1258.2011.00816 [本文引用: 1]

<p><em>Aims</em> Our objective was to construct and use a Ryel model to quantify hydraulic redistribution of <em>Populus euphratica</em>, a species in desert riparian vegetation.</br><em>Methods</em> We constructed a Ryel model to estimate the amount of net water redistribution under the canopy of <em>P. euphratica</em>. We based the model on data from studies measuring the shape and distribution of roots under the canopy of<em> P. euphratica</em> and observing values for volumetric water contents (<em>θ</em><sub>s</sub>), saturated hydraulic conductivity (<em>K</em><sub>s</sub>), maximum radial soil-root conductance of the entire active root system for water (<em>C</em><sub>RT</sub>), soil water potential (<em>ψ<sub>i</sub></em>) and residual volumetric water contents (<em>θ<sub>r</sub></em>) in five soil layers. We used this model to make a short-term simulation of root hydraulic redistribution in different seasons.</br><em>Important findings</em> Hydraulic redistribution under the canopy of <em>P. euphratica</em> reached a maximum at 2:30 am. The amount of net water redistribution under the canopy decreased and moved towards the underlying soil with changes in the growing season. In June, hydraulic redistribution mainly occurred in the 0–40 cm soil layer, the maximum amount of redistribution was 0.022 0 cm and the total at night was up to 0.111 0 cm. In August, hydraulic redistribution mainly occurred in the 10–70 cm soil layer, the maximum amount of redistribution was 0.006 5 cm and the total at night was up to 0.018 4 cm. In October, hydraulic redistribution mainly occurred in the 70–100 cm soil layer, the maximum amount of redistribution was 0.003 9 cm and the total at night was up to</br>0.008 6 cm.</p>
[本文引用: 1]
DOI:10.1007/s10661-014-3796-4URL