
Partitioning of soil respiration components and evaluating the mycorrhizal contribution to soil respiration in a semiarid grassland
LI Wei-Jing1,2, CHEN Shi-Ping
通讯作者:
编委: 朱彪
责任编辑: 李敏
收稿日期:2018-03-29网络出版日期:2018-08-20
基金资助: |
Received:2018-03-29Online:2018-08-20
Supported by: | SupportedbytheNationalNaturalScienceFoundationofChina( |

摘要
关键词:
Abstract
Methods The mini-trenching mesh method was applied to partition soil respiration components of a semi-arid grassland in Inner Mongolia. A shallow collar (measuring soil total respiration), a deep collar (excluding roots and mycorrhizal hypahe) and a deep collar with 40 μm pore mesh window (excluding roots but not mycorrhizal hyphae) were installed in each plot. Soil respiration rate of each collar was measured every two weeks during the growing season from 2014 to 2016. The differences in the rate of soil respiration among different type of collars were used to partition the components of soil respiration.
Important findings The results showed that the contribution of heterotrophic, root and mycorrhizal respiration to total soil respiration was 49%, 28%, and 23%, respectively. Across the three years, the proportion of mycorrhizal respiration varied from 21%-26%, which is comparable with the results reported by other studies recently. Our results demonstrated that the mini-trenching mesh method is a suitable method for separating mycorrhizal respiration component in grassland ecosystems. Evaluating the contribution of mycorrhizal respiration to total soil respiration is very important for predicting the responses of soil carbon release to future climate change.
Keywords:
PDF (2469KB)摘要页面多维度评价相关文章导出EndNote|Ris|Bibtex收藏本文
引用本文
李伟晶, 陈世苹, 张兵伟, 谭星儒, 王珊珊, 游翠海. 半干旱草原土壤呼吸组分区分与菌根呼吸的贡献. 植物生态学报, 2018, 42(8): 850-862 doi:10.17521/cjpe.2018.0068
LI Wei-Jing, CHEN Shi-Ping, ZHANG Bing-Wei, TAN Xing-Ru, WANG Shan-Shan, YOU Cui-Hai.
土壤呼吸是指由土壤产生并向大气中释放CO2的过程。由于从土壤表面释放的CO2有多种来源, 每个组分都包括不同的生物学和生态学过程, 对环境变化的响应可能也不同, 所以精确区分土壤呼吸的各种组分对于理解土壤呼吸机制本身及其对环境变化的响应是很关键的(骆亦其和周旭辉, 2006)。土壤呼吸可分为自养呼吸(根呼吸)和异养呼吸(微生物呼吸和土壤动物呼吸)。严格意义上的自养呼吸只包括根呼吸, 但由于实际研究中很难将根呼吸和根际微生物呼吸区分开, 并且它们的分解底物均来自于植物的光合作用, 所以通常把根呼吸和根际微生物呼吸划分为自养呼吸, 而共生的菌根是根际微生物的重要组成部分, 因此菌根真菌呼吸属于自养呼吸(Hanson et al., 2000; Heinemeyer et al., 2006; Slavíková et al., 2017), 而异养呼吸主要指土壤有机质的分解产生的呼吸组分。异养呼吸和自养呼吸都有各自不同的驱动因素和环境限制因子, 因此区分土壤呼吸组分对于更好地理解和预测它们各自的动态变化是非常重要的(Neumann & Matzner, 2014)。
已经有一些研究在不同的生态系统采用不同的方法区分土壤呼吸组分以及定量每个组分分别对土壤总呼吸的贡献比例。一些方法是直接测定的, 比如离体根法: 直接测定所获得的活根的CO2释放量(Rakonczay et al., 1997; Li et al., 2008)和同位素法: 通过在植物体内添加C的示踪物来实现(Andrews et al., 1999; Hanson et al., 2000; Kuzyakov & Larionova, 2005), 还有一些间接测定方法, 包括壕沟法(Lee et al., 2003), 环割法: 环剥植物韧皮部, 阻断碳水化合物向根系的输入, 从而阻止根呼吸(Högberg et al., 2001; Bhupinderipal-Singh et al., 2003), 回归法(Xu et al., 2001; Rodeghiero & Cescatti, 2006; Shi & Geng, 2014)等。而最常用的区分自养和异养呼吸组分的方法是壕沟法(Fisher & Gosz, 1986), 后来在一些森林、草原和农田生态系统中, 研究者将此方法进一步改进, 结合网孔膜的应用, 阻止植物根系在环内生长但允许菌根真菌的菌丝长入, 成功地估算了菌根呼吸的大小(Heinemeyer et al., 2007; Moyano et al., 2008; Tomè et al., 2016)。
菌根真菌作为生态系统重要的功能群之一, 在未受干扰的土壤中, 构成了植物根际区微生物的主体, 其生物量约占微生物总量的70% (朱教君等, 2003)。而在自然界中分布最为广泛的菌根类型是丛枝菌根(AM), AM菌根是由球囊菌门真菌与植物形成的互惠共生体, AM真菌能与80%以上的陆地植物形成共生关系, 尤其与绝大多数的草本植物(Smith & Read, 2008; Kernaghan, 2013)。由于AM真菌的菌丝网络非常发达, 在每克土壤中能够延伸到30 m (Cavagnaro et al., 2005; Wilson et al., 2009), 并以0.7-1.0 m·d-1的速率生长着, 通过菌丝网络在土壤中的延伸, 菌根真菌为植物提供了广泛的碳通量流动和营养循环的路径(Leake et al., 2004), 因此菌根真菌在地下碳循环过程中发挥着重要的作用(Talbot et al., 2008)。目前的研究已经发现植物光合固定的碳有4%-26%分配至AM真菌(Jakobsen & Rosendahl, 1990; Drigo et al., 2010), 但是关于菌根真菌在土壤碳释放量方面所做贡献的了解非常有限。已有的关于菌根呼吸定量研究的工作多集中在森林生态系统中(Rygiewicz & Anderson, 1994; Heinemeyer et al., 2007; Nottingham et al., 2010; Neumann & Matzner, 2014; Lilleskov, 2017), 而在草原基于菌根呼吸组分的原位定量研究还十分缺乏(Heinemeyer et al., 2012; Balogh et al., 2016)。在国内关于土壤呼吸组分区分的研究主要集中在自养和异养呼吸组分的区分(Wang & Yang, 2007; Yan et al., 2010; Zhang et al., 2013), 目前关于菌根呼吸组分区分的研究还未见报道。
本研究以内蒙古半干旱草原生态系统为研究对象, 采用Johnson等(2001)所提出的在土壤环上打开窗口安装一定规格的网孔膜排除根系的方法, 区分根呼吸、菌根呼吸和异养呼吸这3个土壤呼吸主要组分, 并通过连续3年土壤呼吸各组分季节和年际变化的测定, 定量它们对土壤总呼吸贡献的比例。
1 材料和方法
1.1 试验样地概况
本研究样地位于内蒙古自治区锡林浩特市西乌珠穆沁旗的半干旱草原(44.37° N, 117.58° E, 海拔1 148 m)。本地区属于中温带半干旱大陆性气候, 根据西乌珠穆沁旗气象站1955-2011年的监测数据(离本研究站点距离约30 km), 该地区年平均气温1.5 ℃, 其中月平均气温最高发生在7月为19.8 ℃, 最低月平均气温发生在1月为-19.0 ℃。年降水量为333 mm, 其中约87%的降水发生在生长季的5-9月份。实验期间(2014-2016年生长季)自然降水量分别为281、290和271 mm。本研究样地于2011年8月开始围封, 围封前为打草场, 每年进行一次打草。该地区天然植被以典型草原为主, 土壤类型为栗钙土, 植物种类主要由多年生C3禾草及杂类草构成。优势植物有大针茅(Stipa grandis)、羊草(Leymus chinensis)和知母(Anemarrhena asphodeloides)等。1.2 研究方法
本实验样方于2012年5月建立, 每个样方大小为3 m × 4 m, 样方间隔为1 m, 总共包含4个重复。在每个样方中嵌入3个不同的土壤环, 用于土壤呼吸组分的区分, 分别为浅环、深环和菌丝环(图1)。它们均是直径为11 cm的聚氯乙烯(PVC)环, 浅环的高度是6 cm, 深环和菌丝环高度是40 cm。在实验测定前1年将土壤环砸入土壤中, 高出地表3 cm左右, 并要注意及时清理土壤环中的绿色植物, 以消除地上植物呼吸部分对土壤呼吸测定的干扰。浅环用于测定土壤总呼吸(SRtot)。研究表明, 本区域大部分(约80%以上)的植物根系分布在土壤30 cm深度范围内(马文红等, 2008), 因而本实验中的深环能够隔断大部分的植物根系并阻断新根长入土壤环内, 因而采用深环测定的土壤呼吸是土壤异养呼吸组分(SRh)。菌丝环的高度和深环相同, 在菌丝环距边缘5 cm处设置3个15 cm × 5 cm大小的窗口, 窗口上贴上孔径为40 μm的尼龙网。有研究表明菌丝直径在10-20 μm之间(Friese & Allen, 1991), 这样尼龙网只允许菌丝通过而植物根系不能通过。因而菌丝环测得的土壤呼吸是土壤异养呼吸与菌丝呼吸(SRmy)之和, 浅环土壤呼吸测定值用SRsc表示, 深环土壤呼吸测定值用SRdc表示, 菌丝环土壤呼吸测定值用SRhc表示, 自养呼吸用SRa表示, 根呼吸用SRr表示。然后利用公式(1)-(3)计算土壤呼吸各个组分。图1
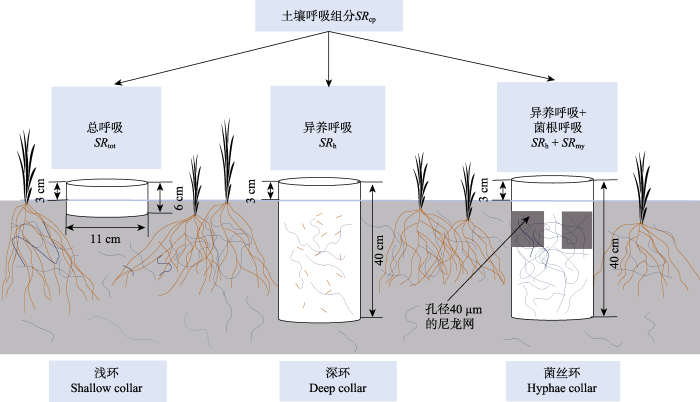
图1改进的深浅环法区分土壤呼吸组分示意图(曲线表示菌丝体, 短线表示碎屑)。浅环用来测定土壤总呼吸, 深环用来测定土壤异养呼吸, 菌丝环用来测定土壤异养呼吸和菌根呼吸的和, 浅环和深环测得的土壤呼吸之差为土壤自养呼吸, 浅环和菌丝环测得的土壤呼吸之差为根呼吸, 菌丝环和深环测得的土壤呼吸之差为菌根呼吸。
Fig. 1Diagram of the modified mini-trenching method in partitioning soil respiration into its components (The curve denotes mycelium and the short line denotes detritus). The shallow and deep collars are used to determine total soil respiration (SRtot) and its heterotrophic component (SRh). Hyphae collar is used for the determination of soil heterotrophic respiration and hyphae respiration (SRh + SRmy). The difference in soil respiration between the shallow and deep collars is used to represent autotrophic soil respiration (SRa), that between the shallow and hyphae collars is used to represent root respiration (SRr), and that between the hyphae and deep collars is used to represent hyphae respiration (SRmy).
采用便携式土壤呼吸自动测定系统(LI-8100, LI-COR, Lincoln, USA)测定土壤呼吸。测定时间为2014-2016年生长季, 每10天一次, 每次测定在8:00-11:00之间进行。同时进行表层土壤(0-10 cm) 的含水量和土壤温度的测定。土壤含水量由便携式TDR-300 (Spectrum Technologies, Plainfield, USA)测定, 土壤温度由LI-8100附带的土壤热电偶探头(LI-8100-201)测定。为了减少土壤温度和水分测定对环内土壤造成的扰动, 深环和菌丝环的环境指标测量仅在2014年生长季进行, 并建立其与浅环土壤温度和水分之间的关系。2015和2016年深环和菌丝环的土壤温度和土壤水分通过其与浅环之间的相关关系方程计算得到。
在2016年生长季旺季8月中旬, 分别在每个样方的浅环、深环和菌丝环采集0-10 cm的土壤样品, 总共有4个重复, 用于菌丝密度的测定。参照Jakobsen等(1992)和Rillig等(1999)的方法进行AM真菌菌丝的提取。具体步骤如下: 称取4 g新鲜的土壤样品溶于100 mL蒸馏水中并加入5 mL 35 g·L-1偏磷酸钠溶液, 振荡土壤悬浮液30 s, 使混合均匀, 然后静置30 min将上清液过400目筛进行过滤。将筛上的残留物用蒸馏水转移到250 mL三角瓶中, 并定容至200 mL。摇动瓶身5 s后静置1 min, 用移液器取2 mL悬浮液进行抽滤, 使之转入25 μm的微孔滤膜上。将滤膜取下(有菌丝的一面朝上)转移到载玻片上, 用酸性品红染色1 h后置于200倍显微镜下进行观察, 并根据菌丝颜色和形态区分AM真菌菌丝和非AM真菌菌丝(Miller et al., 1995)。
1.3 数据统计分析
运用SPSS 21.0软件对数据进行统计分析, 为了解不同土壤呼吸环处理间是否存在显著性差异, 对不同土壤呼吸环内的土壤温度、土壤水分和菌丝密度进行单因素方差分析, 采用最小显著差数法(LSD)进行多重比较。在本研究中土壤呼吸及其组分的季节平均值是由生长季5-8月份所有测定结果平均值得来。采用相关分析研究土壤呼吸及其组分与土壤温度以及土壤水分之间的关系, 采用多元回归分析构建土壤呼吸与土壤温度和土壤水分的拟和方程, 所有检验显著性水平设定为p < 0.05, 由统计分析软件SAS 9.2完成。文中的图采用SigmaPlot 12.5绘制。2 结果
2.1 不同土壤呼吸环对菌丝密度的影响
方差分析结果表明, 浅环、深环和菌丝环内AM菌根真菌菌丝密度有显著差别(图2, p < 0.05), 其中菌丝环内的菌丝密度最高, 为2.83 m·g-1干土, 而深环土壤中的菌丝密度最低,为0.76 m·g-1干土。图2
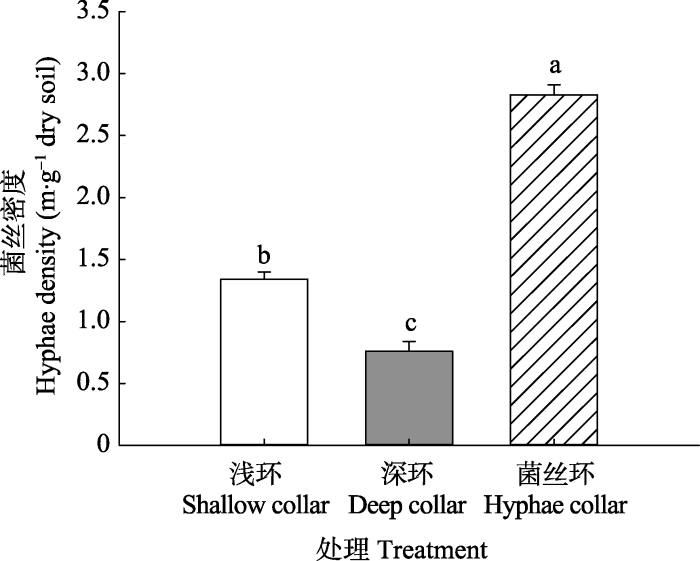
图2不同土壤呼吸环内的菌丝密度(平均值+标准误差)。不同小写字母表示处理间差异显著(p < 0.05)。
Fig. 2Hyphae density under different soil respiration collars (mean + SE). Different letters indicate significant differences among treatments (p < 0.05).
2.2 不同土壤呼吸环对土壤温度、水分的影响
由2014年5、6月份的浅环、深环和菌丝环的土壤温度季节变化和方差分析结果表明: 浅环、深环和菌丝环内的土壤温度没有显著性差异(图3A、3B, p = 0.16), 它们均和浅环内的土壤温度呈显著的线性关系(图4A、4C)。不同土壤环内的土壤含水量存在显著性差异(图3C、3D, p < 0.01)。与浅环相比, 深环和菌丝环内的土壤表层含水量分别显著提高了38%和33%, 且深环和菌丝环内的土壤水分含量分别和浅环内的水分含量呈幂指数关系(y = 4.95x0.50; y = 4.71x0.51), 且相关性较高, 分别为0.77和0.78 (图4B、4D)。图3
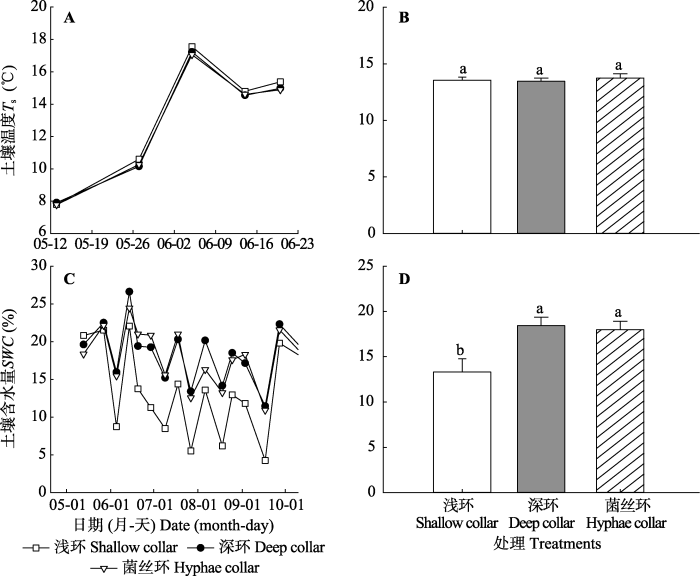
图32014年不同土壤呼吸环内的土壤温度(Ts)、土壤含水量(SWC)的季节动态(A, C)和年平均值(B, D)(平均值+标准误差)。不同小写字母表示处理间差异显著(p < 0.05)。
Fig. 3Seasonal variations of soil temperature (Ts), soil water content (SWC) under different soil respiration collars during 2014 (A, C) and average from the year 2014 (B, D)(mean + SE). Different lowercase letters indicate significant differences among treatments (p < 0.05).
图4
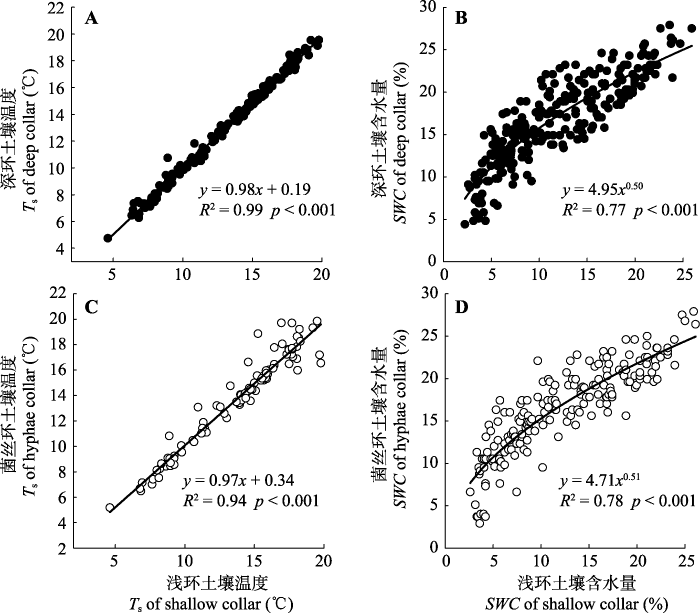
图4不同土壤环内土壤温度之间的关系(A, C)以及不同土壤环内土壤含水量之间的关系(B, D)。
Fig. 4The relationship between soil temperature (Ts) in different soil collars (A, C) and the relationship between soil water content (SWC) in different soil collars (B, D).
2.3 土壤呼吸组分的区分与校正
通过测定浅环、深环和菌丝环的土壤呼吸以及土壤温度和含水量, 2014-2016三年的数据表明浅环、深环和菌丝环的土壤呼吸分别与土壤温度呈幂指数关系(图5), 与土壤含水量呈二次函数关系(图6)。图5
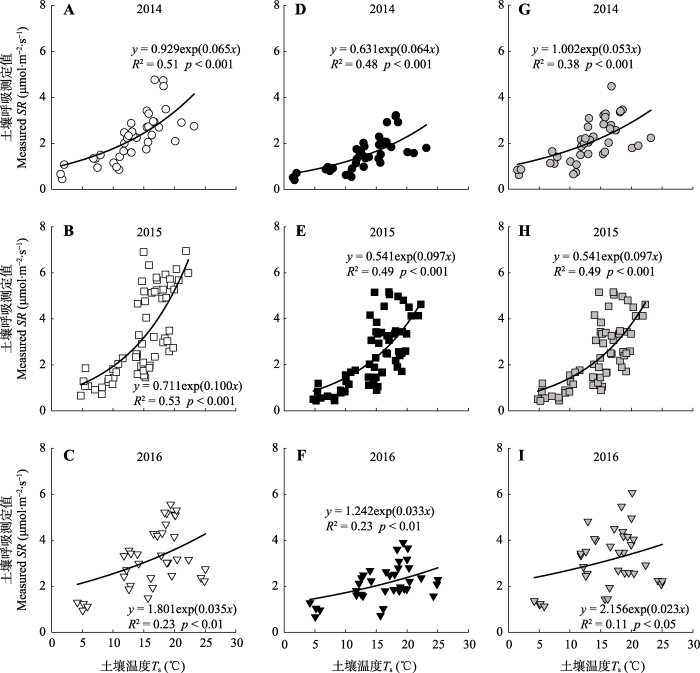
图52014-2016年不同土壤呼吸环测得的土壤呼吸与土壤温度之间的关系。A, B, C, 浅环; D, E, F, 深环; G, H, I, 菌丝环。
Fig. 5The relationship between soil respiration (SR) and soil temperature (Ts) in different soil respiration collars during 2014-2016. A, B, C, Shallow collar. D, E, F, Deep collar. G, H, I, Hyphae collar.
图6
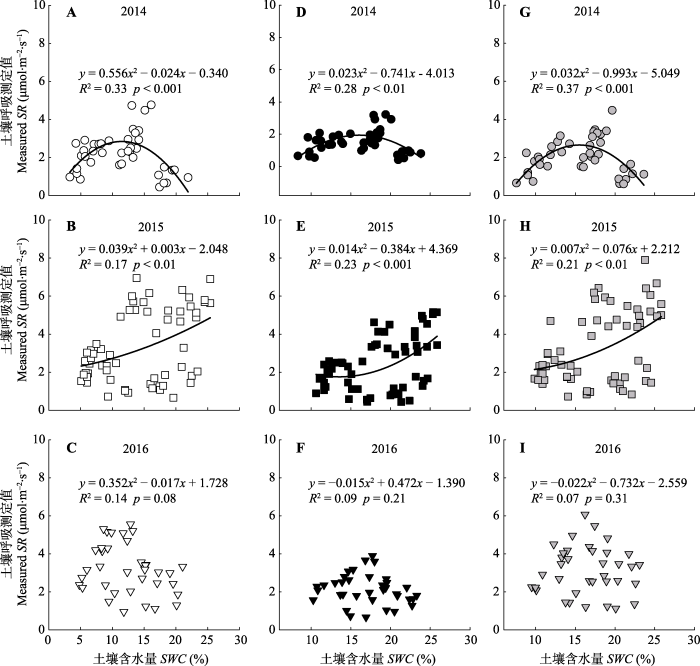
图62014-2016年不同土壤呼吸环测得的土壤呼吸与土壤含水量之间的关系。A, B, C, 浅环; D, E, F, 深环; G, H, I, 菌丝环。
Fig. 6The relationship between soil respiration (SR) and soil water content (SWC) in different soil respiration collars during 2014-2016. A, B, C, Shallow collar. D, E, F, Deep collar. G, H, I, Hyphae collar.
为了消除土壤环境变化对深环所测得土壤呼吸(SRdc)与菌丝环所测得土壤呼吸(SRhc)的影响, 本研究采用一个幂指数-二次方程来模拟它们与土壤温度(Ts)和土壤含水量(SWC)之间的关系(Saiz et al., 2007; Yan et al., 2010)。
其中SRdc为深环土壤呼吸测定值, SRhc为菌丝环土壤呼吸测定值, Ts为土壤温度, SWC为土壤水分。a1-d1和a2-d2为方程模拟出来的参数。通过这一过程得到SRdc与Ts和SWC之间的关系以及SRhc与Ts和SWC之间的关系(表1), 然后将浅环的Ts和SWC代入表1的方程中, 即可求算出与浅环土壤环境相同的异养呼吸组分SRh。将其与浅环中测得的SRtot求差, 即可得到自养呼吸组分SRa。同理, 将浅环的Ts和SWC代入菌丝环土壤呼吸拟和方程中, 即可求算出与浅环土壤环境相同的异养呼吸SRh与菌丝呼吸SRmy之和, 利用公式(2), 将其与浅环中测得的SRtot求差, 即可得到自养呼吸中的根系呼吸组分SRr, 利用公式(3), 将其与深环测得的SRh求差, 即可求得菌丝呼吸组分SRmy。
Table 1
表1
表1浅环(SRsc)、深环(SRdc)和菌丝环呼吸(SRhc)实测值与土壤温度(Ts)和含水量(SWC)拟和方程及相关参数(N, R2和p值)
Table 1
年份 Year | 土壤环类型 Collar type | 样本数量 N | 方程 Function | R2 | p |
---|---|---|---|---|---|
2014 | 浅环 Shallow collar | 64 | Y = 0.032e0.098Ts(-0.084SWC2 + 2.70SWC) | 0.82 | < 0.000 1 |
深环 Deep collar | 64 | Y = 0.011e0.110Ts(0.032SWC2 + 1.20SWC) | 0.75 | < 0.000 1 | |
菌丝环 Hyphae collar | 64 | Y = 0.011e0.099Ts(0.082SWC2 + 2.00SWC) | 0.72 | < 0.000 1 | |
2015 | 浅环 Shallow collar | 60 | Y = 0.020e0.109Ts(-0.037SWC2 + 2.90SWC) | 0.87 | < 0.000 1 |
深环 Deep collar | 60 | Y = 0.011e0.108Ts(0.043SWC2 + 1.55SWC) | 0.86 | < 0.000 1 | |
菌丝环 Hyphae collar | 60 | Y = 0.009e0.113Ts(0.045SWC2 + 2.65SWC) | 0.89 | < 0.000 1 | |
2016 | 浅环 Shallow collar | 36 | Y = 0.095e0.053Ts(-0.090SWC2 + 2.40SWC) | 0.44 | < 0.000 1 |
深环 Deep collar | 36 | Y = 0.032e0.049Ts(-0.064SWC2 + 2.90SWC) | 0.37 | < 0.000 1 | |
菌丝环 Hyphae collar | 36 | Y = 0.061e0.043Ts(-0.043SWC2 + 2.30SWC) | 0.28 | < 0.000 1 |
新窗口打开|下载CSV
2.4 深环和菌丝环土壤呼吸观测值和校正值的季节动态
2014-2016年深环和菌丝环校正后的土壤呼吸, 与实测值相比降低了(图7)。2014年深环土壤呼吸校正值与实测值相比平均降低了0.54 μmol·m-2·s-1, 菌丝环的土壤呼吸速率平均降低了0.72 μmol·m-2·s-1, 2015年深环和菌丝环实测的土壤呼吸校正值与实测值相比分别平均降低了0.68和0.78 μmol·m-2·s-1, 2016年两者分别平均降低了0.41和0.57 μmol·m-2·s-1, 3年中菌丝环的土壤呼吸校正值与实测值的平均差值比深环的更大, 且最大差值达1.95 μmol·m-2·s-1。图7
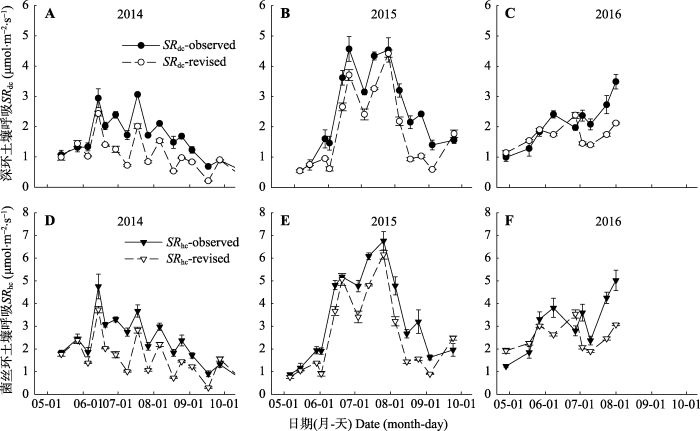
图72014-2016年深环和菌丝环土壤呼吸的观测值与校正值的季节动态(平均值±标准误差)。SRdc-observed、SRdc-revised、SRhc-observed、SRhc-revised分别表示深环土壤呼吸的观测值、深环土壤呼吸的校正值、菌丝环土壤呼吸的观测值、菌丝环土壤呼吸的校正值。
Fig. 7The seasonal dynamics of the observed value of soil respiration and the revised value of deep collar and hyphae collar (mean ± SE). SRdc-observed, SRdc-revised, SRhc-observed and SRhc-revised represent the observed value of soil respiration in the deep collar, the revised value of soil respiration in the deep collar, the observed value of soil respiration in the hyphae collar, and the revised value of soil respiration in the hyphae collar, respectively.
2.5 校正后土壤呼吸各组分的季节动态
在2014-2016年生长季中, 土壤异养呼吸和自养呼吸以及自养组分中的根呼吸和菌根呼吸均呈现出明显的季节变化, 其峰值一般出现在生长季旺季的7-8月份(图8), 最小值一般出现在生长季的初期和末期, 在3年中土壤总呼吸的最大值为6.2 μmol·m-2·s-1, 异养呼吸的最大值为3.71 μmol·m-2·s-1, 自养呼吸的最大值为3.11 μmol·m-2·s-1, 根呼吸和菌根呼吸的最大值分别为2.48和1.56 μmol·m-2·s-1, 菌根呼吸的最小值为0.09 μmol·m-2·s-1。图8
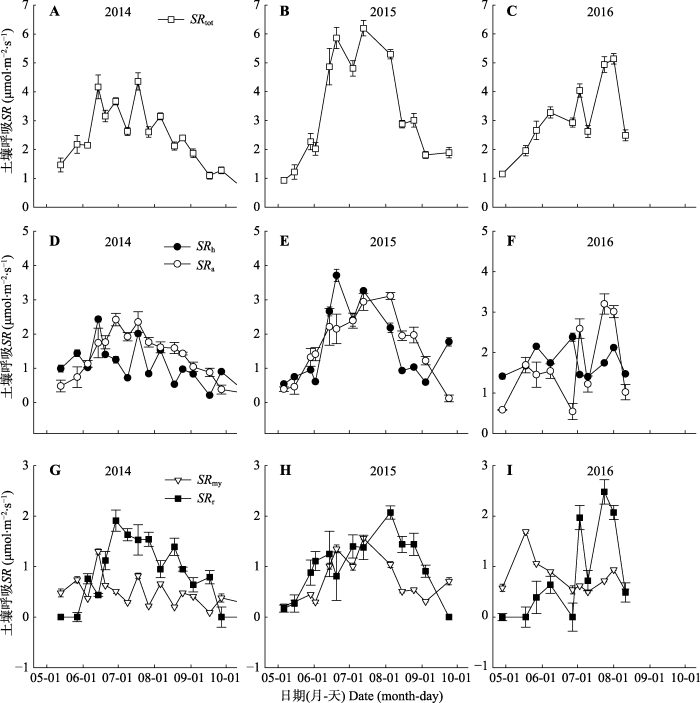
图82014-2016年生长季中不同土壤呼吸组分的季节变化(平均值±标准误差)。SRh、SRa、SRmy、SRr分别表示异养呼吸、自养呼吸、菌根呼吸、根呼吸。
Fig. 8Seasonal variations of different soil respiration components during growing seasons of 2014-2016 (mean ± SE). SRh, SRa, SRmy and SRr represent heterotrophic respiration, autotrophic respiration, mycorrhiza respiration and root respiration, respectively.
2.6 土壤呼吸各个组分以及所占比例的年际变化
土壤呼吸各个组分呈现出明显的年际波动(图9), 并且在2014到2016年, 异养呼吸(SRh)的变化范围是1.29-2.04 μmol·m-2·s-1,菌根呼吸(SRmy)的变化范围是0.61-0.86 μmol·m-2·s-1, 根呼吸(SRr)的变化范围是0.63-0.98 μmol·m-2·s-1。图9
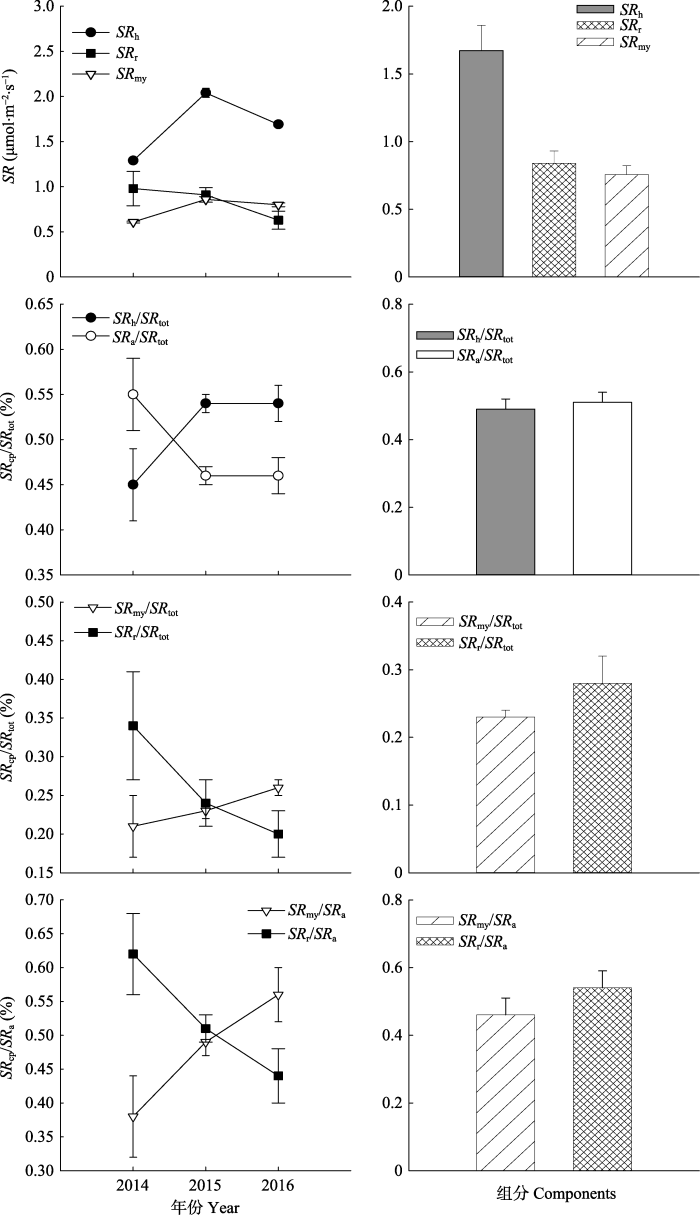
图9土壤呼吸(SR)及其各组分的年际变化(2014-2016)和3年平均值(2014-2016年5-8月份)以及土壤呼吸各组分占总呼吸的比例和菌根呼吸、根呼吸分别占自养呼吸的比例的年际变化和3年平均值(2014-2016年5-8月份)(平均值±标准误差)。SRh、SRa、SRmy、SRr、SRtot分别表示异养呼吸、自养呼吸、菌根呼吸、根呼吸和总呼吸。
Fig. 9Interannual variations of soil respiration (SR) and its components, the contribution of soil respiration components to total soil respiration and the contribution of SRmy and SRr to SRa during 2014-2016 and averages from May to August of the 3 years (mean ± SE). SRh, SRa, SRmy, SRr and SRtot represent heterotrophic respiration, autotrophic respiration, mycorrhiza respiration, root respiration and total soil respiration, respectively.
在2014-2016年, 土壤呼吸各个组分占总呼吸的比例呈现明显的年际变化(图9)。异养呼吸占总呼吸的比例(SRh/SRtot)年均值为51%, 菌根呼吸占总呼吸的比例(SRmy/SRtot)年均值为23%, 根呼吸占总呼吸的比例(SRr/SRtot)年均值为26%, 在2014-2016年菌根呼吸占自养呼吸的比例(SRmy/SRa)年均值为48%, 根呼吸占自养呼吸的比例(SRr/SRa)年均值为52%。
3 讨论
为了区分土壤呼吸组分并定量菌根呼吸在土壤碳释放中的贡献, 本研究采用改进的深浅环网孔法, 通过设置深环和菌丝环, 隔离根系并允许菌丝生长。结果表明深环的嵌入阻止了根系和菌丝进入, 深环土壤中菌丝密度显著低于环外土壤, 而菌丝环因为设置了允许菌丝进入的窗口, 其环内土壤的菌丝密度显著高于环外土壤。以上结果说明菌丝环达到了隔离根系而允许菌根真菌菌丝进入的预定目标。虽然深环和菌丝环达到了隔离菌丝与根系的目的, 但是同时也阻止了土壤水分和能量的侧向交换, 结果表明, 不同类型的土壤呼吸环(浅环、深环和菌丝环)的放置虽没有影响环内土壤温度, 但导致深环和菌丝环内的土壤含水量显著提高, 这可能由于深环和菌丝环的嵌入隔断了植物的根系, 缺少了植物水分的消耗, 改变了环内土壤的水分状况, 同时缺少侧向水分交换使得深环和菌丝环内一直保持较高的土壤水分状况。在半干旱草原地区, 水分和温度是土壤呼吸的最主要限制因子。由于深环和菌丝环内含水量的提高, 使得深环和菌丝环内的土壤呼吸增强, 从而造成对异养呼吸和菌根呼吸组分的高估。本研究中除2016年土壤呼吸与土壤含水量的关系不显著外, 土壤呼吸及其组分与土壤温度和土壤含水量均表现出显著的相关关系, 因此在深环和菌丝环土壤呼吸的校正模型中同时考虑了土壤温度和土壤含水量两个因子。模型的结果表明, 与仅考虑单个因子相比, 同时考虑温度和水分两个因子明显提高了模型的解释度。通过建立土壤呼吸测量值与土壤温度和水分拟和方程, 采用浅环内土壤温度和水分测量值重新计算深环和菌丝环土壤呼吸。校正后深环和菌丝环土壤呼吸速率比测量值分别下降了27%和21%。采用校正值计算出土壤呼吸各个呼吸组分所占比例。通过2014-2016三个生长季的连续测定, 表明内蒙古半干旱草原异养呼吸占土壤总呼吸的比例为45%-54%, 根呼吸占土壤总呼吸的20%-34%, 菌根呼吸占土壤总呼吸的21%-26%。与国内外研究(表2)相比, 这和Heinemeyer等(2012)的研究结果是相似的, 他们在温带草原, 利用相似的研究方法得到根呼吸对土壤总呼吸的贡献是10%-30%, 菌根呼吸对土壤总呼吸的贡献是27%。而Balogh等(2016)研究了干旱草原在干旱条件下, 菌根呼吸对土壤总呼吸的贡献是(13 ± 8)%。Grimoldi等(2006)利用稳定同位素技术, 发现多年生黑麦草的根被AMF侵染后, 土壤中的CO2通量增加了16%。在森林生态系统中, Neumann和Matzner (2014)通过内生长网袋法发现, 在生长季菌根呼吸对土壤呼吸的贡献是18%-44%。Heinemeyer等(2007)和Lilleskov (2017)通过改进的深浅环法发现, 菌根呼吸对土壤呼吸的贡献分别是25%和28%。利用分室系统隔离装置, Rygiewicz和Andersen (1994)及Nottingham等(2010)表明菌根呼吸对土壤总呼吸的贡献分别为19.4%和(14 ± 6)%。在农田中, Tomè等(2016)在苹果园利用同位素技术发现菌根呼吸的贡献是11.6%, Moyano等(2007)通过尼龙网袋法发现菌根呼吸的贡献是25.3%。这些不同的结果可能与实验过程中不同的菌根和细根生物量有关, 而这些是受土壤因素所影响的, 比如土壤水分(Tedersoo et al., 2014), 土壤温度(Tomè et al., 2016), 可利用的营养(Koorem et al., 2014; Lauber et al., 2008)。已有的这些研究表明在不同的生境, 不同的实验方法有关菌根呼吸对总呼吸的贡献估计有所不同, 而对于半干旱草原此方面的研究较少需要进一步的探索。
Table 2
表2
表2菌根呼吸组分对土壤总呼吸贡献比例的相关研究结果
Table 2
生态系统 Ecosystems | 地点 Location | 优势物种 Dominant species | 方法 Methods | 菌根呼吸贡献率 Mycorrhizal contribution (%) | 文献来源 Sources |
---|---|---|---|---|---|
森林 Forest | 德国东南部 Southeastern Germany | 欧洲云杉 Picea abies | 内生长网孔袋法 In growth mesh bags (2 mm and 45 μm) | 18%-44% | Neumann & Matzner, 2014 |
英国约克南部 South of York, UK 53.91° N, 1.00° W | 美国黑松 Pinus contortaDouglas ex Loudon 垂枝桦 Betula pendula | 菌根网孔环设计 Mycorrhizal mesh collar design (41 μm) | 25% | Heinemeyer et al., 2007 | |
美国 宾夕法尼亚州 Pennsylvania, USA | 西黄松 Pinus ponderosa | 根模拟系统和同位素方法 Root-mycocosm and isotopic method | 19.4% | Rygiewicz & Andersen, 1994 | |
巴拿马共和国 The Republic of Panama | Pseudobombax septenatum | 内生长模拟系统 In-growth mesocosms | (14 ± 6)% | Nottingham et al., 2010 | |
德国中部 Central Germany 51.08° N, 10.45° E | Fagus sylvatica 欧洲云杉 Picea abies | 应用不同规格孔径的 尼龙网袋土钻法 Soil cores using nylon mesh bags of 35 and 1 μm pore size | 3%, 8% | Moyano et al., 2008 | |
美国密歇根霍顿 Houghton, Michigan, USA 47.12° N, 88.55° W | 北美乔松 Pinus strobus, Quercus rubra | PVC环和模型 PVC collar and models | 28% | Lilleskov, 2017 | |
草原 Grassland | 匈牙利 Hungary 46.69° N, 19.60° E | Festuca pseudovina, Carex stenophylla, 狗牙根 Cynodon dactylon | 物理分离(40 μm不锈钢网)和 同位素方法 Physical separation: (40 μm inox mesh) and isotopic method | (13 ± 8)% | Balogh et al., 2016 |
英国约克郡北部 North Yorkshire, UK 54.00° N, 1.18° W | 绒毛草 Holcus lanatus | 网孔环嵌插法 Meshcollar insertion (20 μm) | 27% | Heinemeyer et al., 2012 | |
农田 Farmland | 意大利北部 Northern Italy 47.30° N, 11.58° E | Malus domestica | 尼龙膜结合同位素法 Polyester membrane (5 μm and 50 μm) with isotopic method | 11.6% | Tomè et al., 2016 |
德国图林根 Thuringia, Germany 51.10° N, 11.77° E | 大麦 Hordeum vulgare | 尼龙网孔袋法 Nylon mesh bags (1 μm and 35 μm) | 25.3% | Moyano et al., 2007 |
新窗口打开|下载CSV
综上所述, 本研究在内蒙古半干旱草原区分土壤呼吸组分所采用的深浅环网孔法, 操作简便, 资金投入少, 且是原位研究, 对土壤的干扰相对较小, 很适合野外研究实验。并且3年较长时间的土壤呼吸组分的分离研究, 为各个组分对土壤呼吸的贡献提供了一个较为准确的范围, 异养呼吸对总呼吸的贡献比例为51%, 根呼吸的贡献比例为26%, 菌根呼吸的贡献比例为23%。与国内外研究相比, 由于不同的生态系统类型, 不同的研究方法, 菌根呼吸对土壤呼吸的贡献有一定的差异性, 但是本研究能够将菌根呼吸对土壤呼吸的贡献限定在一个相对准确的范围内, 有助于我们更好地理解草原地下碳循环过程, 并预测其对未来气候的响应。
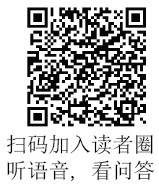
致谢
感谢中国科学院植物研究所孙秀峰老师在实验工作中给予的指导。参考文献 原文顺序
文献年度倒序
文中引用次数倒序
被引期刊影响因子
DOI:10.2136/sssaj1999.6351429xURL [本文引用: 1]
DOI:10.5194/bg-13-5171-2016URL [本文引用: 2]

Summer droughts projected to increase in central Europe due to climate changes strongly influence the carbon cycle of ecosystems. Persistent respiration activities during drought periods are responsible for a significant carbon loss, which may turn the ecosystem from a sink into a source of carbon. There are still gaps in our knowledge regarding the characteristic changes taking place in the respiration of the different components of the ecosystem in response to drought events.<br><br>In the present study, we combined a physical separation of soil respiration components with continuous measurements of soil CO2 efflux and its isotopic (13C) signals at a dry grassland site in Hungary. The physical separation of soil respiration components was performed by means of inox meshes and tubes inserted into the soil. The root-excluded and root- and mycorrhiza-excluded treatments served to measure the isotopic signals of the rhizospheric, mycorrhizal fungi and heterotrophic components, respectively.<br><br>In the dry grassland investigated in the study the three components of the soil CO2 efflux decreased at different rates under drought conditions. During drought the contribution made by the heterotrophic components was the highest (54????????8???%; mean ??SE). Rhizospheric component was the most sensitive to soil drying with its relative contribution to the total soil respiration dropping from 66????????7 (non-stressed) to 35????????17???% (mean ??SE) under drought conditions. According to our results the heterotrophic component of soil respiration is the major contributor to the respiration activities during drought events in the dry grassland ecosystem studied.
DOI:10.1046/j.1365-3040.2003.01053.xURL [本文引用: 1]

Limitations in available techniques to separate autotrophic (root) and soil heterotrophic respiration have hampered the understanding of forest C cycling. The former is here defined as respiration by roots, their associated mycorrhizal fungi and other micro-organisms in the rhizosphere directly dependent on labile C compounds leaked from roots. In order to separate the autotrophic and heterotrophic components of soil respiration, all Scots pine trees in 900 m 2 plots were girdled to instantaneously terminate the supply of current photosynthates from the tree canopy to roots. H gberg et al . ( Nature 411, 789 792, 2001) reported that autotrophic activity contributed up to 56% of total soil respiration during the first summer of this experiment. They also found that mobilization of stored starch (and likely also sugars) in roots after girdling caused an increased apparent heterotrophic respiration on girdled plots. Herein a transient increase in the 13 C of soil CO 2 efflux after girdling, thought to be due to decomposition of 13 C-enriched ectomycorrhizal mycelium and root starch and sugar reserves, is reported. In the second year after girdling, when starch reserves of girdled tree roots were exhausted, calculated root respiration increased up to 65% of total soil CO 2 efflux. It is suggested that this estimate of its contribution to soil respiration is more precise than the previous based on one year of observation. Heterotrophic respiration declined in response to a 20-day-long 6 C decline in soil temperature during the second summer, whereas root respiration did not decline. This did not support the idea that root respiration should be more sensitive to variations in soil temperature. It is suggested that above-ground photosynthetic activity and allocation patterns of recent photosynthates to roots should be considered in models of responses of forest C balances to global climate change.
DOI:10.1111/j.1365-3040.2005.01310.xURL [本文引用: 1]

Most terrestrial plant species form associations with arbuscular mycorrhizal fungi (AMF) that transfer soil P to the plant via their external hyphae. The distribution of nutrients in soils is typically patchy (heterogeneous) but little is known about the ability of AMF to exploit P patches in soil. This was studied by growing symbioses of Linum usitatissimum and three AMF ( Glomus intraradices , G. mosseae and Gigaspora margarita ) in pots with two side-arms, which were accessible to hyphae, but not to roots. Soil in one side-arm was either unamended (P0) or enriched with P; simultaneous labelling of this soil with 32 P revealed that G. intraradices responded to P enrichment both in terms of hyphal proliferation and P uptake, whereas the other AMF did not. Labelling with 33 P of P0 soil in the other side arm revealed that the increased P uptake by G. intraradices from the P-enriched patch was paralleled by decreased P uptake by other parts of the mycelium. This is the first demonstration of variation in growth and nutrient uptake by an AMF as influenced by a localized P enrichment of the soil. The results are discussed in the context of functional diversity of AMF.
DOI:10.1073/pnas.0912421107URLPMID:20534474 [本文引用: 1]

Rising atmospheric CO60 levels are predicted to have major consequences on carbon cycling and the functioning of terrestrial ecosystems. Increased photosynthetic activity is expected, especially for C-3 plants, thereby influencing vegetation dynamics; however, little is known about the path of fixed carbon into soil-borne communities and resulting feedbacks on ecosystem function. Here, we examine how arbuscular mycorrhizal fungi (AMF) act as a major conduit in the transfer of carbon between plants and soil and how elevated atmospheric CO60 modulates the belowground translocation pathway of plant-fixed carbon. Shifts in active AMF species under elevated atmospheric CO60 conditions are coupled to changes within active rhizosphere bacterial and fungal communities. Thus, as opposed to simply increasing the activity of soil-borne microbes through enhanced rhizodeposition, elevated atmospheric CO60 clearly evokes the emergence of distinct opportunistic plant-associated microbial communities. Analyses involving RNA-based stable isotope probing, neutral/phosphate lipid fatty acids stable isotope probing, community fingerprinting, and real-time PCR allowed us to trace plant-fixed carbon to the affected soil-borne microorganisms. Based on our data, we present a conceptual model in which plant-assimilated carbon is rapidly transferred to AMF, followed by a slower release from AMF to the bacterial and fungal populations well-adapted to the prevailing (myco-)rhizosphere conditions. This model provides a general framework for reappraising carbon-flow paths in soils, facilitating predictions of future interactions between rising atmospheric CO60 concentrations and terrestrial ecosystems.
DOI:10.1007/BF00638959URL [本文引用: 1]

Trenching was used to reduce root activity in treeless plots in a New Mexico mixed-conifer forest to examine the effects of plant roots on soil processes. Trenching led to increases in moisture content (104%), inorganic N concentration (115%), and mass loss from cellulose (196%). In laboratory incubations, trenched soils collected in the 1st and 2nd year after trenching evolved 52% and 115% more CO 2 , respectively, than control soils. Amending incubated trenched and control soils with moisture and inorganic N indicated that increased soil moisture content in trenched plots could explain the increased microbial activity. Trenching also had statistically significant but inconsistent effects on net N mineralization in incubated soils. The greatest effect of trenching was to increase net N mineralization under favorable temperature and moisture conditions. Irrigation of field plots increased both CO 2 evolution and net N mineralization. Overall, these data are consistent with the hypothesis that plant roots reduced microbial activity by moisture uptake during the time of the study.
DOI:10.2307/3760351URL [本文引用: 1]

Different forms of vesicular-arbuscular mycorrhizal fungal inoculum are found in soil patches with differing histories. These inoculum types include spores, infected root fragments, and extramatrical hyphae. Utilizing root observation chambers, detailed observations were made of the growth dynamics of mycorrhizal hyphae from inoculum sources to roots and the subsequent radiation out from individual colonized root segments. There were several types of hyphal architecture classified by whether the external hyphae were entering (infecting) a plant root or developing from the root surface and growing out into the soil matrix. Morphologically, individual hyphal filaments within all architectural types looked quite similar and clear architectural differences could only be identified when distinct sets of interconnected hyphal filaments were studied. The primary hyphal architectural types observed in the soil were classified as runner hyphae, hyphal bridges, absorptive hyphal networks, germ tubes, and infection networks produced by spores and root fragments. The specialized hyphal architectures of these fungal endophytes appear to be linked to the unique function of each hyphal type. Runner hyphae and infection networks from either spores or root fragments were observed to be capable of infecting new root segments. Absorptive hyphal networks were never observed to act as units of infection and were classified as structures primarily involved in the acquisition of soil resources. This high degree of specialization may help explain why vesicular-arbuscular mycorrhizal fungi are so efficient in their beneficial role of nutrient and water transport to the host plant.
DOI:10.1111/nph.2006.172.issue-3URL [本文引用: 1]
DOI:10.1023/A:1006244819642URL [本文引用: 2]

Forest soil respiration is the sum of heterotrophic (microbes, soil fauna) and autotrophic (root) respiration. The contribution of each group needs to be understood to evaluate implications of environmental change on soil carbon cycling and sequestration. Three primary methods have been used to distinguish hetero- versus autotrophic soil respiration including: integration of components contributing to in situ forest soil CO2 efflux (i.e., litter, roots, soil), comparison of soils with and without root exclusion, and application of stable or radioactive isotope methods. Each approach has advantages and disadvantages, but isotope based methods provide quantitative answers with the least amount of disturbance to the soil and roots. Published data from all methods indicate that root/rhizosphere respiration can account for as little as 10 percent to greater than 90 percent of total in situ soil respiration depending on vegetation type and season of the year. Studies which have integrated percent root contribution to total soil respiration throughout an entire year or growing season show mean values of 45.8 and 60.4 percent for forest and nonforest vegetation, respectively. Such average annual values must be extrapolated with caution, however, because the root contribution to total soil respiration is commonly higher during the growing season and lower during the dormant periods of the year.
DOI:10.1111/j.1365-2486.2007.01383.xURL [本文引用: 3]

Forests play a critical role in the global carbon cycle, being considered an important and continuing carbon sink. However, the response of carbon sequestration in forests to global climate change remains a major uncertainty, with a particularly poor understanding of the origins and environmental responses of soil CO 2 efflux. For example, despite their large biomass, the contribution of ectomycorrhizal (EM) fungi to forest soil CO 2 efflux and responses to changes in environmental drivers has, to date, not been quantified in the field. Their activity is often simplistically included in the 'autotrophic' root respiration term. We set up a multiplexed continuous soil respiration measurement system in a young Lodgepole pine forest, using a mycorrhizal mesh collar design, to monitor the three main soil CO 2 efflux components: root, extraradical mycorrhizal hyphal, and soil heterotrophic respiration. Mycorrhizal hyphal respiration increased during the first month after collar insertion and thereafter remained remarkably stable. During autumn the soil CO 2 flux components could be divided into 60% soil heterotrophic, 25% EM hyphal, and 15% root fluxes. Thus the extraradical EM mycelium can contribute substantially more to soil CO 2 flux than do roots. While EM hyphal respiration responded strongly to reductions in soil moisture and appeared to be highly dependent on assimilate supply, it did not responded directly to changes in soil temperature. It was mainly the soil heterotrophic flux component that caused the commonly observed exponential relationship with temperature. Our results strongly suggest that accurate modelling of soil respiration, particularly in forest ecosystems, needs to explicitly consider the mycorrhizal mycelium and its dynamic response to specific environmental factors. Moreover, we propose that in forest ecosystems the mycorrhizal CO 2 flux component represents an overflow 'CO 2 tap' through which surplus plant carbon may be returned directly to the atmosphere, thus limiting expected carbon sequestration from trees under elevated CO 2 .
DOI:10.1111/j.1365-2389.2012.01433.xURL [本文引用: 2]

We deployed an automated multiplexed soil-respiration (SR) system to monitor partitioned soil CO2 component fluxes (from roots, mycorrhizal hyphae and heterotrophs) in a UK grassland using a combination of shallow surface (total SR flux), deep (excluding roots and mycorrhizal fungi) and 20-碌m pore mesh window soil collars (excluding roots only). Soil CO2 efflux was monitored during a 3-month period during summer. Repeated cutting of mycorrhizal connections in some of the mycorrhizal treatments enabled assessment of subsequent recovery of mycorrhizal fluxes and a comparison with deep collar fluxes. After soil collar insertion, fluxes in the deep collars were significantly reduced, by approximately 40%. Whereas fluxes in the uncut, mycorrhizal collar treatments remained close to those from the surface collar, cut mycorrhizal treatments showed an immediate reduction after cutting to values close to those from the deep collar with a subsequent recovery of around 4 weeks. Overall, the autotrophic root and mycorrhizal flux was relatively stable throughout. Whereas root fluxes contributed about 10 30% of the total flux during the initial larger flux period, this declined and there was an increased mycorrhizal contribution during the latter part of the measurement period. Moreover, SR flux components differed in their response to key climatic factors, with root fluxes responding equally to temperature and light. Importantly, whereas the heterotrophic flux component responded strongly to temperature and soil moisture, the mycorrhizal component responded much less to those factors, but more to light. We also investigated treatment impacts over time on soil biochemical variables such as microbial biomass C, extractable C, microbial quotient and metabolic quotient, and bacterial community structure, and discussed these in relation to measured SR fluxes and the partitioning technique.
DOI:10.1111/j.1469-8137.2006.01730.xURLPMID:16771991 [本文引用: 1]

090004 Although arbuscular mycorrhizal (AM) fungi are a major pathway in the global carbon cycle, their basic biology and, in particular, their respiratory response to temperature remain obscure. 090004 A pulse label of the stable isotope 13 C was applied to Plantago lanceolata , either uninoculated or inoculated with the AM fungus Glomus mosseae . The extra-radical mycelium (ERM) of the fungus was allowed to grow into a separate hyphal compartment excluding roots. We determined the carbon costs of the ERM and tested for a direct temperature effect on its respiration by measuring total carbon and the 13 C: 12 C ratio of respired CO 2 . With a second pulse we tested for acclimation of ERM respiration after 2 wk of soil warming. 090004 Root colonization remained unchanged between the two pulses but warming the hyphal compartment increased ERM length. 0207 13 C signals peaked within the first 10 h and were higher in mycorrhizal treatments. The concentration of CO 2 in the gas samples fluctuated diurnally and was highest in the mycorrhizal treatments but was unaffected by temperature. Heating increased ERM respiration only after the first pulse and reduced specific ERM respiration rates after the second pulse; however, both pulses strongly depended on radiation flux. 090004 The results indicate a fast ERM acclimation to temperature, and that light is the key factor controlling carbon allocation to the fungus.
DOI:10.1038/35081058URLPMID:11459055 [本文引用: 1]

The respiratory activities of plant roots, of their mycorrhizal fungi and of the free-living microbial heterotrophs (decomposers) in soils are significant components of the global carbon balance, but their relative contributions remain uncertain(1,2). To separate mycorrhizal root respiration from heterotrophic respiration in a boreal pine forest, we conducted a large-scale tree-girdling experiment, comprising 9 plots each containing about 120 trees. Tree-girdling involves stripping the stem bark to the depth of the current xylem at breast height terminating the supply of current photosynthates to roots and their mycorrhizal fungi without physically disturbing the delicate root-microbe-soil system. Here we report that girdling reduced soil respiration within 1-2 months by about 54% relative to respiration on ungirdled control plots, and that decreases of up to 37% were detected within 5 days. These values clearly show that the flux of current assimilates to roots is a key driver of soil respiration; they are conservative estimates of root respiration, however, because girdling increased the use of starch reserves in the roots. Our results indicate that models of soil respiration should incorporate measures of photosynthesis and of seasonal patterns of photosynthate allocation to roots. [References: 18]
[本文引用: 1]
DOI:10.1111/j.1469-8137.1990.tb00924.xURL [本文引用: 1]

Cucumber ( Cucumis sativus L.) plants grown in PVC tubes with a partially sterilized soil-sand mixture were inoculated with the vesicular-arbuscular mycorrhizal fungus Glomus fasciculatum (Thaxter) Gerdemann & Trappe emend. Walker & Koske or left uninoculated. The soil column of each PVC tube was divided into a root and a hyphal compartment by a mesh bag (60 0204m), which retained the roots but allowed external hyphae to pass. Inoculated plants rapidly became infected and an extensive mycelium developed. Three weeks after seedling emergence plants were labelled with 14 CO 2 for 16 h. The distribution of 14 C within the plants and the 14 C flow into external hyphae and soil were measured during an 80 h chase period. Below-ground respiration in mycorrhizal plants accounted for 27% of the photo assimilated 14 C. Organic 14 C in the soil represented 309051 % of the fixed 14 C, and 26 % of this was located in external hyphae. Based on conservative assumptions concerning dry weight of internal mycorrhizal infection and growth yield of the fungus, it was estimated that mycorrhizal events consumed 20 % of photoassimilated 14 C. The specific incorporation of C by the external mycelium in the hyphal compartment was 41 0204g C mg 0908081 dry wt. d 0908081 . The importance of external VA mycorrhizal hyphae for the distribution of plant-derived C in the soil volume and as a substrate source for the soil biota is discussed.
DOI:10.1046/j.0028-646X.2001.00273.xURL [本文引用: 1]

61 A novel in-growth core system, enabling functional studies of natural communities of arbuscular mycorrhizal (AM) mycelia in soil is described and tested. 61 The cores have windows covered with nylon mesh of 35 μm pore size that prevent in-growth of roots but permit penetration of AM hyphae. They were inserted into grassland turf and contained either sterilized sand and a 'bait' seedling of Trifolium repens or nonsterile natural soil without bait plants. The impacts of hyphal severance, achieved by periodic rotation of some of the cores, upon AM colonization of bait plants (experiment 1) and transfer of33P from soil to plants outside the cores (experiment 2) were examined. 61 Severance of AM hyphae reduced both AM colonization of bait plants and their shoot P concentrations. The shoot33P concentrations of plants with mycelial access to33PO4-labelled cores were 10-fold greater than those which had no mycelial access. 61 It is concluded that this novel approach enables the functioning of mycorrhizal mycelial networks to be evaluated under conditions closely simulating those occurring in nature.
DOI:10.1007/s13199-013-0265-8URL [本文引用: 1]

No Abstract available for this article.
DOI:10.1371/journal.pone.0091998URLPMID:3956881 [本文引用: 1]

Small-scale heterogeneity of abiotic and biotic factors is expected to play a crucial role in species coexistence. It is known that plants are able to concentrate their root biomass into areas with high nutrient content and also acquire nutrients via symbiotic microorganisms such as arbuscular mycorrhizal (AM) fungi. At the same time, little is known about the small-scale distribution of soil nutrients, microbes and plant biomass occurring in the same area. We examined small-scale temporal and spatial variation as well as covariation of soil nutrients, microbial biomass (using soil fatty acid biomarker content) and above- and belowground biomass of herbaceous plants in a natural herb-rich boreonemoral spruce forest. The abundance of AM fungi and bacteria decreased during the plant growing season while soil nutrient content rather increased. The abundance of all microbes studied also varied in space and was affected by soil nutrient content. In particular, the abundance of AM fungi was negatively related to soil phosphorus and positively influenced by soil nitrogen content. Neither shoot nor root biomass of herbaceous plants showed any significant relationship with variation in soil nutrient content or the abundance of soil microbes. Our study suggests that plants can compensate for low soil phosphorus concentration via interactions with soil microbes, most probably due to a more efficient symbiosis with AM fungi. This compensation results in relatively constant plant biomass despite variation in soil phosphorous content and in the abundance of AM fungi. Hence, it is crucial to consider both soil nutrient content and the abundance of soil microbes when exploring the mechanisms driving vegetation patterns.
DOI:10.1002/jpln.200421703URL [本文引用: 1]

Partitioning the root-derived CO2 efflux from soil (frequently termed rhizosphere respiration) into actual root respiration (RR, respiration by autotrophs) and rhizomicrobial respiration (RMR, respiration by heterotrophs) is crucial in determining the carbon (C) and energy balance of plants and soils. It is also essential in quantifying C sources for rhizosphere microorganisms and in estimation of the C contributing to turnover of soil organic matter (SOM), as well as in linking net ecosystem production (NEP) and net ecosystem exchange (NEE). Artificial-environment studies such as hydroponics or sterile soils yield unrealistic C-partitioning values and are unsuitable for predicting C flows under natural conditions. To date, several methods have been suggested to separate RR and RMR in nonsterile soils: 1) component integration, 2) substrate-induced respiration, 3) respiration by excised roots, 4) comparison of root-derived 14CO2 with rhizomicrobial 14CO2 after continuous labeling, 5) isotope dilution, 6) model-rhizodeposition technique, 7) modeling of 14CO2 efflux dynamics, 8) exudate elution, and 9) 13C of CO2 and microbial biomass. This review describes the basic principles and assumptions of these methods and compares the results obtained in the original papers and in studies designed to compare the methods. The component-integration method leads to strong disturbance and non-proportional increase of CO2 efflux from different sources. Four of the methods (5 to 8) are based on the pulse labeling of shoots in a 14CO2 atmosphere and subsequent monitoring of 14CO2 efflux from the soil. The model-rhizodeposition technique and exudate-elution procedure strongly overestimate RR and underestimate RMR. Despite alternative assumptions, isotope dilution and modeling of 14CO2-efflux dynamics yield similar results. In crops and grasses (wheat, ryegrass, barley, buckwheat, maize, meadow fescue, prairie grasses), RR amounts on average to 4800±5% and RMR to 5200±5% of root-derived CO2. The method based on the 13C isotopic signature of CO2 and microbial biomass is the most promising approach, especially when the plants are continuously labeled in 13CO2 or 14CO2 atmosphere. The difference methods, i.e. , trenching, tree girdling, root-exclusion techniques, etc., are not suitable for separating the respiration by autotrophic and heterotrophic organisms because the difference methods neglect the importance of microbial respiration of rhizodeposits. <P> Translated Wurzelatmung und rhizomikrobielle Atmung: 0108bersicht 0104ber verschiedene Ans01¤tze zur Absch01¤tzung der Atmung autotropher und heterotropher Organismen im Boden Trennung des wurzelb0104rtigen CO2-Effluxes aus dem Boden (oft als Rhizosph01¤renatmung bezeichnet) in die eigentliche Wurzelatmung (= Atmung der Autotrophen) und die rhizomikrobielle Atmung (= ein Teil der Atmung der Heterotrophen) ist entscheidend f0104r die Bestimmung des Kohlenstoff (C)- und Energiehaushaltes von Pflanzen und B0109den. Sie ist unentbehrlich f0104r die Quantifizierung der C-Quellen f0104r Rhizosph01¤ren-Mikroorganismen und die organische Bodensubstanz, aber auch zur Kopplung der Netto-0102kosystemproduktion (NEP) mit dem Netto-0102kosystemaustausch (NEE). K0104nstliche Wachstumsmedien f0104r Pflanzen (Hydrokultur oder Bodensterilisation) lieferten f0104r die C-Partitionierung unrealistische Ergebnisse und sind f0104r die Vorhersage der C-Fl0104sse unter nat0104rlichen Bedingungen kaum anwendbar. Bis heute sind folgende Methoden zur Trennung der Wurzelatmung und der rhizomikrobiellen Atmung in nicht sterilen B0109den vorgeschlagen worden: 1) Komponenten-Integration, 2) substratinduzierte Atmung, 3) Messung der Atmung von abgeschnittenen Wurzeln, 4) Vergleich des 14CO2-Effluxes aus dem wurzelb0104rtigen CO2 mit dem aus der rhizomikrobiellen Atmung, 5) Isotopen-Verd0104nnung, 6) Anwendung von k0104nstlichen Rhizodepositen, 7) Modellierung der Dynamik des 14CO2-Effluxes, 8) Auswaschung der Exsudate und 9) 13C in CO2 und mikrobieller Biomasse. Dieser 0108bersichtsartikel beschreibt die Grundlagen und Annahmen dieser Methoden und vergleicht die Ergebnisse aus den Originalpublikationen mit Studien, die auf einen Vergleich der Methoden ausgerichtet waren. Die Methode der Komponenten-Integration f0104hrt zu einer starken St0109rung und zu einem nicht proportionalen Anstieg des CO2-Effluxes aus unterschiedlichen Quellen. Vier Methoden (5-8) basieren auf einer Pulsmarkierung von Pflanzen in einer 14CO2-Atmosph01¤re und anschlie0101endem Monitoring des CO2-Effluxes aus dem Boden. Die Methoden der k0104nstlichen Rhizodeposite und der Auswaschung von Exsudaten 0104bersch01¤tzen die Wurzelatmung stark und untersch01¤tzen die rhizomikrobielle Atmung. Trotz gegens01¤tzlicher Annahmen zeigen die Isotopen-Verd0104nnung und die Modellierung der Dynamik des 14CO2-Effluxes 01¤hnliche Ergebnisse. In den untersuchten Pflanzen (Weizen, Lolium perenne , Gerste, Buchweizen, Pr?riegr?ser) betrug die Wurzelatmung 4800±5 % und die rhizomikrobielle Atmung ca. 5200±5 % des wurzelb0104rtigen CO2-Effluxes. Die Methode, die auf der 13C-Isotopensignatur des CO2 und der mikrobiellen Biomasse basiert, scheint viel versprechend zu sein, insbesondere wenn Pflanzen kontinuierlich in der 13CO2- oder 14CO2-Atmosph01¤re markiert werden. Differenzmethoden wie Trenching, Baumg0104rteln (tree girdling) und Wurzelausschlusstechnik (root exclusion) sind f0104r eine Trennung der Atmung von autotrophen und heterotrophen Organismen nicht anwendbar, weil sie die Wichtigkeit der mikrobiellen Veratmung der Rhizodeposite vernachl01¤ssigen.
DOI:10.1016/j.soilbio.2008.05.021URL [本文引用: 1]

Land-use change can have significant impacts on soil conditions and microbial communities are likely to respond to these changes. However, such responses are poorly characterized as few studies have examined how specific changes in edaphic characteristics do, or do not, influence the composition of soil bacterial and fungal communities across land-use types. Soil samples were collected from four replicated ( = 3) land-use types (hardwood and pine forests, cultivated and livestock pasture lands) in the southeastern US to assess the effects of land-use change on microbial community structure and distribution. We used quantitative PCR to estimate bacterial ungal ratios and clone libraries targeting small-subunit rRNA genes to independently characterize the bacterial and fungal communities. Although some soil properties (soil texture and nutrient status) did significantly differ across land-use types, other edaphic factors (e.g., pH) did not vary consistently with land-use. Bacterial ungal ratios were not significantly different across the land-uses and distinct land-use types did not necessarily harbor distinct soil fungal or bacterial communities. Rather, the composition of bacterial and fungal communities was most strongly correlated with specific soil properties. Soil pH was the best predictor of bacterial community composition across this landscape while fungal community composition was most closely associated with changes in soil nutrient status. Together these results suggest that specific changes in edaphic properties, not necessarily land-use type itself, may best predict shifts in microbial community composition across a given landscape. In addition, our results demonstrate the utility of using sequence-based approaches to concurrently analyze bacterial and fungal communities as such analyses provide detailed phylogenetic information on individual communities and permit the robust assessment of the biogeographical patterns exhibited by soil microbial communities.
DOI:10.1139/b04-060URL [本文引用: 1]

Extraradical mycelia of mycorrhizal are normally the hidden half of the , but they are powerful underground influences upon biogeochemical cycling, the composition of plant communities, and agroecosystem functioning. Mycorrhizal mycelial networks are the most dynamic and functionally diverse components of the , and recent estimates suggest they are empowered by receiving as much as 10% or more of the net photosynthate of their . They often constitute 20% 30% of total soil microbial biomass yet are undetected by standard measures of biomass used by soil scientists and agromomists. Mycorrhizal mycelia provide extensive pathways for and nutrient fluxes through soil, often exceeding tens of metres per gram of soil. We consider the amounts of photosynthate power allocated to these mycelial networks and how this is used in fungal respiration, biomass, and and in influencing soil, plant, and ecosystem processes. The costs and functional benefits to linking to these networks are fungal specific and, because of variations in physiology and specificity, are not shared equally; some even depend exclusively on these networks for . We briefly assess the potential contribution of extraradical mycorrhizal mycelium to sustainable agriculture and maintenance of biodiversity and highlight technologies that promise new vistas and improved fine-scale resolution of the dynamic spatial and temporal functioning of these networks in soil.Key words: arbuscular mycorrhiza, ectomycorrhiza, extraradical mycelium, hyphal networks.
DOI:10.1023/A:1026192607512URL [本文引用: 1]
URL [本文引用: 1]

Root respiration is an important component in carbon cycles of forest ecosystems.Quantifying root respiration is necessary for exactly calculating net primary production and carbon balance in forest ecosystems.It has been realized that tree root respiration is related,one way or another,to root system biological parameters.In this study root morphologic parameters and C,N content of four diameter classes(0~2 mm,2~5 mm,5~10 mm,10~20 mm)were analyzed for 16 years old trees of Cunninghamia lanceolata(Lamb.)Hook.by Win RHIZO TRON 2005a and Elementar Vario EL Ⅲ,and excised root respiration rates of different diameter classes were measured by Soil Respiration Measuring System(Li-8100.LI-COR,USA);and characters of root parameters and their relationship with respiration were analyzed;we also explored the most appropriate time period for measuring root respiration after excised based on fluctuation of the excised roots respiration.Results showed that the variation in the respective root parameters for different diameter classes was significant and,the root parameters were correlated with each other;the mean respiration rate(±SE)of roots for diameter of 2 mm(1.145±0.249 nmolCO2 gDM-1 s-1)was significantly larger than that of 2mm(0.35±0.040 nmolCO2 gDM-1 s-1);while C contents and C/N were significantly positive correlative with excised root respiration rate,the N content of fine root presented pronounced negative relationship and so did the parameter Specific Length of roots.We found that excised root respiration of 2 mm diameter should be immediately measured,while for roots of 2 mm the measuring time duration could be extended without significant effect on respiration rates results.
DOI:10.1016/j.funeco.2017.02.001URL [本文引用: 2]

Fungal respiration contributes substantially to ecosystem respiration, yet its field temperature response is poorly characterized. I hypothesized that at diurnal time scales, temperature-respiration relationships would be better described by unimodal than exponential models, and at longer time scales both Q10and mass-specific respiration at 1002°C (Rms10) would show signs of acclimation. I measured respiration on intact sporocarps over the course of several days, and modeled temperature-respiration relationships using exponential and unimodal Gaussian functions. Unimodal models provided a better fit than exponential models. Rms10and Q10also declined with increasing temperature, consistent with longer-term temperature acclimation. There was some evidence of diurnal hysteresis. When exponential models were appropriate,Q10values averaged 653.5, and Rms10averaged 0.0202μmol CO2g611sec611. The observed high mass-specific respiration rates, peaked temperature responses, decline in Rms10and Q10with increasing temperature, and hysteresis could contribute to observed non-exponential and hysteretic patterns in soil and ecosystem respiration.
[本文引用: 1]
[本文引用: 1]
DOI:10.3724/SP.J.1005.2008.01083URL [本文引用: 1]

利用实际观测的113个地面数据,估算了内蒙古温带草地地上、地下生物量的大小,揭示了其空间分布和地下生物量的垂直分布规律,并探讨了不同环境因素对地上、地下生物量的调控作用.主要结果如下:(1)3种草地类型(荒漠草原、典型草原和草甸草原)的生物量存在显著差异;其地上生物量分别为56.6,133.4和196.7g/m2,地下生物量分别为301.0,688.9和1385.2g/m2;(2)地上生物量和地下生物量均呈现自西南向东北增加的空间分布特征;3种草地具有相似的地下生物量垂直分布特征;总体上,温带草地表层(0~10cm)地下生物量约占总地下生物量的一半;(3)降水是导致内蒙古温带草地生物量空间变异的主要因子.地下生物量的垂直分布与降水关系密切,而受土壤质地和草地类型的影响较弱.
DOI:10.3724/SP.J.1005.2008.01083URL [本文引用: 1]

利用实际观测的113个地面数据,估算了内蒙古温带草地地上、地下生物量的大小,揭示了其空间分布和地下生物量的垂直分布规律,并探讨了不同环境因素对地上、地下生物量的调控作用.主要结果如下:(1)3种草地类型(荒漠草原、典型草原和草甸草原)的生物量存在显著差异;其地上生物量分别为56.6,133.4和196.7g/m2,地下生物量分别为301.0,688.9和1385.2g/m2;(2)地上生物量和地下生物量均呈现自西南向东北增加的空间分布特征;3种草地具有相似的地下生物量垂直分布特征;总体上,温带草地表层(0~10cm)地下生物量约占总地下生物量的一半;(3)降水是导致内蒙古温带草地生物量空间变异的主要因子.地下生物量的垂直分布与降水关系密切,而受土壤质地和草地类型的影响较弱.
DOI:10.1007/BF00328420URLPMID:28306940 [本文引用: 1]

External hyphae of vesicular-arbuscular mycorrhizal (VAM) fungi were quantified over a growing season in a reconstructed tallgrass prairie and an ungrazed cool-season pasture. In both sites, hyphal lengths increased throughout the growing season. Peak external hyphal lengths were 111 m cm-3of soil in the prairie and 81 m cm-3of soil in the pasture. These hyphal lengths calculate to external hyphal dry weights of 457 g cm-3and 339 g cm-3of soil for prairie and pasture communities, respectively. The relationships among external hyphal length, root characteristics, soil P and soil moisture were also determined. Measures of gross root morphology [e.g., specific root length (SRL) and root mass] have a strong association with external hyphal length. Over the course of the study, both grassland communities experienced a major drought event in late spring. During this period a reduction in SRL occurred in both the pasture and prairie without a measured reduction in external hyphal length. Recovery for both the pasture and prairie occurred not by increasing SRL, but rather by increasing external hyphal length. This study suggests that growth is coordinated between VAM hyphae and root morphology, which in turn, are constrained by plant community composition and soil nutrient and moisture conditions.
DOI:10.1016/j.agrformet.2007.09.006URL [本文引用: 1]

Soil respiration is a combination of CO 2 fluxes derived from a diversity of belowground sources, many depending directly on the input of carbon from living plants. Here we present data from two different forest ecosystems, a beech and a spruce forest, where a partitioning of soil respiration was carried out. We used soil cores inside micro-pore meshes together with periodic chamber-based measurements to estimate rhizosphere, mycorrhizal fungal and microbial heterotrophic respiration. Calculated mycorrhizal mycelium respiration was 8% at the spruce forest and 3% at the beech forest. Given the nature of the partitioning method these values represent minimum estimates. The ratio of root-derived carbon respiration to heterotrophic respiration was about 1:1 at both forest types. The relationship of each source with temperature and photosynthesis, measured as gross primary productivity derived from eddy covariance measurements, was subsequently explored. Both factors revealed effects specific to the respiration source and the forest type. A response to temperature was evident in all cases except for mycorrhizal mycelium respiration at the spruce forest ( R 2 = 0.06, p = 0.41). Significant correlations of photosynthesis with rhizosphere and mycorrhizal fungal respiration were found in all cases. Peaks in correlation values showed time lags between photosynthetic activity and a respiration response ranging from 1 day for the fungal component and 4 days for the rhizosphere component at the beech forest ( R 2 = 0.70, p < 0.01 and R 2 = 0.42, p < 0.05, respectively) to 5 days for both fluxes at the spruce forest ( R 2 = 0.44, p < 0.01 and R 2 = 0.72, p < 0.01, respectively). Results show that respiration of the mycorrhizal component cannot be predicted by common temperature driven models in some ecosystems. They also indicate a strong influence of forest canopy processes on the activity of roots and associated organisms. The specific response in each vegetation type should be ideally explained by physiological mechanisms inherent to different species as a next step towards understanding belowground carbon dynamics.
DOI:10.1016/j.soilbio.2006.10.001URL [本文引用: 1]

The mycorrhizal, rhizosphere and basal components of soil respiration were partitioned in a barley field experiment with the main objective of determining the controlling effects of photosynthetic activity and temperature on soil respiration sources. Micro-pore meshes were used to create both root and mycorrhiza-free soil cores over which collars for soil respiration measurements were inserted. Differences between mesh treatments were used to determine the contribution of each component. With a focus on the growing season, we analyzed the response of respiration sources to photosynthesis, temperature and moisture, as well as changes in microbial biomass, mineral nitrogen and carbon–nitrogen ratios responding to treatment and time of year. Results gave clear differences between sources in their response to both temperature and photosynthetic activity and showed that several processes are involved in determining respiration rates as well as apparent temperature relations. In particular, the respiration of arbuscular mycorrhizal hyphae was seen to be a significant amount of root derived carbon respiration (25.3%) and consequently of total assimilated carbon (4.8%). This source showed a stronger response to photosynthetic activity than the rhizosphere component ( r 2=0.79, p<0.001 and r 2=0.324, p=0.53, respectively). Q 10 values—the increase in respiration rates with a 10 °C increase in temperature—changed seasonally and showed temperature relations being dependent on the presence of mycorrhizal and rhizosphere respiration sources, as well as on plant development. Respiration from mycorrhizal hyphae and the rhizosphere showed no response ( r 2=0, p<0.99) or low response ( r 2=0.14, p<0.01) to temperature, respectively. We conclude that the potential importance and controls of mycorrhizal fungi respiration in croplands are comparable to those observed in other ecosystems, and that temperature response curves should be carefully interpreted given that substrate availability and plant dynamics strongly regulate respiration rates in ecosystems.
DOI:10.1007/s11104-013-2018-0URL [本文引用: 3]

Background and aims Partitioning of soil respiration is a challenging task when resolving the C cycling in forest ecosystems. Our aim was to partition the respiration of newly grown extramatrical ectomycorrhizal mycelium (ECM) and fine roots (and their associated microorganisms) in a young Norway spruce forest. Methods Ingrowth mesh bags of 1602cm diameter and 1202cm height were placed in the upper soil and left for 12–1602months in 2010 and 2011. The 202mm mesh size allowed the ingrowth of ECM and fine roots whereas a 4502μm mesh size allowed only the ingrowth of ECM. The mesh bags were filled with either homogenized EA horizon soil, pure quartz sand (QS) or crushed granite (CG, only 2011), each with five replicates. Controls without any ingrowth were established for each substrate by solid plastic tubes (2010) and by 102μm mesh bags (2011). Fluxes of CO 2 from the mesh bags and controls were measured biweekly during the growing season by the closed chamber method. Results The contribution of ECM to soil respiration was largest in the QS treatments, reaching cumulatively 1.2 and 2.202Mg C ha 611 6 months 611 in 2010 and 2011, respectively. For EA and CG treatments, the cumulative respiration from ECM was larger than from controls, however the differences being not statistically significant. The respiration of newly grown fine roots in QS amounted to 1.002Mg C ha 611 in 2010, but could not be identified in 2011 since fluxes from 202mm and 4502μm mesh bags were similar. The correlation of total root length in single QS mesh bags to CO 2 fluxes was poor. The contribution of fine root respiration was also not detectable in the EA and CG treatment. No correlation was found between the autumnal biomass of newly grown ECM and its cumulative respiration. Conclusion Our results suggest a substantial contribution of newly grown ECM to soil respiration. Respiration of ECM might be larger than respiration of fine roots.
DOI:10.1111/j.1469-8137.2010.03226.xURLPMID:20345636 [本文引用: 2]

090004Arbuscular mycorrhizal fungi (AMF) are widespread in tropical forests and represent a major sink of photosynthate, yet their contribution to soil respiration in such ecosystems remains unknown.090004Using in-growth mesocosms we measured AMF mycelial respiration in two separate experiments: (1) an experiment in a semi-evergreen moist tropical forest, and (2) an experiment with 6-m-tall Pseudobombax septenatum in 4.5-m3 containers, for which we also determined the dependence of AMF mycelial respiration on the supply of carbon from the plant using girdling and root-cutting treatments.090004In the forest, AMF mycelia respired carbon at a rate of 1.4 t ha0908081 yr0908081, which accounted for 14 00± 6% of total soil respiration and 26 00± 12% of root-derived respiration. For P. septenatum, 40 00± 6% of root-derived respiration originated from AMF mycelia and carbon was respired < 4 h after its supply from roots.090004We conclude that arbuscular mycorrhizal mycelial respiration can be substantial in lowland tropical forests. As it is highly dependent on the recent supply of carbon from roots, a function of aboveground fixation, AMF mycelial respiration is therefore an important pathway of carbon flux from tropical forest trees to the atmosphere.
DOI:10.1016/S0098-8472(97)00014-2URL [本文引用: 1]

Fine roots (diameter =< 2 mm) of Acer rubrum, Quercus rubra and Pinus strobus were used to determine whether respiration rates (measured as COefflux rates) remain sufficiently stable for the 1 min to 10 h interval after excision to allow reliable measurements to be taken. Roots of five individuals of each species were excavated from the upper 10 cm of the soil. From each tree, two samples of roots (dry weights between 0.21 and 1.18 g) were excised and immediately subjected to one of two treatments. The attached soil was removed mechanically with (wet treatment) or without (dry treatment) the further removal of soil by submersion in and rinsing with water. Within 1 min of excision, roots were placed in a gas exchange system and their respiration was measured. The measurements were repeated 5, 15, 30 min and 1, 2 (Acer and Pinus), 3 (Quercus), 6 and 10 h after excision. For all species, carbon efflux rates showed a marked decline over time, dropping significantly (p =< 0.05) over 5 min for Acer and Pinus and over 15 min for Quercus (by 37, 52 and 33%, respectively). The initial rates (12.9, 9.2 and 8.7 nmol COgsat 25 C for Acer, Quercus and Pinus, respectively) declined to a minimum (4.2, 2.9 and 4.4 nmol COgs) in 30 (Pinus) or 60 (Acer and Quercus) min then levelled off (Acer and Quercus) or started to increase (Pinus). Possible mechanisms causing the decline are discussed.
DOI:10.1007/s004420050821URLPMID:28307716 [本文引用: 1]

Root, arbuscular-mycorrhizal (AM), soil faunal (protozoa and microarthropods), and microbial responses to field exposure to CO60 for six growing seasons were measured in spring 1997 in two adjacent grassland communities. The grasslands showed contrasting root responses to CO60 enrichment: whereas root length was not affected in the sandstone grassland, it was greater in the serpentine grassland, as was specific root length. AM fungal hyphal lengths were greater in the sandstone, but were unaffected in the serpentine community. This lent support to the hypothesis that there may be a tradeoff in resource allocation to more fine roots or greater mycorrhizal extraradical hyphal length. AM root infection was greater in both communities at elevated CO60, as was the proportion of roots containing arbuscules. Our data on total hyphal lengths, culturable and active fungi, bacteria, and protozoa supported the hypothesis that the fungal food chain was more strongly stimulated than the bacterial chain. This study is one of the first to test these hypotheses in natural multi-species communities in the field.
DOI:10.1007/s11104-005-5109-8URL [本文引用: 1]

A simple estimation of heterotrophic respiration can be obtained analytically as the y-intercept of the linear regression between soil-surface CO2 efflux and root biomass. In the present study, a development of this indirect methodology is presented by taking into consideration both the temporal variation and the spatial heterogeneity of heterotrophic respiration. For this purpose, soil CO2 efflux, soil carbon content and main stand characteristics were estimated in seven evergreen forest ecosystems along an elevation gradient ranging from 250 to 1740 m. For each site and for each sampling date the measured soil CO2 efflux (Rs) was predicted with the model RS = a × SC + b × RD ± ε, where SC is soil carbon content per unit area to a depth of 30 cm and RD is the root density of the 2–5 mm root class. Regressions with statistically significant a and b coefficients allowed the indirect separation of the two components of soil CO2 efflux. Considering that the different sampling dates were characterized by different soil temperature, it was possible to investigate the temporal and thermal dependency of autotrophic and heterotrophic respiration. It was estimated that annual autotrophic respiration accounts for 16-58% of total soil C02 efflux in the seven different evergreen ecosystems. In addition, our observations show a decrease of annual autotrophic respiration at increasing availability of soil nitrogen.
DOI:10.1038/369058a0URL [本文引用: 2]

PLANTS and soils are a critically important element in the global carbon-energy equation. It is estimated that in forest ecosystems over two-thirds of the carbon is contained in soils and peat deposits. Despite the importance of forest soils in the global car-bon cycle, fluxes of carbon associated with fundamental processes and soil functional groups are inadequately quantified, limiting our understanding of carbon movement and sequestration in soils. We report here the direct measurement of carbon in and through all major pools of a mycorrhizal (fungus-root) coniferous seedling (a complete carbon budget). The mycorrhizal symbiont reduces over-all retention of carbon in the plant-fungus symbiosis by increasing carbon in roots and below-ground respiration and reducing its retention and release above ground. Below ground, mycorrhizal plants shifted allocation of carbon to pools that are rapidly turned over, primarily to fine roots and fungal hyphae, and host root and fungal respiration. Mycorrhizae alter the size of below-ground carbon pools, the quality and, therefore, the retention time of carbon below ground. Our data indicate that if elevated atmos-pheric COand altered climate stressors alter mycorrhizal colonization in forests, the role of forests in sequestering carbon could be altered.
DOI:10.1016/j.geoderma.2006.12.005URL [本文引用: 1]

It is crucial to advance the understanding of soil CO 2 efflux and its components for a better comprehension of carbon dynamics in terrestrial ecosystems. The process-based PATCIS model was applied to a first rotation young Sitka spruce stand in order to simulate the seasonal contribution of soil respiration components to the overall soil CO 2 efflux. We evaluated the performance of the model with observed measurements and compared it with empirically derived regressions. Once the model was parameterised, it explained 75% of the seasonal variation in total soil CO 2 efflux. Similar seasonal trends and annual estimates of soil CO 2 efflux were obtained with either empirical or the process-based PATCIS models. Heterotrophic and autotrophic respiration contributed almost equally to total CO 2 efflux during the early and late part of the year, while a larger contribution of autotrophic respiration to total CO 2 efflux occurred during the growing season. The overall annual contribution of autotrophic respiration to total soil CO 2 efflux was 54.7%. Most of root respiration took place in both the litter–humus layer and the A 1 horizon as a result of their large concentrations of fine roots. We observed an accumulation of organic matter in the litter–humus layer, and a net loss from the mineral soil, which had much larger organic matter content compared to the litter–humus layer. The organic matter turnover rate calculated for the mineral soil was 4502years (mean residence time). The sensitivity analysis showed soil temperature as the most important factor controlling soil respiration. The influence of soil moisture was more variable and had an overall negative effect on soil respiratory rates, except for periods of low soil water content, such as summer drought. The episodic occurrence of very wet conditions at the deeper soil layers was responsible for their low contribution to total soil respiration. In general, gas transport within the soil was not an important constraint for soil CO 2 efflux since most of soil respiration was produced in the highly porous litter–humus and top mineral layers. The autotrophic component was more affected than heterotrophic respiration by changes in soil water content. Other factors such as changes in litterfall inputs were shown to have a more limited impact on soil CO 2 efflux. This work shows that the use of a process-based model to simulate soil CO 2 efflux may be a useful tool to separate soil respiration components.
DOI:10.1158/1055-9965.EPI-04-0846URL [本文引用: 1]

Root biomass exponential regression method was used to distinguish root respiration from soil microbial respiration in a Leymus Chinensis steppe in the Xilin River Basin of Inner Mongolia,China. The contribution of root respiration to soil respiration averaged( 24 3) %,ranging from 13%-52%; while the contribution of microbial respiration to soil respiration averaged( 76 3) %, ranging from 48%-87%. Correlation between soil respiration and root biomass was relatively unstable. Root respiration vitality decreased exponentially with the ratio of root to shoot biomass( R2= 0. 661,P = 0. 20),while it increased exponentially with soil water content at 0-10 cm,10-20 cm,20-30 cm and 30-40 cm( P 0. 000 1). CO 2 released by root respiration and the contribution of root respiration to soil respiration increased exponentially with root respiration vitality( R2= 0. 848,P = 0. 01; R2= 0. 818,P = 0. 01, respectively).
DOI:10.1007/s00572-016-0731-2URLPMID:27549438 [本文引用: 1]

Abstract Quantification of carbon (C) fluxes in mycorrhizal plants is one of the important yet little explored tasks of mycorrhizal physiology and ecology. 13 CO 2 pulse-chase labelling experiments are increasingly being used to track the fate of C in these plant-microbial symbioses. Nevertheless, continuous monitoring of both the below- and aboveground CO 2 emissions remains a challenge, although it is necessary to establish the full C budget of mycorrhizal plants. Here, a novel CO 2 collection system is presented which allows assessment of gaseous CO 2 emissions (including isotopic composition of their C) from both belowground and shoot compartments. This system then is used to quantify the allocation of recently fixed C in mycorrhizal versus nonmycorrhizal Medicago truncatula plants with comparable biomass and mineral nutrition. Using this system, we confirmed substantially greater belowground C drain in mycorrhizal versus nonmycorrhizal plants, with the belowground CO 2 emissions showing large variation because of fluctuating environmental conditions in the glasshouse. Based on the assembled 13 C budget, the C allocation to the mycorrhizal fungus was between 2.3% (increased 13 C allocation to mycorrhizal substrate) and 2.9% (reduction of 13 C allocation to mycorrhizal shoots) of the plant gross photosynthetic production. Although the C allocation to shoot respiration (measured during one night only) did not differ between the mycorrhizal and nonmycorrhizal plants under our experimental conditions, it presented a substantial part ( 10%) of the plant C budget, comparable to the amount of CO 2 released belowground. These results advocate quantification of both above- and belowground CO 2 emissions in future studies.
[本文引用: 1]
DOI:10.1111/j.1365-2435.2008.01402.xURL [本文引用: 1]

1. In the conventional view of soil carbon (C) cycling, mycorrhizal fungi are primarily considered vectors for plant C input to soils. However, there is accumulating evidence that mycorrhizal fungi may also contribute to the direct loss of soil C by acting as decomposers, that is by producing extracellular lytic enzymes and metabolizing soil C. 2. Most of the evidence that mycorrhizal fungi can act as decomposers comes from studies of ericoid and ectomycorrhizal fungi, although there is increasing experimental evidence for a role of arbuscular mycorrhizal fungi in soil C decomposition. Decomposition by mycorrhizal fungi implies that soil C balance is subjected to the ecological factors that affect both plant and fungal symbionts; this interaction has important consequences for how soil C stocks respond to global change. 3. In this synthesis, we propose a new model of soil C cycling, including decomposition of soil C by mycorrhizal fungi, and we evaluate how this new integrative model alters our predictions of soil C feedbacks to global change. We present three hypothetical mechanisms by which mycorrhizal fungi may metabolize significant quantities of soil C. The first hypothesis ('Plan B' hypothesis) is that mycorrhizal fungi metabolize soil C as an alternate C source when supplies of photosynthate from the host plant are low. Our second hypothesis ('Coincidental Decomposer' hypothesis) is that mycorrhizal fungi decompose soil C as a consequence of mining soil for organic nutrients. The third hypothesis ('Priming Effects' hypothesis) is that mycorrhizal fungi decompose soil C when allocation of plant photosynthate to mycorrhizal roots is high, such that plant C 'primes' the growth and activity of mycorrhizal fungi. 4. Further empirical tests of these hypotheses will clarify the role of mycorrhizal fungi in soil C balance and improve our understanding of soil C responses to global change.
[本文引用: 1]
DOI:10.1016/j.apsoil.2016.01.016URL [本文引用: 3]

Soil respiration (Rsoil) partitioning into its components is pivotal to understand C cycling in ecosystems. Notwithstanding the importance of mycorrhizal fungi in the belowground C cycle, their respiration (Rmyc) is poorly studied in agricultural ecosystems. In this study, we applied a modified trenching method and the13C natural abundance technique to distinguishRmycfrom root respiration (Rroot) and from the respiration due to soil organic matter (SOM) degradation (Rsom) in an apple orchard (Malus domesticaBorkh.) located in Northern Italy. Membranes with different mesh size were used to physically exclude roots or mycorrhizae from soil cores with a different 13C than the orchard average value. The CO2efflux from soil cores was determined with an infrared gas analyser, and isotopic measurements were performed on the soil-emitted CO2. The differentRsoilcomponents were determined both by difference and by the isotopic mass balance. Mycorrhizal contribution to soil respiration (11 6%) was of similar magnitude to that of roots (12 4%) whileRsomaccounted for 73 3% ofRsoil. In presence of apple roots, respiration of SOM and mycorrhizae (Rsom+myc) significantly increased in late summer and autumn, likely because of a priming effect of roots on SOM degradation or to a stimulation of mycorrhizal respiration. Our results suggest that respiration of mycorrhizal fungi can significantly contribute toRsoil, and need to be considered to correctly partition soil respiration. Furthermore, as roots increasedRsom+myc, the root exclusion method for soil respiration partitioning may overestimateRroot.
DOI:10.1111/j.1365-2486.2006.01291.xURL [本文引用: 1]

Partitioning soil respiration ( R S ) into heterotrophic ( R H ) and rhizospheric ( R R ) components is an important step for understanding and modeling carbon cycling in forest ecosystems, but few studies on R R and R H exist in Chinese temperate forests. In this study, we used a trenching plot approach to partition R S in six temperate forests in northeastern China. Our specific objectives were to (1) examine seasonal patterns of soil surface CO 2 fluxes from trenched ( R T ) and untrenched plots ( R UT ) of these forests; (2) quantify annual fluxes of R S components and their relative contributions in the forest ecosystems; and (3) examine effects of plot trenching on measurements of R S and related environmental factors. The R T maximized in early growing season, but the difference between R UT and R T peaked in later summer. The annual fluxes of R H and R R varied with forest types. The estimated values of R H for the Korean pine ( Pinus koraiensis Sieb. et Zucc.), Dahurian larch ( Larix gmelinii Rupr.), aspen-birch ( Populous davidiana Dode and Betula platyphylla Suk.), hardwood ( Fraxinus mandshurica Rupr., Juglans mandshurica Maxim. and Phellodendron amurense Rupr.), Mongolian oak ( Quercus mongolica Fisch.) and mixed deciduous (no dominant tree species) forests averaged 89, 196, 187, 245, 261 and 301 g C m 612 yr 611 , respectively; those of R R averaged 424, 209, 628, 538, 524 and 483 g C m 612 yr 611 , correspondingly; calculated contribution of R R to R S ( RC ) varied from 52% in the larch forest to 83% in the pine forest. The annual flux of R R was strongly correlated to biomass of roots <0.5 cm in diameter, while that of R H was weakly correlated to soil organic carbon concentration at A horizon. We concluded that vegetation type and associated carbon metabolisms of temperate forests should be considered in assessing and modeling R S components. The significant impacts of changed soil physical environments and substrate availability by plot trenching should be appropriately tackled in analyzing and interpreting measurements of R S components.
DOI:10.1111/j.1461-0248.2009.01303.xURLPMID:19320689 [本文引用: 1]

We examined the role of arbuscular mycorrhizal fungi (AMF) in ecosystems using soil aggregate stability and C and N storage as representative ecosystem processes. We utilized a wide gradient in AMF abundance, obtained through long-term (17 and 6 years) large-scale field manipulations. Burning and N-fertilization increased soil AMF hyphae, glomalin-related soil protein (GRSP) pools and water-stable macroaggregates while fungicide applications reduced AMF hyphae, GRSP and water-stable macroaggregates. We found that AMF abundance was a surprisingly dominant factor explaining the vast majority of variability in soil aggregation. This experimental field study, involving long-term diverse management practices of native multispecies prairie communities, invariably showed a close positive correlation between AMF hyphal abundance and soil aggregation, and C and N sequestration. This highly significant linear correlation suggests there are serious consequences to the loss of AMF from ecosystems.
DOI:10.1093/treephys/21.5.309URLPMID:11262922 [本文引用: 1]

We estimated total ecosystem respiration from a ponderosa pine (Pinus ponderosa Dougl. ex Laws.) plantation in the Sierra Nevada Mountains near Georgetown, California, from June to October, 1998. We apportioned ecosystem respiration among heterotrophic, root, stem and foliage based on relationships for each component that considered microclimate and vegetation characteristics. We measured each respiration component at selected sampling points, and scaled the measurements up to the ecosystem based on modeled relationships. Over the study period, total mean ecosystem respiration was 5.7 +/- 1.3 mumol m-2 s-1 (based on daily mean), comprising about 67% from soil-surface CO2 efflux, 10% from stem and branch respiration and 23% from foliage respiration. Shrub leaves contributed about 24% to total foliage respiration, and current-year needles (1998 age class) accounted for 40% of total tree needle respiration. Root respiration accounted for 47% of soil-surface CO2 efflux. We conclude that ecosystem respiration can be estimated based on daily mean air and soil temperatures through exponential relationships with r2 values of 0.85 and 0.87, respectively. When based on both air and soil temperatures, about 91% of the variation in total ecosystem respiration could be explained by a linear regression.
DOI:10.1111/j.1365-2486.2009.02091.xURL [本文引用: 2]

Evaluating how autotrophic (SRA), heterotrophic (SRH) and total soil respiration (SRTOT) respond differently to changes of environmental factors is critical to get an understanding of ecosystem carbon (C) cycling and its feedback processes to climate change. A field experiment was conducted to examine the responses of SRA and SRH to water and nitrogen (N) addition in a temperate steppe in northern China during two hydrologically contrasting growing seasons. Water addition stimulated SRA and SRH in both years, and their increases were significantly greater in a dry year (2007) than in a wet year (2006). N addition increased SRA in 2006 but not in 2007, while it decreased SRH in both years, leading to a positive response of SRTOT in 2006 but a negative one in 2007. The different responses of SRA and SRH indicate that it will be uncertain to predict soil C storage if SRTOT is used instead of SRH to estimate variations in soil C storage. Overall, N addition is likely to enhance soil C storage, while the impacts of water addition are determined by its relative effects on carbon input (plant growth) and SRH. Antecedent water conditions played an important role in controlling responses of SRA, SRH and the consequent SRTOT to water and N addition. Our findings highlight the predominance of hydrological conditions in regulating the responses of C cycling to global change in the semiarid temperate steppe of northern China.
DOI:10.1016/j.agrformet.2013.04.028URL [本文引用: 1]

Determining soil respiration from croplands is necessary for evaluating the global terrestrial carbon budget and how it is altered in future climates. This study explored seasonal characteristics and controlling factors of soil respiration in a typical cropland area in the North China Plain. Total soil respiration (RS) was partitioned into heterotrophic (RH) and autotrophic (RA) components using the root exclusion method. The experiments showed that the seasonal average RS values were 5.25μmolm612s611 for the wheat growing season and 6.00μmolm612s611 for the maize growing season. Seasonal average RH and RA values were 3.34μmolm612s611 and 1.91μmolm612s611, respectively, for wheat, and were 4.25μmolm612s611 and 1.75μmolm612s611, respectively, for maize. The seasonal average ratio of RA to RS (RA/RS) was 36% for wheat and 29% for maize. Over a whole year, RH was the dominant component of RS in both the wheat and maize growing seasons. RH increased exponentially with the average soil temperature collected in the upper 10cm (TS0–10), with a Q10 value of 1.65. Soil water content (θ) had no discernible influence on RH when θ was between wilting point (θwp) and field capacity (θfc). A value of θ larger than θfc suppressed RH, which can be characterized by a quadratic curve. RA increased exponentially with TS0–10 in both of the wheat and maize growing seasons, and the corresponding Q10 values were 2.69 and 2.85, respectively. However, the temperature dependence of RA in the two crop seasons cannot be explained by a single temperature response curve. Moreover, the RA values for the wheat and maize growing seasons were more sensitive to temperature changes than RH at the study site. Soil water content had no discernible influence on RA in the wheat growing season but suppressed RA when water logging occurred in the maize growing season. However, RA recovered afterwards even when the soil water content was high. Comparisons between wheat respiration values collected at different sites showed that the seasonal average RS, RH and RA all correlate positively with mean air temperature, indicating that air temperature remains a good indicator for variations in soil respiration in different climates.
[本文引用: 1]
[本文引用: 1]
Separation of root respiration from total soil respiration using carbon-13 labelling during free-air carbon dioxide enrichment (FACE)
1
1999
... 已经有一些研究在不同的生态系统采用不同的方法区分土壤呼吸组分以及定量每个组分分别对土壤总呼吸的贡献比例.一些方法是直接测定的, 比如离体根法: 直接测定所获得的活根的CO2释放量(
Autotrophic component of soil respiration is repressed by drought more than the heterotrophic one in dry grasslands
2
2016
... 菌根真菌作为生态系统重要的功能群之一, 在未受干扰的土壤中, 构成了植物根际区微生物的主体, 其生物量约占微生物总量的70% (
... 通过2014-2016三个生长季的连续测定, 表明内蒙古半干旱草原异养呼吸占土壤总呼吸的比例为45%-54%, 根呼吸占土壤总呼吸的20%-34%, 菌根呼吸占土壤总呼吸的21%-26%.与国内外研究(
Tree root and soil heterotrophic respiration as revealed by girdling of boreal Scots pine forest: Extending observations beyond the first year
1
2003
... 已经有一些研究在不同的生态系统采用不同的方法区分土壤呼吸组分以及定量每个组分分别对土壤总呼吸的贡献比例.一些方法是直接测定的, 比如离体根法: 直接测定所获得的活根的CO2释放量(
Functional diversity in arbuscular mycorrhizas: Exploitation of soil patches with different phosphate enrichment differs among fungal species
1
2005
... 菌根真菌作为生态系统重要的功能群之一, 在未受干扰的土壤中, 构成了植物根际区微生物的主体, 其生物量约占微生物总量的70% (
Shifting carbon flow from roots into associated microbial communities in response to elevated atmospheric CO2
1
2010
... 菌根真菌作为生态系统重要的功能群之一, 在未受干扰的土壤中, 构成了植物根际区微生物的主体, 其生物量约占微生物总量的70% (
Effects of trenching on soil processes and properties in a New Mexico mixed-conifer forest
1
1986
... 已经有一些研究在不同的生态系统采用不同的方法区分土壤呼吸组分以及定量每个组分分别对土壤总呼吸的贡献比例.一些方法是直接测定的, 比如离体根法: 直接测定所获得的活根的CO2释放量(
The spread of VA mycorrhizal fungal hyphae in the soil: Inoculum types and external hyphal architecture
1
1991
... 本实验样方于2012年5月建立, 每个样方大小为3 m × 4 m, 样方间隔为1 m, 总共包含4个重复.在每个样方中嵌入3个不同的土壤环, 用于土壤呼吸组分的区分, 分别为浅环、深环和菌丝环(
Arbuscular mycorrhizal colonization on carbon economy in perennial ryegrass: Quantification by 13CO2/ 12CO2 steady-state labelling and gas exchange
1
2006
... 通过2014-2016三个生长季的连续测定, 表明内蒙古半干旱草原异养呼吸占土壤总呼吸的比例为45%-54%, 根呼吸占土壤总呼吸的20%-34%, 菌根呼吸占土壤总呼吸的21%-26%.与国内外研究(
Separating root and soil microbial contributions to soil respiration: A review of methods and observations
2
2000
... 土壤呼吸是指由土壤产生并向大气中释放CO2的过程.由于从土壤表面释放的CO2有多种来源, 每个组分都包括不同的生物学和生态学过程, 对环境变化的响应可能也不同, 所以精确区分土壤呼吸的各种组分对于理解土壤呼吸机制本身及其对环境变化的响应是很关键的(
... 已经有一些研究在不同的生态系统采用不同的方法区分土壤呼吸组分以及定量每个组分分别对土壤总呼吸的贡献比例.一些方法是直接测定的, 比如离体根法: 直接测定所获得的活根的CO2释放量(
Forest soil CO2 flux: Uncovering the contribution and environmental responses of ectomycorrhizas
3
2007
... 已经有一些研究在不同的生态系统采用不同的方法区分土壤呼吸组分以及定量每个组分分别对土壤总呼吸的贡献比例.一些方法是直接测定的, 比如离体根法: 直接测定所获得的活根的CO2释放量(
... 菌根真菌作为生态系统重要的功能群之一, 在未受干扰的土壤中, 构成了植物根际区微生物的主体, 其生物量约占微生物总量的70% (
... 通过2014-2016三个生长季的连续测定, 表明内蒙古半干旱草原异养呼吸占土壤总呼吸的比例为45%-54%, 根呼吸占土壤总呼吸的20%-34%, 菌根呼吸占土壤总呼吸的21%-26%.与国内外研究(
Partitioning of soil CO2 flux components in a temperate grassland ecosystem
2
2012
... 菌根真菌作为生态系统重要的功能群之一, 在未受干扰的土壤中, 构成了植物根际区微生物的主体, 其生物量约占微生物总量的70% (
... 通过2014-2016三个生长季的连续测定, 表明内蒙古半干旱草原异养呼吸占土壤总呼吸的比例为45%-54%, 根呼吸占土壤总呼吸的20%-34%, 菌根呼吸占土壤总呼吸的21%-26%.与国内外研究(
Respiration of the external myceliumin the arbuscular mycorrhizal symbiosis shows strong dependence on recent photosynthates and acclimation to temperature
1
2006
... 土壤呼吸是指由土壤产生并向大气中释放CO2的过程.由于从土壤表面释放的CO2有多种来源, 每个组分都包括不同的生物学和生态学过程, 对环境变化的响应可能也不同, 所以精确区分土壤呼吸的各种组分对于理解土壤呼吸机制本身及其对环境变化的响应是很关键的(
Large-scale forest girdling shows that current photosynthesis drives soil respiration
1
2001
... 已经有一些研究在不同的生态系统采用不同的方法区分土壤呼吸组分以及定量每个组分分别对土壤总呼吸的贡献比例.一些方法是直接测定的, 比如离体根法: 直接测定所获得的活根的CO2释放量(
External hyphae of vesicular arbuscular mycorrhizal fungi associated with Trifolium subterraneum L. 2. Hyphal transport of 32P over defined distances
1
1992
... 在2016年生长季旺季8月中旬, 分别在每个样方的浅环、深环和菌丝环采集0-10 cm的土壤样品, 总共有4个重复, 用于菌丝密度的测定.参照
Carbon flow into soil and external hyphae from roots of mycorrhizal cucumber plants
1
1990
... 菌根真菌作为生态系统重要的功能群之一, 在未受干扰的土壤中, 构成了植物根际区微生物的主体, 其生物量约占微生物总量的70% (
Novel in-growth core system enables functional studies of grassland mycelial networks
1
2001
... 本研究以内蒙古半干旱草原生态系统为研究对象, 采用
Functional diversity and resource partitioning in fungi associated with the fine feeder roots of forest trees
1
2013
... 菌根真菌作为生态系统重要的功能群之一, 在未受干扰的土壤中, 构成了植物根际区微生物的主体, 其生物量约占微生物总量的70% (
Soil nutrient content influences the abundance of soil microbes but not plant biomass at the small-scale
1
2014
... 通过2014-2016三个生长季的连续测定, 表明内蒙古半干旱草原异养呼吸占土壤总呼吸的比例为45%-54%, 根呼吸占土壤总呼吸的20%-34%, 菌根呼吸占土壤总呼吸的21%-26%.与国内外研究(
Root and rhizomicrobial respiration: A review of approaches to estimate respiration by autotrophic and heterotrophic organisms in soil
1
2005
... 已经有一些研究在不同的生态系统采用不同的方法区分土壤呼吸组分以及定量每个组分分别对土壤总呼吸的贡献比例.一些方法是直接测定的, 比如离体根法: 直接测定所获得的活根的CO2释放量(
The influence of soil properties on the structure of bacterial and fungal communities across land-use types
1
2008
... 通过2014-2016三个生长季的连续测定, 表明内蒙古半干旱草原异养呼吸占土壤总呼吸的比例为45%-54%, 根呼吸占土壤总呼吸的20%-34%, 菌根呼吸占土壤总呼吸的21%-26%.与国内外研究(
Networks of power and influence: The role of mycorrhizal mycelium in controlling plant communities and agroecosystem functioning
1
2004
... 菌根真菌作为生态系统重要的功能群之一, 在未受干扰的土壤中, 构成了植物根际区微生物的主体, 其生物量约占微生物总量的70% (
Seasonal changes in the contribution of root respiration to total soil respiration in a cool-temperate deciduous forest
1
2003
... 已经有一些研究在不同的生态系统采用不同的方法区分土壤呼吸组分以及定量每个组分分别对土壤总呼吸的贡献比例.一些方法是直接测定的, 比如离体根法: 直接测定所获得的活根的CO2释放量(
In vitro root respirations of different diameter classes for Chinese firs in relation to root parameters
1
2008
... 已经有一些研究在不同的生态系统采用不同的方法区分土壤呼吸组分以及定量每个组分分别对土壤总呼吸的贡献比例.一些方法是直接测定的, 比如离体根法: 直接测定所获得的活根的CO2释放量(
How does temperature affect forest “fungus breath”? Diurnal non-exponential temperature- respiration relationship, and possible longer-term acclimation in fungal sporocarps
2
2017
... 菌根真菌作为生态系统重要的功能群之一, 在未受干扰的土壤中, 构成了植物根际区微生物的主体, 其生物量约占微生物总量的70% (
... 通过2014-2016三个生长季的连续测定, 表明内蒙古半干旱草原异养呼吸占土壤总呼吸的比例为45%-54%, 根呼吸占土壤总呼吸的20%-34%, 菌根呼吸占土壤总呼吸的21%-26%.与国内外研究(
1
2006
... 土壤呼吸是指由土壤产生并向大气中释放CO2的过程.由于从土壤表面释放的CO2有多种来源, 每个组分都包括不同的生物学和生态学过程, 对环境变化的响应可能也不同, 所以精确区分土壤呼吸的各种组分对于理解土壤呼吸机制本身及其对环境变化的响应是很关键的(
1
2006
... 土壤呼吸是指由土壤产生并向大气中释放CO2的过程.由于从土壤表面释放的CO2有多种来源, 每个组分都包括不同的生物学和生态学过程, 对环境变化的响应可能也不同, 所以精确区分土壤呼吸的各种组分对于理解土壤呼吸机制本身及其对环境变化的响应是很关键的(
内蒙古温带草地生物量及其与环境因子的关系
1
2008
... 本实验样方于2012年5月建立, 每个样方大小为3 m × 4 m, 样方间隔为1 m, 总共包含4个重复.在每个样方中嵌入3个不同的土壤环, 用于土壤呼吸组分的区分, 分别为浅环、深环和菌丝环(
内蒙古温带草地生物量及其与环境因子的关系
1
2008
... 本实验样方于2012年5月建立, 每个样方大小为3 m × 4 m, 样方间隔为1 m, 总共包含4个重复.在每个样方中嵌入3个不同的土壤环, 用于土壤呼吸组分的区分, 分别为浅环、深环和菌丝环(
External hyphal production of vesicular-arbuscular mycorrhizal fungi in pasture and tallgrass prairie communities
1
1995
... 在2016年生长季旺季8月中旬, 分别在每个样方的浅环、深环和菌丝环采集0-10 cm的土壤样品, 总共有4个重复, 用于菌丝密度的测定.参照
Soil respiration fluxes in relation to photosynthetic activity in broad-leaf and needle-leaf forest stands
1
2008
... 已经有一些研究在不同的生态系统采用不同的方法区分土壤呼吸组分以及定量每个组分分别对土壤总呼吸的贡献比例.一些方法是直接测定的, 比如离体根法: 直接测定所获得的活根的CO2释放量(
Response of mycorrhizal, rhizosphere and soil basal respiration to temperature and photosynthesis in a barley field
1
2007
... 通过2014-2016三个生长季的连续测定, 表明内蒙古半干旱草原异养呼吸占土壤总呼吸的比例为45%-54%, 根呼吸占土壤总呼吸的20%-34%, 菌根呼吸占土壤总呼吸的21%-26%.与国内外研究(
Contribution of newly grown extramatrical ectomycorrhizal mycelium and fine roots to soil respiration in a young Norway spruce site
3
2014
... 土壤呼吸是指由土壤产生并向大气中释放CO2的过程.由于从土壤表面释放的CO2有多种来源, 每个组分都包括不同的生物学和生态学过程, 对环境变化的响应可能也不同, 所以精确区分土壤呼吸的各种组分对于理解土壤呼吸机制本身及其对环境变化的响应是很关键的(
... 菌根真菌作为生态系统重要的功能群之一, 在未受干扰的土壤中, 构成了植物根际区微生物的主体, 其生物量约占微生物总量的70% (
... 通过2014-2016三个生长季的连续测定, 表明内蒙古半干旱草原异养呼吸占土壤总呼吸的比例为45%-54%, 根呼吸占土壤总呼吸的20%-34%, 菌根呼吸占土壤总呼吸的21%-26%.与国内外研究(
Arbuscular mycorrhizal mycelial respiration in a moist tropical forest
2
2010
... 菌根真菌作为生态系统重要的功能群之一, 在未受干扰的土壤中, 构成了植物根际区微生物的主体, 其生物量约占微生物总量的70% (
... 通过2014-2016三个生长季的连续测定, 表明内蒙古半干旱草原异养呼吸占土壤总呼吸的比例为45%-54%, 根呼吸占土壤总呼吸的20%-34%, 菌根呼吸占土壤总呼吸的21%-26%.与国内外研究(
Carbon efflux rates of fine roots of three tree species decline shortly after excision
1
1997
... 已经有一些研究在不同的生态系统采用不同的方法区分土壤呼吸组分以及定量每个组分分别对土壤总呼吸的贡献比例.一些方法是直接测定的, 比如离体根法: 直接测定所获得的活根的CO2释放量(
Soil biota responses to long-term atmospheric CO2 enrichment in two California annual grasslands
1
1999
... 在2016年生长季旺季8月中旬, 分别在每个样方的浅环、深环和菌丝环采集0-10 cm的土壤样品, 总共有4个重复, 用于菌丝密度的测定.参照
Indirect partitioning of soil respiration in a series of evergreen forest ecosystems
1
2006
... 已经有一些研究在不同的生态系统采用不同的方法区分土壤呼吸组分以及定量每个组分分别对土壤总呼吸的贡献比例.一些方法是直接测定的, 比如离体根法: 直接测定所获得的活根的CO2释放量(
Mycorrhizae alter quality and quantity of carbon allocated below ground
2
1994
... 菌根真菌作为生态系统重要的功能群之一, 在未受干扰的土壤中, 构成了植物根际区微生物的主体, 其生物量约占微生物总量的70% (
... 通过2014-2016三个生长季的连续测定, 表明内蒙古半干旱草原异养呼吸占土壤总呼吸的比例为45%-54%, 根呼吸占土壤总呼吸的20%-34%, 菌根呼吸占土壤总呼吸的21%-26%.与国内外研究(
Assessment of soil CO2 efflux and its components using a process-based model in a young temperate forest site
1
2007
... 为了消除土壤环境变化对深环所测得土壤呼吸(SRdc)与菌丝环所测得土壤呼吸(SRhc)的影响, 本研究采用一个幂指数-二次方程来模拟它们与土壤温度(Ts)和土壤含水量(SWC)之间的关系(
Study on the distinguishing of root respiration from soil microbial respiration in a Leymus chinensis steppe in Inner Mongolia, China
1
2014
... 已经有一些研究在不同的生态系统采用不同的方法区分土壤呼吸组分以及定量每个组分分别对土壤总呼吸的贡献比例.一些方法是直接测定的, 比如离体根法: 直接测定所获得的活根的CO2释放量(
Monitoring CO2 emissions to gain a dynamic view of carbon allocation to arbuscular mycorrhizal fungi
1
2017
... 土壤呼吸是指由土壤产生并向大气中释放CO2的过程.由于从土壤表面释放的CO2有多种来源, 每个组分都包括不同的生物学和生态学过程, 对环境变化的响应可能也不同, 所以精确区分土壤呼吸的各种组分对于理解土壤呼吸机制本身及其对环境变化的响应是很关键的(
1
2008
... 菌根真菌作为生态系统重要的功能群之一, 在未受干扰的土壤中, 构成了植物根际区微生物的主体, 其生物量约占微生物总量的70% (
Decomposers in disguise: Mycorrhizal fungi as regulators of soil C dynamics in ecosystems under global change
1
2008
... 菌根真菌作为生态系统重要的功能群之一, 在未受干扰的土壤中, 构成了植物根际区微生物的主体, 其生物量约占微生物总量的70% (
Global diversity and geography of soil fungi
1
2014
... 通过2014-2016三个生长季的连续测定, 表明内蒙古半干旱草原异养呼吸占土壤总呼吸的比例为45%-54%, 根呼吸占土壤总呼吸的20%-34%, 菌根呼吸占土壤总呼吸的21%-26%.与国内外研究(
Mycorrhizal contribution to soil respiration in an apple orchard
3
2016
... 已经有一些研究在不同的生态系统采用不同的方法区分土壤呼吸组分以及定量每个组分分别对土壤总呼吸的贡献比例.一些方法是直接测定的, 比如离体根法: 直接测定所获得的活根的CO2释放量(
... 通过2014-2016三个生长季的连续测定, 表明内蒙古半干旱草原异养呼吸占土壤总呼吸的比例为45%-54%, 根呼吸占土壤总呼吸的20%-34%, 菌根呼吸占土壤总呼吸的21%-26%.与国内外研究(
... ), 土壤温度(
Rhizospheric and heterotrophic components of soil respiration in six Chinese temperate forests
1
2007
... 菌根真菌作为生态系统重要的功能群之一, 在未受干扰的土壤中, 构成了植物根际区微生物的主体, 其生物量约占微生物总量的70% (
Soil aggregation and carbon sequestration are tightly correlated with the abundance of arbuscular mycorrhizal fungi: Results from long-term field experiments
1
2009
... 菌根真菌作为生态系统重要的功能群之一, 在未受干扰的土壤中, 构成了植物根际区微生物的主体, 其生物量约占微生物总量的70% (
Ecosystem respiration in a young ponderosa pine plantation in the Sierra Nevada Mountains, California
1
2001
... 已经有一些研究在不同的生态系统采用不同的方法区分土壤呼吸组分以及定量每个组分分别对土壤总呼吸的贡献比例.一些方法是直接测定的, 比如离体根法: 直接测定所获得的活根的CO2释放量(
Differential responses of auto- and heterotrophic soil respiration to water and nitrogen addition in a semiarid temperate steppe
2
2010
... 菌根真菌作为生态系统重要的功能群之一, 在未受干扰的土壤中, 构成了植物根际区微生物的主体, 其生物量约占微生物总量的70% (
... 为了消除土壤环境变化对深环所测得土壤呼吸(SRdc)与菌丝环所测得土壤呼吸(SRhc)的影响, 本研究采用一个幂指数-二次方程来模拟它们与土壤温度(Ts)和土壤含水量(SWC)之间的关系(
Seasonal variations in soil respiration, heterotrophic respiration and autotrophic respiration of a wheat and maize rotation cropland in the North China Plain
1
2013
... 菌根真菌作为生态系统重要的功能群之一, 在未受干扰的土壤中, 构成了植物根际区微生物的主体, 其生物量约占微生物总量的70% (
外生菌根菌与森林树木的相互关系
1
2003
... 菌根真菌作为生态系统重要的功能群之一, 在未受干扰的土壤中, 构成了植物根际区微生物的主体, 其生物量约占微生物总量的70% (
外生菌根菌与森林树木的相互关系
1
2003
... 菌根真菌作为生态系统重要的功能群之一, 在未受干扰的土壤中, 构成了植物根际区微生物的主体, 其生物量约占微生物总量的70% (
Copyright © 2021 版权所有 《植物生态学报》编辑部
地址: 北京香山南辛村20号, 邮编: 100093
Tel.: 010-62836134, 62836138; Fax: 010-82599431; E-mail: apes@ibcas.ac.cn, cjpe@ibcas.ac.cn
备案号: 京ICP备16067583号-19