HTML
--> --> -->Still, through what mechanisms the ocean dynamics buffers the ITCZ shift remains an issue of debate, especially concerning the relative importance of the wind-driven and the thermohaline dynamical processes. Recently, Green and Marshall (2017) coupled the atmosphere with a dynamic ocean in a highly idealized two-basin model and perturbed the system with a hemispherically asymmetric forcing (that is, heating in the southern hemisphere but cooling in the northern hemisphere). Compared to the motionless slab ocean model, they found the ITCZ shift in the coupled model to be considerably damped by a factor of 4. This damped ITCZ shift response has been ascribed to the coupling between the Hadley cell and the ocean’s wind-driven subtropical cell. Specifically, in response to the southern hemisphere heating and northern hemisphere cooling, the easterly trade winds show a weakening in the southern tropics and strengthening in the northern tropics, both wind anomalies act to induce an anomalous northward Ekman flow in the upper ocean. This cross-equatorial flow transports warm water and energy northward, strongly reducing the burden of the AHT and offsetting the imposed energy contrast between hemispheres, thus buffering the shift of ITCZ. In addition, this important role of wind-driven circulation in muting ITCZ shift has been discussed in other modeling studies (e.g., Held, 2001; Schneider et al., 2014; Kay et al., 2016).
Some other studies suggested that changes in Atlantic meridional overturning circulation (AMOC) can also contribute to damping the ITCZ shift (Frierson et al., 2013; Fu?kar et al., 2013; Marshall et al., 2014; He et al., 2019; Yu and Pritchard, 2019), because the AMOC can transport an energy anomaly across the equator, reducing the hemispherical energy imbalance and thereby muting the ITCZ shift. In particular, a recent work of Yu and Pritchard (2019) performed similar experiments as Green and Marshall (2017) but with a more realistic fully coupled model. Through placing hemispherically asymmetric perturbations in different latitudes, they found increasing importance of the AMOC responses in muting the ITCZ shift, and the AMOC becomes equally important as the wind-driven subtropical cells when the forcing is placed at high latitudes.
The above studies highlighted the important role of ocean dynamics in partitioning cross-equatorial energy transport and in modulating the ITCZ shift, but much work remains to be done to understand the physical mechanisms. Here, we employ the slab ocean and fully coupled version of the Community Earth System Model 1 (CESM1) to systematically compare the climate response to a pair of heating and cooling forcings placed upon the Southern Ocean. The reason we place the forcing in the Southern Ocean is due to its large climatic influence (Hwang et al., 2017; Liu et al., 2018b), and to facilitate the comparison with many previous studies that placed external forcing in similar regions (Kay et al., 2016; Hawcroft et al., 2017; Hwang et al., 2017; Yu and Pritchard, 2019). To understand how ocean dynamics modulate the cross-equatorial energy transport, we further perform a set of partially coupled experiments in which the wind stress is prescribed to a repeating climatology, and thereby the wind-driven oceanic circulation responses can be isolated. As to be shown later, the ocean can transport a similar amount of energy across the equator even after the wind-driven ocean dynamical adjustment is disabled, and the response in ocean heat transport (OHT) is predominantly thermohaline-driven by air-sea thermal interactions.
The remainder of the paper is structured as follows. Section 2 describes the slab ocean and the fully coupled model, the design of experiments, and metrics used in this study. Section 3 compares the climate response in the slab ocean and fully coupled experiments. Section 4 illustrates how ocean dynamics modulates the cross-equatorial energy transport. Section 5 summarizes the main conclusions of the study and provides a brief discussion.
2.1. Coupled and partially coupled experiments
The model we use includes both the fully coupled and the slab ocean version of the Community Earth System Model version 1 (CESM1). The fully coupled model (CESM1-CPL) is comprised of the Community Atmosphere Model version 5, the Community Land Model version 4, the Community Ice CodE, and the Parallel Ocean Program version 2. For both atmosphere and land models, the horizontal resolution is 2.5° longitude × 1.9° latitude, with the atmospheric component discretized on 30 uneven vertical levels. For the sea ice model and ocean models, the horizontal resolution is at a nominal 1°, telescoped meridionally to ~0.3° at the equator. Vertically, the ocean model has 60 uneven levels with the thickness varying from 10 m near the surface to 250 m at the bottom. It should be noted that we run all the following experiments with perpetual equinox conditions, which reduces complexities in the results.Starting from an equilibrium state that is available at NCAR, a control simulation (CTRL-CPL; Table 1) is integrated for 120 years under equinoctial solar radiation. We then perform two experiments with additional heating and cooling band forcing













NAME | RUN (yrs) | DESCRIPTION |
Fully coupled experiments | ||
CTRL-CPL | 120 | Fully coupled control run |
HEAT-CPL | 120 | Perturbed by an additional heating in the Southern Ocean |
COOL-CPL | 70 | Perturbed by an additional cooling in the Southern Ocean |
Wind stress overriding experiments | ||
HEAT-WS | 70 | Same as HEAT-CPL, but wind stress is specified to climatology |
COOL-WS | 70 | Same as COOL-CPL, but wind stress is specified to climatology |
Slab ocean experiments | ||
CTRL-SOM | 100 | Slab ocean control run |
HEAT-SOM | 80 | Perturbed by an additional heating in the Southern Ocean |
COOL-SOM | 80 | Perturbed by an additional cooling in the Southern Ocean |
Table1. Experiments with fully coupled and slab ocean CESM1


To disable the wind-driven oceanic processes, we further perform a pair of partially coupled heating and cooling experiments (HEAT-WS and COOL-WS; Table 1). The partial coupling is realized through overriding the wind stress at the air-sea interface to a daily mean climatology (also see Liu et al., 2017a for details), which is derived from the 120-year CTRL-CPL run. Therefore, the OHT, as well as ocean circulation changes, are a result of air-sea thermal interaction, and the wind stress contribution to the oceanic changes can be estimated by subtracting the results of CPL-WS from those in CPL. This technique has been successfully used to examine the formation processes of SST in response to global warming in many previous studies (Lu and Zhao, 2012; Luo et al., 2015; Liu et al., 2017a, b).
2
2.2. Slab ocean experiments
To test the impact of the active ocean dynamics, we also use a slab ocean version of CESM1 (CESM1-SOM). In this model, the ocean and atmosphere are only thermodynamically coupled, and SST is computed from surface heat flux and q-flux that accounts for the missing ocean dynamics. We integrate a slab ocean control run (CTRL-SOM) for 100 years, both the mixed layer depth and the q-flux are derived from the climatology of CTRL-CPL. The q-flux is allowed to vary in space and has a repeating seasonal cycle, while the mixed layer depth is only allowed to vary in space. Branching out from the 21st year of the control run, a pair of heating and cooling experiments are integrated for 80 years with the same band forcing
The zonal mean climatology of surface temperature (TS), precipitation, and AHT in the coupled and the slab models are compared in Figs. 1b–d. Although some minor differences can be found between them, the large-scale distributions are remarkably similar, legitimizing the following comparison of their responses to the same external forcing.
2
2.3. Oceanic heat transport
Following Yang et al. (2015), the zonally integrated full-depth OHT (
where






The response of the Eulerian-mean OHT can be further decomposed into the advection of mean temperature by the circulation anomaly, the advection of temperature anomaly by mean circulation, and a nonlinear component, i.e.,
Following Yu and Pritchard (2019), we term them as dynamic, thermodynamic, and nonlinear components, respectively.
On the other hand, the total OHT can be separated into contributions from individual basins. Since the mass flow in the Indian Ocean or the Pacific Ocean alone is not closed due to the Indonesian Throughflow, the heat transport of the two basins is summed together.
2
2.4. Meridional Overturning Circulation
The MOC is calculated by integrating meridional velocity zonally and vertically. Since the meridional velocity can be decomposed into Eulerian-mean and eddy-induced components


A heating perturbation in the Southern Ocean will inject energy into the surface ocean and warm both the ocean and the overlying atmosphere, consequently, the TS response in the Southern Ocean is characterized by a circumpolar belt of enhanced warming. Some of the warmth spreads to the northern hemisphere, especially over the monsoonal regions (Figs. 3a, b). As the ITCZ tends to shift towards the warmed hemisphere, there is a clear meridional dipole in the precipitation response, indicative of a southward shift (Figs. 3c, d). The pattern of zonal wind stress anomalies shows a weakening of the trade winds in the southern hemisphere and a strengthening in the northern hemisphere, which is consistent with a southward shift of the ITCZ (Figs. 3c, f). These results agree well with Hwang et al (2017), who examined the global response to a change in the Southern Ocean heat uptake within a slab ocean model. There is a notable spatial similarity in the precipitation response between the SOM and CPL experiments (pattern correlation = 0.63), indicative of the energetic constraint on the ITCZ response. The similarity is also present in the response of zonal wind stress, despite the active ocean dynamical feedback in the CPL case. In response to the surface warming in the Southern Ocean, the sea ice poleward of 55°S experiences a clear decrease in both models. In contrast, the sea ice melting in the northern hemisphere is much weaker, corresponding to the relatively small TS warming there. Note that the heat flux perturbation is specified as a meridionally-oriented cosine hump, the forcing magnitude decreases from 12









Figure 4 shows the response of AHT and the shift of ITCZ. The location of the ITCZ is measured as the latitudinal centroid of precipitation P
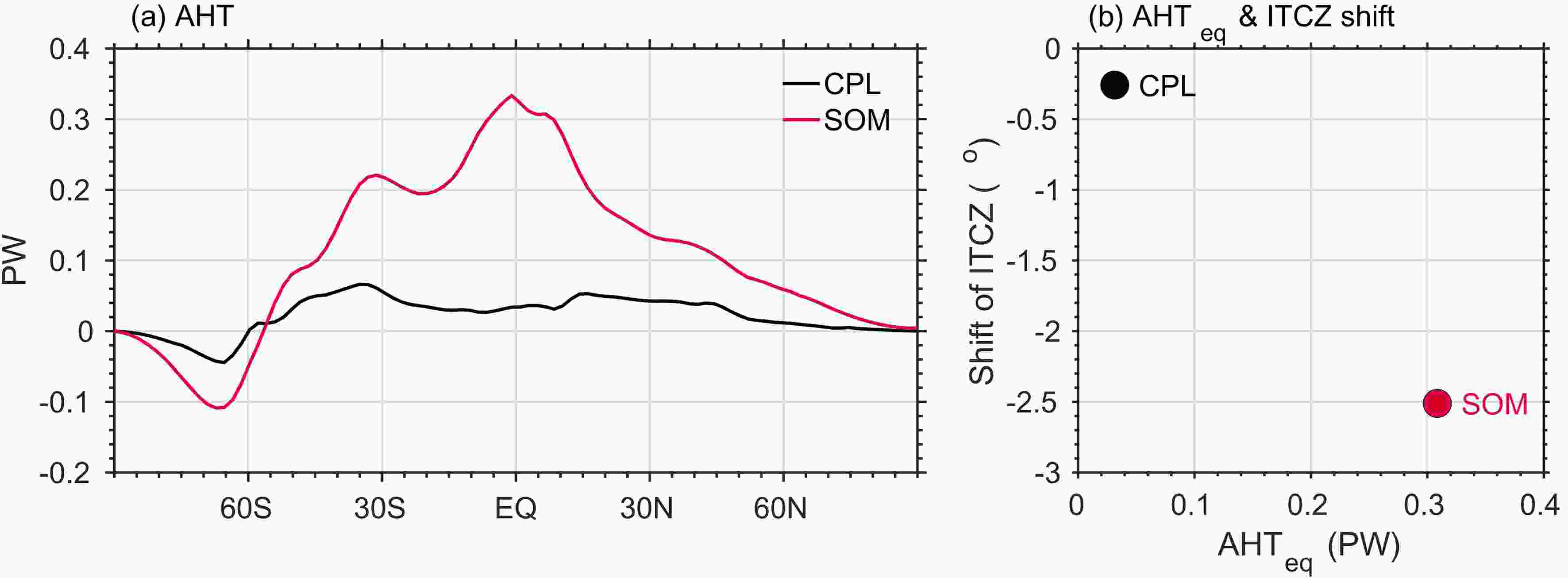
where



The mean OHT and MOC as well as their decomposition are shown in Fig. 5. Overall, the background mean OHT is featured with a hemispherically antisymmetric poleward heat transport, which is in good agreement with many previous studies (Held, 2001; Trenberth and Caron, 2001; Czaja and Marshall, 2006; Yang and Dai, 2015). As explained in Section 2.3, the total OHT can be decomposed into contributions from the Eulerian-mean circulation, the eddies, and diffusive processes. The total OHT is dominated by its Eulerian-mean component except in the Southern Ocean where eddies play a dominant role, as the poleward Eulerian-mean OHT observed there is largely suppressed due to the northward circumpolar Ekman flow. Therefore, the total southward OHT around 40°S is primarily maintained by the mesoscale and sub-mesoscale eddies (Jayne and Marotzke, 2002; Treguier et al., 2007; Yang and Dai, 2015). Figures 5d and 5f show the mean MOC in temperature-latitude coordinates. In this way, we can intuitively examine the heat transport due to different circulation features by comparing the temperature difference between the upper and lower branches (Held, 2001). Another advantage provided by this temperature coordinate plot is a clear separation of warm subtropical cells and cold deep cells. The warm cells are mostly wind-driven and span the temperature range from 10°C in the mid-latitudes to 28°C at the equator, whereas the cold cell is largely the thermohaline AMOC and spans the temperature between 2°C to 10°C. It is also evident that the eddy-induced transport acts to counter the Eulerian transport in the mid-to-high latitudes in the Southern Ocean (Fig. 5f). All of these features are in good agreement with previous studies (Farneti and Vallis, 2013; Yang et al., 2015, Dai et al., 2017).

When extra heat is injected into the Southern Ocean, a dynamically active ocean will transport energy northward, in the same direction as the atmosphere (Fig. 6). The anomalous cross-equatorial OHT largely reduces the burden of AHT compared to SOM (Fig. 4) and hence damps the shifting response of the ITCZ. A further decomposition shows that the change in the total OHT is dominated by its Eulerian-mean component (blue lines in Fig. 6). The eddy and diffusive components individually may not be negligible in the Southern Ocean, yet there is a large cancellation between the two components and their combination contributes negligibly to the total OHT response. Another important feature in the OHT response is that both the Indo-Pacific and Atlantic are important in transporting the anomalous energy northward (Figs. 6b, c). Of the total 0.18 PW cross-equatorial OHT response, the contribution from the Atlantic is 0.08 PW, slightly smaller in magnitude than that from the Indo-Pacific (0.10 PW). This result supports the recent finding of Yu and Pritchard (2019) that the Atlantic is an equally important contributor as the Indo-Pacific when the forcing is placed in high latitudes. On the other hand, due to a much smaller transport in the Pacific through the Bering Strait (Aagaard and Greisman 1975), the Atlantic dominates the anomalous OHT in the high latitudes of the northern hemisphere.

Since the Eulerian-mean component dominates the total OHT response, we then focus on its response and decompose it into the dynamic, thermodynamic, and nonlinear components (Eq. 2). The dynamic OHT response refers to the advection of the mean temperature by the circulation anomaly, while the thermodynamic response can be conceptualized as the advection of the temperature anomaly by the mean circulation. Figure 7a shows the cross-equatorial Eulerian-mean OHT and its breakdown in individual basins as well as its different components. Overall, the dynamic component has a much larger contribution to the global OHT response than the thermodynamic one, though differences exist in different basins. In the Indo-Pacific, the northward dynamic OHT response overwhelms the southward thermodynamic response, resulting in a net northward OHT anomaly. In contrast, the thermodynamic and dynamic components work in tandem to enhance the northward OHT in the Atlantic, although the magnitude of the former is somewhat smaller. The nonlinear response is not discussed here because of its negligible contribution.

In the Indo-Pacific, the ocean can transport large amounts of energy across the equator by changing its circulation (dynamic component), which appears to agree with the mechanism proposed by Green and Marshall (2017) that relates the change in cross-equatorial OHT to the change in wind stress in a highly idealized coupled model. Specifically, in response to the intensified (suppressed) easterly trades to the north (south) of the equator (Fig. 3e), the poleward Ekman flow in the upper ocean will be enhanced (weakened) to the north (south), leading to an anomalous northward Ekman flow across the equator. Since this anomalous northward flow stimulates a clockwise oceanic circulation centered at the equator and transports relatively warm (cold) water northward (southward) in the upper (lower) ocean, the atmosphere does not need to transport as much energy across the equator as in the SOM. Therefore, the oceanic circulation in the tropical Pacific is coupled to the atmospheric circulation through the zonal wind stress (e.g., Held, 2001; Green and Marshall, 2017; Schneider, 2017; Kang et al., 2018).
To examine the above mechanism highlighting the role of wind stress, we rerun the heating and cooling experiments, but with the wind-driven ocean circulation feedback disabled by prescribing the wind stress in every model step (CPL-WS experiments). The resultant OHT response and its decomposition are presented in Fig. 7b. As expected, due to the dominant role of the thermohaline circulation in driving the Atlantic OHT (Yang et al., 2015), shutting down the wind stress effect barely influences the total OHT in the Atlantic basin. However, it is intriguing that the Indo-Pacific is capable of transporting similar amounts of energy across the equator (Fig. 7b), with the wind-driven feedback effect accounting for only 1/6 of the 0.12 PW cross-equatorial OHT response. The wind-induced reduction in the cross-equatorial OHT is consistent in sign with what is proposed in Green and Marshall (2017), but its amplitude is much weaker. This result suggests that the circulation changes, as well as OHT changes in the Indo-Pacific, are largely thermohaline-driven rather than wind-driven.
To elaborate on the circulation-induced energy transport across the equator, we next show the changes in the MOC (Fig. 8) and zonal mean temperature (Fig. 9), which have been identified to be responsible for the dynamic and thermodynamic components of the OHT responses mentioned above. Note that only the Eulerian-mean MOC response is presented because of its dominant role in driving the total OHT (Fig. 6). As expected, the strengthened (weakened) easterlies in the northern (southern) tropics lead to positive stream function anomalies within the subtropical cells in both hemispheres (Figs. 8a, b). However, a more prominent response is the clockwise MOC anomaly near the lower boundary of the southern subtropical cell that is centered between the warm and cold cells (Figs. 8a, d). This anomalous circulation occupies a large fraction of the upper ocean in the southern hemisphere and extends to the northern hemisphere, bounded approximately by the 5°C to 20°C isotherms (0–1000 m, Figs. 2b, c) and spanning a latitudinal range from 50°S to 10°N, with the maximum change exceeding 2.5 Sv (1 Sv



Note both the heat flux perturbation and the related sea ice melting(Figs. 4g, h) can serve as surface buoyancy sources and thus contribute to the thermohaline circulation changes. To quantitatively separate the respective contributions from the heat flux and the freshwater flux, we computed the buoyancy flux

where SHF is the net surface heat flux, FWF is the net freshwater flux into the surface,
































When the surface wind stress is prescribed and the ocean circulation is solely driven by the buoyancy flux, the clockwise MOC anomaly in the subtropical cells largely disappeared (cf. Figs. 8b, d), resulting in only ~20% reduction in the dynamical OHT across the equator (Fig. 7b). This much smaller contribution from the wind-driven response can be explained by the very weak cross-equatorial component of flow in the wind-induced subtropical cell response (Figs 8a, b), in contrast to what is depicted in Green and Marshall (2017; see their Fig. 6). Therefore, the MOC anomaly due to the thermal effect of the warming plays a much more important role in both ocean basins.
As shown in Fig. 7, the advection of temperature anomaly by the mean circulation also contributes to the cross-equatorial OHT response. Figure 9 shows the zonal mean temperature anomalies overlaid on top of the background mean MOC. The positive contribution to OHT from the thermodynamic component in the Atlantic (Fig. 7a) can be readily attributed to the ocean temperature change. The warming in the Atlantic is mainly confined in the upper branch of the Atlantic MOC that is directed northward throughout the basin, giving rise to a net northward ocean heat transport. In the Indo-Pacific, the almost symmetric subtropical cells with respect to the equator only project weakly onto the cross-equatorial flow (in fact, weakly southward in the upper branch), thus the mean subtropical cell transports the anomalous warmth southward, contributing negatively to the total northward OHT response at the equator. Overall, similar thermodynamic contributions still hold in the CPL-WS experiments, because the response of the wind-driven circulation is of secondary importance to begin with regarding the redistribution of heat in the global oceans (comparing the lower panel to the upper panel in Fig. 9). The weak subsurface cooling in the tropics of the Indo-Pacific Ocean is a result of thermocline shoaling, which is in turn caused by the cyclonic wind stress curl anomaly there (e.g., Luo et al., 2015).
Since the wind stress overriding experiments already show that the robust MOC anomaly between warm and cold cells is not wind-driven (Fig. 8), it can therefore be understood by looking into the temperature and density responses. According to Fig. 11, the water density is reduced more in the Southern Ocean than in the tropics, inducing a tilting of the density surface. Conceptually, supposing the circulation anomaly is solely caused by changes in density (Figs. 11d, f), on the same pressure level, the anomalous lighter seawater in the Southern Ocean would be subjected to the same upward pressure gradient force as the anomalous heavier seawater near the tropics. Consequently, the lighter seawater tends to rise more rapidly than the heavier seawater, resulting in a clockwise circulation anomaly between the Southern Ocean and the tropics, which alternatively can be thought of as being driven by a negative solenoidal effect in the zonal direction.


● Perturbing the Southern Ocean with an external thermal forcing leads to northward cross-equatorial energy transport and a southward ITCZ shift. However, the ITCZ shift in a slab ocean model is largely overestimated as the interhemispheric energy imbalance can only be restored by the atmosphere alone. When active ocean dynamics are included, most of the cross-equatorial energy transport takes place in the ocean, thus significantly alleviating the burden of the atmosphere in cross-equatorial energy transport and reducing the shift in ITCZ by a factor of 10. This demonstrates the profound importance of ocean dynamics upon the buffering of global energetics.
● Both the Indo-Pacific and the Atlantic can contribute to the northward cross-equatorial OHT mainly through changing its Eulerian-mean circulation (i.e., the dynamic component), while the changes in the thermodynamic component can explain about 30% of the cross-equatorial OHT response in the Atlantic.
● The major Eulerian-mean circulation change responsible for the dynamic OHT response is a clockwise anomalous overturning circulation below the southern hemisphere subtropical cell. The large temperature difference between its upper and lower branches makes it efficient in transporting energy northward and across the equator in both the Indo-Pacific and the Atlantic Oceans.
● The above circulation change is largely attributed to the thermohaline and is driven by the density anomalies. Therefore, shutting down the wind stress only exerts limited impacts on cross-equatorial OHT response.
The above results first verify the finding in recent studies (Kay et al., 2016; Hawcroft et al., 2017; Green and Marshall, 2017; Yu and Pritchard, 2019), i.e., the inclusion of a dynamical ocean would lead to the partitioning of the cross-equatorial energy transport response to external heating forcing in the Southern Ocean, and thereby largely reduce the ITCZ shift response compared to that in the climate system with a motionless slab ocean model. Most of the existing hypotheses for the ocean’s buffering effect involve the mechanisms related to changes in subtropical cells (Held, 2001; Schneider et al., 2014; Kay et al., 2016; Green and Marshall, 2017) or in the AMOC (Frierson et al., 2013; Fu?kar et al., 2013; Marshall et al., 2014; Yu and Pritchard, 2019). However, through a wind stress overriding experiment and a careful examination of the responses in OHT and ocean circulation, we find that the buffering effect of the ocean has resulted from a circulation anomaly that covers a broad range of the southern hemisphere ocean and extends to the northern hemisphere ocean. This circulation anomaly is largely thermohaline driven and can efficiently transport anomalous energy northward, which in turn reduces the response in the cross-equatorial AHT and the ITCZ shift.
It should be noted that, although the contribution from wind stress changes to the cross-equatorial OHT is relatively small in this study, it may vary according to the location of the forcing. The energy forcing here is placed in the Southern Ocean, where deep ocean convection prevails, and the surface thermal forcing can penetrate deeper and effectively alter the thermal and density structure of the interior ocean and thus induce an anomalous thermohaline circulation (Figs. 9 and 11). However, if the forcing were placed within the tropics or even the entire southern hemisphere, the changes in wind stress and the associated wind-driven subtropical cells may become larger and contribute more to the cross-equatorial OHT. We leave this issue for future investigation.
Acknowledgments. This work is supported by the National Key Research and Development Program of China (2018YFA0605702) and the National Natural Science Foundation of China (NSFC; 41906002, 91858210, 41976006, and 41776009). The model outputs generated and/or analyzed during the current study will be available to the public at (