

Characteristics of maize grain dehydration and prediction of suitable harvest period in Liao River Basin
HUANG Zhao-Fu1,2,*, MING Bo2,*, WANG Ke-Ru2, XIE Rui-Zhi2, YANG Fei1, WANG Zhi-Gang3, XIAO Chun-Hua

通讯作者:
收稿日期:2018-08-20接受日期:2019-01-12网络出版日期:2019-02-07
基金资助: |
Received:2018-08-20Accepted:2019-01-12Online:2019-02-07
Fund supported: |
作者简介 About authors
黄兆福,E-mail:
;明博,E-mail:

摘要
关键词:
Abstract
Keywords:
PDF (3912KB)元数据多维度评价相关文章导出EndNote|Ris|Bibtex收藏本文
本文引用格式
黄兆福, 明博, 王克如, 谢瑞芝, 杨飞, 王志刚, 肖春华, 李少昆. 辽河流域玉米籽粒脱水特点及适宜收获期分析[J]. 作物学报, 2019, 45(6): 922-931. doi:10.3724/SP.J.1006.2019.83062
HUANG Zhao-Fu, MING Bo, WANG Ke-Ru, XIE Rui-Zhi, YANG Fei, WANG Zhi-Gang, XIAO Chun-Hua, LI Shao-Kun.
辽河流域是东北重要的玉米产区, 处于春播一熟制玉米种植区南部, 热量资源相对丰富, 玉米适收期长, 是推广玉米机械粒收技术的优势区域[1,2]。前期宜机收品种鉴选和机械粒收技术示范研究显示, 籽粒破碎和产量损失是辽河流域机械粒收所面临的主要质量问题[3,4,5], 与收获时籽粒含水率偏高密切相关[6,7,8,9,10]。国外机械粒收时籽粒含水率一般在15%~25%, 收获高峰期晚于生理成熟高峰期约1个月[11,12]。玉米品种脱水特性[13]和收获时期[14]是决定收获时籽粒含水率的关键。籽粒脱水过程受温度、湿度、风速、降水等气象因素的推动和影响[15,16,17,18,19]。基于温度、湿度等气象因子可以有效模拟籽粒含水率的变化过程[20,21]。因此, 研究品种的籽粒脱水特征、分析其适收期是解决机械粒收质量问题的关键[22,23]。本研究通过调查多个熟期品种籽粒含水率动态变化, 建立不同品种籽粒脱水模型, 基于区域常年气象条件分析其适宜粒收时期, 为东北春玉米区机械粒收技术推广提供依据。
1 材料与方法
1.1 试验点及气象条件
辽河流域位于中国东北地区南部, 跨内蒙古、辽宁、吉林、河北四省区, 面积21.9万平方千米, 属温带半湿润半干旱的季风气候, 无霜期大于160 d, 年均温7~10°C, 年降水量约为350~1000 mm, 从东南向西北递减, 年降水量的65%集中于4月至9月间。辽河流域西部通辽、赤峰, 及中下游地区的吉林省四平、辽源、辽宁省铁岭、沈阳、辽阳、鞍山、营口一线是东北重要的玉米产区。区域内以春播一熟制玉米种植模式为主[24], 是东北春玉米产区中热量资源相对丰富的地区, 玉米适收期长[25]。辽宁中部地区玉米生育季内(4月至10月)活动积温(≥0°C积温)最高, 为3750~4000°C d, 年降水量450~700 mm; 内蒙古自治区的西辽河平原通辽地区活动积温在3600~3750°C d, 燕山北麓平原赤峰地区活动积温3300~3600°C d, 内蒙古辖区内年降水量较少, 在350~450 mm之间(图1)。图1
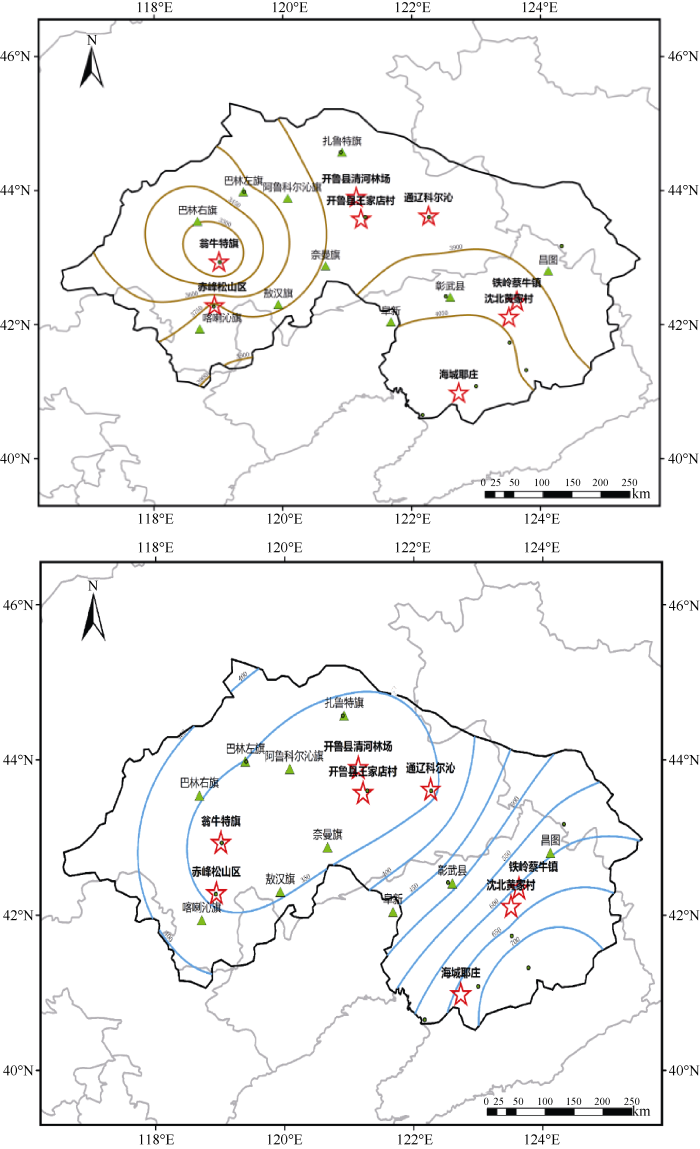
图1辽河流域积温、降水资源分布及试验点
Fig. 1Distribution of thermal and water resources and test yields in Liaohe river basin
1.2 试验设计与参试品种
2013—2017年间在内蒙古自治区通辽市科尔沁区、通辽市开鲁县、赤峰市松山区、赤峰市翁牛特旗, 辽宁省铁岭市蔡牛镇、沈阳市沈北区、鞍山市海城等地开展了2个省区、7个区县、18点次的品种鉴选和机械粒收质量评价试验。2017年, 选择该区域主推的29个不同熟期玉米品种(表1), 分别在内蒙古自治区通辽市开鲁县和辽宁省铁岭市铁岭县开展了籽粒脱水动态观测。其中, 在开鲁试验点5月10日播种, 7月19日进入吐丝期, 7月31日开始取样测定, 10月10日收获; 在铁岭试验点4月28日播种, 7月10日进入吐丝期, 7月26日开始取样测定, 10月20日收获。吐丝前, 选择生长一致、无病虫为害的植株雌穗套袋, 吐丝后0~3 d内统一授粉。观测间隔5~7 d取样1次, 接近生理成熟期时2~3 d测定1次。取样时选择5个套袋植株的果穗, 放入自封袋立即带回实验室。手工脱粒, 取果穗中部籽粒, 称鲜重后放入烘箱, 105°C杀青30 min, 85°C下烘至恒重称量。含水率(%) = (鲜重-干重)/鲜重×100%。Table 1
表1
表1试验品种名称
Table 1
地点Location | 品种 Hybrid |
---|---|
开鲁 | 金珠58, 德育919, 金庆202, 华美1号, 迪卡159, 东单913, ND865, 东单507, 泽玉501, S1651, 宏硕588, 泽玉8911, 东单1331, 金庆Q9, 德丰88, 优迪919, 世宾388, 翔玉998 |
Kailu | Jinzhu 58, Deyu 919, Jinqing 202, Huamei 1, Dika 159, Dongdan 913, ND865, Dongdan 507, Zeyu 501, S1651, Hongshuo 288, Zeyu 8911, Dongdan 1331, Jinqing Q9, Dengfeng 88, Youdi 919, Shibin 388, Xiangyu 998 |
铁岭 | 丰垦139, 丹玉311, 铁研388, 宏育236, 吉单66, 泽玉501, 泽玉8911, 陕单636, 东单913, 东单1311, 华美1号, 中迪702, 中迪710, 翔玉998, 优迪919, 迪卡159, 辽单575, 京农科728, 京科968 |
Tieling | Fengken 139, Danyu 311, Tieyan 388, Hongyu 236, Jidan 66, Zeyu 501, Zeyu 8911, Shaandan 636, Dongdan 913, Dongdan 1331, Huamei 1, Zhongdi 702, Zhongdi 710, Xiangyu 998, Youdi 919, Dika 159, Liaodan 575, Jingnongke 728, Jingke 968 |
新窗口打开|下载CSV
1.3 籽粒含水率模型构建
采用Logistic Power非线性增长模型[21]模拟不同品种籽粒含水率变化过程, 吐丝后活动积温(≥0°C积温)为自变量, 籽粒含水率为因变量, 建立籽粒含水率模型。$\text{MC}=\frac{a}{1+{{\left( \frac{T}{b} \right)}^{c}}}$
式中, MC为籽粒含水率(%); T (°C d)为授粉后积温, 以临近的国家气象地面观测台站数据计算; a、b、c为模型参数。a为模型极值, 即籽粒初始含水率, 本研究将a参数设定为90。使用CurveExpert Professional 2.2软件的非线性曲线拟合功能, 得出不同品种回归模型b、c参数的最优估计值, 拟合结果经模型显著性检验并计算决定系数R2。以区域内多年多点机械粒收品种鉴选试验的实收籽粒含水率与模型模拟含水率的均方根误差RMSE检验模型准确性。
1.4 适宜机械粒收日期的时空分析
以该区域春玉米常年播种日期为起点, 通过试验确定不同品种生长发育及籽粒脱水的活动积温需求, 结合历史气象数据估算累积相应活动积温所需的天数, 累加得到不同品种在辽河流域籽粒含水率降至适宜机械粒收标准的日期。各地玉米播种日期由玉米产业技术体系生产调研数据统计分析得出, 包含区域内20个主产县市区。历史气象资料包括区域内20个气象站点(去除高海拔气象站点) 1961— 2015年数据[26]。利用ArcGIS地统计分析软件进行多源数据融合、空间插值及作图[27,28]。1.5 数据处理
采用Microsoft Excel 2010处理机械粒收品种鉴选及籽粒脱水动态观测试验数据和作图, 使用SPSS17.0进行方差和模型显著性分析。2 结果与分析
2.1 不同玉米品种籽粒脱水与积温的关系
2017年在铁岭、开鲁的籽粒脱水动态观测试验, 选用不同熟期类型的玉米品种29个, 其中泽玉501、优迪919、东单1331、迪卡159、翔玉998、东单913、华美1号和泽玉8911为两地共用品种。以该品种雌穗统一授粉时间为起点, 计算籽粒含水率测定时的累积活动积温, 将活动积温与籽粒含水率进行模型拟合[21], 得到上述29个玉米品种的籽粒含水率模型特征参数。全部品种模型显著性检验均达到极显著水平(P<0.01), 决定系数R2均高于0.95 (表2)。共有品种在两地的脱水动态趋势一致, 可用相同的特征参数模拟两地的含水率变化动态。Table 2
表2
表2不同玉米品种的含水率-积温方程参数值
Table 2
品种 Hybrid | 地点 Location | b | c | R2 | 样本量 n |
---|---|---|---|---|---|
ND865 | 开鲁 Kailu | 1265.97 | 2.25 | 0.98 | 19 |
泽玉501 Zeyu 501 | 开鲁, 铁岭 Kailu, Tieling | 1093.89 | 1.66 | 0.98 | 18 |
世宾388 Shibin 388 | 开鲁 Kailu | 1087.05 | 1.82 | 0.99 | 19 |
德丰88 Defeng 88 | 开鲁 Kailu | 1085.03 | 2.27 | 0.99 | 19 |
优迪919 Youdi 919 | 开鲁, 铁岭 Kailu, Tieling | 1081.16 | 1.75 | 0.98 | 18 |
中迪710 Zhongdi 710 | 铁岭 Tieling | 1079.05 | 1.87 | 0.99 | 20 |
东单1331 Dongdan 1331 | 开鲁, 铁岭 Kailu, Tieling | 1060.52 | 1.89 | 0.97 | 18 |
S1651 | 开鲁 Kailu | 1053.26 | 1.73 | 0.99 | 19 |
迪卡159 Dika 159 | 开鲁, 铁岭 Kailu, Tieling | 1051.99 | 1.49 | 0.98 | 18 |
金庆Q9 Jinqing Q9 | 开鲁 Kailu | 1049.91 | 2.11 | 0.99 | 19 |
中迪702 Zhongdi 702 | 铁岭 Tieling | 1040.69 | 2.17 | 0.98 | 20 |
丹玉311 Danyu 311 | 铁岭 Tieling | 1039.33 | 1.65 | 0.97 | 20 |
宏硕588 Hongshuo 588 | 开鲁 Kailu | 1025.91 | 1.85 | 0.99 | 19 |
翔玉998 Xiangyu 998 | 开鲁, 铁岭 Kailu, Tieling | 1023.58 | 1.79 | 0.98 | 20 |
陕单636 Shaandan 636 | 铁岭 Tieling | 1018.71 | 1.90 | 0.98 | 20 |
铁研388 Tieyan 388 | 铁岭 Tieling | 1005.38 | 1.69 | 0.97 | 20 |
吉单66* Jidan 66* | 铁岭 Tieling | 1002.67 | 1.92 | 0.98 | 20 |
宏玉236 Hongyu 236 | 铁岭 Tieling | 1002.67 | 1.92 | 0.98 | 20 |
丰垦139 Fengken 139 | 铁岭 Tieling | 992.81 | 2.05 | 0.98 | 20 |
德育919* Deyu 919* | 开鲁 Kailu Kailu, Tieling | 986.47 | 2.22 | 0.99 | 19 |
京农科728* Jingnongke 728* | 铁岭 Tieling | 979.46 | 1.95 | 0.99 | 20 |
东单507 Dongdan 507 | 开鲁 Kailu | 975.56 | 1.38 | 0.98 | 19 |
东单913 Dongdan 913 | 开鲁, 铁岭 Kailu, Tieling | 973.42 | 1.46 | 0.98 | 18 |
辽单575 Liaodan 575 | 铁岭 Tieling | 966.18 | 1.59 | 0.99 | 20 |
华美1号 Huamei 1 | 开鲁, 铁岭 Kailu, Tieling | 957.27 | 1.78 | 0.96 | 18 |
泽玉8911* Zeyu 8911* | 开鲁, 铁岭 Kailu, Tieling | 946.40 | 1.87 | 0.99 | 18 |
金庆202 Jinqing 202 | 开鲁 Kailu | 943.39 | 1.57 | 0.96 | 19 |
京科968 Jingke 968 | 铁岭 Tieling | 859.91 | 1.30 | 0.97 | 20 |
金珠58 Jinzhu 58 | 开鲁 Kailu | 845.78 | 1.69 | 0.96 | 19 |
新窗口打开|下载CSV
分析2013—2017年在辽河流域2个省区、7个试验点开展的机械粒收品种鉴选试验, 筛选其中已建立籽粒含水率预测模型的品种数据, 共有45点/次的机械粒收实收含水率测定结果。根据鉴选试验的吐丝期和收获期, 计算其间的活动积温。利用各品种的籽粒含水率模型, 模拟收获时的籽粒含水率。不同品种籽粒实收含水率与模拟含水率间存在极显著的线性关系(图2), 线性方程决定系数R2为0.916 (n = 45), 均方根误差RMSE为1.217。表明不同品种籽粒含水率模型具有较高的模拟精度。
由于积温资源不同, 2个省区试点实收籽粒含水率也存在极显著差异(P<0.01)。内蒙古试验点的籽粒含水率介于22.6%~30.2%之间, 高于辽宁试点17.6%~28.2%的含水率范围。模型的模拟结果较好地反映出气候资源不同造成的籽粒含水率差异(图2), 表明含水率模拟模型适于在该区域应用。
图2
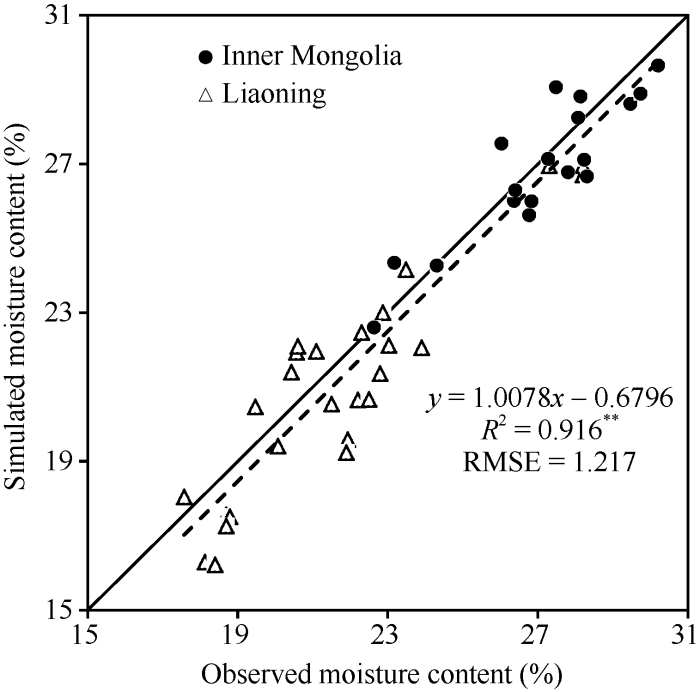
图22013-2017年辽河流域不同品种机械粒收实测籽粒含水率与模拟含水率的关系
Fig. 2Relationships between observed and simulated moisture contents of different cultivars in mechanical grain harvest, which collected at seven locations in Liao River Basin from years 2013 to 2017
2.2 不同区域玉米品种积温需求差异
2017年铁岭、开鲁两地共用的8个品种播种到吐丝、吐丝到生理成熟和播种到生理成熟所需活动积温及方差分析如表3所示。同一年份不同品种之间在从播种至吐丝、吐丝到生理成熟和播种到生理成熟等各阶段所需积温差异显著(P<0.05); 而同一品种各阶段所需积温在区域间差异不显著(P>0.05), 说明不同区域同一品种从播种到吐丝、吐丝到生理成熟及播种到生理成熟所需积温相对稳定, 可以利用铁岭、开鲁两地各品种测定的生育期积温需求在区域内估算玉米生育进程。Table 3
表3
表3不同玉米品种不同区域各生育时期积温需求
Table 3
地点 Location | 品种 Hybrid | 播种-吐丝所需积温 Accumulated temperature from sowing to silking | 吐丝-生理成熟所需积温 Accumulated temperature from silking to physiological maturity | 播种-生理成熟所需积温 Accumulated temperature from sowing to physiological maturity |
---|---|---|---|---|
内蒙古通辽市开鲁县 Kailu county, Tongliao city, Inner Mongolia autonomous region | 华美1号 Huamei 1 | 1623.7 | 1334.5 | 2938.2 |
迪卡159 Dika 159 | 1646.7 | 1552.9 | 3156.6 | |
东单913 Dongdan 913 | 1624.6 | 1350.5 | 2954.2 | |
泽玉501 Zeyu 501 | 1646.7 | 1520.0 | 3123.7 | |
泽玉8911 Zeyu 8911 | 1646.7 | 1484.4 | 3088.1 | |
东单1331 Dongdan 1331 | 1720.6 | 1617.7 | 3378.4 | |
优迪919 Youdi 919 | 1695.8 | 1611.8 | 3378.4 | |
翔玉998 Xiangyu 998 | 1695.8 | 1611.8 | 3378.4 | |
辽宁省铁岭市铁岭县 Tieling county, Tieling city, Liaoning province | 华美1号 Huamei 1 | 1616.9 | 1331.1 | 3078.2 |
迪卡159 Dika 159 | 1644.9 | 1550.2 | 3306.4 | |
东单913 Dongdan 913 | 1644.9 | 1331.1 | 3087.2 | |
泽玉501 Zeyu 501 | 1673.5 | 1550.2 | 3306.4 | |
泽玉8911 Zeyu 8911 | 1644.9 | 1495.3 | 3251.4 | |
东单1331 Dongdan 1331 | 1700.9 | 1683.3 | 3439.5 | |
优迪919 Youdi 919 | 1700.9 | 1683.3 | 3439.5 | |
翔玉998 Xiangyu 998 | 1700.9 | 1683.3 | 3439.5 | |
区域间 Interregional | ns | ns | ns | |
不同品种 Different hybrid | * | * | * |
新窗口打开|下载CSV
基于上述结果, 利用试验获取的播种、出苗、吐丝、生理成熟等关键生育期记载数据, 以及各品种的籽粒含水率预测模型, 分别计算各品种不同生育阶段的积温需求。选取2017年首次国审的宜机收品种德育919、泽玉8911、吉单66和京农科728, 与当地主栽的辽单575 (辽宁)、京科968 (内蒙古通辽和赤峰)对比分析。根据试验记载的生育期数据, 计算各品种自播种至吐丝期的积温需求, 再利用籽粒含水率预测模型, 估算自吐丝至达到机械粒收适宜含水率(25%)所需要的活动积温, 两者相加得到播种至适宜机械粒收时期所需活动积温[9,10](表4)。结果显示, 播种至籽粒含水率降至25%活动积温, 德育919为3122°C d, 京农科728为3178°C d, 属低于3200°C d积温的品种; 泽玉8911为3331°C d, 吉单66为3356°C d, 属低于3400°C d积温的品种; 当地主推品种辽单575为3558°C d, 京科968为3697°C d, 积温需求均大于国审宜机收品种200°C d以上。
Table 4
表4
表4不同玉米品种在不同阶段对活动积温的需求
Table 4
品种 Hybrid | 播种-出苗 Sowing-Emergence | 出苗-吐丝 Emergence-Silking | 吐丝-生理成熟 Silking-Physiological maturity | 吐丝-含水率25% Silking-25% MC | 播种-含水率25%* Sowing-25% MC* |
---|---|---|---|---|---|
德育919 Deyu 919 | 237 | 1366 | 1351 | 1519 | 3122 |
京科728 Jingke 728 | 470 | 1126 | 1497 | 1582 | 3178 |
泽玉8911 Zeyu 8911 | 265 | 1411 | 1578 | 1655 | 3331 |
吉单66 Jidan 66 | 310 | 1395 | 1662 | 1651 | 3356 |
辽单575 Liaodan 575 | 379 | 1419 | 1655 | 1760 | 3558 |
京科968 Jingke 968 | 379 | 1529 | 1622 | 1789 | 3697 |
新窗口打开|下载CSV
2.3 不同玉米品种适宜收获期的时空分布
基于区域内常年播种日期分析、不同品种降至适宜粒收含水率积温以及区域内近54年气候数据资料, 估算降至适宜机械粒收籽粒含水率25%日期的空间分布, 表明4个国审宜机收品种中, 德育919和京农科728, 籽粒脱水速率较快, 在9月中下旬即可在辽河流域大部降至25%含水率, 包括内蒙古辽河流域中部部分地区、辽宁南部地区、吉林部分地区, 仅辽河流域西部地区需延后至10月中旬达到。泽玉8911和吉单66脱水速率较上述两品种慢, 辽河流域大部地区需在10月上中旬降至25%含水率, 而流域西部地区无法在11月前脱水至适宜含水率水平(图3)。图3
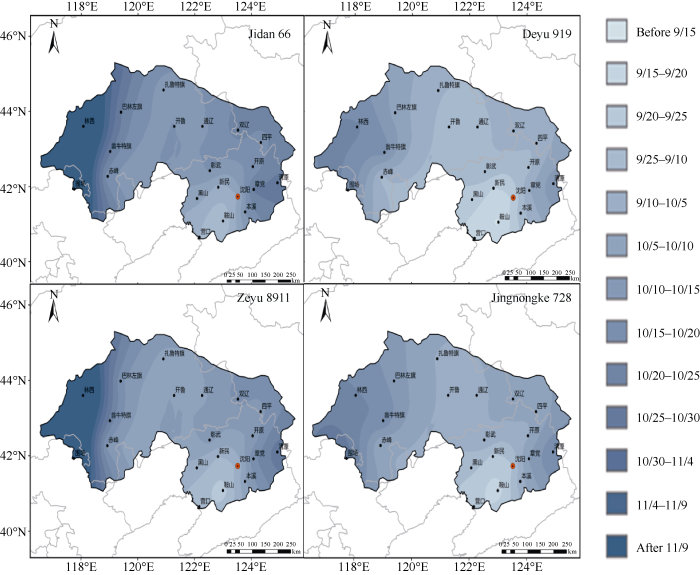
图3辽河流域春玉米国审宜机收品种籽粒降水至25%的时间分布图
Fig. 3Time map when grain moisture content reduces to 25% of four national trial maize cultivars suitable for mechanical grain harvesting in Liao River Basin
辽单575和京科968分别是辽河流域辽宁及内蒙通辽、赤峰地区种植面积较大的主栽品种。基于两地生育期及籽粒脱水动态观测结果(图4), 这两品种虽然在当地普遍种植, 但其生育期长、籽粒脱水较慢, 除辽宁中部的营口、鞍山至沈阳一线地区能够在10月中下旬达到适宜机械粒收的含水率要求外, 其他大部地区难以在11月前常规收获期实现高质量的机械粒收。
图4
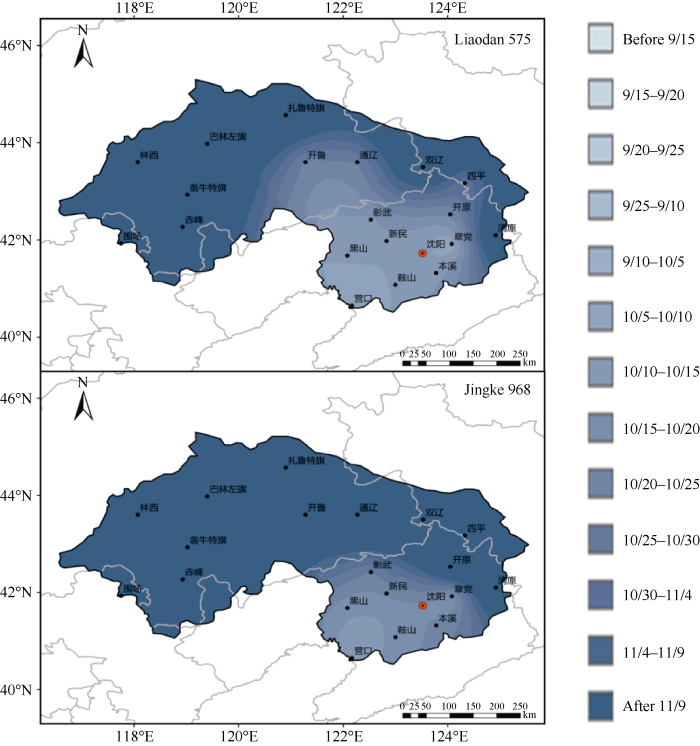
图4辽河流域主栽玉米品种籽粒降水至25%的时间分布图
Fig. 4Time map when grain moisture content reduces to 25% of two main maize cultivars in Liao River Basin
就区域总体而言, 与籽粒脱水较快的德育919相比, 京农科728籽粒含水率降至25%的时间约晚3~6 d, 平均4 d; 泽玉8911约晚8~15 d, 平均晚13 d; 而吉单66约晚12~22 d, 平均晚19 d。而当地主栽品种辽单575和京科968, 较国审粒收品种的平均宜机收时间晚约17~36 d, 平均约30 d。
3 讨论
籽粒含水率高低与机械粒收的籽粒破碎率、杂质率和损失率密切相关[6,7], 是影响机械粒收质量、制约机械粒收技术推广的重要因素。前人研究表明, 籽粒含水率在17%~25%之间进行机械粒收作业, 破碎率、杂质率和损失率等机收质量指标较高, 能够达到国标要求[8,10]。当籽粒含水率降至25%左右时收获, 可协调适期早收、提高收获质量、降低烘干成本之间的矛盾, 是判定玉米机械粒收时间的关键标准, 也是本研究采用的适宜收获期的判定指标。在辽河流域主要玉米产区内蒙古及辽宁省的机械粒收品种鉴选和技术示范研究显示[3,5], 籽粒破碎和产量损失是区域内机械粒收所面临的主要质量问题, 与收获时籽粒含水率偏高密切相关。其主要问题, 一是当地主栽玉米品种的生育期偏长、脱水速率较慢, 二是区域内机械粒收时期偏早。因此, 本研究围绕区域内主要种植品种的脱水动态, 分析其达到适宜机械粒收含水率要求的时间, 以明确区域适宜品种的筛选条件及其合理的收获时期。本研究基于开鲁、铁岭两地不同品种籽粒脱水动态观测试验, 和Logistic Power模型, 分析并建立了各品种籽粒含水率模型, 可以很好地模拟春玉米的籽粒含水率变化过程, 效果较好。表明用Logistic Power模型模拟籽粒含水率变化过程具有极佳的区域生态条件和栽培模式的适应性。以此为基础, 明确了区域内主栽品种的脱水特性及降至机械粒收适宜含水率的积温需求。本研究与黄淮海夏玉米区研究结果相比较[21,23], 辽河流域主栽品种自播种至25%含水率的积温需求在3100~3700°C d, 明显高于黄淮海区品种。特别是在两种熟制模式下共有品种京农科728和辽单575的积温需求差异明显。其中, 京农科728在辽河流域春播一熟制模式下, 自授粉至生理成熟的积温需求为1497°C d, 至25%含水率的积温需求为1582°C d; 在黄淮海区夏播两熟制模式下, 至生理成熟的积温需求为1200°C d, 至25%含水率的积温需求为1354°C d, 两地相差297°C d和228°C d。而辽河流域主栽品种辽单575两个阶段积温需求分别为1655°C d和1760°C d, 在黄淮海区则表现为1389°C d和1528°C d, 两地相差266°C d和232°C d。表明虽然两种生态环境和熟制模式下籽粒脱水的动态趋势相似, 但脱水进程有差异, 后续可开展相关研究, 深入分析其中的生理机制和限制因子, 为选育和配置宜机收品种、收获期决策、栽培技术改进和扶持政策制定提供理论依据, 有效推动玉米机械粒收技术在我国各产区应用推广。
辽河流域处于东北玉米产区南部, 玉米生产以春播一熟制为主, 热量资源相对优越[25]。区域内以辽宁中部营口、鞍山、沈阳地区积温资源最为丰富, 其他地区渐次减少。传统生产方式下, 玉米播期集中在4月下旬至5月中旬之间, 常年收获期在10月上、中旬, 期间活动积温在3100~3600°C d之间, 能够满足区域内主要品种正常的生理成熟和大部分品种脱水至25%含水率, 说明辽河流域热量资源不是收获期籽粒含水率高的最关键限制因素。但从品种的脱水特性分析, 当前主栽的辽单575和京科968虽然熟期与区域热量资源匹配较好, 但生理成熟期籽粒含水率较高, 且脱水速率较慢, 难以在当地常规收获期内降至25%的籽粒含水率。2017年首批国审宜机收品种中, 德育919、京农科728脱水较快, 在大部地区可于9月中下旬达到25%含水率要求; 泽玉8911、吉单66脱水则相对较慢, 可于10月上中旬达到25%含水率要求。可见, 更新当地的主栽品种, 选择生理成熟期与当地积温资源相匹配, 且生理成熟时籽粒含水率较低、后期籽粒脱水快的品种, 可以在辽河流域实现高质量的机械籽粒收获。同时, 根据各地的积温资源情况, 合理确定机械粒收时期, 为籽粒充分成熟和熟后脱水留足时间, 可有效降低收后烘干和储运的成本。
玉米籽粒脱水过程, 不仅受温度条件驱动, 还受大气相对湿度、降水、风速等多种气象因素影响[29,30]。特别是生理成熟后的物理散失过程中[31], 籽粒脱水速率与大气的饱和气压差、干湿球温度差和相对湿度密切相关[32]。针对辽河流域温度资源相对丰富的区域特点, 以驱动籽粒脱水的主控因素入手开展分析, 暂未考虑相对湿度、降水、风速等其他气象因素对籽粒脱水的影响, 后续研究还需要结合更为精细的设计试验加以解决。
4 结论
研究分析了辽河流域29个玉米品种的籽粒脱水特性并分别建立了含水率预测模型。以多年多点籽粒含水率测定数据对预测模型的精度进行了验证, 并分析了6个典型品种常年达到适宜机械粒收日期的空间分布规律。国审宜机收品种可以在9月下旬至10月上旬达到适宜机械粒收的籽粒含水率条件, 较当地主栽品种早20~30 d。根据辽河流域积温条件, 合理更新品种、确定机械粒收时期, 可有效提高机械粒收质量, 降低收后烘干和储运的成本, 有助于提高当地玉米生产效益。致谢: 感谢国家玉米产业技术体系生产调研提供的辽河流域玉米播种日期的数据。
参考文献 原文顺序
文献年度倒序
文中引用次数倒序
被引期刊影响因子
[本文引用: 1]
[本文引用: 1]
[本文引用: 1]
[本文引用: 1]
[本文引用: 2]
[本文引用: 2]
[本文引用: 1]
[本文引用: 1]
[本文引用: 2]
[本文引用: 2]
[本文引用: 2]
[本文引用: 2]
[本文引用: 2]
[本文引用: 2]
[本文引用: 2]
[本文引用: 2]
[本文引用: 2]
[本文引用: 2]
[本文引用: 3]
[本文引用: 3]
[本文引用: 1]
[本文引用: 1]
[本文引用: 1]
[本文引用: 1]
[本文引用: 1]
[本文引用: 1]
[本文引用: 1]
[本文引用: 1]
DOI:10.2134/agronj1959.00021962005100060003xURL [本文引用: 1]

Synopsis: Husk and shank characteristics and shape or size of ear were not found to be major factors associated with differing rates of drying among strains of corn.
DOI:10.4141/cjps94-097URL [本文引用: 1]

The relationship between grain moisture at harvest and the amount of kernel cracking was evaluated at Brandon, Manitoba. Grain moisture at harvest was closely correlated to percentage kernel cracking. Least amount of kernel cracking occurred with grain moisture ranging from 16.7% for K730 to 22.1% for 3979.
DOI:10.1016/j.fcr.2005.03.001URL [本文引用: 1]

Development of maize ( Zea mays L.) kernels follows a predictable pattern involving rapid increase in dry weight and large changes in water content (WC). We showed previously that final kernel weight (KW) was closely correlated with maximum WC achieved during rapid grain filling. The objectives of the current work were (i) to test if percent moisture content (MC, measured on a fresh weight basis) could be used to normalize genetic and environmental variations in kernel development shown to affect final KW and (ii) to determine whether final KW could be predicted from kernel WC prior to rapid grain filling. The data examined included results from five hybrids varying more than 2-fold in final KW grown in the field, and from previously published results. When KW and WC were expressed relative to their maximum values obtained during kernel development, a single model described the relationship between dry weight accumulation and MC for the larger seeded hybrids (199–352 mg kernel 611) and published results (222–359 mg kernel 611). Two smaller seeded yellow-flint popcorn hybrids, however, accumulated less dry matter per unit moisture than expected. Nonetheless, all genotypes exhibited a common developmental relationship between kernel WC (expressed as a percent of the maximum value) and MC under well-watered conditions. A new model was developed to couple this developmental relationship to final KW. This model accurately predicted final KW from kernel WC values measured prior to rapid grain filling (6580% MC; root mean square error, RMSE, of 28.9 mg kernel 611) for all hybrids examined and all published results for which KW and kernel WC data were available. The model also provided a simple means to determine whether final KW was limited by photosynthate supply during kernel development.
DOI:10.1016/j.fcr.2006.09.001URL [本文引用: 1]

Kernel water relations play a key role in controlling the duration of grain filling. This duration is controlled by the relationship between kernel water and biomass development, as it determines the timing kernels reach a critical percent moisture content (MC, measured on a fresh weight basis) at which biomass accumulation stops. The time in which this critical percent MC is attained can be affected by the timing kernel net water uptake stops (i.e. maximum water content is reached), or by the relationship between water loss and biomass deposition after maximum water content is attained. Which of the two mechanisms could be behind genotypic differences in maize ( Zea mays L.) grain-filling duration was unknown. We also studied the relationship between kernel water and volume development, as it was unknown in this species. Thirteen commercial hybrids were evaluated under different growing environments, and weight, water content and volume of their kernels were measured throughout grain filling. There were no differences among hybrids in their kernel percent MC at physiological maturity ( p > 0.05), showing that hybrid differences in grain-filling duration (from 1117 to 1470 C day) were related to variations in the accumulated thermal time from flowering to this critical percent MC. There were no differences in the accumulated thermal time from silking to kernel maximum water content, and this stage was always reached at the same kernel percent MC (ca. 540 g kg 1). Differences in grain-filling duration were explained by the pattern of percent MC decline after maximum water content was reached. This percent MC decline was dependent upon the relationship between water loss and biomass deposition; the higher the water loss rate and the higher the kernel growth rate the shorter the duration ( r 2 = 0.60; p < 0.001). Maximum kernel volume was achieved after maximum water content, and close to physiological maturity. Hybrids differed ( p < 0.05) in the kernel volume generated after maximum water content, and this was also related to the relationship between biomass and water development late in grain filling. Results showed the importance of understanding and predicting percent MC development throughout grain filling, as there were no differences between hybrids and environments in their kernel percent MC at specific developmental stages (i.e. maximum water content or physiological maturity). Our results highlighted the importance of the relationship between water loss and biomass deposition during late kernel development in the duration of maize grain filling.
[本文引用: 1]
[本文引用: 1]
DOI:10.1016/j.eja.2014.05.011URL [本文引用: 1]

Moisture content influences harvest timing and the consequent drying process and drying costs, and the development of spoilage fungi during pre- and post-harvest phases. Maize kernel development in the field can be partitioned into three phases: i) lag phase, ii) grain filling and maturation drying, and iii) post-maturity dry-down. A model simulating maize kernel moisture content during maturation can help either monitoring or foreseen maize kernel humidity during the harvest period. Also, it would be useful in simulation studies via crop models to estimate the infield feasibility of harvest but also the interaction with diseases responsible for mycotoxin production, against weather scenarios. A process-based model was developed, called MIMYCS.Moisture. When the hybrids were analyzed all together, MIMYCS.Moisture showed a good general predictive capability with an average error in moisture estimation of +/- 3.28% moisture (considering the root mean square error - RMSE). The model efficiency (EF) was positive (0.85) and the model was able to explain the 89.7% of variation. When the two sub-models were analyzed separately, the RMSE remained approximately at the same level of the general model, while the other indicators changed revealing the different characteristics of the two models. The developmental moisture sub-model has a slight tendency to overestimate, while the dry-down sub-model tended to underestimate final moisture content. However, when the model was analyzed separately for each hybrid, both calibration and validation results suggested that more data are needed to improve the model likely with respect to kernel characteristics of hybrids. Finally, the equilibrium moisture content equation used, taken from industrial drying models, might not be adequate for simulating the field conditions where temperature is well below the one in dryers and environmental air humidity may vary considerably across sites and harvest periods. (C) 2014 Elsevier B.V. All rights reserved.
[本文引用: 4]
[本文引用: 4]
[本文引用: 1]
[本文引用: 1]
[本文引用: 2]
[本文引用: 2]
[本文引用: 1]
[本文引用: 1]
[本文引用: 2]
[本文引用: 2]
.
URL [本文引用: 1]
[本文引用: 1]
[本文引用: 1]
[本文引用: 1]
[本文引用: 1]
URL [本文引用: 1]
DOI:10.2135/cropsci1966.0011183X000600030003xURL [本文引用: 1]

Moisture data for 12 years from the period 1940 to 1963 were summarized to determine the relation between kernel moisture and time. The rate of kernel moisture reduction was determined for five arbitrary moisture phases and used to predict when specified moisture levels would be attained from pollination date. Correlation studies were made between rate of kernel moisture reduction and four weather factors (air temperature, saturation deficit, wet bulb depression, and relative humidity).
[本文引用: 1]
[本文引用: 1]
[本文引用: 1]
[本文引用: 1]