

*通讯作者(Corresponding authors): 张小红, E-mail:zhxh2493@126.com; 闵东红, E-mail:mdh2493@126.com 收稿日期:2014-06-16 基金:
摘要
关键词:谷子; F-box蛋白; 亚细胞定位; 干旱响应; 诱导机制
Identification, Classification, and Drought Response of F-box Gene Family in Foxtail Millet
HUO Dong-Ying


Fund:
Abstract
Keyword:Foxtail millet; F-box protein; Subcellular localization; Drought response; Induction mechanism
Show Figures
Show Figures
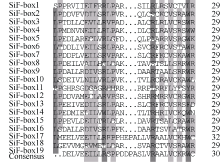
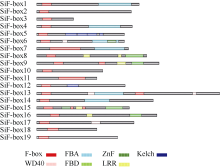
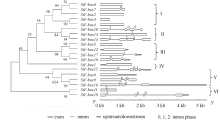
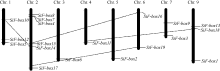
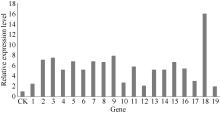
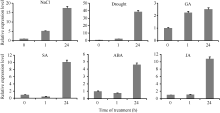
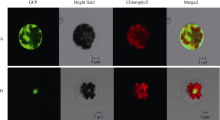
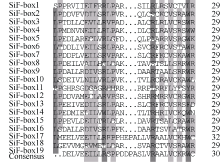
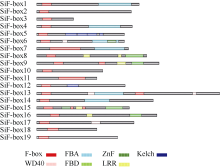
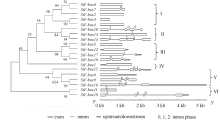
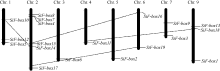
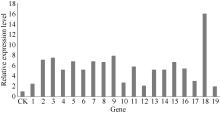
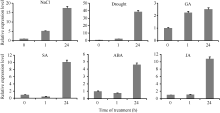
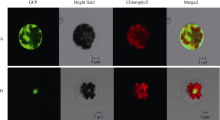
在适应干旱等非生物逆境胁迫中, 植物不断进化自身防御系统以减少水分损失或增加水分吸收等[ 1]。蛋白质的降解在植物逆境信号的响应、细胞信号转导等过程中发挥重要作用, 对于维持植物正常生长发育具有重要意义[ 2]。大多数蛋白通过泛素/26S蛋白酶体途径降解。泛素介导的蛋白质降解过程主要有3种酶参与, 包括泛素活化酶(E1), 泛素结合酶(E2)及泛素连接酶(E3)。在ATP供能的情况下, E1的半胱氨酸残基与泛素C末端的甘氨酸形成高能硫酯键, 使泛素激活, 被转移到E2的丝氨酸残基上, 随后E2和E3共同识别底物蛋白, 对其进行泛素化修饰。E3在泛素化-酶体途径的底物特异识别过程中起着关键作用。目前已经发现不同类型的E3[ 3], 主要的一种是Skp1-Cullin1-F-box (SCF)复合体, 由4个亚基组成, 分别为Cullin、SKP1、RBX1和F-box蛋白[ 4, 5]。每个F-box蛋白家族成员的N端都至少含1个大约40~50个保守氨基酸的F-box基序, 并与Skp1结合; C端是结构不同的蛋白-蛋白相互作用结构域, 包括亮氨酸拉链(LRR)、Kelch和WD40等。
F-box蛋白最早在cyclin F的N端被发现而命名[ 6], 广泛存在于真核生物中, 参与多种植物激素信号的传导、逆境胁迫应答等, 在调节光形态建成、光周期节律、花器官发育及自交不亲和中发挥着重要功能。植物中发现的第一个F-box蛋白是Unusual Floral Organs (UFO), 参与了植物花分生组织与花器官的建成[ 7, 8, 9, 10]。LKP1/ZTL、LKP2/FKL和FKF1可以调控光形态建成、调节生物钟的昼夜周期节律及开花时间[ 11, 12, 13, 14]。F-box蛋白还与植物的自交不亲和性相关, 许多植物的自交不亲和性由S位点控制, 研究表明, 与S位点相关的S-locus-F-box genes (SLF)能在花粉中特异表达[ 15, 16, 17, 18]。
许多F-box蛋白还参与激素的信号传导, TIR1是生长素受体蛋白, 与AUX/IAA发生相互作用, 导致AUX/IAA降解[ 19, 20, 21, 22]; EBF1过量表达引起植物对乙烯不敏感, ebf1和 ebf2突变株则表现出组成型乙烯反应, EBF1/EBF2两者之一发生突变都导致植株对乙烯反应增强, 而双突变体则表现出三重反应[ 23, 24]。赤霉素(GA)的信号通路也可以通过F-box蛋白(SLY1)调节[ 25, 26]。COI1编码F-box蛋白, 在茉莉酸(JA)信号通路中起关键作用[ 27, 28, 29]。
F-box蛋白参与非生物胁迫应答的调控, 如DOR1 (drought tolerance repressor 1)是S位点类F-box蛋白家族的基因, 在保卫细胞中表达, dor使ABA表达量上调, 气孔关闭, 从而增加抗旱性[ 30]。MAX2 (more axillary growth 2)对干旱响应明显, 与野生型相比, max2的保卫细胞对ABA敏感程度降低, 角质层变薄, 在MAX2突变的情况下, 与ABA合成、运输及代谢有关的基因表达量都降低[ 31]。
随着全球水资源短缺和气候变暖形势的日益严峻, 对作物的抗旱节水性进行遗传改良, 提高作物水分利用率, 已成为目前研究的一个热点。谷子具有耐旱、耐贫瘠等特点, 是一种抗逆性很强的作物。然而, 目前, 植物中关于F-box蛋白的研究主要集中在拟南芥、水稻、玉米中。植物已知功能的F-box蛋白只有30个, 其中22个来源于拟南芥(见附表), 谷子中尚未见报道。本研究对谷子进行了转录组测序分析, 筛选出19个在干旱胁迫下表达量上调的F-box基因, 对其基因结构、系统发育关系及染色体定位等进行分析, 并选择对干旱应答最强的基因 SiF-box18作了具体分析, 为进一步研究F-box家族的功能及 SiF-box18基因的功能提供了依据。
1 材料与方法1.1 试验材料谷子Tie-8396种子由中国农业科学院作物科学研究所刁现民研究员课题组提供。谷子幼苗在温度22℃、相对湿度65%、光照周期16 h/8 h的条件下培养。生长3周的幼苗经干旱处理1周, 送华大基因进行转录组测序。
1.2 保守域预测谷子( Setaria italica)基因组数据和cDNA数据均下载于谷子最新数据库版本Phytozome (http://www.phytozome.net/search.php)。利用SMART (http://smart.embl-heidelberg.de/)在线工具分析F-box蛋白结构域, 利用DOG 2.0[ 32]绘制19个F-box家族蛋白的功能结构域示意图。
1.3 进化树与基因结构分析使用MEGA5对19个谷子F-box基因进行多序列比对分析, 将分析结果用邻接法生成系统进化树, Bootstrap值设置为1000。利用GSDS在线工具(http:// gsds.cbi.pku.edu.cn/)制作谷子F-box家族基因外显子- 内含子结构示意图。
1.4 染色体定位与启动子顺式作用元件分析通过MapDraw程序显示每个基因在染色体上的位置, 得到各个基因在染色体上分布状况。采用Yang等[ 33]的方法, 判别同源基因, 即2个基因联配部分序列的长度要覆盖2个基因中较长序列的80%和联配部分序列的相似性大于70%。利用DNAMAN分析谷子中F-box家族基因中的重复片段, 并将分析结果标注在染色体上。从谷子基因组数据库 Phytozome (http://www.phytozome.net/search.php)中选取谷子F-box基因起始密码子上游2000 bp序列作为启动子区, 利用植物顺式作用元件数据库PLACE26.0 (http://www.dna.affrc.go.jp/PLACE/)分析基因的顺式作用元件。
1.5 基因的扩增根据预测的谷子 SiF-box18基因的CDS序列, 用Primer Premier 5.0设计引物F: 5′-ATG GCG GAG CGG AAG-3′和R: 5′-TCA CCA TAC TGG GAA CAA AGG-3′。用TRIzol试剂盒(TIANGEN, 北京)提取叶片总RNA, 用Primer Script (TaKaRa)反转录试剂盒获得cDNA为模板扩增 SiF-box18, 并将其连接到pEASY-Blunt载体上。
1.6 RNA提取和Real-time PCR分析对生长3周的谷子幼苗分别进行干旱(6% PEG)、NaCl (100 mmol L-1)、茉莉酸(JA, 100 μmol L-1)、脱落酸(ABA, 100 μmol L-1)、赤霉素(GA, 100 μmol L-1)和水杨酸(SA, 100 μmol L-1)处理, 并于0、和24 h分别取样。利用TRIzol试剂盒(TIANGEN, 北京)提取总RNA, 分别利用6种胁迫下的谷子总RNA作为模板, 以SYBR Green染料法进行荧光定量Real-time PCR。用实时荧光定量PCR仪(ABI Prism 7300)进行PCR扩增。反应体系包括2× TaqPCR Master Mix (含荧光染料) 9 μL、10 μmol L-1引物各0.5 μL、ddH2O 9 μL和cDNA模板1 μL。反应条件为95℃ 5 min; 95℃ 15 s, 56℃ 20 s, 72℃ 30 s, 60℃ 30 s, 35个循环。PCR引物序列为F: 5′-GGA TGG AAA ACG GAG TCG-3′和R: 5′-TCA GGG TGA AAG TAG CGC-3′。以谷子 Actin基因( Si001873)为内标, 引物序列为Actin-F: 5′-GGC AAA CAG GGA GAA GAT GA-3′和Actin-R: 5′-GAG GTT GTC GGT AAG GTC ACG-3′。用2-∆∆Ct法计算各基因表达量。
1.7 亚细胞定位采用In-Fusion (TaKaRa)单酶切技术( BamH I)构建SiF-box18::hGFP融合表达蛋白(F: 5′-TAT CTC TAG AGG ATC CAT GGC GGA GCG GAA G -3′, R: 5′-TGC TCA CCA TGG ATC CCC ATA CTG GGA ACA AAG G-3′)。取4周左右的拟南芥叶片切成细条, 放入15 mL新鲜酶解液中, 避光, 23℃抽真空酶解3 h, 制备原生质体。取融合表达的重组质粒转化原生质体[ 34]。12~16 h后于激光共焦显微镜下观察。
2 结果与分析2.1 F-box家族成员识别及命名通过NCBI搜索, 谷子F-box基因家族有525个成员。通过谷子干旱转录组测序, 发现有19个F-box基因上调表达。用Pfam进行结构域分析发现这19个基因都具有完整的F-box结构域(图1), 并将这19个基因命名为 SiF-box1~ SiF-box19。这些F-box家族成员中蛋白最长有767个氨基酸, 最短的有155个氨基酸, 其等电点范围在4.90~11.48之间(表1)。
图1
Fig. 1
Figure OptionViewDownloadNew Window | |
![]() | 图1 谷子19个F-box家族蛋白保守域Fig. 1 Conserved domains of F-box in 19 proteins of foxtail millet |
表1
Table 1
表1(Table 1)
![]()
| 表1 谷子F-box家族基因的基本信息 Table 1 Basic information of F-box family genes in foxtail millet |
2.2 谷子F-box家族的基因结构及蛋白结构域分析通过Pfam结构域分析显示, 19个谷子F-box蛋白家族均含有F-box保守域(图1)。每个F-box蛋白的N端均有一段保守的F-box基序, 而C端包含不同的蛋白-蛋白相互作用结构域(图2)。根据图中显示, 19个F-box蛋白中, 26.3%的蛋白只含有F-box结构域, 其他蛋白含有多个功能域, 包括Fibrinogen- like domain (FBD)、WD40 repeats (WD40)、F-box associated (FBA)、Zinc finger domain (ZnF)、Kelch repeats (Kelch)和Leucine-rich repeat (LRR)。例如, SiF-box1除了含有F-box基序外, 还包括FBA结构域; SiF-box16含有F-box、LRR和ZnF 3个结构域。有些蛋白不只包含1个F-box基序, SiF-box9与SiF- box13分别包含2个F-box基序, 而SiF-box15中有3个F-box基序。
图2
Fig. 2
Figure OptionViewDownloadNew Window | |
![]() | 图2 谷子F-box家族蛋白的保守结构域Fig. 2 Conserved domain organization of F-box proteins |
2.3 进化树与基因结构分析对谷子19个F-box基因进行多重序列比对和系统进化分析后, 19个F-box基因划分为6类(图3)。内含子-外显子结构分析显示, 同一亚族的基因具有相似内含子-外显子结构。第I类包含 SiF-box6、SiF-box7、SiF-box2、SiF-box3和 SiF-box4, 这5个基因中, 没有或只包含1个内含子; 第II类中, SiF-box13和 SiF-box14含有3个内含子, 都有FBA结构; 第III类包含 SiF-box5、 SiF-box12、 SiF-box17和 SiF-box19, 其中, SiF-box5和 SiF-box12都含有kelch repeats; 第IV类只包含 SiF-box1一个基因, 没有内含子, 包含FBA结构; 第V类中, SiF-box8、 SiF-box9、 SiF-box15和 SiF-box16都包含F-box、LRR和ZnF结构域, 除 SiF-box8外, 其他3个基因都含有2个或2个以上内含子; 第VI类包含 SiF-box11与 SiF-box18, 只含有F-box基序, 没有其他结构域。
图3
Fig. 3
Figure OptionViewDownloadNew Window | |
![]() | 图3 谷子F-box基因家族进化树以及内含子外显子结构Fig. 3 Phylogenetic tree and intron-exon structures of F-box genes in foxtail millet |
2.4 染色体分布根据基因位置信息绘制了19个F-box家族基因在谷子染色体上的定位图(图4)。19个基因分布在8条谷子染色体上, 其中第8染色体上不含谷子F-box家族基因, 第2染色体上含F-box家族基因最多, 第1和第9染色体上都有3个F-box家族基因, 其他各染色体上含1~2个F-box家族基因。
图4
Fig. 4
Figure OptionViewDownloadNew Window | |
![]() | 图4 谷子F-box家族基因的染色体分布Fig. 4 Chromosome distribution of F-box genes in foxtail millet genome |
为了确定可能的进化机制, 研究了基因的复制现象, 依据系统发育树及序列相似性, 发现了4对旁系同源基因, 分别为 SiF-box10与 SiF-box14, SiF-box15与 SiF-box16, SiF-box17与 SiF-box19, 以及 SiF-box11与 Si-Fbox18基因 。
2.5 启动子顺式作用元件分析目前发现的与干旱胁迫相关的元件主要有ABRE (ABA-responsive element)、DRE (dehydration- responsive element)、LTRE (low temperature responsive element)、MYB和MYC元件。本研究选择起始密码子上游2000 bp序列作为启动子区, 研究19个F-box家族基因启动子区元件。结果显示, 谷子F-box家族基因启动子区域均含多个MYB和MYC元件, 其中, MYB数量最少为9个, 最多为78个, MYC的数量12~40个。MYB和MYC都属于转录因子, 与干旱、ABA的诱导表达相关。此外, 在这19个F-box家族基因中, 除 SiF-box12外, 在启动子区均含ABRE作用元件; 63.2%含DRE作用元件; 84.2%含LTRE作用元件(表2), 说明F-box家族基因与植物的逆境胁迫应答具有很强的关联。
表2
Table 2
表2(Table 2)
![]()
| 表2 谷子F-box家族基因启动子逆境相关顺式作用元件的数量分布 Table 2 Distribution of cis-acting elements in foxtail millet F-box gene promoters |
2.6 谷子F-box转录组结果分析19个上调表达的谷子 F-box基因中, 表达量差异明显(图5), SiF-box1、 SiF-box4、SiF-box6、SiF- box10、SiF-box12、SiF-box17和 SiF-box19的相对表达量介于1~5倍; SiF-box2、SiF-box3、SiF-box5、SiF- box7、SiF-box8、 SiF-box9、SiF-box11、SiF-box13、SiF- box14、SiF-box15、SiF-box16、SiF-box17、SiF-box19基因的表达量介于5~10倍之间; 高于10倍的只有 SiF-box18基因。与对照相比 SiF-box18基因的表达倍数是16倍, 因此选择 Si-Fbox18基因进一步分析。
图5
Fig. 5
Figure OptionViewDownloadNew Window | |
![]() | 图5 谷子F-box基因转录组测序结果分析Fig. 5 Transcriptome analysis of F-box genes in foxtail millet1-19: SiF-box1, …, SiF-box19. |
2.7 SiF-box18基因在各种逆境胁迫下的表达模式 为了进一步解析非生物胁迫和外源激素对 SiF-box18基因表达的影响, 对谷子幼苗分别进行干旱、NaCl、茉莉酸(JA)、脱落酸(ABA)、赤霉素(GA)和水杨酸(SA)胁迫处理, 通过荧光定量PCR检测不同处理条件下 SiF-box18的表达量。如图6所示, 在不同处理条件下, SiF-box18基因的表达量在24 h均达到最高。干旱胁迫下的表达倍数最高达到42倍, GA处理条件下表达量最低, 其他胁迫条件下的表达倍数, NaCl为18倍, ABA为5倍, JA与SA均为10倍。
图6
Fig. 6
Figure OptionViewDownloadNew Window | |
![]() | 图6 SiF-box18表达模式Fig. 6 Expression patterns of the SiF-box18 gene under various treatments |
2.8 亚细胞定位将SiF-box18构建到荧光表达蛋白GFP的N端。利用原生质体转化技术, 将SiF-box18-hGFP融合表达载体和空载体分别转入拟南芥原生质体, 在激光共聚焦显微镜下观察。绿色荧光蛋白在拟南芥原生质体中获得高效瞬时表达, 可以观察到对照GFP蛋白在细胞核、细胞膜、细胞质中均有绿色荧光信号; 而SiF-box18融合蛋白只在细胞核中有绿色荧光信号, 表明SiF-box18蛋白定位在细胞核中(图7)。
图7
Fig. 7
Figure OptionViewDownloadNew Window | |
![]() | 图7 SiF-box18蛋白的亚细胞定位A: 对照GFP定位在整个细胞; B: GFP-SiF-box18定位在细胞核中。Fig. 7 Subcellular localization of SiF-box18 proteinA: control GFP is distributed in the cell; B: GFP-SiF-box18 is localizated on the nuclear. |
3 讨论F-box蛋白是一个庞大的蛋白家族, 广泛存在于真核生物中。酵母、果蝇、人类、老鼠中分别有11、22、68和74个F-box蛋白成员; 而线虫中包含326个F-box蛋白家族成员[ 35]。植物中有大量的F-box蛋白存在, 拟南芥中大约有600~700个F-box基因[ 36, 37], 水稻中包含687个F-box基因[ 38]; 最近研究表明, 玉米中有359个F-box蛋白成员[ 39]。根据C端蛋白-蛋白相互作用结构域的不同, 可以把拟南芥F-box家族分为19个亚族[ 36, 37]; 水稻F-box家族分为10个亚族[ 38]; 玉米中分为13个亚族[ 39]。谷子F-box家族通过NCBI搜索, 发现有525个F-box基因, 进一步研究了其中19个受干旱诱导表达的F-box基因。
除N端保守的F-box基序外, 谷子F-box蛋白C端含有不同的蛋白-蛋白相互作用结构域, 例如, FBD、FBA、LRR、WD40、PPR和ZnF。本研究根据序列相似性, 将响应干旱胁迫的谷子19个F-box蛋白分为6类(图3)。第II类中, SiF-box13和SiF-box14包含FBA结构, FBA在泛素化中起重要作用, 可以识别靶蛋白; 第III类中SiF-box5和SiF-box12都含有Kelch repeats, Kelch是植物F-box蛋白特有的结构域[ 38]; 第IV只有SiF-box1一个蛋白, 包含FBA结构; 第V类中, 4个成员都包含F-box、LRR和FBD结构域, LRRs是Leu重复单元, 含有LRRs结构的蛋白一般与信号传导, DNA修复、重组、转录以及抗病等功能相关[ 40]; 第VI类含有F-box基序。此外, SiF-box6和SiF-box15蛋白还包含ZnF结构; 在谷子19个F-box家族中, 3个蛋白包含WD40结构, WD40结构存在于所有真核生物中, 在信号传导、细胞周期调控、细胞凋亡方面起着重要作用[ 41]。说明受干旱诱导表达的谷子F-box蛋白可能同样发挥着调控细胞周期、细胞信号传导等功能。
基因重复是基因组进化及基因家族扩大的重要因素。基因重复后会产生3种结果: (1)基因功能的缺失, 基因的2个拷贝中的一个拷贝由于突变失去功能; (2)产生新的功能, 基因的2个拷贝中的一个拷贝获得新的功能并通过自然选择被保留下来; (3)基因功能互补, 基因的2个拷贝都发生退化突变, 使得2个拷贝功能产生互补作用。本研究发现19个F-box基因有4对旁系同源基因, 这4对基因位于不同的染色体上, 可能是复制后功能产生了分化。
据报道, F-box基因不仅参与信号传导等生命活动[ 42], 还介导干旱等非生物胁迫响应。例如, 干旱条件下, dor转基因拟南芥植株表现出比野生型更强的抗性, 证明 DOR对干旱胁迫起负调控作用[ 30]; 在渗透胁迫和高盐胁迫下, DEL3 (EID1-like protein3)高效、快速表达[ 43]。本研究发现19个受干旱诱导表达的谷子F-box基因, 启动子区都包含多种非生物逆境相关元件, 而MYB和MYC的数量远远大于其他元件的数量, 说明F-box基因对干旱胁迫的响应主要通过MYB和MYC转录因子调控。
植物对干旱的响应是一个复杂的过程, 受不同分子和细胞通路的调控。根据干旱胁迫信号转导过程中, 胁迫相关基因的表达是否依赖ABA, 存在两种途径, 其中, ABA依赖型途径通过ABRE结合bZIP转录因子或者通过结合MYB/MYC转录因子激活下游基因的表达; 非ABA依赖型途径则由信号激活传感蛋白, 传递给DREB后结合DRE元件启动下游抗逆基因的表达。研究表明, 与干旱胁迫相关的F-box基因家族基因, 对ABA有不同程度的响应, 例如, ABA处理条件下, DOR表达量降低, 对ABA起负调作用[ 30]; 与对照相比, DEL3的表达量上升, edl3-1表达量下降[ 43]。在ABA胁迫下, SiF-box18基因表达量在24 h达到最高, 是对照的5倍(图6)。推测 SiF-box18基因可能通过依赖ABA途径参与谷子的干旱胁迫。
在干旱和高盐胁迫下, SiF-box18基因的表达量在24 h达到最高, 分别是对照的42倍和18倍(图6)。在植物外源激素GA、SA、JA、ABA处理下, SiF-box18基因表达量均有不同程度的增加(图6), 说明 SiF- box18基因可能参与了GA、SA、JA等植物激素的信号传导过程。 SiF-box18基因的启动子区包含1个ABRE、4个DRE、3个LTRE、45个MYB和14个MYC等多种非生物胁迫响应元件。说明 Si-Fbox18基因可能参与干旱、低温等多种非生物胁迫响应。
4 结论19个上调表达的谷子F-box基因位于8条染色体上, 其中SiF-box18蛋白被定位在细胞核中, 对干旱、高盐和ABA等都有响应, 推测 SiF-box18基因可能在非生物胁迫响应中起重要的作用。
附表
F-box protein | Gene identifier | Name | Organism | Function | Reference | |
---|---|---|---|---|---|---|
COI1 | At2g39940 | Coronatine Insensitive 1 | Arabidopsis | Jasmonate-Regulated defense responses, pollen fertility | [ 1] [ 2] [ 3] | |
ORE9/ MAX2 | At2g42620 | Oresara 9/ More Axillary 2 | Arabidopsis | Regulation of leaf senescence, selective repression of axillary shoots | [ 4] [ 5] [ 6] | |
TIR1 | At3g62980 | Transport Inhibitor Response 1 | Arabidopsis | Auxin receptor | [ 7] [ 8] [ 9] [ 10] | |
AFB1/GRH1 | At4g03190 | Auxin signaling F Box protein 1 | Arabidopsis | Auxin receptor, plant growth and development | [ 11] [ 12] [ 13] | |
AFB2 | At3g26810 | Auxin signaling F Box protein 2 | Arabidopsis | Auxin receptor, plant growth and development, glucose repression | [ 11] [ 12] [ 13] | |
AFB3 | At1g12820 | Auxin signaling F Box protein 3 | Arabidopsis | Auxin receptor, plant growth and development | [ 11] [ 12] [ 13] | |
AFB4 | At4g24390 | Auxin-signaling F-box protein 4 | Arabidopsis | Plant growth, development and innate immunity | [ 14] | |
SON1 | At2g17310 | Suppressor Of nim1-1 | Arabidopsis | Defense responses | [ 15] | |
UFO | At1g30950 | Unusual Floral Organs | Arabidopsis | Determination of floral meristem and floral organ identity | [ 16] [ 17] [ 18] [ 19] | |
EID1 | At4g02440 | Empfindlicher Im Dunkelroten Licht 1 | Arabidopsis | Negative regulator in phytochrome A-specific light signaling | [ 20] [ 21] | |
AFR | At2g24540 | Attenuated Far-Red Response | Arabidopsis | Positive regulator of phytochrome A-mediated light signaling | [ 22] | |
EBF1 | At2g25490 | EIN3-Binding F box protein 1 | Arabidopsis | Negative regulator of ethylene-signaling, plant growth | [ 23] [ 24] | |
EBF2 | At5g25350 | EIN3-Binding F box protein 2 | Arabidopsis | Negative regulator of ethylene-signaling, plant growth | [ 23] [ 24] | |
ZTL/LKP1 | At5g57360 | Zeitlupe | Arabidopsis | Circadian rhythm, photomorphogenesis and flowering time | [ 25] [ 26] | |
LKP2/FKL/ FKF1 | At2g18915 | Lov Kelch Protein 2 | Arabidopsis | Circadian clock regulation and flowering time control | [ 27] [ 28] [ 29] [ 30] [ 31] | |
SLY1 | At4g24210 | Sleepy 1 | Arabidopsis | Positive regulator of Gibberellic acid signaling | [ 32] [ 33] | |
SNE | At5g48170 | Sneezy | Arabidopsis | Positive regulator of Gibberellic acid signaling | [ 34] [ 35] | |
CEG (AtSFL61) | At3g22650 | Cegenduo | Arabidopsis | Negative regulator of auxin-mediated lateral root formation | [ 36] | |
DOR | At2g31470 | Drought Tolerance Repressor 1 | Arabidopsis | Negative regulate drought stress | [ 37] | |
CFK1 | At5g42350 | COP9 INTERACTING F-BOX KELCH 1 | Arabidopsis | Controlling hypocotyl elongation | [ 38] | |
FOA1 | AT3G17320 | F-box overexpressed/ oppressed ABA signaling | Arabidopsis | Play a negative role in ABA signaling. | [ 39] | |
EDL3 | At3g63070 | EID1-like protein 3 | Arabidopsis | Negative acting factors in ABA nsignalling to degradation via the proteasome. | [ 40] | |
GID2 | AB100246 | Gibberellin Insensitive Dwarf 2 | Oryza sativa | Positive regulator of Gibberellic acid signaling | [ 41] [ 42] | |
D3 | AK065478 | Dwarf 3 | O. sativa | Control of tiller bud activity | [ 43] [ 44] | |
FBK12 | OS03g03570 | F-box protein containing a kelch repeat motif | O. sativa | Delay in leaf senescence and germination, and increased seed size | [ 45] | |
DDF1 | Dwarf and deformed flower 1 | O. sativa | Regulate vegetative growth and floral organ specification | [ 46] | ||
MAIF1 | Os02g44990 | miRNAs regulated and abiotic stress induced F-box gene | O. sativa | Plays the negative role in response to abiotic stress | [ 47] | |
FIM | S71192 | Fimbriata | Antirrhinum majus | Floral differentiation | [ 48] [ 49] | |
AhSLF-S2 | AJ297974 | Self-incompatibility Locus-encoded F-box protein | A. hispanicum | Controls pollen function of S-RNase-based self-incompatibility | [ 50] [ 51] [ 52] | |
SFB3 | AY571665 | S haplotype-specific F-box protein | Prunus avium | Male determinant of gametophytic self incompatibility | [ 53] [ 54] [ 55] |
The authors have declared that no competing interests exist.
作者已声明无竞争性利益关系。The authors have declared that no competing interests exist.
参考文献View Option
原文顺序
文献年度倒序
文中引用次数倒序
被引期刊影响因子
[1] | |
[2] | |
[3] | |
[4] | |
[5] | |
[6] | |
[7] | |
[8] | |
[9] | |
[10] | |
[11] | |
[12] | |
[13] | |
[14] | |
[15] | |
[16] | |
[17] | |
[18] | |
[19] | |
[20] | |
[21] | |
[22] | |
[23] | |
[24] | |
[25] | |
[26] | |
[27] | |
[28] | |
[29] | |
[30] | |
[31] | |
[32] | |
[33] | |
[34] | |
[35] | |
[36] | |
[37] | |
[38] | |
[39] | |
[40] | |
[41] | |
[42] | |
[43] |