Wide-bandgap copolymer donors with fused-ring acceptor units (FAUs) present excellent performance in nonfullerene organic solar cells due to their complementary light-absorption with nonfullerene acceptors, deep the highest occupied molecular orbital (HOMO) levels and high hole mobilities[1-4]. A bunch of FAU-based copolymer donors were developed in recent years, such as PM6[5], PM7[6], PBQx-TCl[7], PTQ10[8], PBQ6[9], P2F-EHp[10], D16[11], L1-S[12], D18[13, 14] and D18-Cl[15, 16]. They delivered >16% power conversion efficiencies (PCEs) in solar cells. To develop good FAUs is the key toward efficient FAU-based copolymer donors. A good FAU generally has a strong electron-withdrawing character that leads to a low HOMO level and a high open-circuit voltage (Voc), and a relatively large molecular plane that facilitates polymer stacking and enhances hole mobility. Recently, we developed copolymer donors D18 and D18-Cl by using dithieno[3',2':3,4;2'',3'':5,6]benzo[1,2-c][1,2,5]thiadiazole (DTBT) unit[13] (Fig. 1(a)). Thanks to the strong electron-withdrawing property and the rigid and extended molecular plane of DTBT, D18 and D18-Cl deliver outstanding PCEs up to 18.69%[13-16]. The success of D18 polymers stimulated us to design more high-performance copolymer donors with novel FAUs. In this work, we designed a wide-bandgap copolymer donor P1 by using a fused-ring imide building block, 5-methyl-4H-dithieno[3,2-e:2',3'-g]isoindole-4,6(5H)-dione (MDTID). Compared with the thiadiazole moiety in DTBT, the imide moiety in MDTID is more electron-withdrawing. The density functional theory (DFT) calculations show that MDTID has deeper HOMO and the lowest unoccupied molecular orbital (LUMO) levels than DTBT, suggesting the stronger electron-accepting capability of MDTID (Fig. 1(a)). DFT calculations also indicate that MDTID leads to a deeper HOMO for P1 than that of D18, thus benefiting Voc (Fig. S1).

class="figure_img" id="Figure1"/>
Download
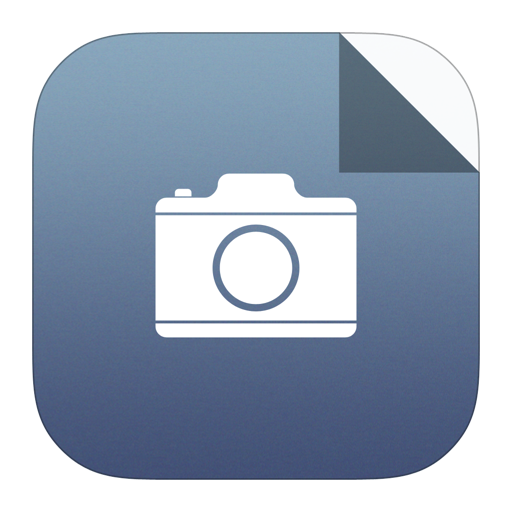
Larger image
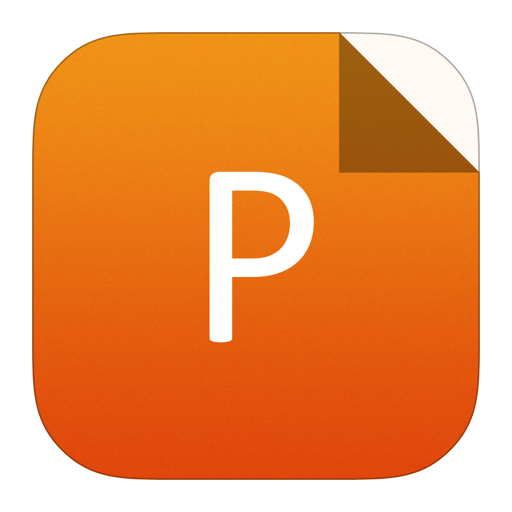
PowerPoint slide
Figure1.
(a) The structures of D18 and P1, and DFT-predicted molecular geometries, HOMO and LUMO of DTBT and MDTID units. (b) The synthetic route for P1. (c) Absorption spectra for P1 in CHCl3, and P1, N3 and IT-4F films. (d) J–V curves for P1:N3 and P1:IT-4F solar cells. (e) EQE spectra for P1:N3 and P1:IT-4F solar cells.
The synthetic route for P1 is shown in Fig. 1(b). Stille coupling of 3,4-dibromo-1-methyl-1H-pyrrole-2,5-dione and tributyl(thiophen-3-yl)stannane gave compound 1 in 83% yield. Scholl reaction[17] of compound 1 with FeCl3 afforded MDTID in 43% yield. Bromination of MDTID with NBS gave MDTID-Br in 90% yield. Stille coupling of MDTID-Br and tributyl(4-(2-butyloctyl)thiophen-2-yl)stannane gave compound 2 in 41% yield. Bromination of compound 2 with NBS gave monomer M1 in 81% yield. Finally, copolymerization of M1 with (4,8-bis(5-(2-ethylhexyl)-4-fluorothiophen-2-yl)benzo[1,2-b:4,5-b']dithiophene-2,6-diyl)bis(trimethylstannane) gave P1 in 75% yield. The number-average molecular weight (Mn) for P1 is 69.7 kDa, and the polydispersity index (PDI) is 1.73. P1 is soluble in chloroform and chlorobenzene.
The optical, electrochemical and hole-transporting properties of P1 were investigated. In solution, P1 shows an absorption band at 400–620 nm, with a peak at 536 nm (Fig. 1(c)). For the film, this peak shifts to 523 nm. The 13 nm-blueshift suggests the H-aggregation of P1 in film[18]. The absorption onset of P1 film is 601 nm, corresponding to an optical bandgap (Egopt) of 2.06 eV. The absorption spectra for acceptors N3[19] and IT-4F[20] are also shown in Fig. 1(c). They are complementary with that of P1. The HOMO and LUMO energy levels of P1 were estimated from cyclic voltammetry (CV) measurements (Fig. S11). An energy level diagram is given in Fig. S12. P1 has a HOMO of –5.54 eV and a LUMO of –2.78 eV. From CV, the HOMO of P1 is deeper than that of D18[10], similar to the result of DFT calculation (Fig. S1). The hole mobility (μh) of P1 was measured by using space-charge limited current (SCLC) method (Fig. S13)[21-25]. Pure P1 film presented a μh of 7.10 × 10–4 cm2/(V·s). The good μh of P1 could be due to the extended molecular plane of MDTID, which facilitates polymer packing and enhances charge transport.
Solar cells were made with a structure of ITO/PEDOT:PSS/active layer/PDIN/Ag. The D/A ratio, active layer thickness and additive content were optimized (Tables S1–S6). J–V curves and external quantum efficiency (EQE) spectra for the best cells are presented in Figs. 1(d) and 1(e), respectively. Performance data are listed in Table 1. The best P1:N3 cell gave a PCE of 14.52%, with a Voc of 0.90 V, a Jsc of 24.52 mA/cm2 and an FF of 65.8%, while the best P1:IT-4F cell gave a PCE of 12.46%, with a Voc of 0.95 V, a Jsc of 20.31 mA/cm2 and an FF of 64.6%. Both cells gave high Voc (> 0.9 V) due to the deep HOMO of P1. The Voc of P1:IT-4F cells is 0.05 V higher than that of P1:N3 cells due to the higher LUMO level of IT-4F (Fig. S12). Compared with P1:IT-4F cells, P1:N3 cells gave much higher Jsc due to the broader light-absorption of N3 than that of IT-4F. P1:N3 cells presented higher EQE at 760–960 nm (Fig. 1(e)). The integrated photocurrent densities from EQE spectra are 23.34 and 19.83 mA/cm2 for P1:N3 and P1:IT-4F cells, respectively, consisting with Jsc. From SCLC measurements (Figs. S14 and S15, Table S7), we found the μh and electron mobility (μe) are both higher in P1:N3 film than those in P1:IT-4F film, suggesting more efficient charge transport in the former cells. The μh/μe are 1.48 and 2.06 for P1:N3 and P1:IT-4F cells, respectively, suggesting charge transport is more balanced in P1:N3 cells, thus explaining the higher FF. The bimolecular recombination was studied by plotting Jsc against light intensity[26-29]. The α value is closer to 1 for P1:N3 cells, suggesting less bimolecular recombination (Fig. S16). We studied the morphology of blend films by using atomic force microscope (AFM) (Fig. S17). The root-mean-square roughnesses for P1:N3 and P1:IT-4F films are 0.80 and 1.76 nm, respectively. Typical nano-fibers were observed. The P1:N3 film might have more favorable phase separation.
D/A | Voc (V) | Jsc (mA/cm2) | FF (%) | PCE (%) |
P1:N3 | 0.90 | 24.52 (23.34)a | 65.8 | 14.52 (14.25)b |
P1:IT-4F | 0.95 | 20.31 (19.83)a | 64.6 | 12.46 (12.29)b |
a The data in the parentheses are integrated photocurrent densities from EQE spectra; b the data in the parentheses are averages for 10 cells. |
Table1.
Performance data for P1:N3 and P1:IT-4F solar cells.
Table options
-->

Download as CSV
D/A | Voc (V) | Jsc (mA/cm2) | FF (%) | PCE (%) |
P1:N3 | 0.90 | 24.52 (23.34)a | 65.8 | 14.52 (14.25)b |
P1:IT-4F | 0.95 | 20.31 (19.83)a | 64.6 | 12.46 (12.29)b |
a The data in the parentheses are integrated photocurrent densities from EQE spectra; b the data in the parentheses are averages for 10 cells. |
In short, we designed a copolymer donor by using a fused-ring imide unit MDTID. The polymer delivered high Voc (> 0.9 V) and decent PCEs (up to 14.52%) in organic solar cells. This work indicates that MDTID is a promising building block.
Acknowledgements
We thank the National Key Research and Development Program of China (2017YFA0206600) and the National Natural Science Foundation of China (51773045, 21772030, 51922032 and 21961160720).
Appendix A. Supplementary data
Supplementary data to this article can be found online at