Remarkable progress has been made in conjugated copolymer donors due to the development of novel fused-ring acceptor (FRA) building units[1-15]. The copolymers based on FRA units have delivered excellent power conversion efficiencies (PCEs) up to 18.69% in organic solar cells (OSCs)[16]. Fused-ring aromatic lactones are promising FRA units[17-19]. Their merits include rigid and extended molecular geometry, strong electron-withdrawing capability and facile synthesis. These merits render the copolymer donors good hole mobility, deep HOMO levels (the highest occupied molecular orbital) and low cost. Our group first reported a copolymer donor L1 based on a fused-ring aromatic lactone, 5H-dithieno[3,2-b:2',3'-d]pyran-5-one (DTP)[17]. Inverted and conventional solar cells based on L1 and a nonfullerene acceptor Y6[20] afforded 14.36% and 14.63% PCEs, respectively. To further improve the performance, we designed a fused-ring aromatic thiolactone unit, 5H-dithieno[3,2-b:2',3'-d]thiopyran-5-one (DTTP), and synthesized the thiolactone copolymer donor D16[18]. It was found that the replacement of oxygen by sulphur on lactone moiety enhanced π–π stacking and hole mobility of the copolymer. Consequently, conventional solar cells based on D16 and Y6 gave an improved PCE of 16.72%. However, compared with the commercially available DTP unit, the DTTP unit shows synthetic complexity[21], thus increasing the cost of D16. In this work, we report a simple and effective post-sulphuration strategy to enhance the performance of L1. By directly reacting with Lawesson reagent, the carbonyl group on L1 can be transformed to thiocarbonyl group (Fig. 1(a)). The resulting sulphurated copolymer L1-S exhibits a deeper HOMO level, stronger light absorption, higher hole mobility and superior photovoltaic performance. Solar cells based on L1-S and a nonfullerene acceptor BTP-eC9[22] gave a PCE of 17.73% (certified 17.1%). L1-S is among the few donors with >17% certified PCEs to date[1, 23-25].

class="figure_img" id="Figure1"/>
Download
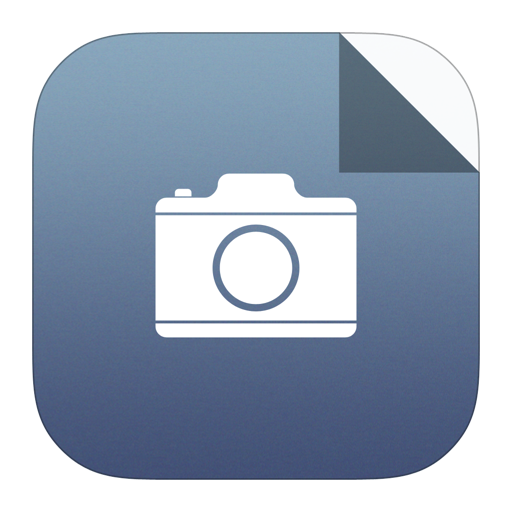
Larger image
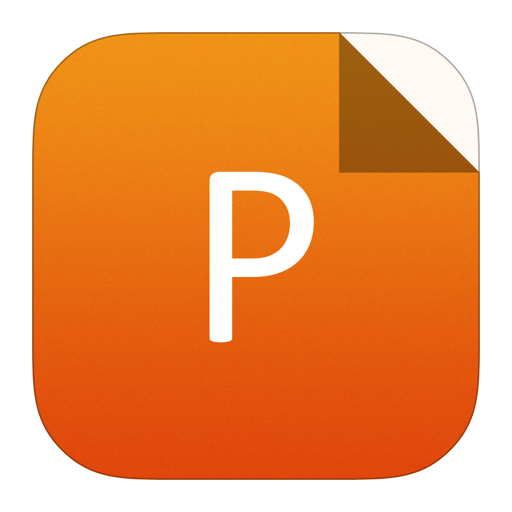
PowerPoint slide
Figure1.
(a) The post-sulphuration of L1 to L1-S. (b) Absorption spectra for L1 and L1-S films. (c) Energy level diagram. (d) J–V curves for L1:BTP-eC9 and L1-S:BTP-eC9 solar cells. (e) EQE spectra for L1:BTP-eC9 and L1-S:BTP-eC9 solar cells.
The process of the post-sulphuration is very simple (Scheme S1). Heating a mixture of L1 and Lawesson reagent in chlorobenzene overnight smoothly produced a dark-red solution. The solution passed through a short silica gel column to remove small molecule impurities. It was then added into methanol and the precipitate was collected to give L1-S as a brown solid. We also tried a pre-sulphuration route to synthesize L1-S via a sulphurated monomer M1-S (see Supporting Information). However, the final Stille copolymerization failed probably due to the coordination of the thiocarbonyl of M1-S with Pd, which poisoned the Pd catalyst and terminated the polymerization (Scheme S2). Compared with the starting material L1 that with a number-average molecular weight (Mn) of 42.5 kDa and a polydispersity index (PDI) of 2.03, L1-S presents similar Mn of 42.8 kDa and PDI of 2.05, suggesting that the polymer chain was not cleaved during the reaction. The FT-IR spectra for L1 and L1-S are shown in Fig. S5. From L1 to L1-S, the characteristic peak at 1736 cm?1 (C=O stretching vibration) disappears, indicating that carbonyl group was converted to thiocarbonyl group.
The optical and electrochemical properties of L1-S are quite different from L1. Absorption spectra for L1 and L1-S films are shown in Fig. 1(b). Compared with L1, L1-S shows a bathochromic-shifted spectrum and a smaller bandgap. The optical bandgaps estimated from the absorption onsets are 1.96 and 1.88 eV for L1 and L1-S, respectively. The absorption coefficients for L1 and L1-S films are 7.66 × 104 and 8.01 × 104 cm?1, respectively, suggesting the stronger light absorption of L1-S. HOMO and the lowest unoccupied molecular orbital (LUMO) levels were estimated from cyclic voltammetry (CV) measurement (Fig. S6) and an energy diagram is shown in Fig. 1(c). Compared with L1, L1-S presents deeper HOMO and LUMO levels of –5.50 and –2.94 eV, respectively. The deeper HOMO favors to produce higher open-circuit voltage (Voc) in solar cells. We also performed density functional theory (DFT) calculations for L1 and L1-S (Fig. S7). The DFT-predicted HOMO and LUMO levels of L1-S are lower than the HOMO and LUMO levels of L1, respectively, consisting with the experimental results. Charge-transporting property of the polymers was evaluated by the space charge limited current (SCLC) measurements (Fig. S8, Table S7). Compared with L1 (hole mobility (μh) 5.93 × 10?4 cm2 V?1 s?1), L1-S shows a higher μh of 1.17 × 10?3 cm2 V?1 s?1. The higher mobility of L1-S might originate from S···S interactions[18, 26].
The solar cells with a structure of ITO/PEDOT:PSS/active layer/PDIN/Ag were made to evaluate the photovoltaic performance of L1-S. L1 was used as the reference. The D/A ratio, active layer thickness and additive content were optimized for L1:BTP-eC9 and L1-S:BTP-eC9 solar cells (Tables S1–S6). J–V curves and external quantum efficiency (EQE) spectra for the best L1:BTP-eC9 and L1-S:BTP-eC9 cells are shown in Figs. 1(d) and 1(e), respectively. The best L1 cells gave a PCE of 16.12%, with a Voc of 0.831 V, a short-circuit current density (Jsc) of 25.94 mA cm–2 and a fill factor (FF) of 74.8%, while the best L1-S cells afforded a higher PCE of 17.73%, with a Voc of 0.845 V, a Jsc of 27.22 mA cm–2 and a FF of 77.1%. The L1-S cells were also measured at the National Institute of Metrology (NIM), and a certified PCE of 17.1% (Voc, 0.827 V; Jsc, 26.47 mA cm–2; FF, 78.3%; effective area, 2.580 mm2) was recorded (Fig. S11). L1-S is among the few donors with >17% certified PCEs to date. Compared with the L1 cells, L1-S cells gave simultaneously improved Voc, Jsc and FF. The higher Voc can be attributed to the deeper HOMO of L1-S. The EQE spectra indicate higher response of L1-S cells than L1 cells in a wide spectral range, consisting with the higher Jsc for L1-S cells. By plotting photocurrent density (Jph) versus efficient voltage (Veff), we found the exciton dissociation probabilities (Pdiss) for L1 and L1-S cells are 98.3% and 98.7%, respectively, suggesting more efficient exciton dissociation in the latter (Fig. S12). Charge transport and recombination were further investigated to understand why L1-S cells gave higher FF. The SCLC measurements indicate that in L1-S cells the μh and electron mobility (μe) are both enhanced, and charge transport are more balanced (Figs. S9 and S10, Table S7). The bimolecular recombination study indicated that the α values for L1 and L1-S cells are 0.968 and 0.980, respectively, suggesting less bimolecular recombination in L1-S cells (Fig. S13). Therefore, owing to the enhanced and more balanced charge transport and the suppressed charge recombination, L1-S cells offered better FF. Finally, we studied the morphology of L1:BTP-eC9 and L1-S:BTP-eC9 blend films by using atomic force microscope (AFM) (Fig. S14). It was found that both films present clear nano-structures. The diameters for the nanofibers are ~28 and ~18 nm for L1:BTP-eC9 and L1-S:BTP-eC9 films, respectively. The root-mean-square roughnesses for L1:BTP-eC9 and L1-S:BTP-eC9 films are 9.94 and 2.07 nm, respectively. The finer nanofibers and the much smoother surface of L1-S:BTP-eC9 film suggest a higher miscibility between L1-S and BTP-eC9. The morphology of L1-S:BTP-eC9 film could be more favorable for charge generation and transport, thus improving Jsc and FF.
In summary, treating lactone polymer L1 with Lawesson reagent smoothly turns the carbonyl group into thiocarbonyl group and produces the sulphurated polymer L1-S. Compared with L1, L1-S presents a deeper HOMO level, a smaller bandgap, a higher absorption coefficient and a higher hole mobility, leading to simultaneously improved Voc, Jsc and FF in solar cells. An impressive PCE of 17.73% was achieved from L1-S:BTP-eC9 cells, higher than that from L1:BTP-eC9 cells (16.12%). Considering that many high-performance donors contain carbonyl groups, this post-sulphuration strategy may be applied to develop new efficient polymer donors.
Acknowledgements
We thank the National Key Research and Development Program of China (2017YFA0206600) and the National Natural Science Foundation of China (51773045, 21772030, 51922032 and 21961160720) for financial support. X. Zhang thanks China Petrochemical Corporation (2020GKF0657).
Appendix A. Supplementary materials
Supplementary materials to this article can be found online at