Thin-film transistors (TFTs) based on oxide semiconductors have gained a lot of attention in applications such as displays and sensors particularly in recent years due to the advantages of oxide semiconductors like high mobility, good uniformity over large area and low deposition temperature[1-4]. However, the defects/traps at dielectric/channel interface and top surface of oxide TFTs might dramatically degrade device performance including current on/off ratio, mobility and most importantly stability[5, 6], making it quite urgent to systematically make effective interface engineering to improve TFT performance.
Traps on the top channel surface are mainly caused by the adsorbed water and oxygen molecules from air[7], which could be reduced by applying a passivation layer. One effective passivation layer is organic self-assembled monolayer (SAM), which can be formed densely on the surface of oxide semiconductors through the reaction with –OH groups, ensuring a reliable interface coupling between SAM and the channel layer and hence a good chemical stability[5, 8]. Compared with conventional inorganic passivation layers, SAMs can be easily applied on the top channel surface via vapor- or solution-based methods[8-10], which are plasma-free processes and can avoid the potential plasma damage to oxide semiconductors.
SAMs with different functional groups might give very different surface energy and dramatically affect the resulting device performance, especially the stability and hysteresis. Recently, Kim et al. investigated InGaZnO (IGZO) TFTs treated by SAMs with CH3, NH2 and CF3 functional groups, namely trimethoxy(propyl)silane (TPS), (3-aminopropyl)trimethoxysilane (APTMS), and trimethoxy(3,3,3-trifluoropropyl)silane (TFP) SAMs, respectively, as shown in the insets of Fig. 1(a)[11]. The untreated IGZO film shows a contact angle of 22.5°, after the treatment, it changes to 55.2° ± 1.7°, 81.9° ± 2.1° and 98.1° ± 2.3° for APTMS, TPS, and TFP treated IGZO films, respectively, suggesting a reduced surface energy. Such a reduced surface energy makes oxygen molecules being difficult to be adsorbed on the surface of the treated IGZO films. As a result, a decrease of both clockwise hysteresis and threshold voltage shift under the positive bias was observed after the treatment with a lowest value of 0.11 ± 0.06 V and 0.32 ± 0.26 V, respectively, achieved in TFP-treated IGZO TFTs (the lowest surface energy case), as shown in Fig. 1(a).

class="figure_img" id="Figure1"/>
Download
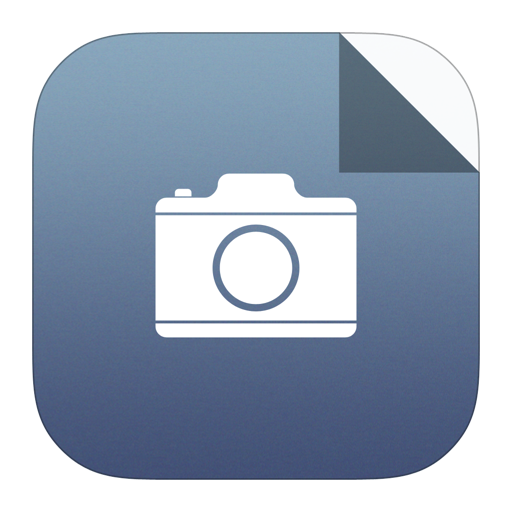
Larger image
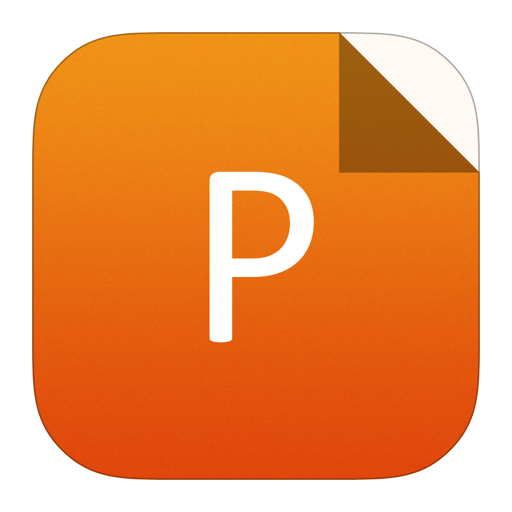
PowerPoint slide
Figure1.
(Color online) (a) Transfer characteristics of IGZO TFTs treated with different SAMs under positive bias stress. Insets show the chemical structures of SAM molecules. Reproduced with permission[11], Copyright 2021, IOP Publishing. (b) Transfer characteristics of IGZO TFTs with and without OTS treatment. (c) OTS-treated IGZO TFTs before and after being stored in air for a year. Reproduced with permission[8], Copyright 2021, American Chemical Society.
Alkyl chain lengths also affect device performance, as reported by Peng et al. who studied the relationship between SAM chain lengths and TFT performance by using triethoxysilane (TES) with three different alkyl chains, namely C1-TES, C8-TES and C18-TES[12]. All treated devices show an increased mobility and a decreased hysteresis compared with the untreated one. Among all treated devices, TFTs treated with C18-TES showed best performance with a mobility of 26.6 cm2/(V·s), which might be due to the formation of a well-ordered and more hydrophobic IGZO surface when treated with SAMs with longer alkyl chains. Similar effects were also reported by Chen et al. in InSnZnO TFTs treated with vapor-phase SAMs[9].
At smaller channel thicknesses, the accumulation layer approaches near the adsorbed water molecules on the top channel surface, inducing a strong carrier scattering and a more pronounced influence of top surface. To study whether the SAM treatment also works in TFTs with a thin channel layer, Song et al. made n-octadecyltrichlorosilane (OTS)-treated IGZO TFTs with different IGZO thicknesses[8]. As shown in Fig. 1(b), even at an IGZO thickness down to 5 nm, the treated devices show a high mobility of 10 cm2/(V·s) with a low subthreshold swing of 64 mV/dec and a high current on/off ratio larger than 106. Also, the device maintains a high performance even after being stored in air for a year (Fig. 1(c)), indicating that the top surface has been effectively passivated.
Besides being used as an effective passivation layer on the top surface of oxide TFTs, SAMs can also be applied at the dielectric/channel interface, which affects not only the dynamic performance but also the stability. SAM treatment is now a standard process in organic TFTs to reduce dielectric/channel interface traps and surface energy, but was seldom reported in oxide TFTs due to the potential damage to SAMs. By treating AlOx gate dielectrics with an n-octadecylphosphonic acid (ODPA), Bashir et al. reported high-performance ZnO TFTs made by spray pyrolyzing[13]. To study the survival of ODPA after the high-temperature ZnO deposition, they performed a contact angle measurement, and found that a high contact angle maintained even after a heat treatment of the sample at 400–450 °C in N2 (Fig. 2(a)), demonstrating the high stability of the ultra-thin SAM against heat. The SAM treatment here significantly reduces the gate leakage current, and as a result, the devices show a low operating voltage of 1.5 V with a current on/off ratio of 103 and a mobility of 8.3 cm2/(V·s) (Fig. 2(b)).

class="figure_img" id="Figure2"/>
Download
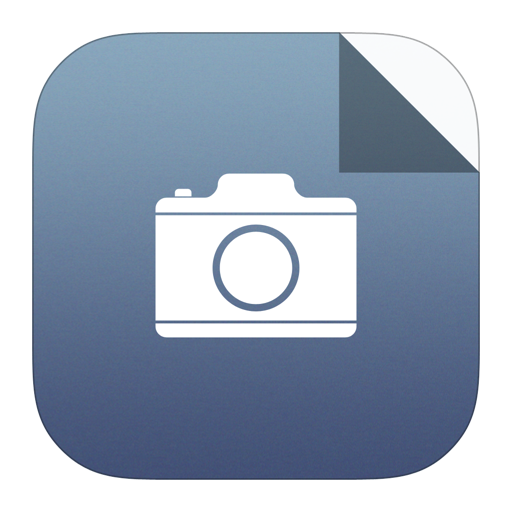
Larger image
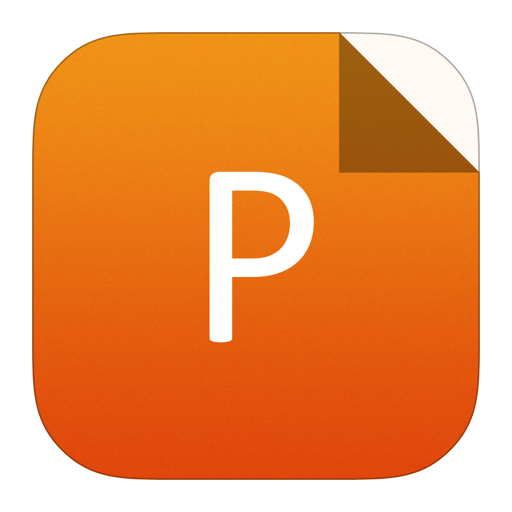
PowerPoint slide
Figure2.
(Color online) (a) Chemical structure of ODPA and contact angles of AlOx, SAM-treated AlOx before and after annealing. (b) Transfer characteristics of ZnO TFTs. Reproduced with permission[13], Copyright 2021, Wiley-VCH. (c) Transfer characteristics of IGZO TFTs with bare AlxOy and OTS-treated AlxOy as gate dielectrics. (d) Transfer characteristics of IGZO TFTs with bare HfOx and OTS-treated HfOx under positive bias stress. Reproduced with permission[14], Copyright 2021, Wiley-VCH.
However, for commercialization, high-performance oxide semiconductors are still mainly deposited by sputtering. To study the effectiveness of a SAM treatment on gate dielectrics in TFTs with a sputtered channel layer, in 2020, Song et al. prepared OTS-treated AlxOy and HfOx as the gate dielectrics in sputtered IGZO TFTs[14]. Surprisingly, they found that by carefully controlling the sputtering condition, a reduced interface trap density and hence an enhanced device performance could be realized. Under optimized conditions, the devices exhibit a more than two-fold increase of mobility, an increase of current on/off ratio by ~100 times and a reduction of trap density by >50% (Fig. 2(c)). The bias stress stability of the TFTs also showed a substantial improvement after the OTS treatment (Fig. 2(d)), mainly due to the significantly reduced interface trap density, demonstrating the potential of the method in manufacturing display back plane drivers.
In summary, the SAM treatment, as a simple and yet effective interface engineering method, gains wide attention in oxide TFTs not only on the top surface but most importantly at the dielectric/channel interface. To make this method a standard process in the manufacture of low-cost, oxide-based electronic devices, it is necessary to further study the large-area compatibility as this method may require scrupulously choosing SAMs and carefully controlling the deposition condition. Further enhancement in device performance could be realized through the combination of the treatments at both top surface and dielectric/channel interface.
Acknowledgements
W. Cai and Z. Zang thank National Natural Science Foundation of China (11974063), Natural Science Foundation of Chongqing (cstc2020jcyj-jqX0028), China Postdoctoral Science Foundation (2020M683242), and Chongqing Special Postdoctoral Science Foundation (cstc2020jcyj-bshX0123) for financial support. L. Ding thanks National Key Research and Development Program of China (2017YFA0206600) and National Natural Science Foundation of China (51773045, 21772030, 51922032, and 21961160720) for financial support.