1.
Introduction
A conventional MOSFET maintains its performance beyond 100 nm. Several adverse effects arise due to reduction in channel length reduction depending on scaling trends[1]. Due to the scaling of microelectronic technologies, the electrical performance of the device and effects of short channels should be reduced[2]. New structures are designed such as double-gate MOSFET[3], tri-gate MOSFET, multi-gate MOSFET to compensate the effects of short channel lengths. These MOSFETs were proposed as an alternative to bulk MOSFET beyond 45 nm. Double gate MOSFET has many advantages over the bulk MOSFET[1, 2]. It reduces the short channel effects, junction capacitance and provides dielectric isolation SOI (silicon on insulator) is also used in CMOS technology due to its high-speed performance and low power consumption[4]. Surround gates used in MOSFET allow more channel width which increases the drive current[5]. Capacitance model of material engineered CGT has also been proposed for improvement of short channel effects[6]. Square GAA MOSFET was proposed to be used in the device simulator and can easily be incorporated in compact models[7]. The gate all around junctionless MOSFET has been designed for high RF performance[8]. A TM-DG SOI MOSFET improves RF performance, linearity and analog performance[9]. A thermal model is proposed to predict the transfer of phonon in tri-gate SOI MOSFET and 3D MOSFET[10]. DG MOSFET has been used to design amplifier for better control of short channel effects[11]. JLDG MOSFET was proposed which reduces the short channel effects significantly[12]. A CSDG MOSFET was designed to store more energy and increase the current flow between source to drain[13].
In this paper, a comparison is made among different advance MOSFET structures on the basis of their ON and OFF-state performance. The ON and OFF-state performance are the deciding factor in future scaling trends of new transistor structures. The design of MOSFET structures also depends on different applications such as digital circuit, memory, analog/RF and biomedical applications. The paper describes application based MOSFET designs by exploring DC and AC performance parameters. Several MOSFET designs are implemented to check their circuit performance using circuit simulators available in TCAD tool. Such a prefabrication transistor structure and circuit design analysis plays an important role in achieving the desired performance and also to reduce failure or defect in the fabricated sample.
2.
Performance parameter
In general, the MOSFET structures are evaluated on the basis of their subthreshold performance and analog/RF performance. The important MOSFET parameters are needed to be discussed before any comparison among different MOSFET structures.
2.1
Subthreshold performance parameters
The region of operation before MOSFET channel inversion is known as the subthreshold region. The subthreshold parameters are a deciding factor to obtain a desired and reliable MOSFET performance.
2.1.1
Threshold voltage
The minimum amount of gate to source voltage required for channel inversion is known as MOSFET threshold voltage (VT). The value of threshold voltage depends mainly on surface potential which is the voltage of MOSFET capacitor surface (top layer of polysilicon or metal above the oxide) and voltage in the bulk of MOSFET.
2.1.2
OFF-state current
When the voltage of the gate is less than the threshold voltage the MOSFET is considered to be in OFF-state. However, in OFF-state there is a flow of current due to minority charge carriers between the drain and source. This current is known as subthreshold current.
2.1.3
ON-state current
When the voltage of the gate is more than the threshold voltage of the MOSFET. The MOSFET is said to be in ON-state. The flow of current in this state is known as ON current denoted by Ion. The movement of electrons takes place from source to drain.
2.1.4
DIBL (drain induced barrier lowering)
It is a short channel effect in which threshold voltage reduces originally at high drain voltage. When the length of the channel is short drain it is close enough to the gate, at high drain voltage the bottleneck opens and the transistor turns on prematurely. The value of DIBL should be as low as possible to obtain ideal output characteristic of MOS transistors reducing threshold voltage variations due to drain field effect on channel potential.
$${ m{DIBL}} = frac{{V_{{ m{th}}}^{{ m{dd}}} - V_{{ m{th}}}^{{ m{low}}}}}{{{V_{{ m{dd}}}} - V_{ m{d}}^{{ m{low}}}}}.$$ ![]() | (1) |
2.1.5
Subthreshold slope (SS)
In the subthreshold region, the gate terminal controls the drain current and the current is exponentially decreased. The slope of the drain current plot and gate voltage with drain, bulk and source voltages fixed gives subthreshold slope. A suitable value of subthreshold slope (~60 mV/decade) is required to limit the heating effect in short channel devices. The subthreshold slope can be expressed as:
$${ m{S}}{{ m{S}}_{{ m{th}}}} = { m{ln}} left( {10} ight)frac{{kT}}{q}left( {1 + frac{{{C_{ m{d}}}}}{{{C_{{ m{ox}}}}}}} ight).$$ ![]() | (2) |
2.2
Analog/RF performance parameters
The analog and RF performance mainly depends on transconductance, transistor capacitances, stability factor and cutoff frequency, etc.
2.2.1
Transconductance
Transconductance is the ratio of drain current variation with respect to the gate voltage of transistor over a small interval of time in the drain current versus gate voltage curve. It is represented as gm[10]. The higher value of transconductance is required to obtain a suitable range of amplifier gain.
$${g_{ m{m}}} = frac{{2{I_{{ m{ds}}}}}}{{left| {{V_{ m{p}}}} ight|}}left( {1 - frac{{{V_{{ m{gs}}}}}}{{{V_{ m{p}}}}}} ight).$$ ![]() | (3) |
2.2.2
Junction capacitance
Due to the depletion of charge between the source/drain and substrate the junction capacitances in MOSFET is formed. The charged depletion is changed according to the source/drain voltage. When the voltage of the gate exceeds the threshold voltage there is a formation of the channel at the surface. The junction capacitances are deciding factors for small signal analysis of transistor in RF range of frequencies.
2.2.3
Stability factor
The stability factor (K) mainly depends on two-port equivalent circuit parameters of MOS transistors. It decides the conditional or unconditional stability of transistors in RF range of frequencies. The stability factor can be expressed as[14]:
$$K = frac{{2{ m{Re}}[{Y_{11}}left] {{ m{Re}}} ight[{Y_{22}}left] { ;+; { m{Re}}} ight[{Y_{12}}{Y_{21}}]}}{{left| {{Y_{12}}{Y_{21}}} ight|}}.$$ ![]() | (4) |
Here, Y11 and Y22 are input and output admittance parameters at port 1 and 2 respectively. The Y12 and Y21 are called as transfer admittances.
2.2.4
Critical frequency
The critical frequency (fk) is essential for a small signal transistor model and also important to maintain a suitable AC transistor gain and frequency bandwidth. The critical frequency can be calculated at stability factor K = 1. The critical frequency mainly depends on MOSFET capacitance (Cgs, Cgd, and Cds etc.) and other parasitic capacitances. The critical frequency can be expressed as[15]:
$${f_{ m{k}}} cong frac{{{f_{ m{T}}}N}}{{sqrt {{g_{{ m{ds}}}}{g_{ m{m}}}{R_{{ m{gs}}}}{M^2} + NMleft( {{g_{ m{m}}}{R_{{ m{gd}}}} + 1} ight)} }}.$$ ![]() | (5) |
Here, fT, gds, Rds and Rgs are the frequency at unity gain, output conductance, drain to source and gate to source resistances respectively. Also, the M and N values are calculated in terms of Cgs and Cgd respectively.
3.
Device structure and dimensions
Sarkar et al.[6] presented a paper on the effect of engineering on DG MOSFET (Fig. 1). In this paper, a fully depleted tri material double gate MOSFET is used. Improvement in the RF performance, linearity and analog performance compared to the DM DG MOSFET and single material DG MOSFET. The structure is designed in 35 nm CMOS technology. In n-MOSFET, three different gate materials of different work functions are chosen. The work function of the materials are ΦM1 = 5.0 eV, ΦM2 = 4.75 eV, ΦM3 = 4.5 eV.

class="figure_img" id="Figure1"/>
Download
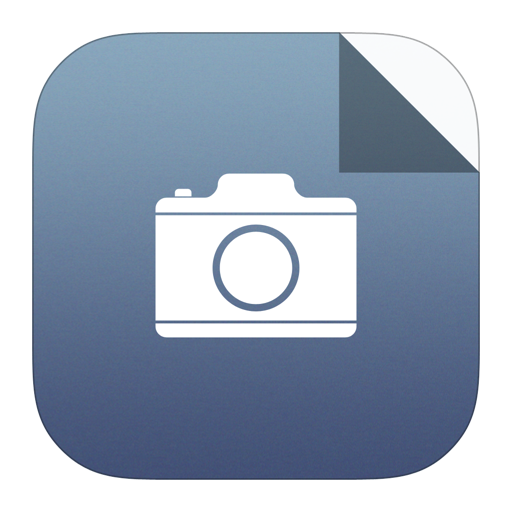
Larger image
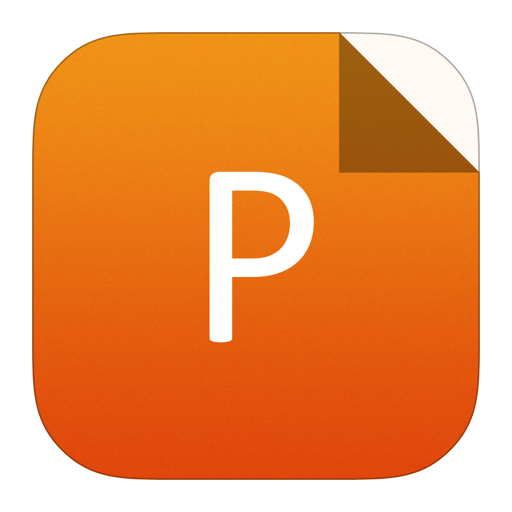
PowerPoint slide
Figure1.
TM-DG MOSFET structure.
The material of the gate having the larger work function in place close to the source and the material which is having the lesser work function is placed close to the drain. Polysilicon gates have depletion width of polysilicon and penetration effect of dopant so metal gates are used. The thickness of the Si film is 10 nm and SiO2 is 2 nm. The concentration of dopant of the source and drain is considered to be 1020 cm–3. The ratio of the length of the three material is taken as (L1 : L2 : L3 = 1 : 1 : 0). The doping concentration of the p substrate is taken as 1016 cm–3. Djeffal et al.[3] presented a paper on gate all around junctionless MOSFET (Fig. 2) with source and drain extension to improve analog and RF performances. In this structure, the source and drain extensions are heavily doped compared to the channel doping. The doping concentration is given as n++/n+/n++. A long channel structure is considered with film thickness of silicon less than 5 nm. The doping concentration Nd = 1018 cm–3, the concentration of the extension is taken as Next = 1019 cm–3, R = 5 nm, L = 100 nm, tox = 5 nm.

class="figure_img" id="Figure2"/>
Download
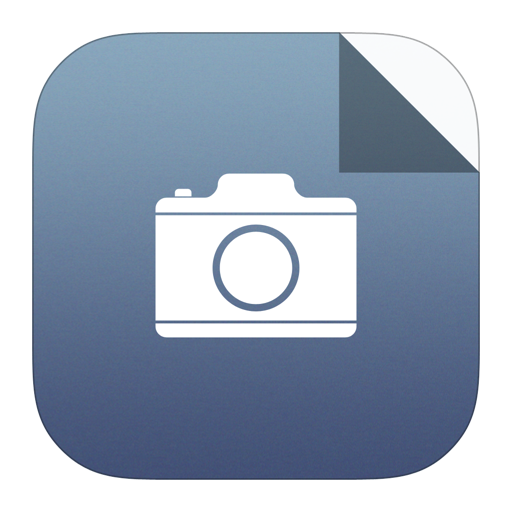
Larger image
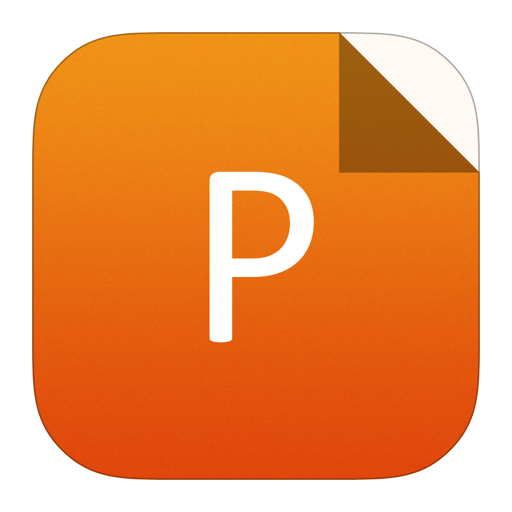
PowerPoint slide
Figure2.
GAAJ MOSFET with S/D extensions regions.
By including the heavily doped extensions the drain current improves. The GAAJ MOSFET having extensions has high current when compared to conventional GAAJ. The highly doped regions have increased ion current magnitude by 70%. Abhinav et al.[9] have discussed the reliability issues concerning junctionless double gate (JLDG) MOSFET (Fig. 3). In this paper, the gate misalignment and thermal stability between 200 to 500 K have been studied. The gate misalignment in this structure reduces the current which in turn reduces JLDG MOSFET's performance. The front and the back gate alignment effects the performance of the MOSFET. The effect of misalignment occurs due to the shifting of the back gate towards the source or drain side. The misalignment of gate produces non-ideal effects.

class="figure_img" id="Figure3"/>
Download
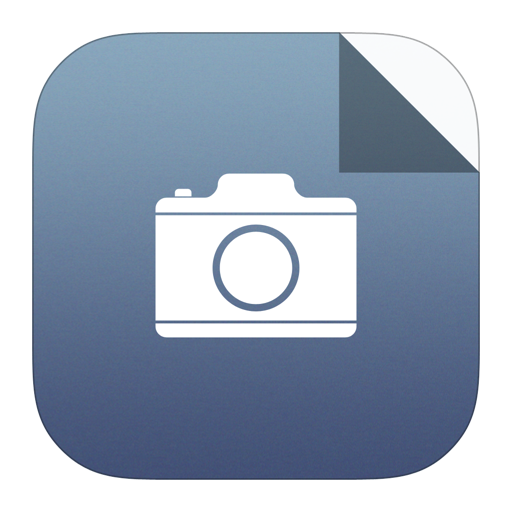
Larger image
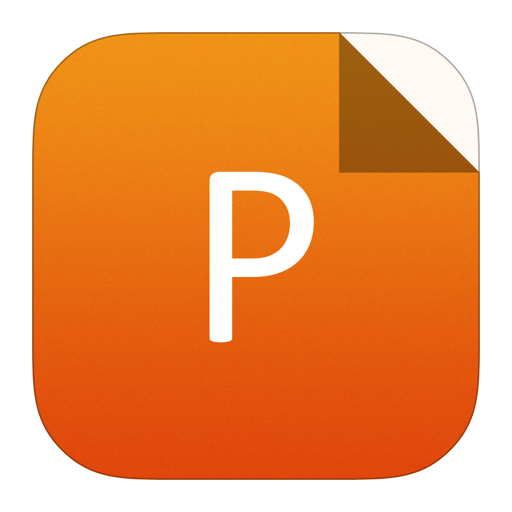
PowerPoint slide
Figure3.
(Color online) n-type JLDG MOSFET structure.
The dimensions of device parameters and gate work function is 5.2 eV, the thickness of front gate oxide is 1 nm, the thickness of back gate is 1 nm, the thickness of silicon substrate 5 nm, doping concentration Nd is 3 × 1019 cm–3, length of the channel L is 20 nm. Ouruji et al.[16] proposed a double step buried oxide (DSBO) SOI MOSFET (Fig. 4). This structure has both the advantages of bulk MOSFET and SOI structure. This structure is designed to reduce the self-heating by changing the shape of buried oxide into a double step shape for reducing the thickness of silicon dioxide. Heat exchange is easily done between the channel to substrate. In this structure there is increase in drain current irrespective of the self-heating effects. The parameter of the device are N+ source/drain doping is 1020 cm–3, N+ source/ drain extension doping is 1019 cm–3, P-type silicon film doping is 1015 cm–3, thin-film thickness is 10 nm, raised source/drain thickness is 50 nm, oxide thickness under the source/drain is 100 nm, oxide thickness under the channel is 20 nm, channel length is 30 nm, thickness of gate oxide is 1.5 nm.

class="figure_img" id="Figure4"/>
Download
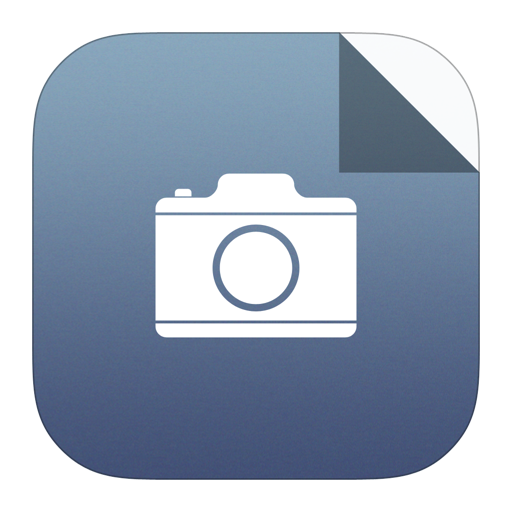
Larger image
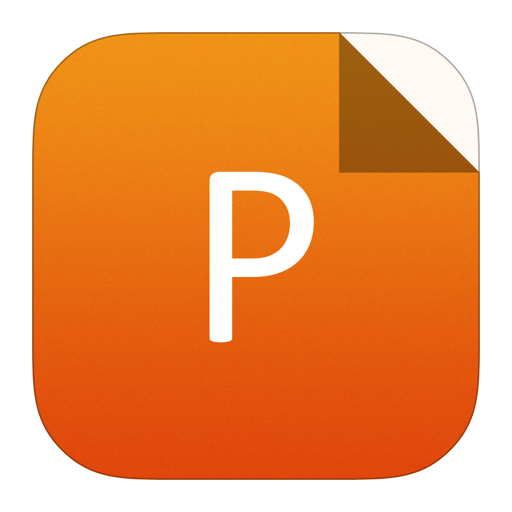
PowerPoint slide
Figure4.
(Color online) Structure of DSBO-SOI MOSFET.
Ajay et al.[12] presented a paper on two types of gate underlap junctionless double-gate MOSFET (JL DG MOSFET). In this paper, two cases are taken. The first case includes the gate underlap region present at the end portion of source of the JL DG MOSFET's (Fig. 5) channel region. The second case consists of the underlapped gate region is present at the end portion of the drain of the JL DG MOSFET (Fig. 6) channel region. Both types of structure are used to detect biomolecules using dielectric modulation techniques. The charged biomolecules produce an effect on the potential of the surface of JL DG MOSFET. When the biomolecules are positively charged the surface potential move upwards and when the biomolecules are negatively charged the surface potential move downwards. The parameters of the device are, gate length 50 nm, length of the cavity 50 nm, the thickness of cavity 19 nm, thickness of channel of 20 nm, thickness of gate oxide of 10 nm, the doping in source/drain and channel is 1 × 1024 m–3, the thickness of oxide layer in open cavity region is 1 nm.

class="figure_img" id="Figure5"/>
Download
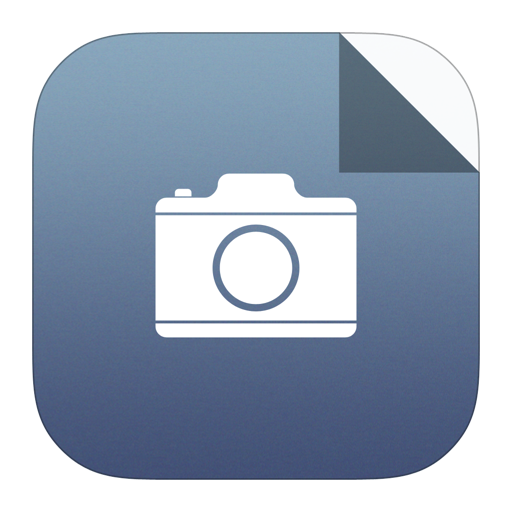
Larger image
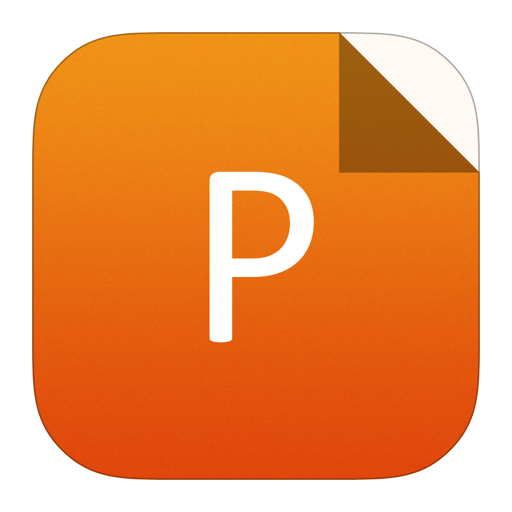
PowerPoint slide
Figure5.
JL DG MOSFET for underlapping at the source end of the channel region.

class="figure_img" id="Figure6"/>
Download
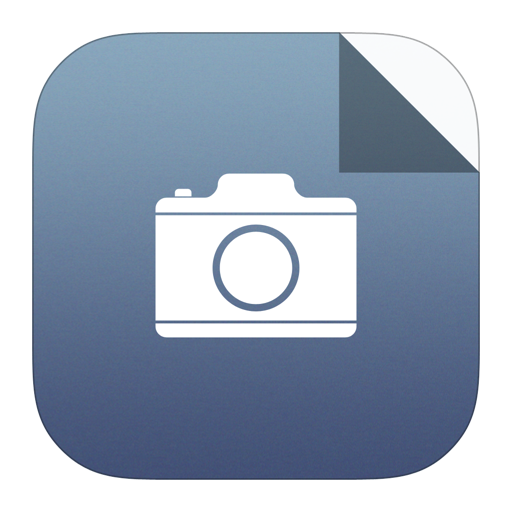
Larger image
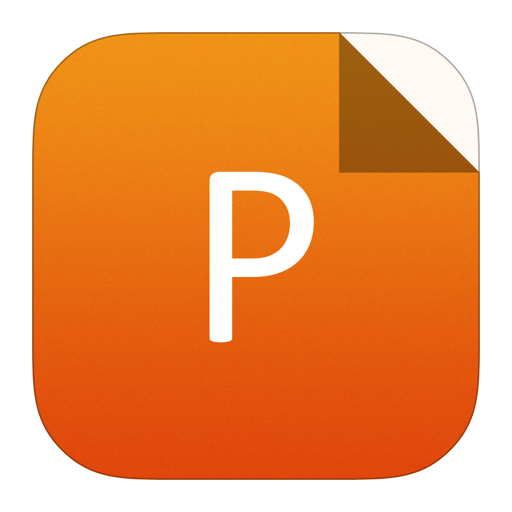
PowerPoint slide
Figure6.
JL DG MOSFET for underlapping at the drain end of the channel region.
Kwon et al.[17] proposed a paper on silicon-based MOSFET (Fig. 7) for the improvement of operation at high temperatures. The MOSFET is designed for harsh environmental applications[18]. Wide bandgap material is inserted locally between the regions of source and channel for high-temperature operation. The proposed SOI MOSFET structure has buried oxide (BOX) that prevents the leakage of current flowing in the substrate.

class="figure_img" id="Figure7"/>
Download
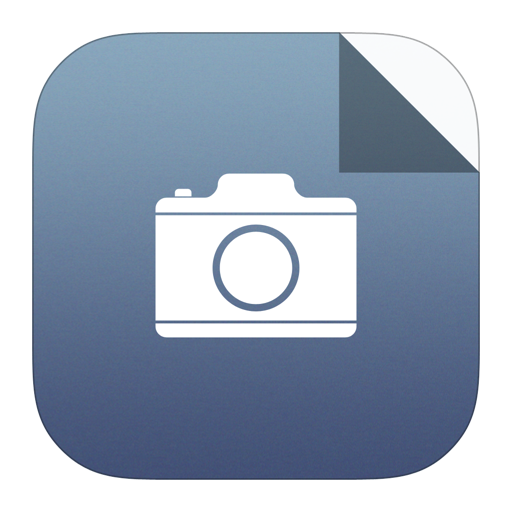
Larger image
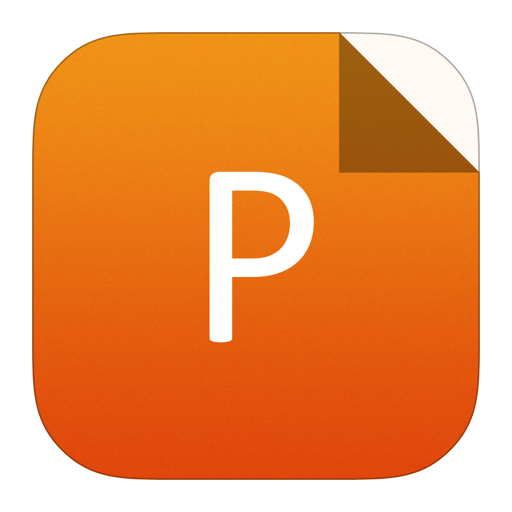
PowerPoint slide
Figure7.
(Color online) Structure of silicon-based MOSFET.
The device parameters are the length of gate given as 100 nm, thickness gate oxide is 3 nm, bottom oxide thickness is 10 nm, width of barrier is 10 nm, depth of barrier 75 nm, drain and source doping and substrate are 1 × 1020 and 1 × 1017 cm–3, respectively. Kumar et al.[19, 20] proposed a black phosphorus junctionless recessed channel MOSFET (Fig. 8) for RF application using 45 nm technology. Black phosphorus is integrated with the junctionless recessed MOSFET. In this structure drain current increases up to 0.3 mA. The OFF current reduces and there is improvement in subthreshold slope. The black phosphorus material has high ON current and low OFF current. The device parameters are length of gate is 20 nm, length of channel is 44 nm, length of drain and source region is 30 nm, device width is 200 nm, depth of groove is 38 nm, negative junction depth (NJD) is 10 nm, substrate doping is 5 × 1016 cm–3, doping of drain and source is 5 × 1016 cm–3, thickness of physical oxide is 2 nm, work function of metal is 5.16 eV, voltage of gate to source is 1.5 V, voltage of source to drain is 0.2 V.

class="figure_img" id="Figure8"/>
Download
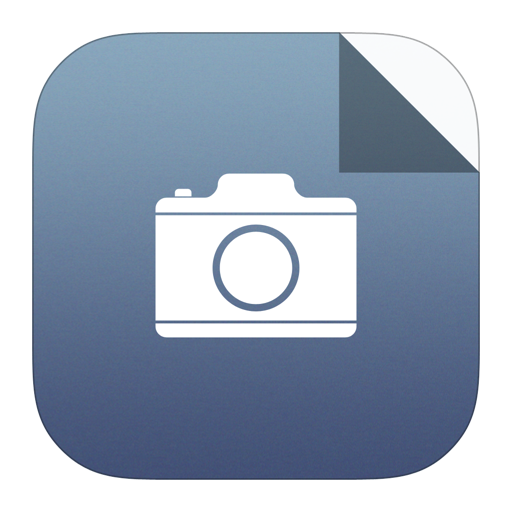
Larger image
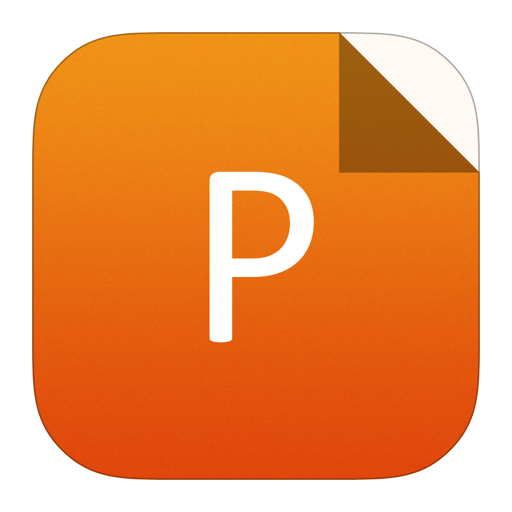
PowerPoint slide
Figure8.
(Color online) Design of BP JL RC MOSFET.
Djeffal et al.[21] proposed a dual material surrounded gate MOSFET of 10 nm for digital applications. The advantages of DMSG MOSFET's (Fig. 9) of 50 nm and multi-objective genetic algorithms (MOGAs) optimization technique has been combined. The MOGAs approach optimizes and improves the electrical behavior of 10 nm DMSG MOSFET. This provides low power consumption and nanoscale high speed digital applications. The formula for DIBL is given as

class="figure_img" id="Figure9"/>
Download
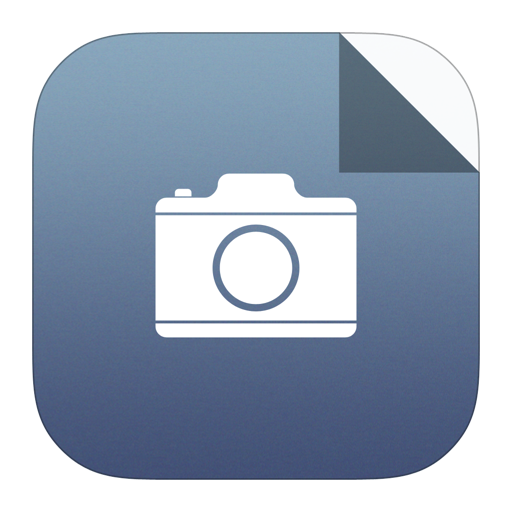
Larger image
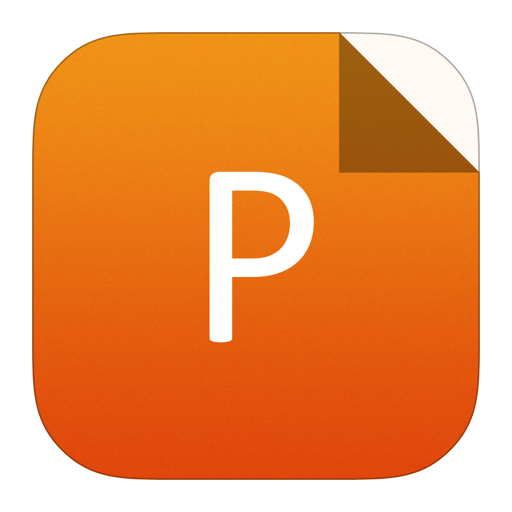
PowerPoint slide
Figure9.
Structure of DMSG MOSFET.
$${ m{DIBL}} = frac{{{V_{{ m{th}}}}left( {{V_{{ m{ds}}2}} = 0.4;{ m{V}}} ight) - {V_{{ m{th}}}}left( {{V_{{ m{ds}}1}} = 0.1;{ m{V}}} ight)}}{{{V_{{ m{ds}}2}} - {V_{{ m{ds}}1}}}}.$$ ![]() |
The device parameters are Na is 1015 cm–3, drain and source doping is 1020 cm–3, the length L is 10 nm, the thickness of oxide is 2 nm, silicon thickness is 10 nm, L1 and L2 is L/2.
Pathak et al.[22] proposed a GC-DMGJL MOSFET (graded channel, dual material gate junctionless MOSFET) shown in Fig. 10 for applications in the analog domain. In this paper GC-DMGJL performance is compared with uniform channel dual material gate junctionless (UC-DMGJL) MOSFET. The GC-DMGJL MOSFET gives high drain current and transconductance and also reduces short channel effects. The device consists of high doping area Ngd = 2.5 × 1019 cm–3 near drain region of the channel, rest of the regions are uniformly doped with Nd = 2 × 1019 cm–3. The ratio of length of the metal LM1 : LM2 (nm) = 15 : 15. The work function of the metal is given as WM1 : WM2 (eV) = 5.353 : 4.8. The thickness of oxide is given as 2 nm. Length of the spacer is Wsp = 10 nm. The thickness of silicon is Tsi = 10 nm.

class="figure_img" id="Figure10"/>
Download
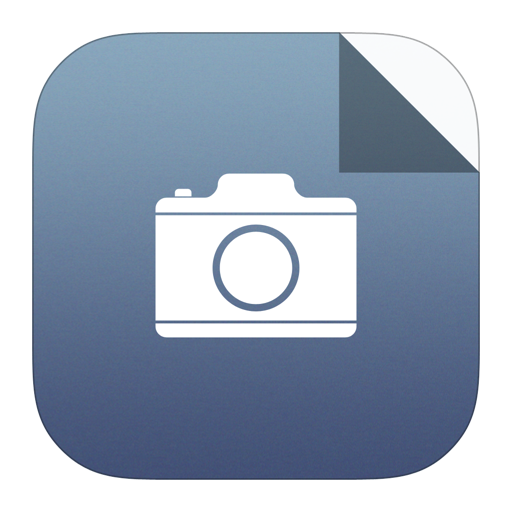
Larger image
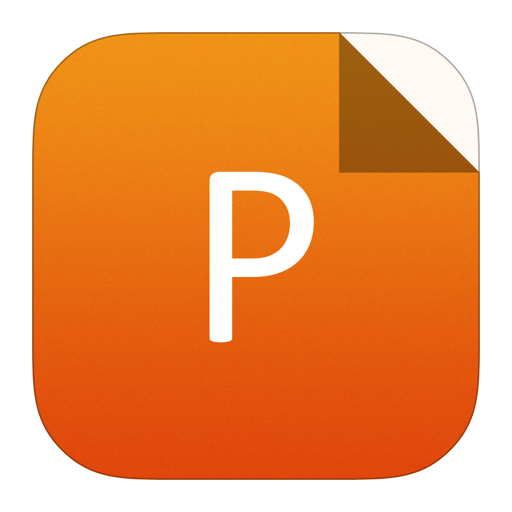
PowerPoint slide
Figure10.
(Color online) Structure of an n-type GC-DMGJLT.
Ajay et al.[23] proposed a junctionless metal oxide semiconductor field effective transistor structure (Fig. 11) which is capable of detecting biomolecules such as DNA, enzymes, cells, etc., using dielectric modulation technique. Formation of a nanogap cavity by using the process of gate oxide etching in the channel from both the sides of source and drain. The biomolecules affect the potential of the surface in the channel beneath the nanocavity that bind the SiO2 layer present in the cavity.

class="figure_img" id="Figure11"/>
Download
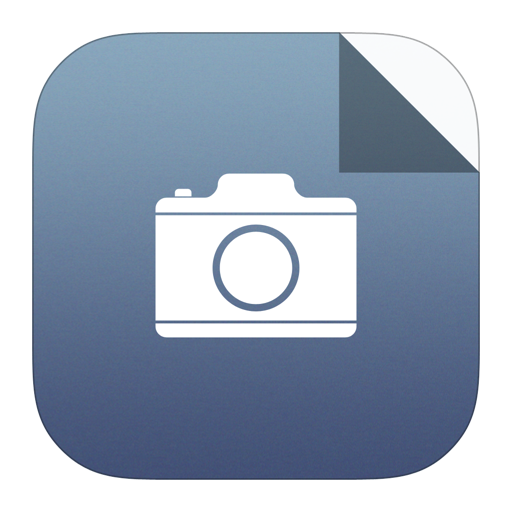
Larger image
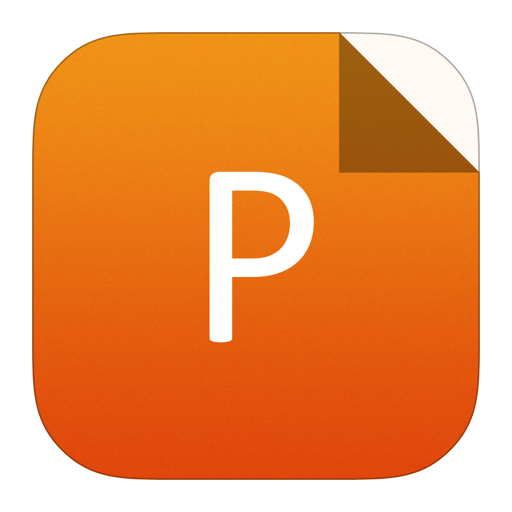
PowerPoint slide
Figure11.
(Color online) Junctionless MOSFET with a cavity for detecting biomolecules.
The dimensions of the device are tbio = 9 nm, tox1 = 1 nm, tsi = 10 nm, doping of the source, drain and channel is 1 ×1025 m–3. Length of the cavity is L1 and L3 is 25 nm, and L3 length of the oxide Al2O3 is 50 nm. Pang et al.[24] proposed a structure of 0.1 μm pocket of n-MOSFETs (Fig. 12) for applications of low voltage. In this structure a pocket region is constructed close to the drain and source region and is heavily doped and at the center of the structure center region is constructed which is lightly doped. This design provides good immunity from short channel effects and is able to meet the specifications of OFF current and ON current.

class="figure_img" id="Figure12"/>
Download
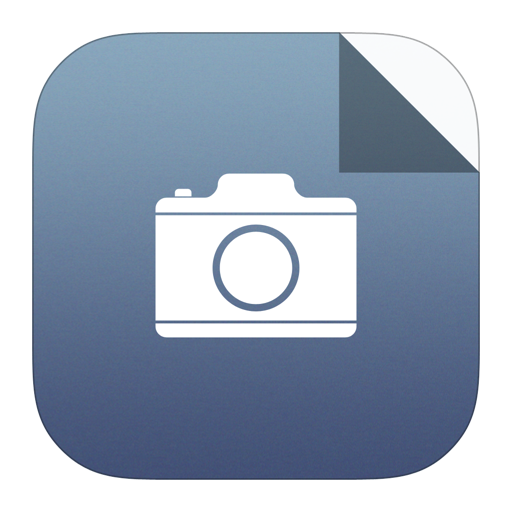
Larger image
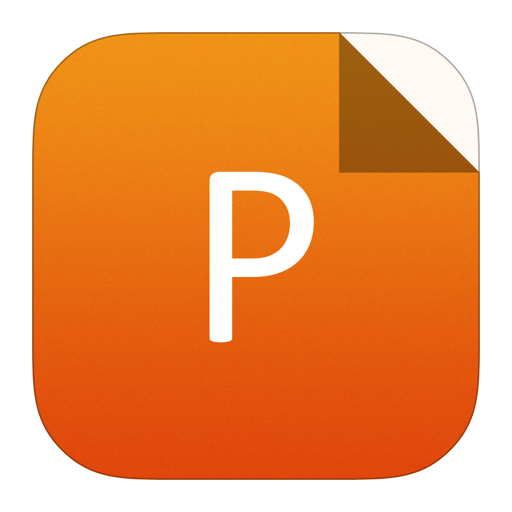
PowerPoint slide
Figure12.
Structure of a pocket n-MOSFET.
The device parameters are channel length which is 0.1 μm, the thickness of oxide is 4 nm, the junction depth (rj) is 0.06 μm, the doping concentration of the pocket (NP) is 1.906 × 1018 cm–3 and doping concentration in the center region (Nc) is 2.175 × 1017 cm–3, length of the pocket (LP) is 0.024 μm. Orouji et al.[25] proposed a structure of nanoscale SOI MOSFET which has electrically induced source and drain extensions for suppressing short channel effects length of channel less than 50 nm and also suppresses hot electron effects. The formation of shallow drain and source by fabrication is very difficult but EJ SOI MOSFET (Fig. 13) is able to form virtual drain and source electrically. This structure consists of triple gate, one main gate with two side gates. The biasing of side gates are independent of the main gate. These side gates form inversion layers which further forms virtual drain and source.

class="figure_img" id="Figure13"/>
Download
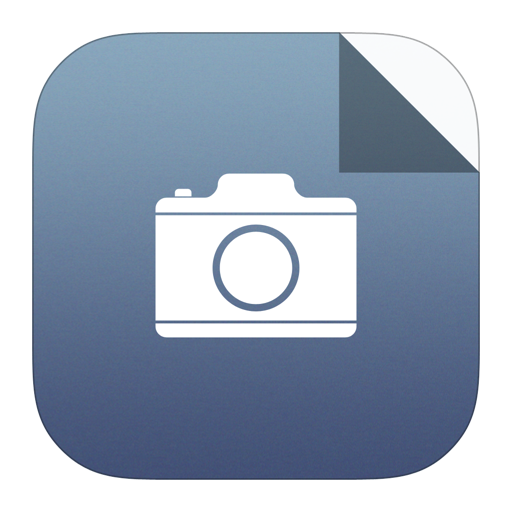
Larger image
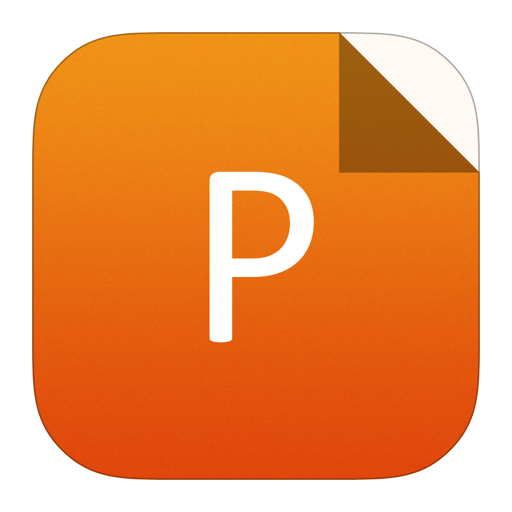
PowerPoint slide
Figure13.
Structure of EJ-SOI MOSFET.
The device parameters given are doping of silicon thin layer is 6 × 1016 cm–3, source and drain doping is 5 × 1019 cm–3, the side gates work function is 4.7 eV, the main gate work function is 4.9 eV, thickness of silicon thin layer is 50 nm, thickness of buried oxide is 500 nm, thickness of gate oxide is 2 nm, thickness of barrier diffusion layer is 2 nm, length of main gate is 50 nm and length of total side gate is 50 nm. Pal et al.[26] presented a paper on the study of the surrounding gate MOSFET with dual material (Fig. 14) to overcome short-channel effects. The parameters like threshold voltage, potential of the surface, and distribution of electric field are analytically modeled using parabolic approximation method. The comparison between DMSG and SMSG device structures having equal dimensions is taken out in terms of SCE's. The result shows that DMSG MOSFET suppresses (SCEs) more efficiently in comparison with SMSG MOSFET. The formula for DIBL calculation is given as

class="figure_img" id="Figure14"/>
Download
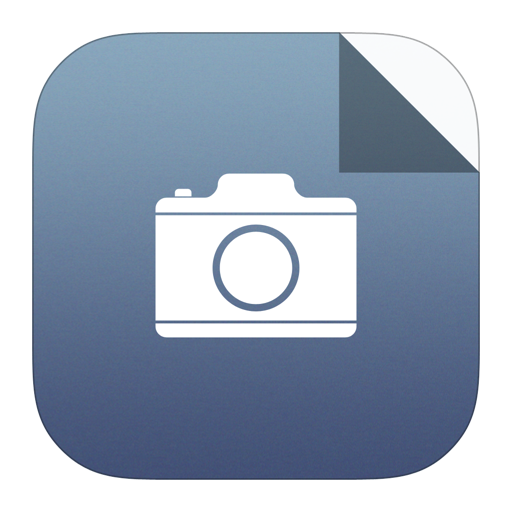
Larger image
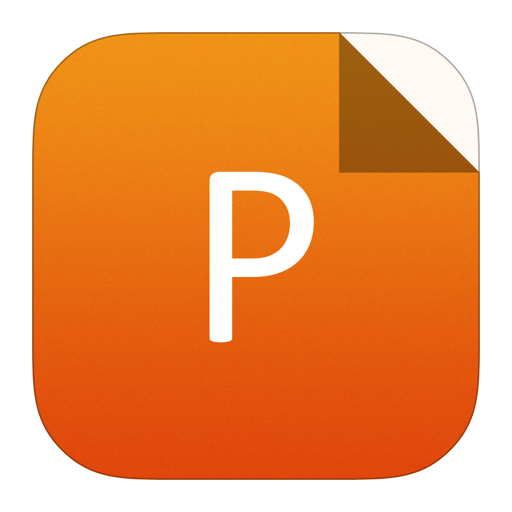
PowerPoint slide
Figure14.
Design of cylindrical surrounding gate MOSFET using dual material.
$${ m{DIBL}} = frac{{{ m{Delta }}{V_{{ m{th}}}}}}{{{ m{Delta }}{V_{{ m{ds}}}}}} = frac{{ {{V_{{ m{th}}1}} - {V_{{ m{th}}2}}} }}{{{V_{{ m{ds}}1}} - {V_{{ m{ds}}2}}}}.$$ ![]() |
The work function of gold (Au ΦM1 4.8 eV), work function of cadmium (Cd) is 4.0 eV. The channel doping of p-type is 6 × 1016 cm–3, n+ source and drain doping region is 5 ×1019 cm–3. Chebaki et al.[27] proposed a paper on double-gate junctionless MOSFET (Fig. 15) using engineered gate material and source/drain extensions. This structure is able to produce high drain current and improves RF and analog performance. The figure of merit is also increased compared to the conventional double gate junctionless MOSFET.

class="figure_img" id="Figure15"/>
Download
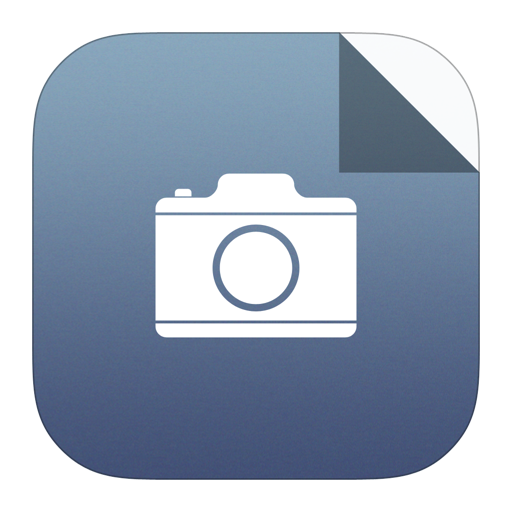
Larger image
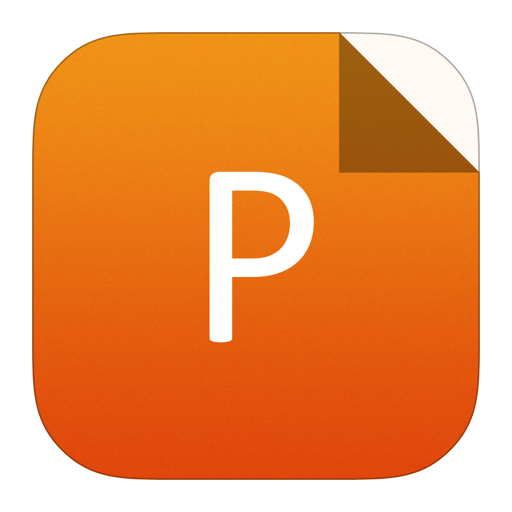
PowerPoint slide
Figure15.
Double Gate junctionless MOSFET with extensions and engineering of gate material.
The parameters of the devices are doping concentration Nd is 5 × 1018 cm–3, doping of the extension is 5 × 1019 cm–3, thickness of silicon tSi is 10 nm, L is 100 nm, L1 and L2 is L/2, the metal (M1) work function is 5.1 eV, metal (M2) work function is 4.5 eV. Wang et al.[28] proposed a junctionless MOSFET with asymmetrical gate (Fig. 16) to improve the functioning of the device. The device has two gates with a lateral offset[29] between them. In this structure the channel length depends upon the ON and OFF state of the MOSFET. The channel length of the MOSFET during the ON state is equal to the overlap length of the gate, and the channel length during the OFF state is combined length of the two gates minus the overlap length of the gate. This structure increases the ION to IOFF ratio and decreases the sub-threshold slope and DIBL.

class="figure_img" id="Figure16"/>
Download
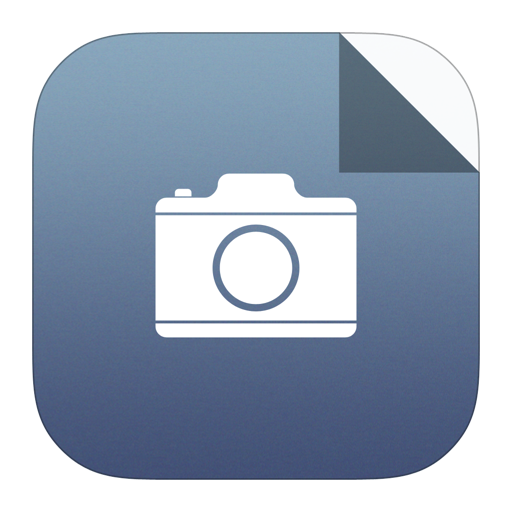
Larger image
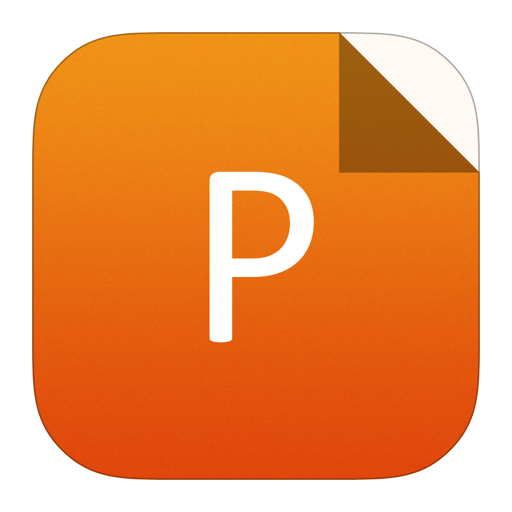
PowerPoint slide
Figure16.
(Color online) Junctionless MOSFET with asymmetric gate (AG-JL MOSFET).
The structure parameters are an oxide (HfO2) with EOT 1 nm, channel doping is 1 × 1019 cm–3, length of gate is 20 nm, thickness of silicon is 6 nm. Kumar et al.[30] proposed a paper on RF performance of the recessed channel with a transparent gate (Fig. 17). The values of transconductance, cut-off frequency, DIBL, and maximum oscillator frequency have been calculated. The outcomes of the structure are compared with the conventional recessed channel MOSFET. The MOSFET gate is made up of indium tin oxide which is a transparent material. The results show that there is increase of cut-off frequency by 42% and oscillator frequency is increased by 132%. Due to the improvement in the gate control the short channel effects are reduced by using the transparent gate material.

class="figure_img" id="Figure17"/>
Download
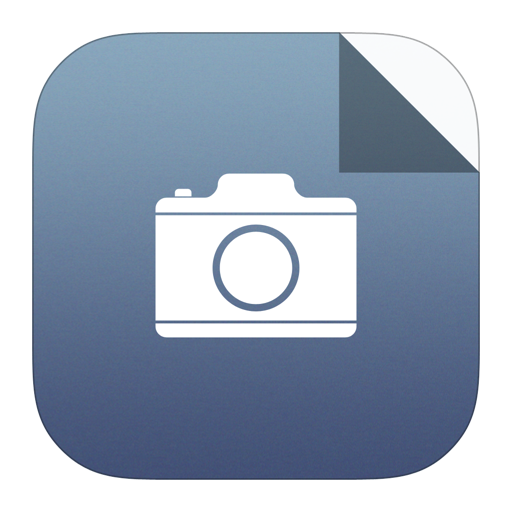
Larger image
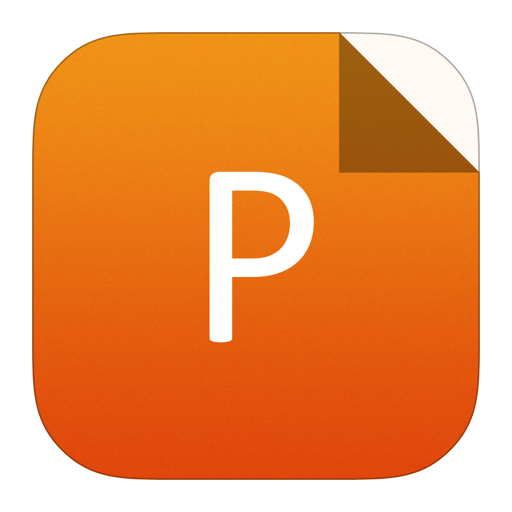
PowerPoint slide
Figure17.
Structure of recessed channel MOSFET with transparent gate.
The length of the channel (Lg) is 30 nm, width of the device is 200 nm, depth of the groove is 38 nm, junction depth of source and drain is 30 nm, Negative junction depth (NJD) is 10 nm, doping of the substrate (Na) is 1 × 1016 cm–3, doping of source and drain (Nd) is 1 × 1019 cm–3, thickness of physical oxide (tox) is 2 nm. SiO2 permittivity εox is 3.9, gate to source voltage (Vgs) is 0.7 V, drain to source voltage (Vds) 0.5 V, TGRC-MOSFET work function for (ΦITO) is 4.7 eV, CRC-MOSFET work function (ΦM) is 4.2 eV. Mishra et al.[31] proposed a structure of junctionless transistor-based 6-T SRAM cell (Fig. 18) using silicon on inductor. This structure reduces the area of the devices and increases performance. There is increase in the ION current and decrease in IOFF compared to double-gate junctionless. This structure occupies only half the area of the conventional structure. Read and write operation is also improved using the proposed structure. The ratio of ION/IOFF is 106. The area of the of junctionless transistor-based 6-T SRAM cell using silicon on inductor is 6.9 μm2 and that of conventional structure is 11.3 μm2.

class="figure_img" id="Figure18"/>
Download
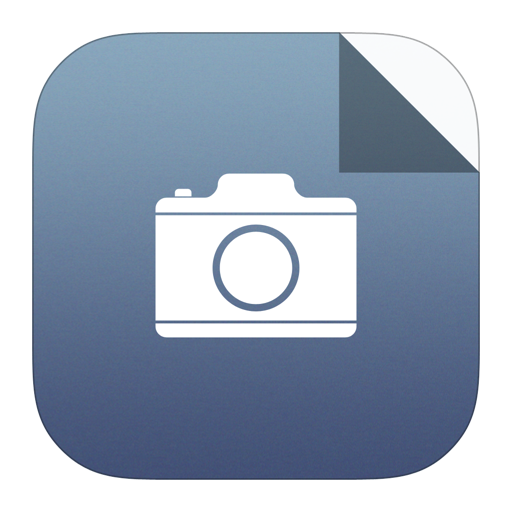
Larger image
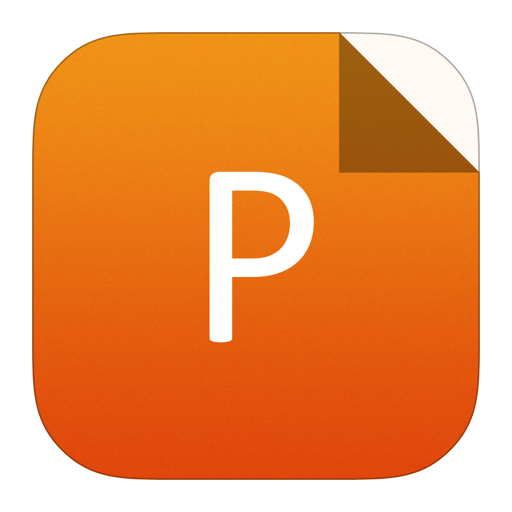
PowerPoint slide
Figure18.
(Color online) Proposed 6-T SRAM cell using junctionless SOI transistor with the connection.
The dimensions of the structure is gate length 18 nm, Tox 1 nm, Tsi channel thickness 10 nm, substrate thickness 10 nm, doping density in substrate regions 1 × 1018 cm–3, doping density channel 1 × 1018 cm–3, work function of gate material 4.9 eV.
Roy et al.[32] proposed a short channel junctionless double-gate MOSFET (Fig. 19). This structure does not contain any p-n junction because the doping of the channel is same as that of the drain and source. The RF and analog performance of the structure has been investigated. The voltage of the front and back gate provided are same. The DIBL value obtained is 75.98 mV/V, the sub-threshold slope is 62.32 mV/decade and the ION and IOFF ratio obtained is 4.86 × 109. From the result it is observed that sub-threshold slope is decreased by 1.61%, the ION and IOFF ratio is increased by 17.08% and DIBL is decreased by 4.52%. The dimensions of the device are gate work function is 5.2 eV, front gate oxide thickness is 1 nm, back gate thickness is 1 nm, silicon substrate thickness 5 nm, doping concentration ND is 1019 to 4 × 1019 cm–3, channel length L is 20 nm.

class="figure_img" id="Figure19"/>
Download
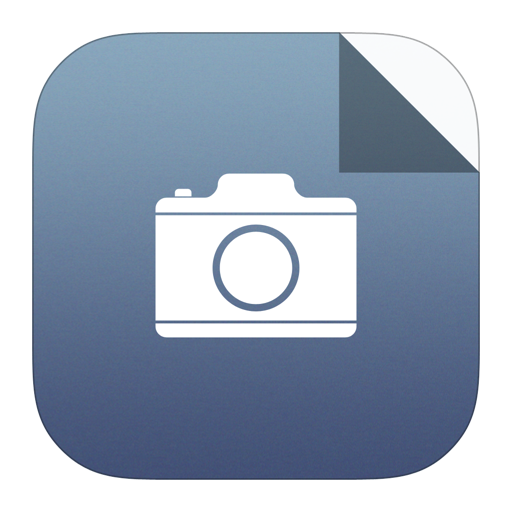
Larger image
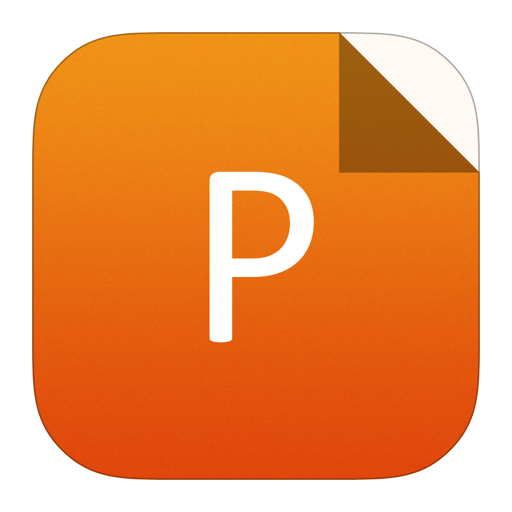
PowerPoint slide
Figure19.
(Color online) Structure of n-type junctionless double gate MOSFET.
Saramekala et al.[33] proposed a dual metal gate with a short channel having recessed source and drain silicon on insulator MOSFET (Fig. 20). This device provides high ON current, low DIBL value. The channel region is lightly doped and the drain and source region is heavily doped.

class="figure_img" id="Figure20"/>
Download
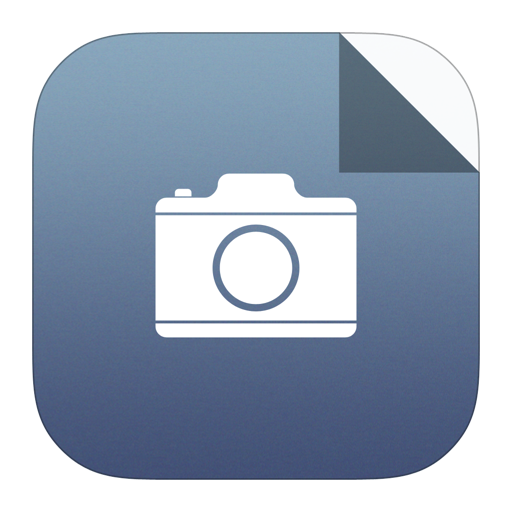
Larger image
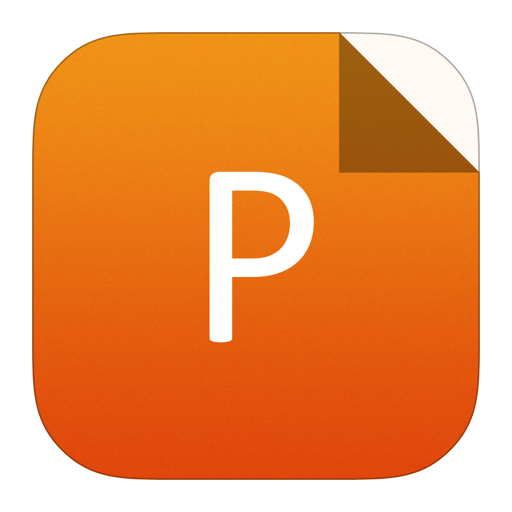
PowerPoint slide
Figure20.
(Color online) Dual metal gate (DMG) with recessed source and drain UTB SOI MOSFET.
Work-function of control gate (ΦM1) is 4.8 eV (gold), work-function of screen gate (ΦM2) is 4.6 eV (molybdenum), doping of the channel (Na) is 1015 cm–3, doping of source and drain (Nd) is 1020 cm–3, doping of substrate (Nsub) is 1015 cm–3, oxide thickness of channel (tox) is 1.5–4 nm, thickness of buried (tbox) is 100–300 nm, thickness of recessed is (trsd) 30–100 nm, length of recessed (dbox) is 3 nm, length of the channel (L) is 30–300 nm.
4.
Performance comparison and discussion
The performance comparison of different double gate MOSFET has been shown in Table 1 for sub 20 nm technology node including their applications. Since multiple gate MOSFETs have more control of gate over the channel, therefore different DG MOSFET have been considered for performance comparison. Metal gates with high work function are suitable for low OFF-state leakage. The underlap asymmetrical gate increases fringing electric field and leads to better ON-state transistor performance. The absence of depletion region between source/channel and drain in junction-less transistor, improves transistor current drive capability by increasing ON-state current. The high-K dielectric material is preferred as oxide region under gate to improve subthreshold performance parameters and improved switching behavior of transistor. The comparison 20 nm junctionless double gate (JLDG) MOSFET[32] provides the lowest subthreshold slope and maximum ION/IOFF ratio. The lowest DIBL obtained from graded channel dual material gate junctionless (GC-DMGJL) with channel length 15 nm[22]. Therefore, multi-gate juntionless transistors with low leakage and good ION/IOFF ratio can be preferred as low power digital and memory applications. Several researchers have explored bio-sensing ability of double gate MOSFET by including nano-gap cavity region. The dielectric constant of these cavity regions depends on changes occurred in bio-species that adds to a very interesting feature to the biomedical applications of these devices[12, 23, 34].
Parameter | ION (A/μm) | IOFF (A/μm) | ION/IOFF | SS (mV/dec) | DIBL (mV/V) | Channel length (nm) | Application |
(GC-DMGJL) MOSFET[22] | 7.695 × 10–4 | 3.741 × 10–10 | 2.057 × 106 | 73.42 | 21 | 15 | Analog circuit |
AG-JL MOSFET[28] | 127 × 10–4 | 1 × 10–15 | 1.27 × 105 | 68 | 65 | 20 | Digital circuit |
(DMSG) MOSFET[21] | – | 1.053 × 10–16 | – | 64.7978 | – | 10 | Digital circuit |
JLDG[32] | – | – | 4.86 × 109 | 62.32 | 75.98 | 20 | Analog/RF |
DGJL MOSFET[27] | – | – | 4.03 × 109 | 63.34 | 79.58 | 20 | Analog/RF |
Table1.
Comparison of performance parameters of different MOSFET structures.
Table options
-->

Download as CSV
Parameter | ION (A/μm) | IOFF (A/μm) | ION/IOFF | SS (mV/dec) | DIBL (mV/V) | Channel length (nm) | Application |
(GC-DMGJL) MOSFET[22] | 7.695 × 10–4 | 3.741 × 10–10 | 2.057 × 106 | 73.42 | 21 | 15 | Analog circuit |
AG-JL MOSFET[28] | 127 × 10–4 | 1 × 10–15 | 1.27 × 105 | 68 | 65 | 20 | Digital circuit |
(DMSG) MOSFET[21] | – | 1.053 × 10–16 | – | 64.7978 | – | 10 | Digital circuit |
JLDG[32] | – | – | 4.86 × 109 | 62.32 | 75.98 | 20 | Analog/RF |
DGJL MOSFET[27] | – | – | 4.03 × 109 | 63.34 | 79.58 | 20 | Analog/RF |
5.
Conclusion
Various structures of MOSFET have been explored with their structural details and dimensions including applications. Modification in the structure of MOSFET has been done mainly to reduce the short channel effects that include DIBL and SS values. The main motive of these structures is to increase the ON-state current and reduce the OFF-state current. The MOSFET structures are also analyzed for suitable analog/RF performance parameters to obtain a desired range of transconductance, transistor gain, stability factor and critical frequencies. The comparisons between different structures are done on the basis of subthreshold and analog/RF performance parameters. As per comparison made, the junctionless double gate (JLDG) MOSFET provides the lowest subthreshold slope and maximum ION/IOFF ratio for channel length 20 nm. The lowest DIBL obtained from graded channel dual material gate junctionless with channel length 15 nm. Gate engineered transistors of high work function metal contact with various high-K dielectric regions are found suitable to obtain improved subthreshold performance. This shows that gate-engineered multi-gate juntionless MOSFET has good potential to meet future scaling trends with increased compatibility in CMOS technology for any digital/analog and portable IoT or biomedical applications.