1.
Introduction
The development of power electronics technology is closely related to the performance of power devices. The emergence of new-generation power devices and power integrated circuits has greatly improved the efficiency and power density of power electronic systems[1]. However, plenty of scientific and technical problems of power semiconductor electronics have to be settled urgently due to continuous development requirements including high operation voltage, large current density, fast switching speed, low energy loss etc.[2]. The range of power semiconductor has been extended from silicon to wide bandgap semiconductor (such as SiC, GaN, etc.), recently to ultra-wide bandgap semiconductor (such as Ga2O3, diamond, etc.)[3–6]. New semiconductor materials provide new development opportunities for power electronics technology.
As a promising ultra-wide band gap semiconductor, Ga2O3 has an enormous potential for the next-generation power electronic application due to its large bandgap (~4.8 eV), high breakdown field (8 MV/cm), adjustable n-type doping concentration (1015–1019 cm?3) and availability of high quality large area native substrate from low cost melt-based growth methods[7–10]. As shown in Table 1, owing to its advantages, the Ga2O3 material is very suitable for fabricating high power devices, including Schottky barrier diode (SBD) and field effect transistor (FET)[11–20]. In this case, there is an increasing number of papers that report the substrate and epitaxial growth technology, doping and defect properties, and devices fabrication in the last several years.
Semiconductor material | Si | GaN | 4H-SiC | β-Ga2O3 |
Bandgap Eg (eV) | 1.1 | 3.4 | 3.3 | 4.7–4.9 |
Electron mobility μ (cm2·V?1·s?1) | 1400 | 1200 | 1000 | 300 |
Breakdown electric field Eb (MV/cm) | 0.3 | 3.3 | 2.5 | 8 |
Baliga’s FOM (εμEb3) | 1 | 870 | 340 | 3444 |
Thermal conductivity λ (W·cm?1·K?1) | 1.5 | 2.1 | 2.7 | 0.11 |
Table1.
Comparison of the physical properties of Si, GaN, SiC and β-Ga2O3 semiconductor[6].
Table options
-->

Download as CSV
Semiconductor material | Si | GaN | 4H-SiC | β-Ga2O3 |
Bandgap Eg (eV) | 1.1 | 3.4 | 3.3 | 4.7–4.9 |
Electron mobility μ (cm2·V?1·s?1) | 1400 | 1200 | 1000 | 300 |
Breakdown electric field Eb (MV/cm) | 0.3 | 3.3 | 2.5 | 8 |
Baliga’s FOM (εμEb3) | 1 | 870 | 340 | 3444 |
Thermal conductivity λ (W·cm?1·K?1) | 1.5 | 2.1 | 2.7 | 0.11 |
As well-known, field effect transistor is a significant component of power electronics for high voltage and high frequency applications, such as inverters, amplifiers and power switches. In this paper, we firstly review the physical properties of Ga2O3 semiconductor, and then summarize the recent development of Ga2O3 based FETs through comparing the device performances including breakdown voltage (Vbr), transconductance (gm), maximum current density (Jmax) and on/off ratio, as well as device structures. Though the investigation on Ga2O3 based FET is still in the early stage, the material potential has been preliminarily revealed. Therefore, an overview is beneficial for the further development of Ga2O3 based power electronics.
2.
Basic properties of gallium oxide semiconductor material
Though Ga2O3 single crystal growth technology is developed recently, its compound has a relatively long research history. As early as 1952, the investigation on the phase equilibria in the Al2O3-Ga2O3-H2O system was carried out and various polymorphs were determined by Roy et al.[21]. In 1965, Tippins et al. observed the optical absorption and photoconductivity of β-Ga2O3 and confirmed its band gap of 4.7 eV[22], which is in good agreement with recent absorption and angle-resolved photoemission spectroscopy measurements[23–25]. Since the 1990s, plenty of bulk growth and epitaxy growth technologies of single crystal Ga2O3 have been developed, especially in last five years, an increasing number of researchers paying their attention on ultra-wide bandgap Ga2O3.
In fact, there have been five phases of Ga2O3, all of which can exist stably under normal condition and will convert into other phases at high pressures or high temperatures, as shown in Fig. 1[21]. From this figure, we can observe that the β phase is the most stable one and other phases will translate into it under high temperature and high moisture. The ε phase is the second-most stable phase of Ga2O3[26, 27], and this phase will keep it steady until 870 °C. The bandgap of ε-Ga2O3 is also approximately 4.9 eV, and it has the same hexagonal structure as the common wide bandgap semiconductors GaN and SiC[28, 29]. The α phase is the third stable single crystal of Ga2O3, and its highest stable temperature is 600 °C under dry condition, which drops to 300 °C under wet condition. The α phase have the same corundum crystal structure as α-Al2O3, leading to the possibility of epitaxial growth of high quality Ga2O3 layer on sapphires. In addition, the melting point of β-Ga2O3 is about 1793 °C[30]. Therefore, only β-Ga2O3 can be grown through melting methods, while others not due to phase transition, which is the major reason for the rapid development of β-Ga2O3. What’s more, the β phase has the lowest volume expansion and bulk moduli through theoretical calculation[31]. In this paper, we mainly review the research progress on β-Ga2O3 and its power FET devices.

class="figure_img" id="Figure1"/>
Download
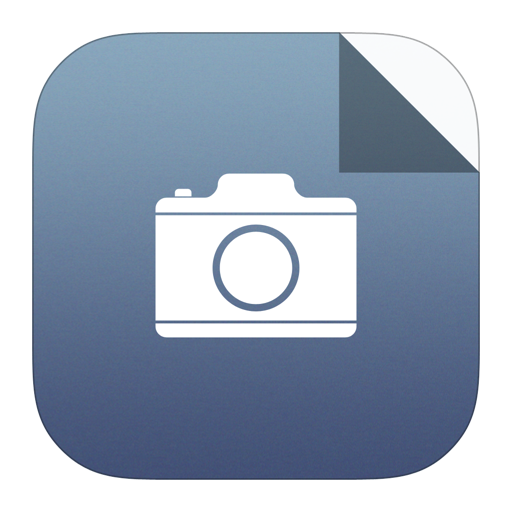
Larger image
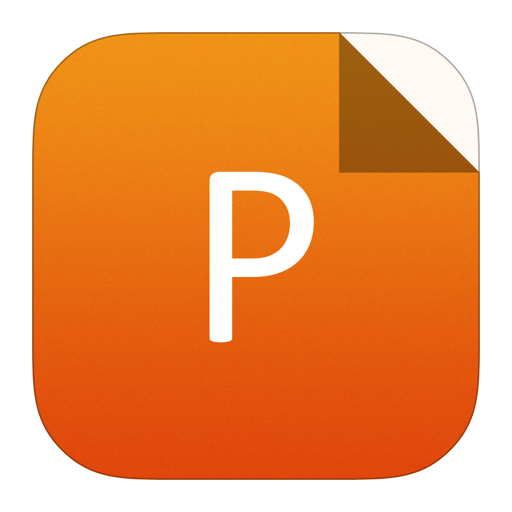
PowerPoint slide
Figure1.
(Color online) Transformation relationships among Ga2O3 in different crystalline phases and their hydrates[21].
β-Ga2O3 belongs to monoclinic system, C2/m space group, and its lattice constants are a = 1.22 nm, b = 0.3 nm, c = 0.58 nm, and β = 103.83°. The lattice constant value in the direction of (100) is much larger than those in (001) and (010), which means that it is easy to exfoliate the ultra-thin film from its (100) direction for device preparation. For the crystal structure, the double chain composed of [GaO6] octahedron is arranged along the b axis, and the chains are connected by [GaO4] tetrahedron. Ga atoms exists in the crystal in two kinds of forms of four and six ligands, as shown in Fig. 2[32]. The crystalline structure of β-Ga2O3 is helpful for the movement of free carriers. However, the conductivity of Ga2O3 is limited by its ultra-wide bandgap (4.7–4.9 eV). In fact, the intrinsic electrical conduction of β-Ga2O3 originates from the free electrons led by the point defects in the crystal, which is the same as ZnO[33]. As a kind of oxide crystal, oxygen vacancies are pervasive in β-Ga2O3, and plenty of theoretical and experimental studies indicate that the oxygen vacancies play the key role in the electrical conduction[34–36]. It’s worth mentioning that it is the oxygen vacancies in Ga2O3 crystal that drive many researches on gas sensors, because they can easily adsorb certain gases, such as H2, CH4, CO, O2.etc, and will change the resistivity upon adsorption[37–40].

class="figure_img" id="Figure2"/>
Download
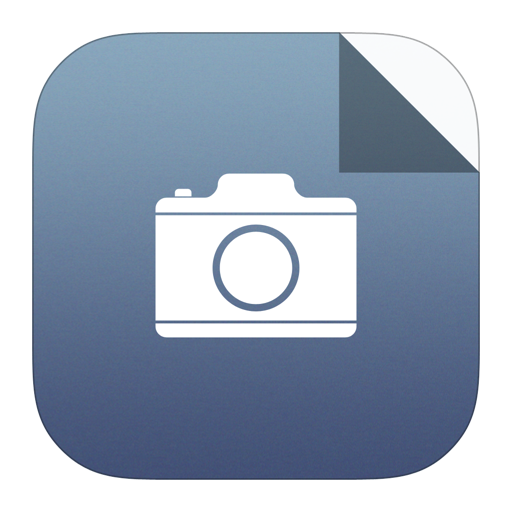
Larger image
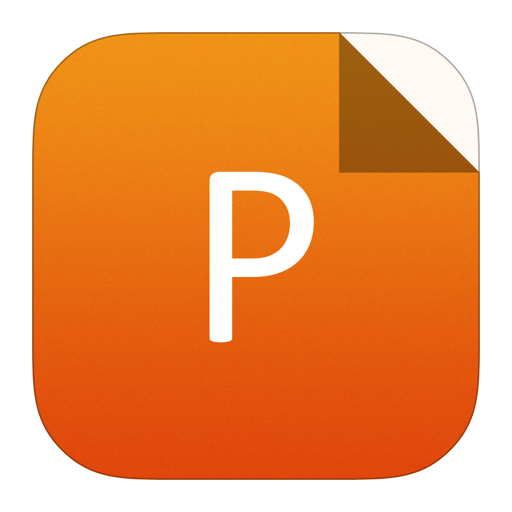
PowerPoint slide
Figure2.
(Color online) Crystal structure of β-Ga2O3[32].
β-Ga2O3 has a large bandgap of approximately 4.8 eV and corresponding large estimated critical electric field strength of about 8 MV/cm, about twice that of SiC and GaN. The large bandgap of β-Ga2O3 allows its high temperature operation and the large critical field can sustain high voltage operation. However, the poor thermal conductivity of 0.1–0.3 W·cm?1·K?1 will limit high temperature capability of Ga2O3 based power devices and thermal management will be especially significant[41–43]. In order to assess the appropriateness of various semiconductors for power electronics application, there are different figures-of-merit (FOM), such as Baliaga’s figure of merit (BFOM) for dc conduction losses, Huang’s material figure of merit (HMFOM) for dynamic switching losses, etc, most of which involve breakdown field strength[44, 45]. Through calculation, we find that the BFOM for β-Ga2O3 is four times larger than GaN and ten times larger than SiC. Except for its high voltage capability, β-Ga2O3 has a predicted high theoretical electron velocity of 2 × 107 cm/s and a measured mobility of 100 cm2/(V·s)[46, 47]. These properties are beneficial for low power under high frequency switching in the radio frequency regime, where its power density would be limited by the low thermal conductivity.
As the same as other wide bandgap materials, it is significant for β-Ga2O3 to control its electrical conductivity by doping or reduce defects in crystal. The n-type doping technology has been widely used in substrate, and epitaxial layer growth. Ion implantation has been used to reduce the contact resistance. Several common n-type dopants, such as Si, Ge and Sn, are proved to have low activation energy and can be effectively activated at room temperature. In addition, doping concentration can be flexibly modulated in the range of 1015–1019 cm?3, with the highest value of 1020 cm?3 reported[48]. As for p-type doping, similar to other wide-band semiconductors, it is difficult to obtain low activation energy of acceptors in β-Ga2O3. Though Na, Mg, Ca, Cu, Ag, Zn, Cd, etc are likely to be potential candidates for usable p-type dopants[49], there still have been no breakthrough reported. In addition, the results from theoretical prediction and calculation indicate that the electron effective mass is 0.34m0 (here m0 is the free electron mass), while the hole effective mass is rather large due to local lattice distortions[50, 51].
Additionally, it’s worth noting that the future development of β-Ga2O3 needs to overcome the following two difficulties. (1) Develop effective p-type doping process, which determines the destiny of β-Ga2O3 based power electronics to some extent. It’s well-known that carrier inversion is the unique characteristic of semiconductor and reverse p-n junction can effectively undertake high voltage to give full play to the material advantages of β-Ga2O3. (2) Severe self-heating effects caused by the relatively low thermal conductivity of β-Ga2O3 must be mitigated in high voltage and high frequency application, though ultra-wide bandgap of β-Ga2O3 improve electronics thermal tolerance. What’s more, the mobility of carrier will also be reduced due to anabatic scatterings[46], and will lower switch speed.
3.
Field effect transistor based on β-Ga2O3
Summing up the above discussion, the physical properties suggest that β-Ga2O3 has a broad prospect in high voltage and high frequency power devices. At present, due to the absence of p-type doping, researchers focus on two kinds of unipolar devices, i.e. Schottky barrier diode (SBD) and field effect transistor (FET). FET is the conventional power switch device in which the resistance between source and drain electrodes is modulated by gate electrical field, and it is mainly used in converters and inverters.
The researching process of β-Ga2O3 based field effect transistors is shown in Fig. 3. Transistors develop from MESFET[12, 52] to planar MOSFET, then to well-performance devices with termination structure as well as vertical ones. Most early transistors work in depletion mode due to the absence of inversion layer in β-Ga2O3. Afterwards, researchers take advantage of depletion layer from interface states to realize enhancement mode devices. Recently, vertical transistor with a breakdown voltage of 1 kV have been reported[14]. Some typical works on transistors will be introduced in the following parts.

class="figure_img" id="Figure3"/>
Download
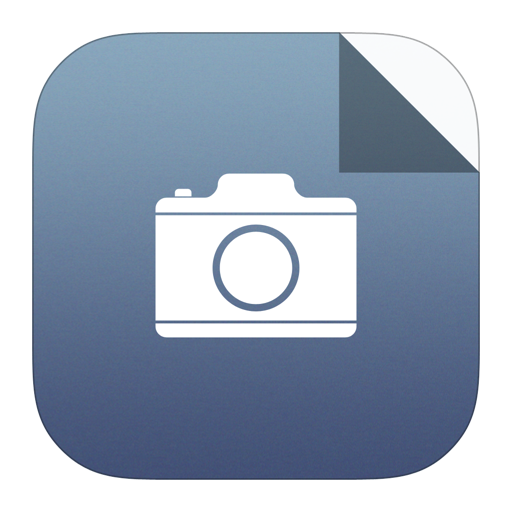
Larger image
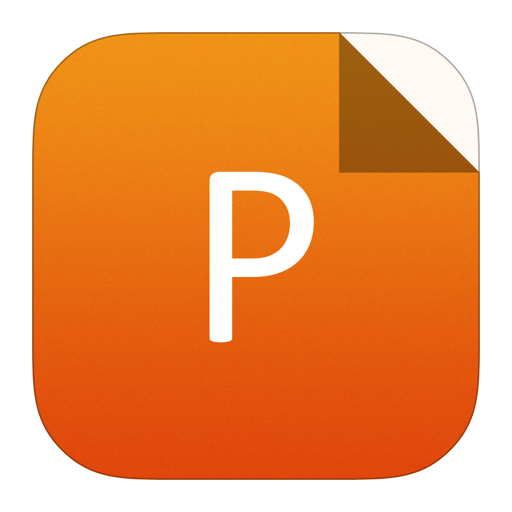
PowerPoint slide
Figure3.
(Color online) The development of β-Ga2O3 transistor in recent years.
In 2012, Higashiwaki et al. (from the National Institute of Information and Communications Technology (NICT)) manufactured a metal semiconductor field effect transistor (MESFET) on molecular-beam (MBE) epitaxial layer with Sn doping (7.0 × 1017 cm?3) on (010) oriental β-Ga2O3, as shown in Fig. 4(a)[12]. This transistor’s breakdown voltage reaches 250 V with Pt/Ga2O3 Schottky barrier as gate structure, and serious off-state leakage current of 3 μA, leading to an on/off ratio of 104. Therefore, in 2013, 20 nm Al2O3 is used as the gate dielectrics to solve this problem, as Fig. 4(b) shows [11], and the source and drain regions are highly doped (5.0 × 1019 cm?3) by Si to improve Ohmic contact. The device doesn’t breakdown until 370 V, and the on/off ratio reaches 1010.

class="figure_img" id="Figure4"/>
Download
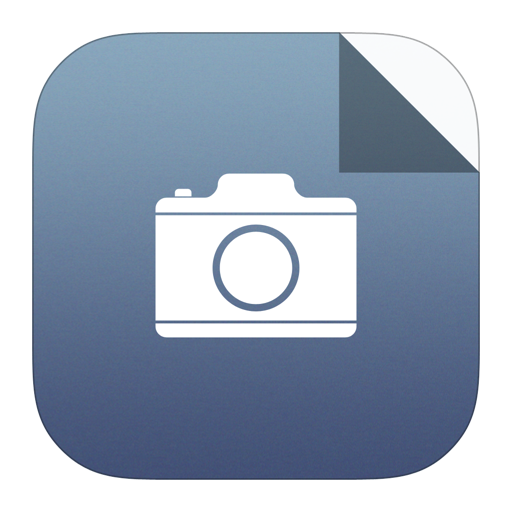
Larger image
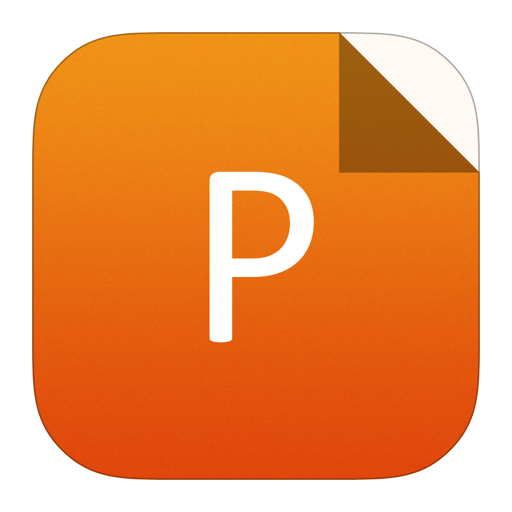
PowerPoint slide
Figure4.
(Color online) Schematic cross-section of β-Ga2O3 (a) MESFET[12] and (b) MOSFET[11].
Depletion-mode MOSFET with Vbr of 750 V was fabricated on MBE epitaxial (010) β-Ga2O3 layer with Sn doping of 3.0 × 1017 cm?3 by Wong et al. (from Novel Crystal Technology Inc.) in 2016, as shown in Fig. 5(a)[16]. The highly resistive unintentionally-doped (UID) Ga2O3 was implemented to isolate the planar devices successfully. Fig. 5(b) shows that the off-state current primarily comes from drain leakage current with gate leakage being 1–2 orders of magnitude lower. The on/off ratio decreases from 109 to 103 with rising temperature, as shown in Fig. 5(c), which indicates the transistor can operate stably against thermal stress up to at least 300 °C. In addition, current collapse phenomenon caused by self-heating effect is observed in output curves measured in DC and pulse condition as shown in Fig. 5(d). Therefore, heat management is significant for devices on Ga2O3 with relatively low thermal conductivity. The characterization of channel temperature and radiation hardness against gamma ray irradiation of similar devices were also tested and analyzed[53, 54].

class="figure_img" id="Figure5"/>
Download
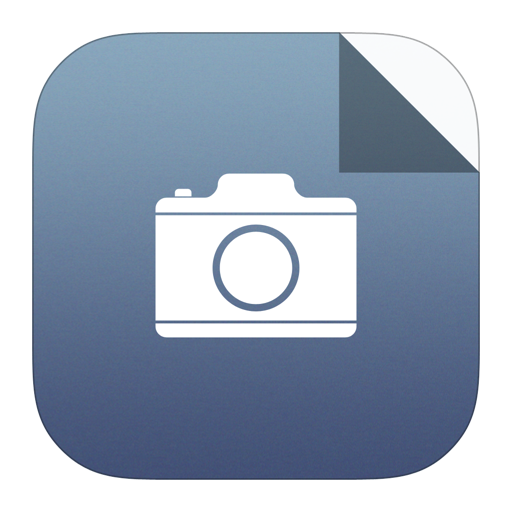
Larger image
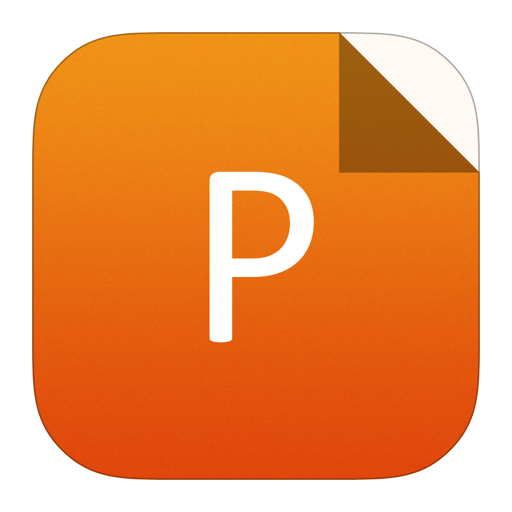
PowerPoint slide
Figure5.
(Color online) (a) Schematic cross-section, (b) the off-state drain/gate leakage and breakdown curves, (c) temperature-dependent transfer characteristics at Vds = 30 V, and (d) DC and pulsed output curves of the β-Ga2O3 FP-MOSFET[16].
In 2016, Green et al. (from Wright-Patterson Air Force Base of USA) fabricated a depletion mode transistor on Sn doping (1.7 × 1018 cm?3) epitaxial layer of (100) oriental β-Ga2O3, which was grown via metal-organic vapor phase epitaxy (MOVPE). The transistor has a tremendous breakdown voltage of 230 V with a small gate-drain distance of 0.6 μm[55]. The experimentally measured breakdown electrical field of 3.8 MV/cm is the highest reported value for Ga2O3 transistors, surpassing SiC and GaN theoretical limits, though this value is far smaller than Ga2O3’s theoretical value of 8 MV/cm. The representative two-finger structure is adopted, as shown in Fig. 6. This achievement strongly inspired the researchers to make further effort to exploit the advantages of Ga2O3.

class="figure_img" id="Figure6"/>
Download
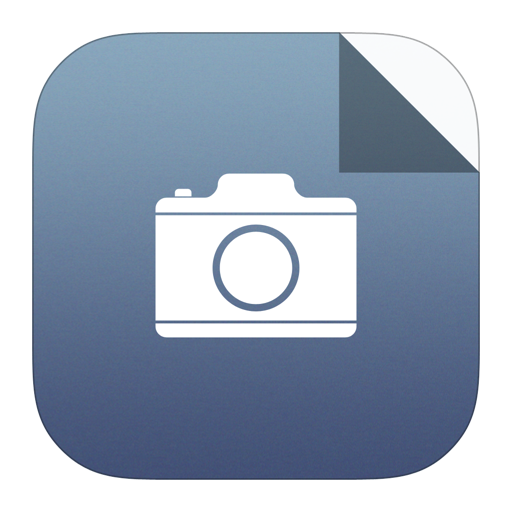
Larger image
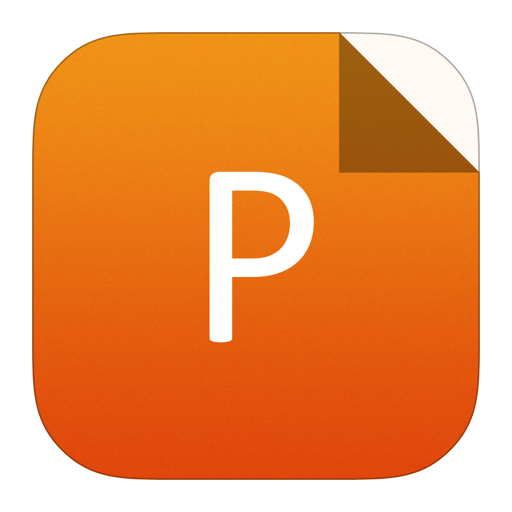
PowerPoint slide
Figure6.
Top–down SEM image of the two-finger MOSFET on (100) β-Ga2O3[55].
Though depletion-mode transistors show an excellent performance, including high current density, high breakdown voltage, low on-resistance etc, amounts of researches on enhancement-mode transistors have been also reported due to its unique advantages in application.
In 2016, a wrap-gate fin field-effect transistor operated on enhancement-mode was fabricated on (100) β-Ga2O3 by Chabak et al. (from Air Force Research Laboratory of USA). Fin array channels are beneficial for depletion by interface states to turn off without gate bias, but on current is limited to ~1 mA/mm[15]. The device achieved a three-terminal breakdown voltage exceeding 600 V with 21 μm gate-drain spacing. In December 2017, they reported another recessed-gate enhancement-mode β-Ga2O3 MOSFETs, as shown in Fig. 7(a). In this device, the gate recess is self-aligned to the gate region, so the partially removed region is fully depleted by interface state and the channel for normally-off operation is formed. As a result, the on current is promoted to 40 mA/mm with on/off ratio of ~109 and Vbr of 505 V. From the breakdown current curves in Fig. 7(b), it can be observed that the breakdown performance is limited by gate dielectric failure.

class="figure_img" id="Figure7"/>
Download
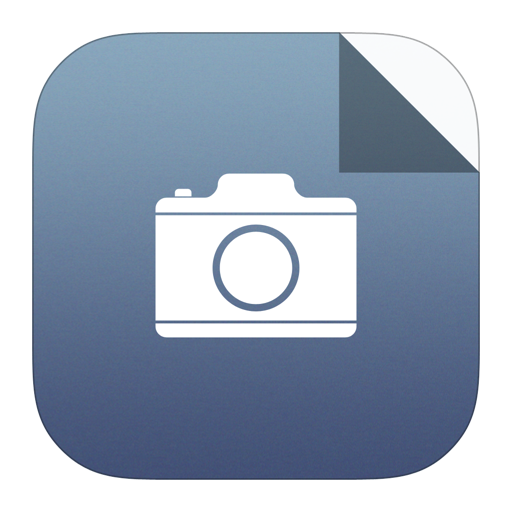
Larger image
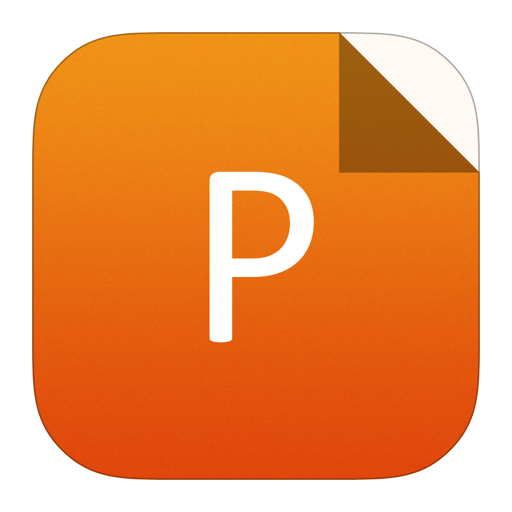
PowerPoint slide
Figure7.
(Color online) (a) SEM false-colored cross-section view of recessed-gate MOSFETs and HR-TEM of (b) its sidewall and (c) bottom facets of the gate-recess contact, (d) its gate-source and drain-source breakdown curves of both source-drain distances[15].
In March 2017, Wong et al. (from NICT) took advantage of fully depleted unintentionally doping (010) β-Ga2O3 (with a background carrier concentration of 4 × 1014 cm?3) to realize transistor’s operation in enhancement mode[56]. In addition, box-like ion implantation of 5 × 1019 cm?3 was applied to decrease contact resistance in source and drain regions. As a consequence, transistors have a maximum saturation current of 1.4 mA/mm when Vgs = 38 V and Vds = 15 V, and on/off ratio near 106. The peak extrinsic transconductance of 0.38 mS/mm is imputed to charge trapping of unoptimized ALD interface.
From the above, we can conclude that effective p-type doping is very significant for investigation of transistors. Without p–n junction structure, the thick gate dielectric is required to undertake too high voltage to excavate the excellent property of Ga2O3. In order to enhance breakdown voltage, vertical field effect transistor is a promising option.
In 2017, Wong et al. (from NICT) fabricated a vertical β-Ga2O3 MOSFET, and a buried current blocking layer (CBL) was used to electrically isolate the source and drain electrodes[57]. However, the device has a large source-drain leakage current due to the difficulty in activating p-type dopants in CBL.
In 2017, Hu et al. (from Cornell University) attempted to fabricate vertical fin transistor, and though the device has an on/off ratio of 109, the field crowding near the bottom of the column limited Vbr to 185 V[58]. Whereafter, in June 2018, they reported a high-voltage (over 1 kV) vertical β-Ga2O3 MISFET, distinguished from conventional p-n junction based MOSFET due to no p-region in this device. As shown in Fig. 8(a)[14], the MISFET was fabricated on halide vapor phase epitaxial (HVPE) layers with low charge concentration of ~1016 cm?3 on bulk β-Ga2O3 (001) substrates, and has a nano bar structure to realize enhancement operation with a threshold voltage of ~1.2–2.2 V, and a current on/off ratio of ~108, which demonstrate the well gate modulation of transistor. In addition, an on-resistance of ~13–18 mΩ·cm2 and an output current of over 300 A/cm2 were also extracted. Fig. 8(b) shows the three-terminal off-state breakdown curves and indicates that both the drain and gate leakage currents remain low until the hard breakdown near 1057 V. Field plate and ion implantation edge termination techniques are expected to further increase the breakdown voltage. To sum up, this is the first high-breakdown voltage vertical transistor reported and is a milestone for the application of β-Ga2O3 based power electronics.

class="figure_img" id="Figure8"/>
Download
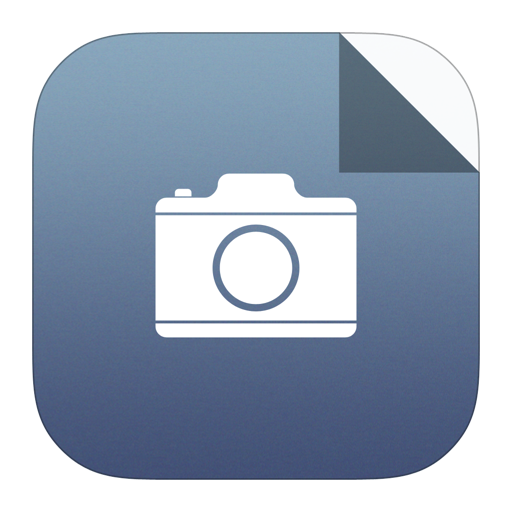
Larger image
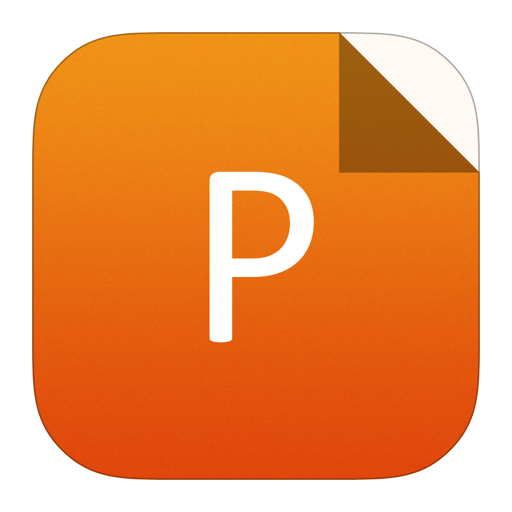
PowerPoint slide
Figure8.
(Color online) (a) Schematic cross-section and SEM image, and (b) three-terminal off-state breakdown curves of vertical β-Ga2O3 Fin-MISFET[14].
In addition to high voltage, high theoretical electron velocity and high breakdown electrical field make β-Ga2O3 a potentially useful material in radio frequency (RF) and mm-wave region, and its power density at high frequency would not be limited by the low thermal conductivity. Therefore, some works in this direction have been reported in succession.
In 2017, Green et al. (from the Wright-Patterson Air Force Base of USA) used a highly doped cap layer to decrease contact resistance, and an incompletely depleted sub-micro gate recess to form modulation channel and attained a RF Ga2O3 transistor, as shown in Figs. 9(a) and 9(b)[59]. A transconductance (gm) of 21 mS/mm, extrinsic cutoff frequency (fT) of 3.3 GHz, and maximum oscillating frequency (fmax) of 12.9 GHz are measured experimentally, as shown in Fig. 9(c). With a passive source and load tuning at 800 MHz, RF performances including Pout of 0.23 W/mm, power gain of 5.1 dB, and power-added efficiency of 6.3%, were confirmed in detail. All results preliminarily suggest that Ga2O3 has a potential in applications for power switch and RF electronics.

class="figure_img" id="Figure9"/>
Download
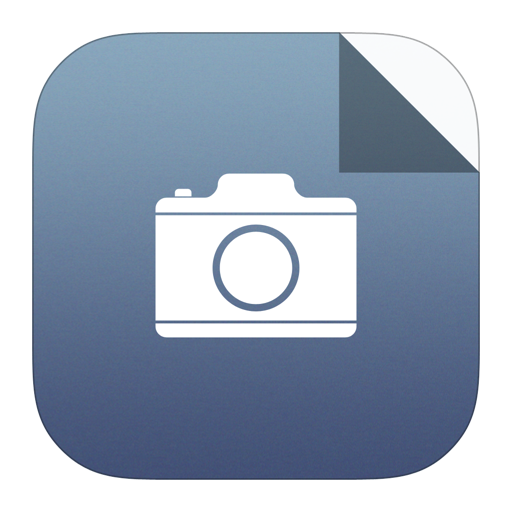
Larger image
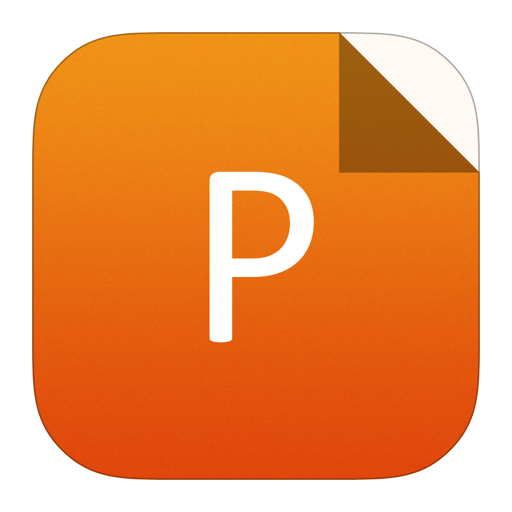
PowerPoint slide
Figure9.
(Color online) (a) Cross section schematic, (b) focused ion beam (FIB) cross sectional image, and (c) extrinsic small signal RF gain performance of RF β-Ga2O3 MOSFET[59].
The Ohio State University devoted themselves to utilize 2-dimensinal electron gas from silicon delta-doped β-Ga2O3 to fabricate high frequency transistors. In 2017, a delta-doping β-Ga2O3 MOSFET with integrated sheet charge of 2.4 × 1017 cm?2, mobility of 83 cm2V?1s?1, was manufactured by Krishnamoorthy et al., and drain current Imax of 236 mA/mm and transconducance gm of 26 mS/mm were obtained[60]. In 2018, Xia et al. from the same department fabricated a silicon delta-doped MESFETS with low regrown source/drain contact resistance and UID Ga2O3 as gate insulator, as shown in Fig. 10(a)[61]. The turn-off voltage of 4 V, a peak drain current of 140 mA/mm, gm of 34 mS/mm and Vbr of 170 V are obtained. Fig. 10(b) shows the correlation between mobility and 2-D electron gas density. The detailed scattering mechanisms that limit 2DEG mobility is required to further analyze theoretically. Though high frequency parameters weren’t reported in the literature, the results indicate that delta doping can be a potential process of the scaled Ga2O3 high frequency field effect transistors.

class="figure_img" id="Figure10"/>
Download
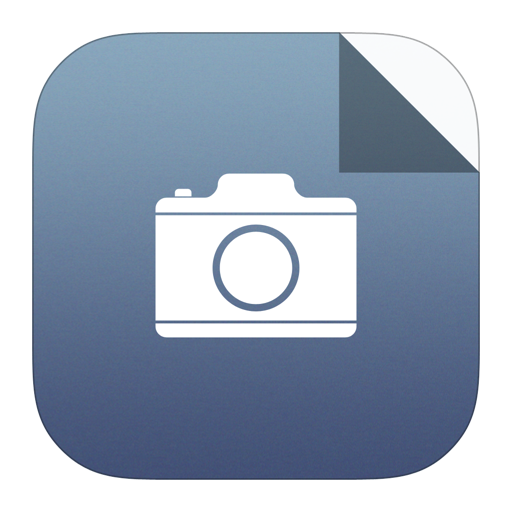
Larger image
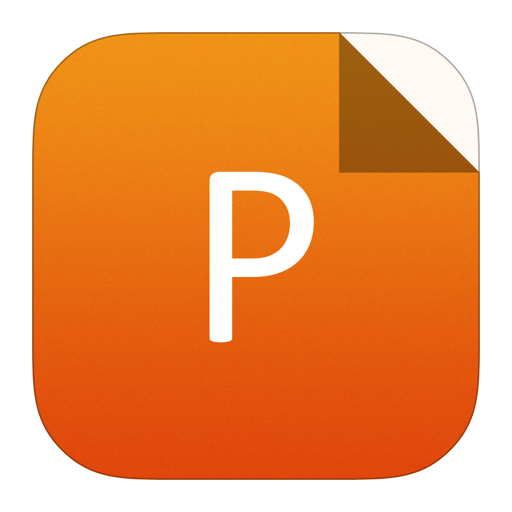
PowerPoint slide
Figure10.
(Color online) (a) Schematic and (b) density-dependent field effect mobility of Silicon delta-doped β-Ga2O3 MESFET.
In addition, as mentioned above, β-Ga2O3 belongs to monoclinic system with the lattice of a axis longer than other two ones, and nano membrane can be acquired mechanically the same as 2-D materials[13, 17, 62–65]. Therefore, plenty of film transistors are reported for power application. For instance, in January 2017, the back-gate transistor fabricated by Zhou et al. from Purdue University has a high record drain current of 600/450 mA/mm, a high on/off ratio of 1010 in depletion/enhancement modes, as shown in Fig. 11[17]. An average breakdown electric field of 2 MV/cm was realized in E-mode devices with a breakdown voltage of 185 V, which shows the great promise of FETs based on β-Ga2O3 on insulator for power electronics.

class="figure_img" id="Figure11"/>
Download
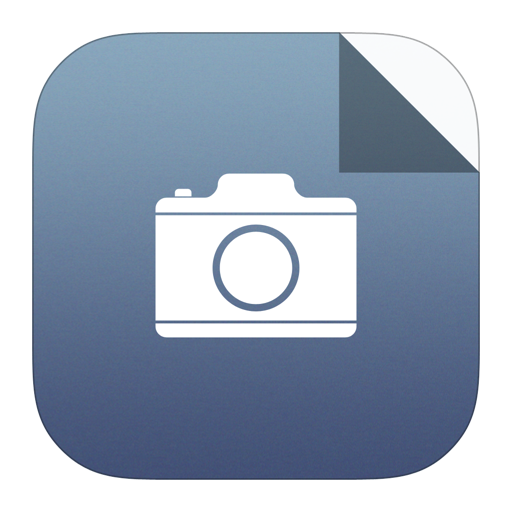
Larger image
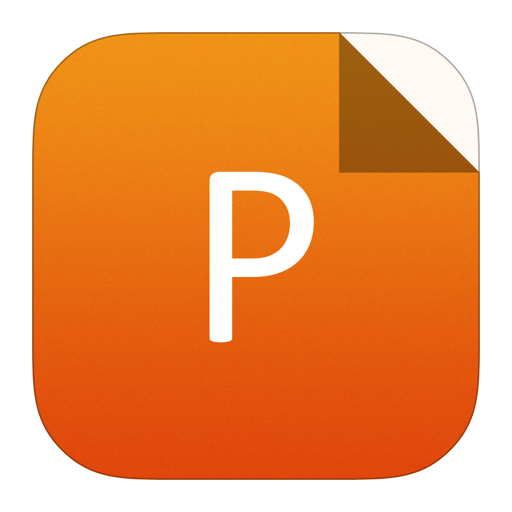
PowerPoint slide
Figure11.
(Color online) Depletion/enhancement-mode β-Ga2O3 on insulator (GOOI) FETs[17].
4.
Conclusion and future outlook
In conclusion, early researches on transistors mainly focus on realizing gate modulation and high breakdown voltage no matter planar and vertical structures. Up to now, the material properties of Ga2O3 have not been fully demonstrated experimentally, more investigations on p-type doping and device fabrications are in demand. In addition, low carrier mobility and self-heating effect are also research hotspots. There is no doubt that all efforts taken in FET will be beneficial for future improvement of Ga2O3 FET. Table 2 shows the basic performance parameters of some typical β-Ga2O3 based FETs reported up to now and apparently indicates their gradual improvement in performances. Based on the available achievements reported, we can summary that
Device type | Substrateorientation | Gate dielectrics | Vbr (V) | Jmax (mA/mm) | On/off ratio | gm (mS/mm) | Reference |
D-MESFET | (010) β-Ga2O3 | — | 250 | — | 104 | 1.4 | [12] |
D-MOSFET | (010) β-Ga2O3 | Al2O3 | 370 | 39 | 1010 | — | [11] |
E-Fin FET | (100) β-Ga2O3 | Al2O3 | 600 | - | 105 | — | [15] |
Two-finger D-MOSFET | (100) β-Ga2O3 | Al2O3 | 230 | 60 | 107 | 1.1 | [55] |
Field plate D-MOSFET | (010) β-Ga2O3 | Al2O3 | 750 | 78 | 109 | 3.4 | [16] |
Recessed-gate D-MOSFET | (100) β-Ga2O3 | Al2O3 | — | 150 | 106 | 21.2 | [59] |
E-MOSFET | (010) β-Ga2O3 | Al2O3 | — | 1.4 | 106 | 0.38 | [56] |
D-MOSFET | (010) β-Ga2O3 | HfO2 | 400 | 45 | 108 | — | [74] |
Vertical trench D-MOSFET | (001) β-Ga2O3 | HfO2 | — | — | 103 | — | [75] |
Vertical Fin D-MOSFET | (-201) β-Ga2O3 | Al2O3 | 185 | 1 kA/cm2 | 109 | — | [58] |
D-MOSFET | β-Ga2O3 | SiO2 | 382 | 40 | 108 | 1.23 | [76] |
Recessed-gate E-MOSFET | (010) β-Ga2O3 | SiO2 | 505 | 40 | 109 | 7 | [15] |
Vertical Fin E-MISFET | (001) β-Ga2O3 | Al2O3 | 1057 | 300–500 kA/cm2 | 108 | — | [14] |
Delta doped D-MOSFET | (010) β-Ga2O3 | — | 170 | 140 | 106 | 34 | [61] |
Table2.
Development of Ga2O3 FETs and the corresponding performances.
Table options
-->

Download as CSV
Device type | Substrateorientation | Gate dielectrics | Vbr (V) | Jmax (mA/mm) | On/off ratio | gm (mS/mm) | Reference |
D-MESFET | (010) β-Ga2O3 | — | 250 | — | 104 | 1.4 | [12] |
D-MOSFET | (010) β-Ga2O3 | Al2O3 | 370 | 39 | 1010 | — | [11] |
E-Fin FET | (100) β-Ga2O3 | Al2O3 | 600 | - | 105 | — | [15] |
Two-finger D-MOSFET | (100) β-Ga2O3 | Al2O3 | 230 | 60 | 107 | 1.1 | [55] |
Field plate D-MOSFET | (010) β-Ga2O3 | Al2O3 | 750 | 78 | 109 | 3.4 | [16] |
Recessed-gate D-MOSFET | (100) β-Ga2O3 | Al2O3 | — | 150 | 106 | 21.2 | [59] |
E-MOSFET | (010) β-Ga2O3 | Al2O3 | — | 1.4 | 106 | 0.38 | [56] |
D-MOSFET | (010) β-Ga2O3 | HfO2 | 400 | 45 | 108 | — | [74] |
Vertical trench D-MOSFET | (001) β-Ga2O3 | HfO2 | — | — | 103 | — | [75] |
Vertical Fin D-MOSFET | (-201) β-Ga2O3 | Al2O3 | 185 | 1 kA/cm2 | 109 | — | [58] |
D-MOSFET | β-Ga2O3 | SiO2 | 382 | 40 | 108 | 1.23 | [76] |
Recessed-gate E-MOSFET | (010) β-Ga2O3 | SiO2 | 505 | 40 | 109 | 7 | [15] |
Vertical Fin E-MISFET | (001) β-Ga2O3 | Al2O3 | 1057 | 300–500 kA/cm2 | 108 | — | [14] |
Delta doped D-MOSFET | (010) β-Ga2O3 | — | 170 | 140 | 106 | 34 | [61] |
(1) Al2O3 and SiO2 are widely used as gate insulators due to their larger conduction band offset with β-Ga2O3 and can effectively prevent gate leakage current. In addition, interfacial property in gate channel region affect the performance of FET, such as gate control ability and switch speed. Therefore, there are many studies focusing on evaluating and improving the interfacial property[66–72].
(2) High doping by ion implantation, spin-on-glass, or regrown layer are common methods to reduce contact resistance, and annealing can further improve Ohmic contact. Titanium is proved to be the best ohmic electrode metal directly contacting with β-Ga2O3[73].
(3) Depletion-mode transistors have been realized earlier. As for the enhancement-mode, either a low unintentional doping channel or a thin depletion layer by gate region interface states is proved to be effective solution when p-type doping is absent.
(4) The thermal damage should be unswervingly considered as the significant issue in β-Ga2O3 based power devices due to its relatively low thermal conductivity.
(5) The large theoretical breakdown electrical field of β-Ga2O3 can make a tradeoff between mobility and power loss during the high voltage and high frequency operation. In order to exert the advantages of β-Ga2O3, it’s necessary to scale down the gate length for high frequency application, and to develop vertical FETs for high voltage application.
?
Acknowledgements
This work was supported by the National Natural Science Foundation of China (Nos. 61521064, 61522408, 61574169, 61334007, 61474136, 61574166), the Ministry of Science and Technology of China (Nos. 2016YFA0201803, 2016YFA0203800, 2017YFB0405603), the Key Research Program of Frontier Sciences of Chinese Academy of Sciences (Nos. QYZDB-SSW-JSC048, QYZDY-SSW-JSC001), the Beijing Municipal Science and Technology Project (No. Z171100002017011), and the Opening Project of the Key Laboratory of Microelectronic Devices & Integration Technology, Institute of Microelectronics of Chinese Academy of Sciences