1.
Introduction
The discovery of conducting polymer (CP) in the 19th century was one of the turning points in the scientific world due to its metal-polymer combined properties and wide range of applicability. Currently CP are used in sensors[1, 2], membranes[3], capacitors[4, 5], solar cells[6], optical displays[7, 8], light emitting diodes[9, 10], as rechargeable batteries[11, 12], enzyme immobilization matrices[13, 14], gas separation membranes[15], and electrochromic devices[16]. Conducting polymers are very rigid in nature due to their extended delocalization. Thus, the unsubstituted CP is found to be infusible and intractable, and is less soluble in nature. To overcome this issue there are some methods such adding a matrix of insulating polymers into CP[17–23]. For the production of such polymers (eg: polypyrrole copolymer) copolymerization can be used since they improve the chemical stability of the polymer[22, 23]. The copolymer with new functional group will widen the application of conducting polymer when compared with the polypyrrole homopolymer.
Many researches have developed polypyrrole with enhanced physical and mechanical properties. They have synthesized different copolymers containing pyrrole and different insulating units such as ε-caprolactone, acryloyl chloride, methyl methacrylate, styrene, tetrahydrofuran etc.[23–28]. But applicability of these copolymers is limited due to their difficult synthesizing conditions and fabrication steps. However, a direct electrochemical copolymerization technique can be used in a proper monomer solution[29–31].
Different initiators were used in the copolymerization of pyrrole with miscellaneous benzaldehydes substituted such as: the trifluoro of boron etherate BF3(OEt)2, the sulphuric acid H2SO4, the acid hydrochloric HCl and oxidizers as the FeCl3, and the DDQ [(2,3-dicyano-5,6-dichloro-1,4-benzoquinone)]. These initiators and oxidizers are expensive, toxic, and difficult to use. For these above reasons, a natural catalyst Maghnite-H+ (Mag-H+), which is an Algerian proton exchanged montmorillonite clay[32–35] is proposed in this work. It is an effective, nontoxic, less polluting, and easily recoverable catalyst (Mag-H+) for the copolymerization of the two monomers (pyrrole with the pyrrole-2-carboxaldehyde) in solution. It has already been utilized for the cationic polymerization of a number of vinylic and heterocyclic monomers[32–35].
In the current research, the proposed technique to synthesise poly {(2,5-diyl pyrrole) (2-pyrrolyl methine)} (PPPM) copolymer is performed by condensation of pyrrole and pyrrole- 2-carboxaldehyde catalyzed by Mag-H+ is introduced. The effect of different parameters such as solvents, the molar ratio (pyrrole/pyrrole-2-carboxaldehyde), the time, the temperature, and the catalyst/reagents ratio have been studied.
2.
Materials and procedures
2.1
Materials
Pyrrole monomer (supplied by Aldrich) which was distilled under reduced pressure was used. Pyrrole-2-carboxaldehyde and solvents are commercially available (supplied by Aldrich) and used as received. The synthetic method and different stages for the formation of Poly {(2,5-diyl pyrrole) (2- pyrrolyl methine)} copolymer is presented in Fig. 1.

class="figure_img" id="Figure1"/>
Download
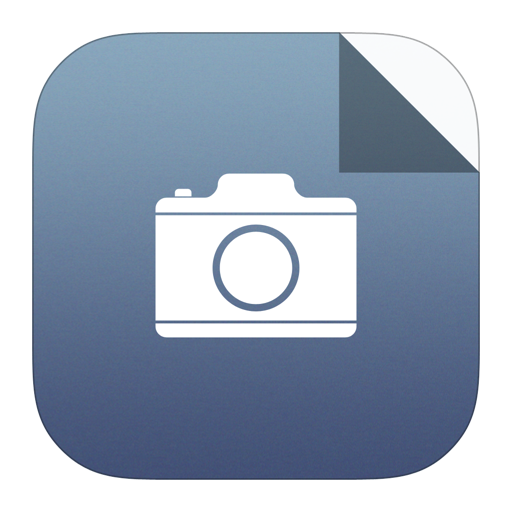
Larger image
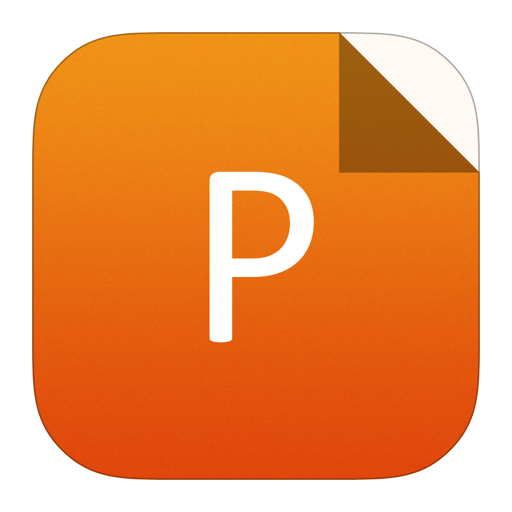
PowerPoint slide
Figure1.
Synthetic method of Poly {(2,5-diyl pyrrole) (2-pyrrolyl methine)} copolymer.
2.2
Preparation of Maghnite-H+ catalyst
Maghnite-H+ was prepared by following the steps reported by Belbachir et al.[32–36].
At first a prolabo ceramic balls grinder was used to crush the raw Maghnite (20 g) for 20 min. Then it was kept for drying at 105 °C for 2 h. Then an Erlenmeyer flask filled with 500 mL of distilled water is taken and Maghnite is placed in it. The mixture is then transferred to 0.25 M sulfuric acid solution and stirred using a magnetic stirrer. Saturation was attained during two days at room temperature. Distilled water was used to wash away minerals and to make it sulfate free. It is then dried at 105 °C. Finally, the sulfate was eliminated by rinsing with water and testing with barium nitrate.
2.3
Preparation of the copolymer
In a 100 mL flask, 10 mL of chloroform is contained and pyrrole (8 mmol) and pyrrole-2-carboxaldehyde (8 mmol) were dissolved. 10% by weight of Mag-H+ were added to this solution. The reaction conditions were fixed at 20 °C for 6 h. After this reaction, the clay is filtered out of the mixture and it is added to cold methanol slowly with continues stirring. Finally the polymer was kept for vacuum drying for 24 h at room temperature. The produced poly (2,5-diyl pyrrole -2- pyrrolyl methine) semiconductor copolymer material (yield %) from the reaction was found to be 56.29%.
3.
Results and discussion
The effect of different process parameters have been studied to determine to what extent they affect the good profitability of the copolymerization. The main parameters are the solvents, the molar ratio (pyrrole/pyrrole-2-carboxaldehyde), the time, the temperature, and the catalyst/reagents ratio. The experimental results are summarized in Table 1. Discussion on these results will be presented in the preceding sections.
Mag-H+(%) | Time (h) | Temperature (°C) | Molar ratio | Solvant | Yield (%) |
1 | 24 | Room | 50/50 | Chloroform | 46.47 |
3 | 24 | Room | 50/50 | Chloroform | 60.43 |
5 | 24 | Room | 50/50 | Chloroform | 60.65 |
7 | 24 | Room | 50/50 | Chloroform | 61.09 |
10 | 24 | Room | 50/50 | Chloroform | 64.00 |
10 | 2 | Room | 50/50 | Chloroform | 33.63 |
10 | 3 | Room | 50/50 | Chloroform | 34.62 |
10 | 4 | Room | 50/50 | Chloroform | 35.72 |
10 | 5 | Room | 50/50 | Chloroform | 44.70 |
10 | 6 | Room | 50/50 | Chloroform | 56.29 |
10 | 9 | Room | 50/50 | Chloroform | 61.12 |
10 | 12 | Room | 50/50 | Chloroform | 62.64 |
10 | 15 | Room | 50/50 | Chloroform | 63.04 |
10 | 18 | Room | 50/50 | Chloroform | 63.67 |
10 | 24 | Room | 50/50 | Chloroform | 64.00 |
10 | 6 | 10 | 50/50 | Chloroform | 35.68 |
10 | 6 | 20 | 50/50 | Chloroform | 56.29 |
10 | 6 | 30 | 50/50 | Chloroform | 39.91 |
10 | 6 | 40 | 50/50 | Chloroform | 37.43 |
10 | 6 | 50 | 50/50 | Chloroform | 36.05 |
10 | 6 | 60 | 50/50 | Chloroform | 32.25 |
10 | 6 | Room | 20/80 | Chloroform | 35.89 |
10 | 6 | Room | 40/60 | Chloroform | 49.61 |
10 | 6 | Room | 50/50 | Chloroform | 56.29 |
10 | 6 | Room | 60/40 | Chloroform | 46.11 |
10 | 6 | Room | 80/20 | Chloroform | 20.67 |
10 | 6 | Room | 50/50 | Chloroform (dielectric constant = 4.8) | 56.29 |
10 | 6 | Room | 50/50 | N-Methyl-2-pyrrolidone (dielectric constant = 32.2) | 58.21 |
10 | 6 | Room | 50/50 | Dimethylformamide (dielectric constant = 36.7) | 62.43 |
Table1.
Results of the effect of different process parameters on the copolymerization.
Table options
-->

Download as CSV
Mag-H+(%) | Time (h) | Temperature (°C) | Molar ratio | Solvant | Yield (%) |
1 | 24 | Room | 50/50 | Chloroform | 46.47 |
3 | 24 | Room | 50/50 | Chloroform | 60.43 |
5 | 24 | Room | 50/50 | Chloroform | 60.65 |
7 | 24 | Room | 50/50 | Chloroform | 61.09 |
10 | 24 | Room | 50/50 | Chloroform | 64.00 |
10 | 2 | Room | 50/50 | Chloroform | 33.63 |
10 | 3 | Room | 50/50 | Chloroform | 34.62 |
10 | 4 | Room | 50/50 | Chloroform | 35.72 |
10 | 5 | Room | 50/50 | Chloroform | 44.70 |
10 | 6 | Room | 50/50 | Chloroform | 56.29 |
10 | 9 | Room | 50/50 | Chloroform | 61.12 |
10 | 12 | Room | 50/50 | Chloroform | 62.64 |
10 | 15 | Room | 50/50 | Chloroform | 63.04 |
10 | 18 | Room | 50/50 | Chloroform | 63.67 |
10 | 24 | Room | 50/50 | Chloroform | 64.00 |
10 | 6 | 10 | 50/50 | Chloroform | 35.68 |
10 | 6 | 20 | 50/50 | Chloroform | 56.29 |
10 | 6 | 30 | 50/50 | Chloroform | 39.91 |
10 | 6 | 40 | 50/50 | Chloroform | 37.43 |
10 | 6 | 50 | 50/50 | Chloroform | 36.05 |
10 | 6 | 60 | 50/50 | Chloroform | 32.25 |
10 | 6 | Room | 20/80 | Chloroform | 35.89 |
10 | 6 | Room | 40/60 | Chloroform | 49.61 |
10 | 6 | Room | 50/50 | Chloroform | 56.29 |
10 | 6 | Room | 60/40 | Chloroform | 46.11 |
10 | 6 | Room | 80/20 | Chloroform | 20.67 |
10 | 6 | Room | 50/50 | Chloroform (dielectric constant = 4.8) | 56.29 |
10 | 6 | Room | 50/50 | N-Methyl-2-pyrrolidone (dielectric constant = 32.2) | 58.21 |
10 | 6 | Room | 50/50 | Dimethylformamide (dielectric constant = 36.7) | 62.43 |
3.1
Characterization
3.1.1
FTIR spectra
The Fourier transform infrared (FTIR) spectrum[37–39] of poly (2,5-diyl pyrrole-2-pyrrolyl methine) is illustrated in Fig. 2. The figure shows the presence of a strong absorption at 742 cm?1 of (PPPM). This corresponds to the Cβ–H out-of-plan vibration band which is the representative of α-linkage in the pyrrole ring. The IR peak observed at 1037 cm?1 corresponds to =C–H in the plane vibration band. The double bands at 1351 and 1397 cm?1 are assigned for the C–N stretching vibration band of pyrrole and pyrrole-2-carboxaldehyde. The peak at 1346 cm?1 is assigned to the C=C symmetric stretching vibration bands of the pyrrole rings. The broad band at 3143 cm?1 may be assigned for N–H stretching vibration of the pyrrole ring. The strong absorption at 1618 cm?1, which is attributed to the stretching vibration of conjugated C=C is clearly observed in the FTIR spectra of the copolymer. This confirms that the developed material is a copolymer.

class="figure_img" id="Figure2"/>
Download
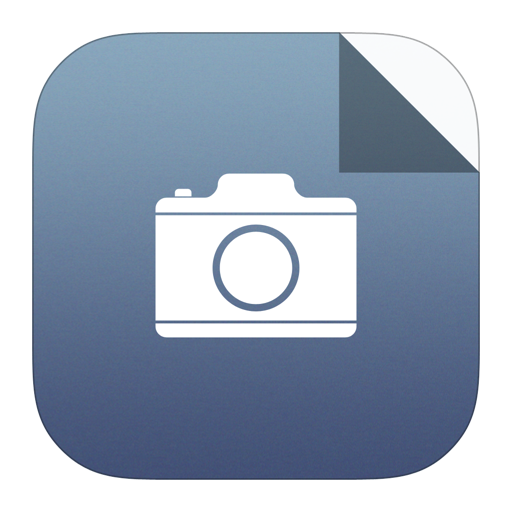
Larger image
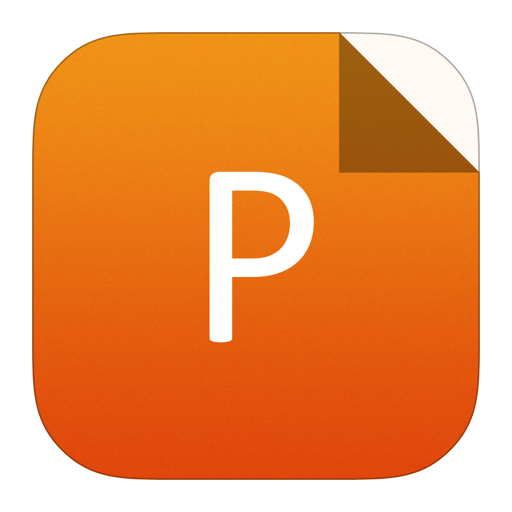
PowerPoint slide
Figure2.
FTIR spectrum of PPPM.
3.1.2
UV/visible spectra
The ultraviolet visible[40, 41] absorbance spectra of the copolymer, prepared using the polycondensation method, in CHCl3 solution is presented in Fig. 3. It can be seen that, two absorption bands appear in the UV–vis spectra of the virgin copolymer. The strong absorption band (band I) at 233 nm corresponding to 5.32 eV is assigned to the chromophore (C = C) of the pyrrole rings. Also, the second peak at 257 nm corresponding to 4.82 eV of energy is assigned to the π–π* band gap transition, which illustrates that the developed copolymer has a semiconductor band gap lower than 5 eV. This optical band gap is relatively higher than those obtained for semi-conductors used for the photovoltaic applications such as the silicon and germanium[42, 43] and organic semiconductors[44, 45]. This band gap energy can be considerably reduced by the doping process.

class="figure_img" id="Figure3"/>
Download
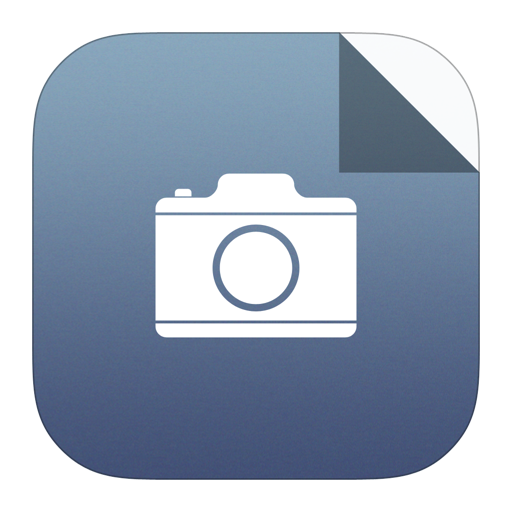
Larger image
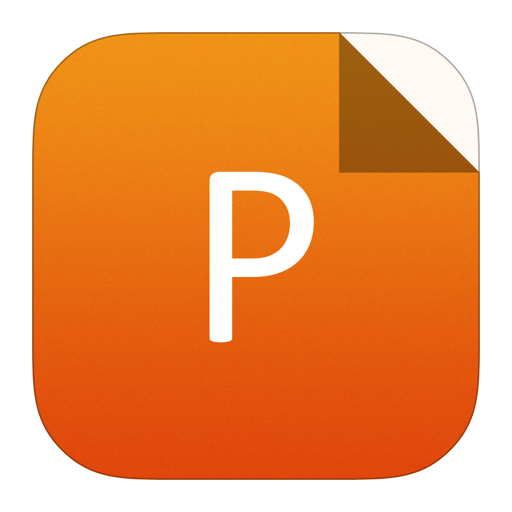
PowerPoint slide
Figure3.
The UV visible spectra of Poly [(2,5-diyl pyrrole 2-pyrrolyl methine)] in CHCl3.
3.1.3
1HNMR spectrum
The structure of Poly [(2,5-diyl pyrrole 2-pyrrolyl methine)] can be determined from the 1H NMR spectrum (Fig. 4). It is noted that hydrogen-1 nuclei is presented within the molecules of a substance. In the structure of the copolymer spectrum (Fig. 4), the peaks at 6.365, 7.040, and 7.215 ppm are presented and can be ascribed to protons in the a, b, and c positions; they are specific resonance signals of pyrrole ring protons. The peaks at 9.522 and 11.048 ppm can be ascribed to N–H protons in the e and d positions of the pyrrole rings, respectively. The same results are described by Rahmni et al.[45]. They have reported that 1H-NMR spectra of conductive, anticorrosive, and soluble polyaliniline exchanged by an eco-catalyst layered (Mag-H+). In their work, the 1H-NMR spectra of the PANIS-ES polymer exhibited a strong and sharp peak centered at 7 and 7.8 ppm due to proton on phenyline. Also, the medium broad peaks at 6.2 and 6.4 ppm were due to the (–NH2) end group, and the other broad peak located at 8 ppm was due to the water proton bonded by the (–NH2) group and (H–N+) respectively.

class="figure_img" id="Figure4"/>
Download
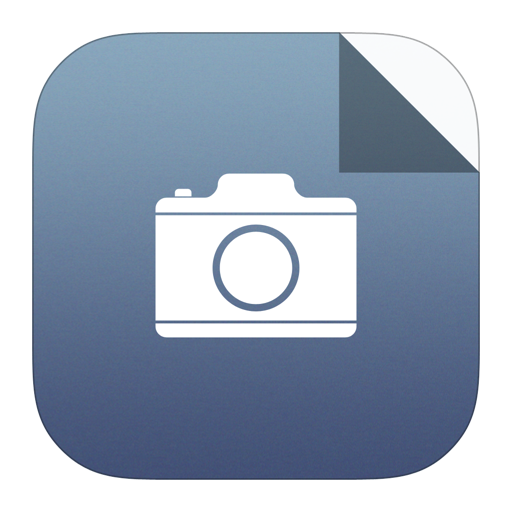
Larger image
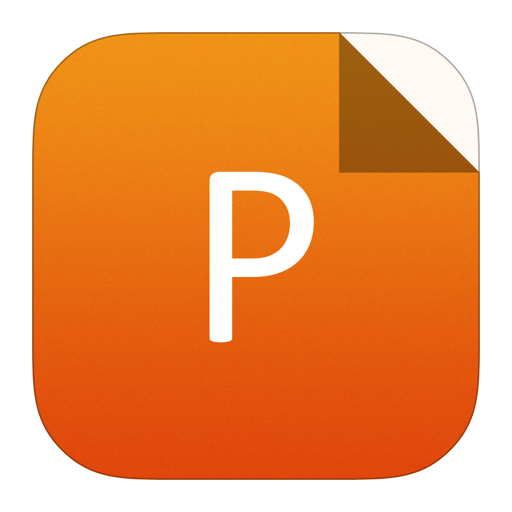
PowerPoint slide
Figure4.
300 MHz-1H-NMR spectrum of the PPPM in CDCl3.
3.1.4
13C NMR spectrum of the PPPM copolymer
Fig. 5 demonstrates the 13C-NMR spectrum of the poly [(2,5-diyl pyrrole 2-pyrrolyl methine)] prepared by using the Maghnite-H+ as a catalyst. Six signals are shown in Fig. 5. The signal at 111.363 ppm corresponds to (–C = C) of the pyrrole and the signals at 122.327, 127.491, 132.870, and 179.677 ppm correspond to (C = C) of the pyrrole rings. While the signal at 77.154 ppm corresponds to CDCl3. The majority of 13C chemicals fall in the range from 0.0 to 220 ppm. A rough grouping can be made according to the hybridization of the carbon atom, with sp3 carbons at the lowest frequency (0–70 ppm), sp carbons of the acetylene type at 70–100 ppm, sp2 carbons (bonded to C and H) at 100–150 ppm, sp2 carbons of carbonyl groups at 160–220 ppm, and sp carbons of the allene type at 210–220 ppm. For the developed copolymer spectrum only the signals corresponding to (C = C) and (–C = C) are present. The same results are described by Nan et al.[46] in the synthesis and characterization of new functionalized pyrrole copolymers, 13C-NMR experiments with PPy-co 1 and PPy-co 3. They have also found that the major peak appeared at 120–150 ppm, which corresponds to α and β carbon atoms of pyrrole rings.

class="figure_img" id="Figure5"/>
Download
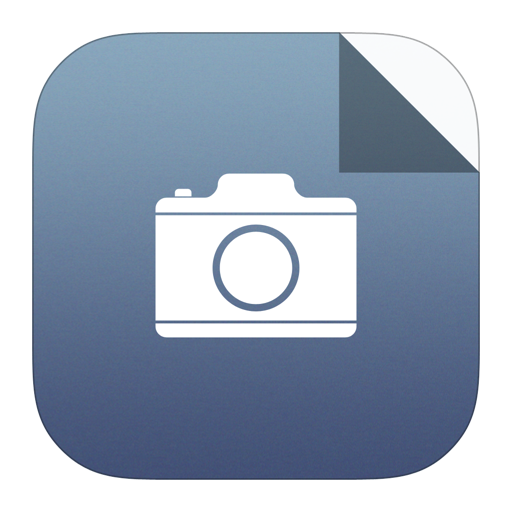
Larger image
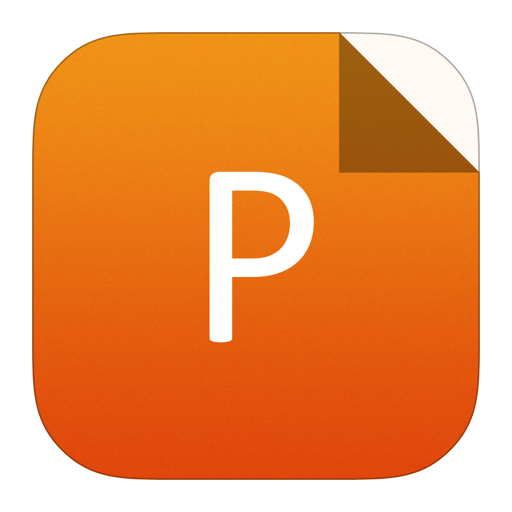
PowerPoint slide
Figure5.
300 MHz-13C NMR spectrum of the PPPM in CDCl3.
The number average molecular weight (Mn) and polydispersity index (PDI) of synthetized copolymer was calculated using (GPC) Gel Permeation Chromatography; the obtained results are: MnPPPM = 1066 g.mol?1 and PDI = 1.32.
3.2
Effect of the amount of Mag-H+ clay
The effect of the amount of Mag-H+ clay on the synthesized PPPM copolymer was investigated. Fig. 6 demonstrates the effect of the amount of Mag-H+ catalyst, in terms of various weight ratios of Mag-H+/monomer on the polymerization rate. The time of the polymerization was 24 h at room temperature. It is noticed that there is a rapid increase in the produced copolymer (yield%) from 46.47% to 60.4% as the amount of Mag-H+ catalyst increases from 1% to 3%. Then, the yield % increases with a relatively slow rate from 60.43% up to 64 with the amount of the Mag-H+ (from 3% to 10%). The polymerization was carried out in CHCl3 solution. We can observe from Fig. 6 that for 10% of Mag-H+ a yield of 64% was reached. Similar observations are reported by Boutaleb et al.[36] in the polymerization of the two monomers o-anisidine and aniline using Mag-H+ as a catalyst. This phenomenon is probably the result of the number of “initiating active sites” responsible for inducing polymerization. The number of active sites depends on the amount of the catalyst used in the reaction. The results shows that the optimum value of Mag-H+ clay catalyst is within the range from 3% to 10% and the amount used in this work is 10%.

class="figure_img" id="Figure6"/>
Download
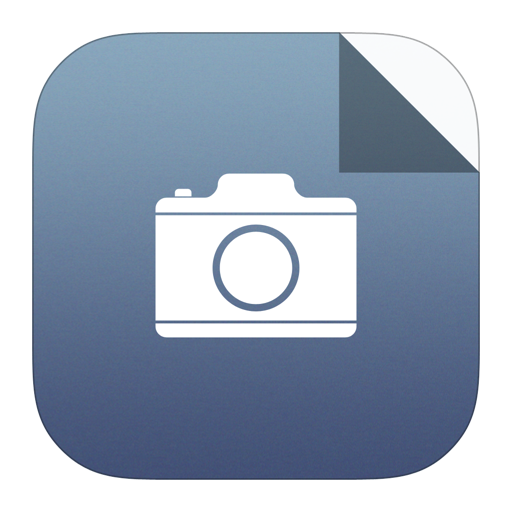
Larger image
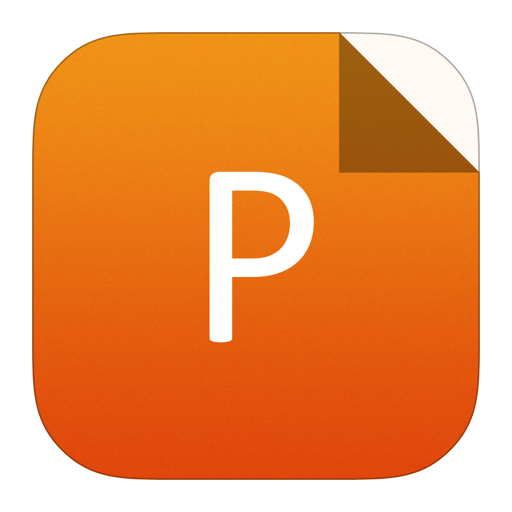
PowerPoint slide
Figure6.
Effect of the amount of Mag-H+ on the yield % of the PPPM. t = 24 h, T = 20 °C, and molar ratio of 1 : 1 in chloroform solution.
3.3
Effect of time on polymerization
The reaction resultant of the copolymer (yield%) versus the time for polymerization (by condensation) of pyrrole and pyrrole-2-carboxaldehydein presence of 10% of Mag-H+ is presented in Fig. 7. The curve shows four stages of polymerization with time. In the first stage (from 2 to 4 h) the yield % increases linearly with time with a rate of ≈ 1%/h, then the curve becomes steep with a rate of polymerization of ≈ 10.3%/h during the second stage (from 4 to 6 h). The rate of polymerization (≈ 1%/h) reduces during the third stage (from 6 to 12 h) prior the variation becoming approximately constant (≈ 0.5%) during the fourth stage (12 to 24 h). This is probably the result of an increase in the medium viscosity. Therefore, 6 hours of polymerization was used in this study.

class="figure_img" id="Figure7"/>
Download
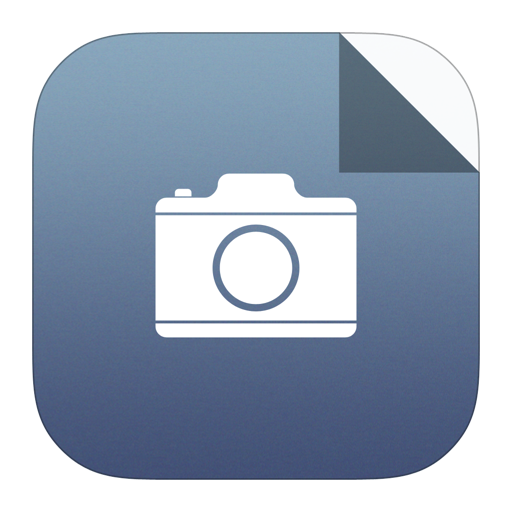
Larger image
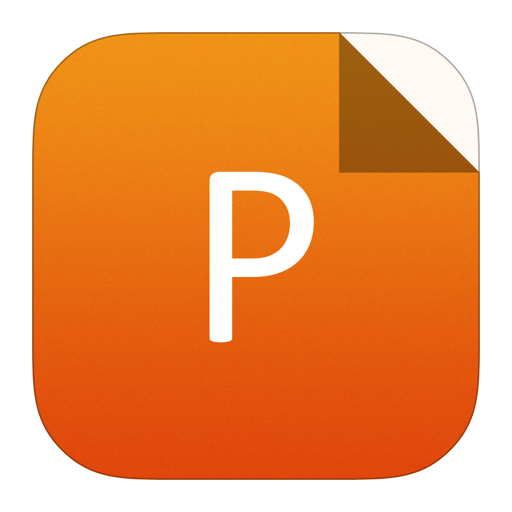
PowerPoint slide
Figure7.
Yield% of the PPPM copolymer versus time for polymerization of pyrrole and pyrrole-2-carboxaldehyde. 10% of Mag-H+, T = 20 °C, and molar ratio of 1 : 1 in chloroform solution.
3.4
Effect of temperature on polymerization
The temperature during the polymerization reaction will also play an important role in the initiation process and its influence is worth studying. The polymerization reaction has been conducted in chloroform solution with a molar ratio of 1 : 1, at different temperatures (10, 20, 30, 40, 50, and 60 °C) for 6 h in the presence of Mag-H+. Fig. 8 reflects the significant effect of the polymerization temperature on the yield % of PPPM. The yield% rises from 36% to 56% when the temperature increases from 10 to 20 °C, then the yield% decreases dramatically with temperature up to a temperature of 30 °C. This is followed by a relatively slow decrease in the yield% to achieve a value of 32.25% at 60 °C. This decrease in produced copolymer is essentially due to the increase in the intramolecular chain transfer and the formation of the macrocycles. These macrocycles evaporate with the solvent-non-solvent mixture. This results in the precipitation of linear polymer chains of large mass and eliminate oligomers (macrocycles). This is the reason that high temperatures lead to the formation of macrocycles which are not recoverable by the purification technique employed. This indicates that, the optimum polymerization reaction temperature is around 20 °C.

class="figure_img" id="Figure8"/>
Download
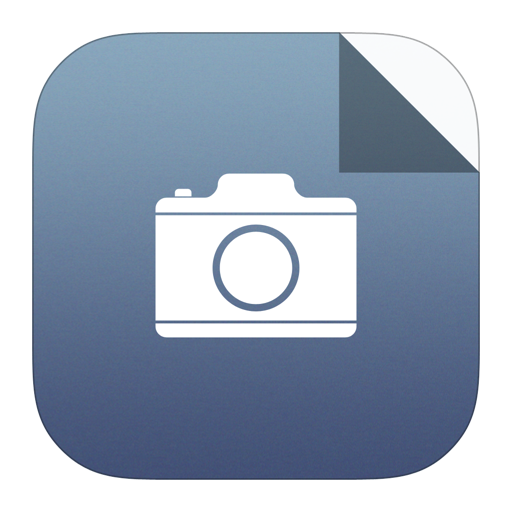
Larger image
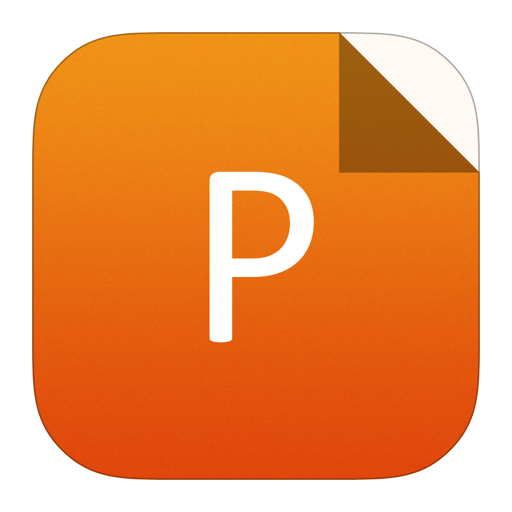
PowerPoint slide
Figure8.
Variation of the yield% of PPPM with polymerization reaction temperature. t = 24 h, 10% of Mag-H+ and molar ratio of 1 : 1 in chloroform solution.
3.5
Effect of molar ratio on polymerization
In the presence of Maghnite-H+ and using different [pyrrole]/[pyrrole-2-carboxaldehyde] molar ratios the yield% of the PPPM has been obtained. The test has been carried out at the obtained optimum polymerization reaction conditions (namely, 6 hours polymerization time at room temperature and 10% of Mag-H+). The results are demonstrated in Fig. 9. Two different stages can be recognized. In the first stage as the ratio of the pyrrole/pyrrole-2-carboxaldehyde increases from 20/80 to 50/50 the yield% increases from 35% to 56%. In contrast, for the high percentage of pyrrole (for a ratio higher than 50/50) the yield% decreases sharply from 56% to 20% at a ratio of 80/20. It can be concluded that the yield% of the PPPM copolymer was significantly influenced by the monomer ratio. The copolymer exhibits the highest amount of yield at the [pyrrole]/[pyrrole-2-carboxaldehyde] ratio of 50/50. It is known that, in polymerization by condensation, one mole of pyrrole monomer reacts with one mole of pyrrole-2-carboxaldehyde monomer, and as the content of any increases, the rate of polymerization declines.

class="figure_img" id="Figure9"/>
Download
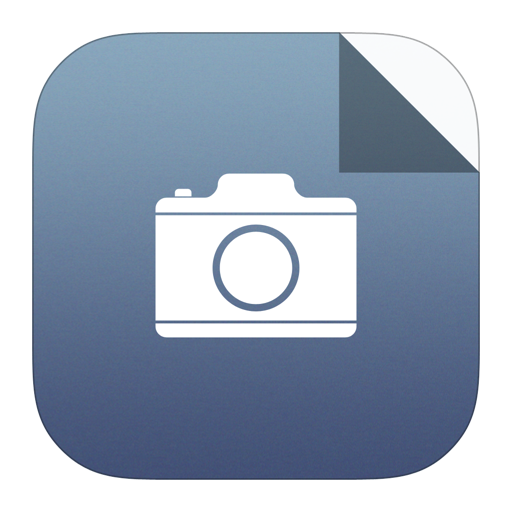
Larger image
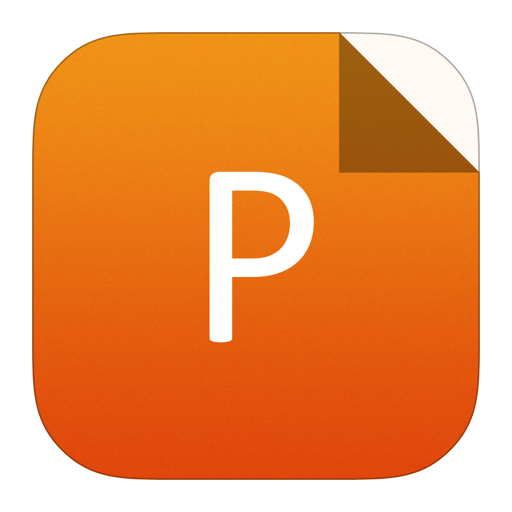
PowerPoint slide
Figure9.
Variation of PPPM yield% with molar ratio. t = 24 h, 10% of Mag-H+ and T = 20 °C in chloroform solution.
3.6
Effect of solvent on polymerization
The synthesis of PPPM copolymer was carried out in three different solvents. These are CHCl3 (dielectric constant = 4.8), NMP (dielectric constant = 32.2), and DMF (dielectric constant = 36.7). Fig. 10 shows the effect of the solvent type on the synthesis of PPPM copolymer. The rate of polymerization in DMF solvent was found to be much higher than that in NMP and CHCl3 solvents. There is a significant effect of the dielectric constant of solvent (CHCl3, NMP and DMF) on the yield% of the copolymer. Most of polar solvents produce a copolymer with high yield.

class="figure_img" id="Figure10"/>
Download
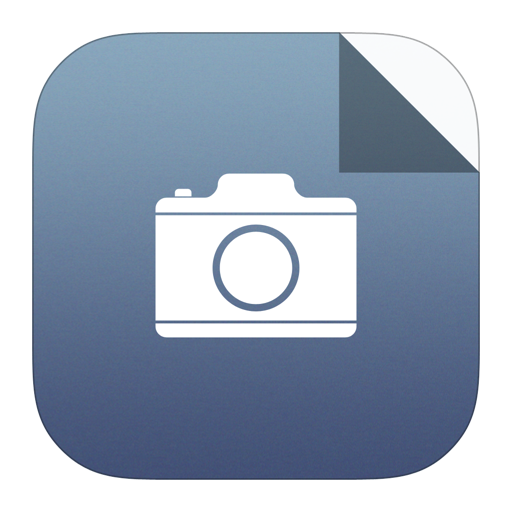
Larger image
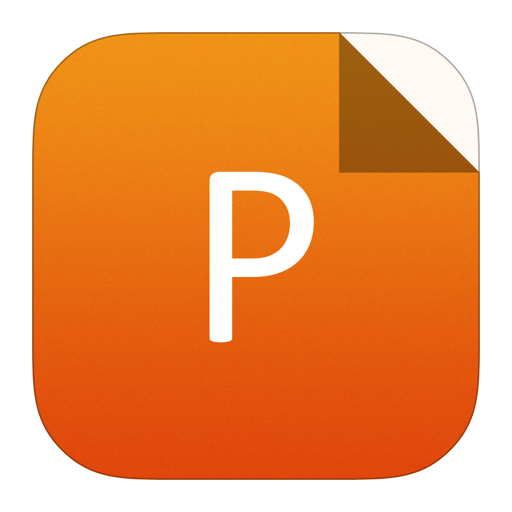
PowerPoint slide
Figure10.
Effect of the solvent on the synthesis of PPPM copolymer. t = 24 h, T = 20 °C, molar ratio of 1 : 1 and 10% of Mag-H+.
3.7
Solubility of the PPPM copolymer
Solubility tests have been carried out to ensure that the newly developed copolymer is soluble in most organic solvents. A small amount of copolymer powder (black color) was put into an amount of solvent (transparent). All the results show the formation of a homogeneous black solution as evidence of the solubility of the developed copolymer. The results of these tests are grouped in Table 2.
Solvent | Test results |
Chloroform | + |
Dimethylformamide, DMF | + |
N-Methyl-2-pyrrolidone, NMP | + |
Acetone | + |
Toluene | – |
Methanol | – |
Soluble (+) Insoluble (–) |
Table2.
Solubility test results.
Table options
-->

Download as CSV
Solvent | Test results |
Chloroform | + |
Dimethylformamide, DMF | + |
N-Methyl-2-pyrrolidone, NMP | + |
Acetone | + |
Toluene | – |
Methanol | – |
Soluble (+) Insoluble (–) |
4. Conclusions
The PPPM copolymer, poly [(2,5-diyl pyrrole-2-pyrrolyl methine)], which has a π- conjugated chain was synthesized by a new method of polycondensation of pyrrole and pyrrole-2 carboxaldehyde using Maghnite-H+ as a catalyst.
The effect of the different parameters on the polymerization of the produced PPPM copolymer was investigated. The results revealed clearly that all parameters play an important role on the synthesis of this copolymer. The polymerization yield% exhibits an obvious dependency on the amount of Mag-H+, [pyrrole]/[pyrrole-2-carboxaldehyde] molar ratio, time, temperature, and type of solvent. The optimum values of the reaction parameters were 10 wt % of Mag-H+, 6 h polymerization time at room temperature, and 50/50 molar ratio. A yield of 56 wt% was achieved using these optimum parameters. The polymerization rate in DMF was also found to be much greater than that in NMP and CHCl3, due to the effect of the dielectric constant of the solvent on the yield of the copolymer. The insolubility of polypyrrole can be overcome by copolymerizing pyrrole with pyrrole-2-carboxaldehyde. The resultant copolymer showed good solubility in common organic solvents and good film formability. The FTIR, UV visible spectra, and 1H, 13C NMR confirmed that the developed copolymer is a real copolymer consisting of two monomers. This developed copolymer has many potential industrial applications.