1.
Introduction
Powder perfume spray pyrolysis is the best method that does not require any specific arrangement at high cost and a separate place to perform the experiment. Ravichandaran et al.[1] reported a simplified spray pyrolysis method for thin film making in their work. Investigations on microstructural and optical properties of CdS films are studied by a low-cost, simplified spray technique using a perfume atomizer for solar cell applications[2]. In this aspect, this method is not only applicable for making thin film only, but also to prepare nanoparticles or nanograins and nanocomposites in powder format using the MSP method in very little time. Samples in a powder format are very much essential in the fields of biotechnology[3, 26–34]. Ayeshamariam et al.[4] reported that synthesis and antibacterial investigation of ZnO/CNPs nanocomposite powder by a hot nickel plate assisted cost effective spray pyrolysis method and its characterizations. Synthesis of ZnO-CNT and hot copper plate assisted synthesis of ZnO nanoflakes by a cost effective Modified Spray Pyrolysis method and its structural, morphological and optical studies have been reported. Nanoparticles are specifically different from their fundamental elements, which are derived by altering their dimension in the range of 10?9 nm by means of changing the atomic and molecular properties of elements[35–37]. Nanotechnology research has a vibrant positive impulse in the current trends by offering enhanced solutions in the field of materials science, optoelectronics, and biomedical technology[5, 38–42]. Generally, the nanocomposite such as binary and ternary nano crystals exhibit optical and electrical, gas sensing, photo voltaic (solar cell), ceramic and antimicrobial properties[6]. Zinc oxide powder is an important source of the popularly known calamine solution for curing ordinary skin disease. It is also used in the ceramic industry, food products preservatives and paints[43–46]. ZnO NPs is one of the most essential transition metal oxide semiconducting materials for wide range application with bandgap energy 3.37 eV[7]. Due to its excellent electrical and optical property, this metal oxide nano composite is commonly used in nano electronics and optoelectronic devices[8]. CuO NPs have a wide range of applications in gas sensor, ceramic, surface antigen magnetic storage devices and the biomedical field. Due to its good electrical, optical and magnetic properties, it is used as a semiconductor, high tech solar energy transformer, photo conductor and chemical sensing device[9]. In the conventional spray pyrolysis technique, we need costly equipment and spraying the solution must be done in the controlled temperature and surrounding. Also, thin film is obtained on a glass or transparent substrate only. But in the present investigation the precursor solution is sprayed on hot copper plate employing an ordinary perfume sprayer. At the end, we get nano powder. We call this cost-effective technique the modified spray pyrolysis (MSP) method.
2.
Experimental technique
Double deionized water was used as the solvent for both precursor materials zinc acetate dehydrates Zn(CH3COO)2· 2H2O and copper acetate dehydrate Cu(CO2CH3)2·2H2O, which were purchased from Sigma Aldrich. The ZnO–CuO nanoparticles were synthesized via the MSP method, ZnO and CuO reactants were mixed in a 2 : 1 molar ratio. 40 mL of Zn(CH3COO)2·2H2O and 20 mL of Cu(CO2CH3)2·2H2O were stirred in a separate beaker for 50 min at 50 °C. The ZnO and CuO solution were mixed and stirred for 30 min at 70 °C. Finally, the mixture was transferred into a well cleaned perfume spray bottle for spraying the solution at a copper plate with dimension 150 × 150 × 2 mm3 at 500 °C. After the manual spraying with equal intervals of time at an ambient temperature, the resulting product was calcined at 250 °C for 1 h in a muffle furnace. Crystallographic data such as the structural characterization of the synthesized samples was carried out by X-ray diffraction using an X'Pert Pro with minimum step size Omega: 0.001° and CuKα radiation, λ = 1.5406 ?. Field emission scanning electron microscopy images were recorded on an FEI-Quanta-FEG 250 operating at 30 kV and equipped with an energy dispersive X-ray spectroscopy (EDX) detector. Optical data like absorption spectra for functional groups were recorded using the Fourier transform infrared spectrometer of the Bruker Tensor 27 at TR mode in the wave number range 400–4000 cm?1 and absorption spectra were recorded using a JOSCO UV–Vis spectrophotometer in the wavelength range 190–900 nm.
3.
Result and discussion
In different approaches to produce the ZnO–CuO heterostructure, including the sol-gel method, the direct combustion method, and the co-precipitation method followed by the calcination, soft chemical route technique[10], and solvothermal techniques[11] have been investigated. The ZnO–CuO NPs which are analyzed in this work were prepared by the modified spray pyrolysis method. To the best of our knowledge, the first report on ZnO–CuO nanocomposite was synthesized by the Modified spray pyrolysis method.
3.1
XRD analysis
X-ray diffraction of undoped and doped combined metal oxide nano powder with two different molar ratios are shown in Fig. 1. The peaks at 2θ positions of 31.72°, 34.32°, 36.17°, 47.52°, 56.42°, and 62.72° are found to be relative to ZnO with a hexagonal wurtzite structure (JCPDS card number 36-1451) and CuO with a monoclinic structure (JCPDS card number 05-0661), and the 2θ position of CuO peaks appeared at 35.58° and 38.52°. The diffraction peaks of both composite and pure ZnO are sharp and intense, indicating the highly crystalline nature[12]. The crystallite size (D) values are calculated using Debye-Scherrer’s formula D = 0.9λ/βcosθ in nm, where D is the crystallite size, λ is the wavelength of the X-ray employed CuKα radiation (0.154 nm), β is the full width at half maximum (FWHM) of the peaks and θ is the Bragg angle obtained from the 2θ value. Lattice constants a and c for ZnO hexagonal wurtzite structure are calculated using the relation
ight) + frac{{{l^2}}}{{{c^2}}}$

m{sin}}{^2}beta }}left( {frac{{{h^2}}}{{{a^2}}} + frac{{{k^2}{
m{sin}}{^2}beta }}{{{a^2}}} + frac{{{l^2}}}{{{c^2}}} - frac{{2hl{
m{cos}}beta }}{{ac}}}
ight)$

ight)sin 60^circ $

m{sin beta }}$



m cos}theta }}{4}.$


class="figure_img" id="Figure1"/>
Download
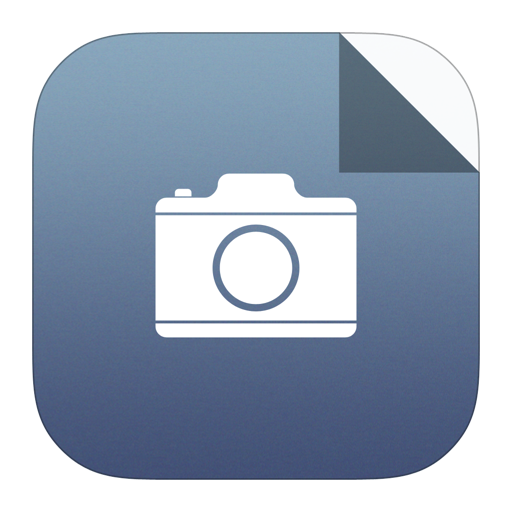
Larger image
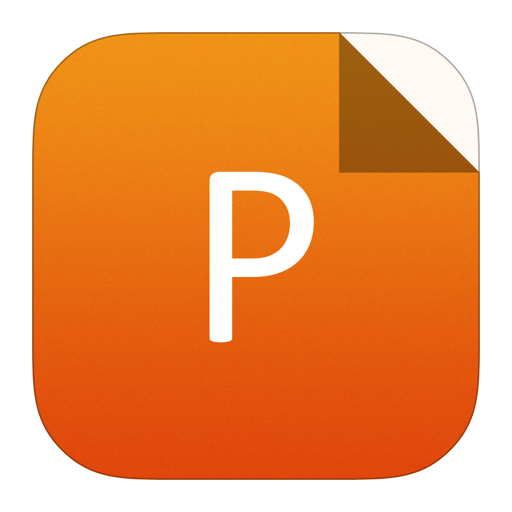
PowerPoint slide
Figure1.
(Color online) X-ray diffraction patterns of (a) ZnO, (b) & (c) ZnO–CuO NPs at different concentrations prepared by the MSP method.
Samples | Metal oxides | 2θ (deg) | hk1 | FWHM (deg) | d (?) | a (?) | b (?) | c (?) | |
Std | Expt | ||||||||
ZnO | ZnO | 36.2527 | 101 | 0.3428 | 2.4759 | 2.4755 | 3.246 | 0 | 5.2018 |
ZnO : CuO | ZnO | 36.2507 | 101 | 0.2460 | 2.4759 | 2.4781 | 3.256 | 0 | 5.2154 |
2 : 1 M | CuO | 35.5901 | 002 | 0.1968 | 2.5300 | 2.5225 | 4.661 | 3.462 | 5.1156 |
38.6308 | 111 | 0.3444 | 2.3130 | 2.3308 | |||||
48.6877 | 202 | 0.2952 | 1.8660 | 1.8702 | |||||
ZnO : CuO | ZnO | 36.2275 | 101 | 0.1968 | 2.4759 | 2.4796 | 3.246 | 0 | 5.2219 |
3 : 1 M | CuO | 35.4516 | 002 | 0.1476 | 2.5300 | 2.5252 | 4.672 | 3.439 | 5.1192 |
38.9001 | 200 | 0.2460 | 2.3230 | 2.3276 | |||||
Samples | Metal oxides | c/a | v (?3) | D (nm) | V (l03 nm3) | Nu (106) | l (?) | ε ( 10?3) | δ (1014 lines/m2) |
ZnO | ZnO | 1.6020 | 47.492 | 24 | 13.824 | 0.29 | 1.883 | 81.45 | 17.36 |
ZnO : CuO | ZnO | 1.6017 | 47.886 | 34 | 39.304 | 0.82 | 1.890 | 58.45 | 08.65 |
2 : 1 M | CuO | 1.0975 | 81.443 | 42 | 74.088 | 0.90 | – | 46.85 | 05.66 |
28 | 21.952 | 0.27 | – | 81.25 | 12.75 | ||||
24 | 13.824 | 0.16 | – | 67.24 | 17.36 | ||||
ZnO : CuO | ZnO | 1.6082 | 47.675 | 38 | 54.872 | 0.67 | 1.887 | 46.76 | 06.92 |
3 : 1 M | CuO | 1.0920 | 81.143 | 51 | 132.651 | 1.63 | – | 35.15 | 03.84 |
30 | 27.000 | 0.33 | – | 57.99 | 11.11 |
Table1.
Structural studies of (a) ZnO, (b) ZnO–CuO nanocomposite 2 : 1 M and (c) ZnO–CuO nanocomposite 3 : 1 M.
Table options
-->

Download as CSV
Samples | Metal oxides | 2θ (deg) | hk1 | FWHM (deg) | d (?) | a (?) | b (?) | c (?) | |
Std | Expt | ||||||||
ZnO | ZnO | 36.2527 | 101 | 0.3428 | 2.4759 | 2.4755 | 3.246 | 0 | 5.2018 |
ZnO : CuO | ZnO | 36.2507 | 101 | 0.2460 | 2.4759 | 2.4781 | 3.256 | 0 | 5.2154 |
2 : 1 M | CuO | 35.5901 | 002 | 0.1968 | 2.5300 | 2.5225 | 4.661 | 3.462 | 5.1156 |
38.6308 | 111 | 0.3444 | 2.3130 | 2.3308 | |||||
48.6877 | 202 | 0.2952 | 1.8660 | 1.8702 | |||||
ZnO : CuO | ZnO | 36.2275 | 101 | 0.1968 | 2.4759 | 2.4796 | 3.246 | 0 | 5.2219 |
3 : 1 M | CuO | 35.4516 | 002 | 0.1476 | 2.5300 | 2.5252 | 4.672 | 3.439 | 5.1192 |
38.9001 | 200 | 0.2460 | 2.3230 | 2.3276 | |||||
Samples | Metal oxides | c/a | v (?3) | D (nm) | V (l03 nm3) | Nu (106) | l (?) | ε ( 10?3) | δ (1014 lines/m2) |
ZnO | ZnO | 1.6020 | 47.492 | 24 | 13.824 | 0.29 | 1.883 | 81.45 | 17.36 |
ZnO : CuO | ZnO | 1.6017 | 47.886 | 34 | 39.304 | 0.82 | 1.890 | 58.45 | 08.65 |
2 : 1 M | CuO | 1.0975 | 81.443 | 42 | 74.088 | 0.90 | – | 46.85 | 05.66 |
28 | 21.952 | 0.27 | – | 81.25 | 12.75 | ||||
24 | 13.824 | 0.16 | – | 67.24 | 17.36 | ||||
ZnO : CuO | ZnO | 1.6082 | 47.675 | 38 | 54.872 | 0.67 | 1.887 | 46.76 | 06.92 |
3 : 1 M | CuO | 1.0920 | 81.143 | 51 | 132.651 | 1.63 | – | 35.15 | 03.84 |
30 | 27.000 | 0.33 | – | 57.99 | 11.11 |
Bacteria | Zone of inhibition diameter in mm (3 : 1) M | |
10 μL | 20 μL | |
a) pseudomonas aeruginosa | 7 | 16 |
b) Proteus mirabilis | 15 | 20 |
c) E.coli | 6 | 19 |
d) Staphylococcus aureus | 12 | 16 |
Table2.
Bacterial analysis of ZnO-CuO nanocomposite 3 : 1 M.
Table options
-->

Download as CSV
Bacteria | Zone of inhibition diameter in mm (3 : 1) M | |
10 μL | 20 μL | |
a) pseudomonas aeruginosa | 7 | 16 |
b) Proteus mirabilis | 15 | 20 |
c) E.coli | 6 | 19 |
d) Staphylococcus aureus | 12 | 16 |
3.2
SEM and EDX analysis
The cleared morphology of the synthesized ZnO–CuO nanocomposite powder surface was examined by scanning with a beam of high energy electron field emission scanning electron microscopy (FESEM) and the results are depicted in Fig. 2(a). From the observed images, it can be confirmed that the prepared powder has a nanoparticle shape, which are agglomerated due to growth in high density. Moreover, the agglomerated ZnO nanoparticles are incorporated with CuO nanoflakes are shown in the manually magnified image separately in Fig. 2(b). The FESEM images revealed that the typical size of the NPs is in the range 35 ± 10 nm (Fig. 2(a)). In Fig. 2(c), the inset shows the elemental composition of the prepared ZnO–CuO nanocomposite powder, which is investigated by using Energy Dispersive X-ray Spectroscopy (EDX). This observed spectrum shows that the presence of many well-defined peaks are related with Zn, Cu and O only. Also, it is observed that no other peaks related with impurities are present in the EDX spectrum, which confirmed the formation and purity of ZnO–CuO nano composite[14].

class="figure_img" id="Figure2"/>
Download
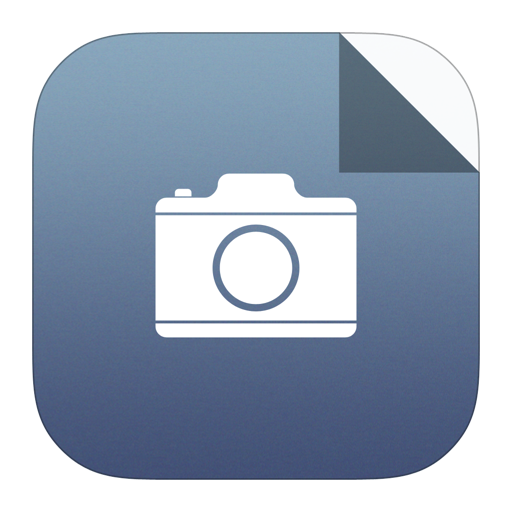
Larger image
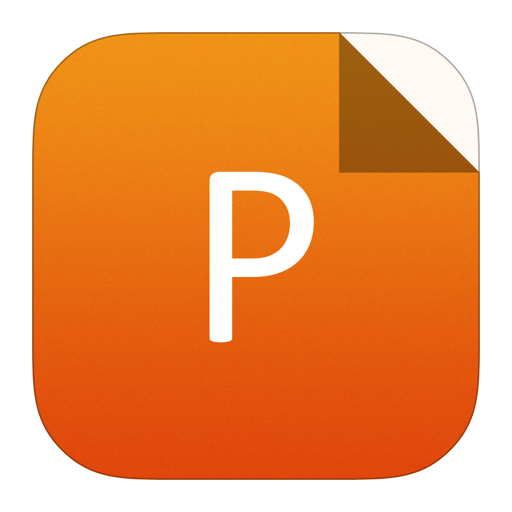
PowerPoint slide
Figure2.
(Color online) FESEM images of ZnO–CuO nanocomposite of 3 : 1 M, 60 000 × magnification, sandwich like morphology. (b) Describing image of (a) and (c) EDX spectrum of ZnO–CuO nanocomposite synthesized by the MSP method where the inset represents the percentage of elemental composition.
3.3
AFM 2D and 3D analysis
Figs. 3(a)–3(d) show 2D and 3D AFM micrographs of ZnO–CuO nanocomposites of different ratios that were subjected to an annealing temperature of 300 °C; the AFM micrographs clearly illustrate that the grains of CuO NPs are covered by ZnO NPs that cluster at the surface with the most roughness. Due to the high concentration of ZnO NPs, agglomeration gets increased. This led to the increase in the thickness as well as the surface roughness of the ZnO composite which were bonded on the surface of CuO NPs. However, surface roughness is uniform for some regions but not uniform for most regions. It is observed that surface roughness decreases when the concentration of ZnO increases[15].

class="figure_img" id="Figure3"/>
Download
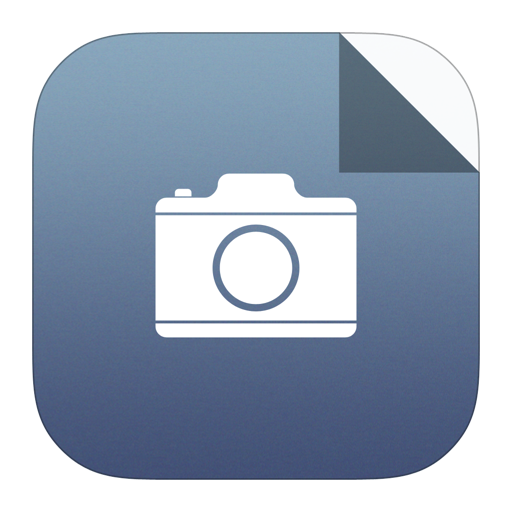
Larger image
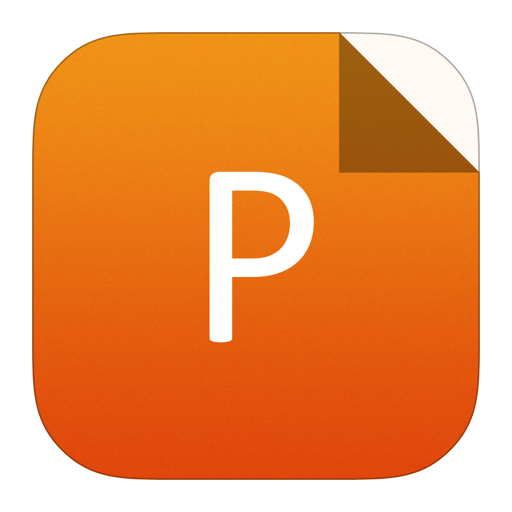
PowerPoint slide
Figure3.
(Color online) AFM images of the 2 : 1 M (roughness ?3.8 nm RMS, at 300 °C), and 3 : 1 M (roughness ?2.4 nm RMS, at 300 °C) ZnO–CuO nanocomposites respectively. (AFM image scans are 3 × 3 μm.)
3.4
FTIR analysis
The Fourier transform infrared spectroscopy (FTIR) analysis used to examine the chemical composition and the functional group of ZnO–CuO nanocomposite. Figs. 4(a)?4(c) shows that the functional group of samples was recorded under the infrared wavenumber of 4000–400 cm?1. The main functional group such as ZnO, CuO and CuO–ZnO nanocomposite can be seen in Figs. 4(a)?4(c). In this figure, the absorption band at 443 cm?1 is attributed to the Zn–O stretching mode vibration for these three samples. The Cu–O stretching is represented by absorption bands 616 cm?1 for samples b and c. The O–H stretching mode vibration could be observed at 3600–3100 cm?1 for sample b and c as shown in Fig. 4, which confirms the presence of hydroxyl groups. Stretching modes of both C–O and C=O could be observed at 1110 cm?1 (υs) and 1626 cm?1 (υs) respectively. This functional group appeared may be due to the improper decomposition of the acetate group present in the precursor solution[16].

class="figure_img" id="Figure4"/>
Download
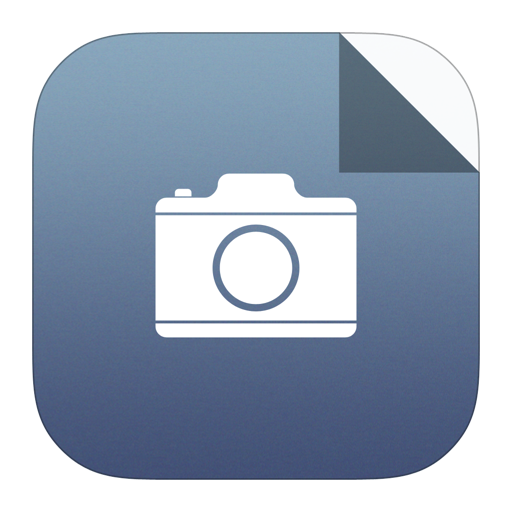
Larger image
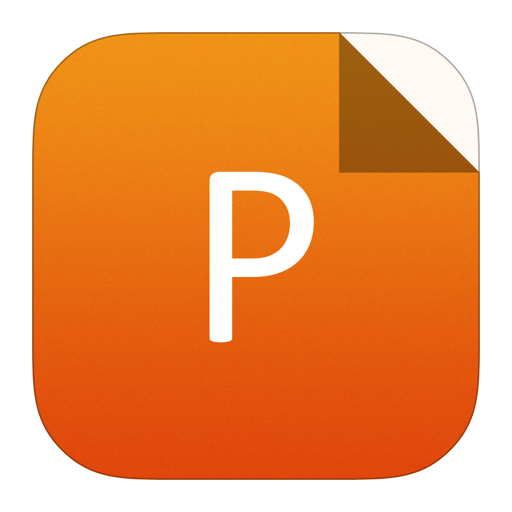
PowerPoint slide
Figure4.
(Color online) FTIR spectra of ZnO (a), and ZnO–CuO nanocomposite (2 : 1 M) (b) & (3 : 1 M) (c) synthesized by the MSP method.
3.5
UV–vis analysis
Figs. 5(a)–5(c) show the UV–vis spectra of the ZnO NPs and ZnO–CuO nanocomposite. The absorption curve for pure ZnO depicts absorption at about 346 nm. Bulk ZnO has a direct-gap of 3.15 eV[17]. In a nanostructures aspect, the electronic states become completely discrete, as per atoms and molecules. But in the case of the electronic population between a pair of discrete states, controlling the transition whether it is allowed or not leads to the red shift in the peak centered at ~336 nm in the absorption spectra in Figs. 5(a)–5(c). Therefore, it is obvious that the peak position is highly blue shifted compared to bulk Zn and Cu oxide material with a direct-gap transition. The direct bandgap of ZnO and ZnO/CuO is estimated by Tauc’s formula, (αhυ)n = B(hυ – Eg), where B is a constant related to the material, hν is the photon energy in eV, h is Planck’s constant, Eg is the optical bandgap in eV, n is an exponent that can take a value of either 2 for a direct transition or 1/2 for an indirect transition, and α is the absorption coefficient (in cm?1). The transmittance is calculated from measured absorbance (Aλ) at a certain wavelength of light (λ) using the formula: Aλ =


class="figure_img" id="Figure5"/>
Download
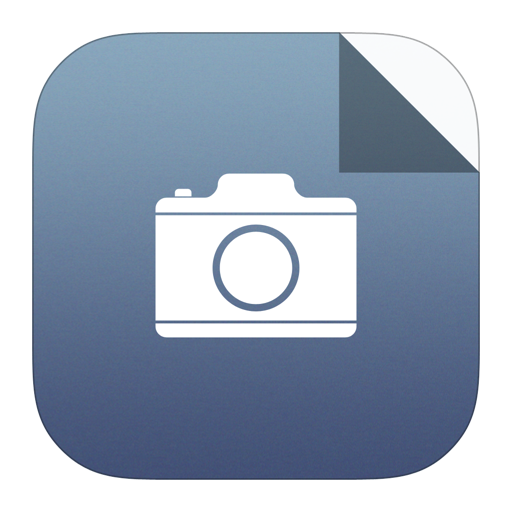
Larger image
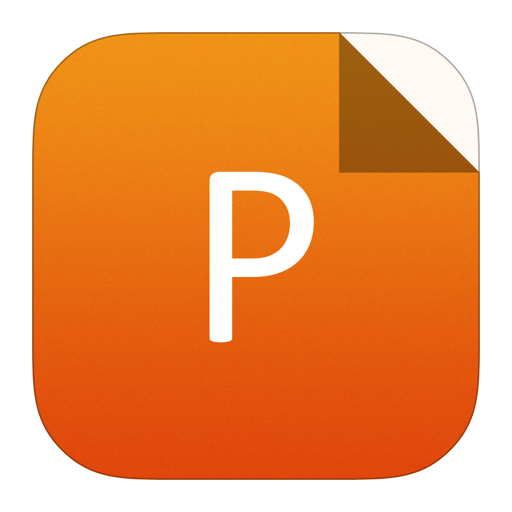
PowerPoint slide
Figure5.
(Color online) UV–vis optical absorption of ZnO NPS (a) ZnO–CuO nanocomposite (2 : 1 M) (b) & (3 : 1 M) (c) synthesized by the MSP method.

class="figure_img" id="Figure6"/>
Download
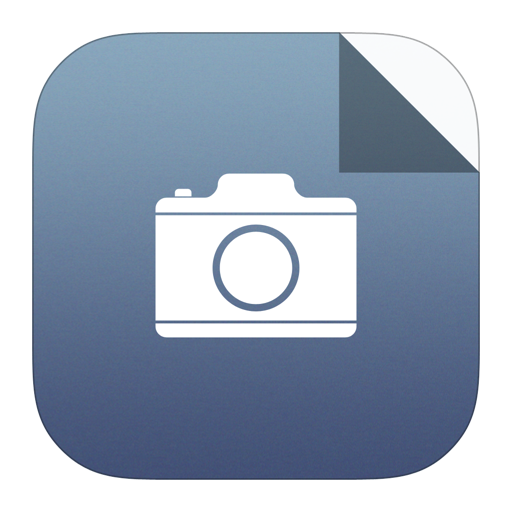
Larger image
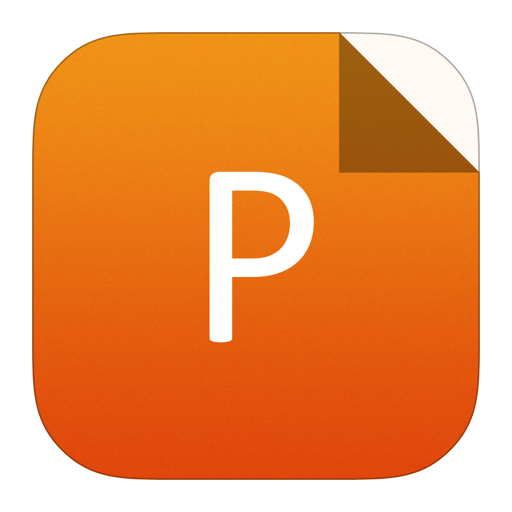
PowerPoint slide
Figure6.
(Color online) Tauc plot ((αhν)2 versus the hν plot) for ZnO NPS (a), ZnO–CuO nanocomposite (2 : 1 M) (b) & (3 : 1 M) (c) synthesized by the MSP method.
3.6
Antibacterial activity
In the disc diffusion method, the inoculums for the experiment were prepared in a fresh nutrient broth from a preserved slant culture. The inoculums were standardized by adjusting the turbidity of the culture to that of McFarland standards. The turbidity of the culture may be adjusted by the addition of sterile saline or broth (if excessive or by further incubation to get the required turbidity)[19]. The standardized inoculums are inoculated in the plates prepared earlier (aseptically) by dipping a sterile swab in the inoculums, removing the excess of inoculums by pressing and rotating the swab firmly against the side of the culture tube above the level of the liquid and finally streaking the swab all over the surface of the medium 3 times and rotating the plate through an angle of 60° after each application. Finally, pass the swab round the edge of the agar surface. Leave the inoculums to dry at room temperature with the lid closed. Each Petri dish is divided into 3 parts: one is standard while the other two are in different quantities such as 10 and 20 μL, where each part samples the disc such as a, b, c, d (discs are soaked overnight in the sample solution), and the standard ciprofloxacin 10 μL was placed in the plate. Here the three samples are (i) standard drug 10 μL, (ii) ZnO–CuO nanocomposite (3 : 1 M) sample 10μL and (iii) ZnO–CuO nanocomposite (3 : 1 M) sample 20μL. Then the Petri dishes are placed in the refrigerator at 4 °C or at room temperature for 1 h for diffusion. Incubate at 37 °C for 24 h. Observe the zone of inhibition produced by a sample 3 : 1 M. Measure it using a scale and record the average of two diameters of each zone of inhibition[20]. Fig. 7(e) shows the size of the zone of inhibition formed around each disc, loaded with test samples, indicating the antibacterial activity towards the same for ZnO–CuO nanocomposite 3 : 1 M. The CuO doped ZnO NPs exhibited the antibacterial activity[21]. The antibacterial activity of the doped ZnO NPs is explained as follows. Firstly, the greater number of ROS is mainly ascribed to the small crystallite size of the NPs, and an increase in oxygen vacancies with the reactant molecules diffusivity. In the present antimicrobial investigation, the antibacterial effect of the ZnO NPs samples is mainly due to the generation of different factors such as ROS and the release of Zn2+. Since a ZnO composite with defects can be perturbed by UV–visible light, electron–hole pairs (e? and h+) are created. After that the first phenomenon is the generation of hydrogen peroxide (H2O2) from the ZnO surface, where the generation of highly reactive species such as OH?, H2O2 and O22? occurs. In the second phenomenon, the holes split up H2O molecules into OH? and H+. Then dissolved oxygen molecules are transformed to superoxide radical anions (?O2–), which in turn react with H+ to generate (HO2?) radicals, that upon subsequent collision with electrons produce hydrogen peroxide anions (HO2?). Therefore, they reacted with hydrogen ions to produce molecules of H2O2. Finally, the generated H2O2, which can penetrate the cell membrane by collapsing inter cell system bacteria, will be inhibited[22–24]. The CuO doped ZnO composite shows the highest antibacterial activity due to released highly active Cu2+ that can be responsible for the biocide activity of the CuO doped ZnO samples. The smaller crystallite size (ca. 33 nm) caused more antibacterial effects because it is well known fact that Cu2+ has a lower ionic radius (1.4 ?) than that of the host ion Zn2+ (1.39 ?). From the XRD patterns, the crystallite size CuO doped ZnO NPs are found to be 47 and 33 nm respectively[25].

class="figure_img" id="Figure7"/>
Download
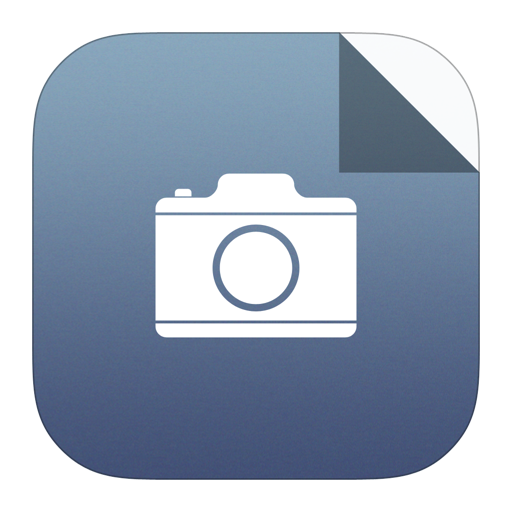
Larger image
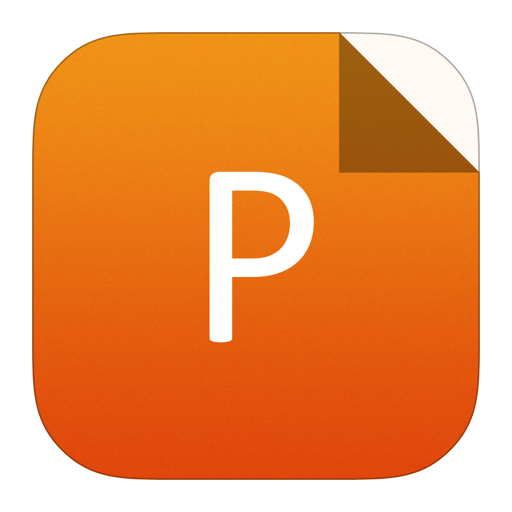
PowerPoint slide
Figure7.
(Color online) The photographic image of an inhibition zone produced by ZnO–CuO nanocomposite (3 : 1 M) for (a) Pseudomona saeruginosa, (b) Proteus mirabilis, (c) E.coli and (d) Staphylococcus aureus. (e) Bar graph representing the size of the zone of inhibition formed around each disc, loaded with test samples, indicating the antibacterial activity towards the same for ZnO–CuO nanocomposite 3 : 1 M.
4.
Conclusion
ZnO–CuO nanocomposite has been successfully synthesized through the MSP method by considering different molar concentration. The prepared phase was discovered with the help of X-ray diffraction (XRD), transmission electron microscopy (TEM), energy dispersive X-ray spectroscopy (EDAX), field emission scanning electron microscopy (FESEM) and atomic force microscopy (AFM). Also, some optical features were conducted by UV–vis and FT-IR techniques. The results of the XRD and TEM showed that the average particle size of ZnO particles increases with increasing molar concentration, and the FESEM results showed the formation of cluster and flakes like a nanocomposite. The EDX analysis confirmed the elemental compositions of the ZnO–CuO nanocomposite. The recorded AFM images show that the nature of the roughness varies for different molar concentrations in association with annealing temperature. Furthermore, the FTIR spectra show a broad absorption band related to Zn–O vibration as well as Cu–O vibration and the presence of OH groups. The band gaps of the zinc oxide nanoparticles were estimated from the UV–vis absorption curve. It was observed that the bandgap of the samples varied (i.e. 3.29 to 3.39 eV) for pure and synthesized ZnO samples. In the present investigation, CuO doped ZnO NPs exhibit effective antibacterial activity, and the zones of inhibition have been studied for four different bacteria. The presence of hydroxyl ions is essentially responsible for the enhanced antibacterial activities. The outcomes of antimicrobial activities revealed the maximum zone of inhibition against proteus mirabilis (20 mm for 20μL) and are followed by the other microorganisms. From the excellent antibacterial properties of the samples, the ZnO–CuO nanocomposite can be used as an efficient material for bacteria-free water systems. We hope that this work will be helpful in developing novel semiconductor based electronic and optical devices with excellent performance, low-cost and an effective way to get a new opportunity for ZnO–CuO based nanomaterials for the next generation of applications. Moreover, this explored synthesis technique can be effectively applicable to other complex oxides.