The rapid development of low-bandgap (LBG) nonfullerene acceptors and wide-bandgap (WBG) copolymer donors in recent years has boosted the power conversion efficiency (PCE) of organic solar cells (OSCs) to the 18% level[1-21]. The commercialization of OSCs is highly expected. However, critical issues like the cost and the stability also determine whether OSCs can enter the market or not[22]. Active materials, i.e. donors and acceptors, are the key materials determining the performance and cost of OSCs[23]. Nowadays, the state-of-the-art donors and acceptors like D18[4], PM6[24], Y6[3], IT-4F[25] and COi8DFIC[11] generally contain fluorine atoms, presenting high synthesis cost. Replacing fluorine with chlorine to make chlorinated donors or acceptors is an effective strategy to lower the cost while maintain the high efficiency for organic solar cells [26]. In the past few years, remarkable progress has been made in Cl-containing donors. In 2014, Wang et al. reported a chlorinated phenazine copolymer PCTClP with a low bandgap and a deep HOMO level[27]. Solar cells based on PCTClP and a fullerene acceptor PC71BM gave a PCE of 4.06%. In 2015, Pei et al. designed a chlorinated isoindigo copolymer Cl-IIDT[28]. Thanks to the chlorination, Cl-IIDT shows reduced crystallinity and a preferred face-on orientation, delivering a PCE of 4.60% in fullerene-based solar cells. In 2017, He et al. used monochlorinated benzothiadiazole unit as the building block to construct an asymmetric copolymer donor PBDTHD-ClBTDD[29]. The PBDTHD-ClBTDD:PC71BM cells afforded decent PCEs up to 9.11%. In the same year, Peng et al. developed an efficient small molecular donor BDTTS-Cl-R by introducing Cl atoms into the sulfuretted benzodithiophene unit[30]. BDTTS-Cl-R:PC71BM solar cells gave high PCEs up to 10.78%. In 2018, Hou et al. reported an efficient chlorinated copolymer PBDB-T-2Cl (also called PM7)[31]. Solar cells based on PBDB-T-2Cl and a nonfullerene acceptor IT-4F delivered a high PCE of 14.4% (certified 13.9%). In 2020, Yan et al. reported 17.04% efficiency solar cells by combining PM7 with a LBG nonfullerene acceptor Y6[32]. This is the highest efficiency from chlorinated-donor-based solar cells to date. Besides the works mentioned above, many research groups also made contributions in the development of chlorinated donors[33-43]. Previously, our group reported a highly efficient fluorinated copolymer donor D18, which delivered outstanding PCEs up to 18.22% (certified 17.6%) in Y6-based solar cells[4, 44]. In this work, we report the photovoltaic performance of a chlorinated analogue of D18, the D18-Cl (Fig. 1(a)). Compared with D18, D18-Cl shows a deeper HOMO level. This led to higher open-circuit voltages (Voc) for D18-Cl:Y6 solar cells. When combining D18-Cl with another LBG nonfulllerene acceptor N3[45], an outstanding PCE of 18.13% (certified 17.6%) was achieved, setting a new record for the chlorinated-donor-based organic solar cells.

class="figure_img" id="Figure1"/>
Download
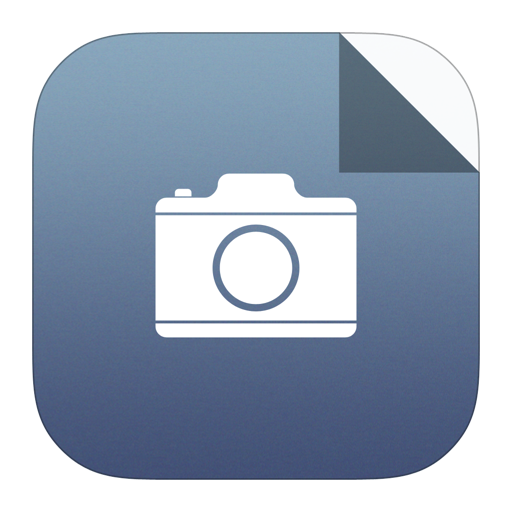
Larger image
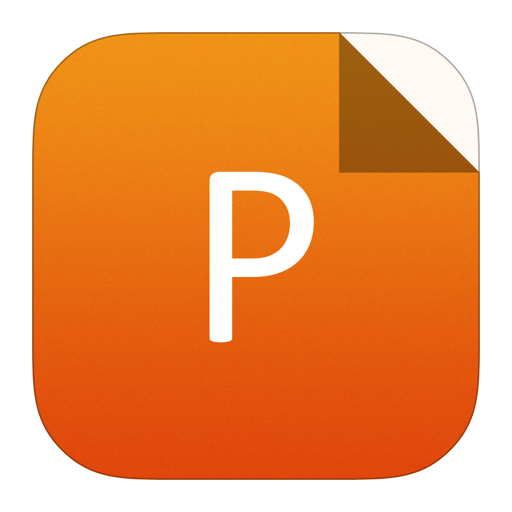
PowerPoint slide
Figure1.
(Color online) A highly efficient chlorinated copolymer donor D18-Cl. (a) The chemical structures for D18, D18-Cl, Y6 and N3. (b) Absorption spectra for D18-Cl solution, D18-Cl film, Y6 film and N3 film. (c) J–V curves for D18-Cl:Y6 and D18-Cl:N3 solar cells. (d) EQE spectra for D18-Cl:Y6 and D18-Cl:N3 solar cells. (e) Progress of chlorinated-donor-based OSCs.
D18-Cl was synthesized via the Stille copolymerization of the dithieno[3',2':3,4;2'',3'':5,6]benzo[1,2-c][1,2,5]thiadiazole (DTBT) monomer[4] with (4,8-bis(5-(2-ethylhexyl)-4-chlorothiophen-2-yl)benzo[1,2-b:4,5-b']dithiophene-2,6-diyl)bis(trimethylstannane) (Scheme S1). After Soxhlet extraction, D18-Cl was obtained in 82% yield. The number-average molecular weights (Mn) and the polydispersity index (PDI) for D18-Cl are 102.7 kDa and 1.95, respectively. D18-Cl is soluble in chloroform and chlorobenzene. The normalized absorption spectra for D18-Cl in chloroform and as thin film are shown in Fig. 1(b). In solution, D18-Cl shows an absorption band at 410–640 nm, with a peak at 574 nm. The D18-Cl film shows two peaks at 535 and 568 nm, respectively. The additional peak at 535 nm suggests the H-aggregation tendency of D18-Cl in solid state[46]. The optical bandgap (Egopt) estimated from the absorption onset of D18-Cl film is 1.99 eV, which is similar to that of D18 (1.98 eV)[4]. The absorption spectra for the acceptors Y6 and N3 are also shown in Fig. 1(b). They are well complementary with that of D18-Cl. The frontier orbital energy levels of D18-Cl were estimated from cyclic voltammetry (CV) measurements (Fig. S2). The HOMO and LUMO energy levels for D18-Cl are –5.56 and –2.78 eV, respectively. Compared with D18[4], D18-Cl shows deeper HOMO and LUMO levels. We also investigated the frontier orbital energy levels by using density functional theory (DFT) calculation. As shown in Fig. S3, the DFT-predicted HOMO and LUMO levels are –4.94 and –2.59 eV for D18, and –4.97 and –2.61 eV for D18-Cl, respectively.
Next, we evaluated the performance of D18-Cl by fabricating conventional solar cells with a structure of ITO/PEDOT:PSS/D18-Cl:Y6 (or D18-Cl:N3)/PDIN/Ag. The D/A ratio, active layer thickness and additive content for the cells were optimized. The best D18-Cl:Y6 solar cells gave a PCE of 17.12%, with a Voc of 0.863 V, a short-circuit current density (Jsc) of 27.08 mA cm–2, and a fill factor (FF) of 73.3% (Fig. 1(c)). These cells have a D/A ratio of 1 : 1.4 (w/w), an active layer thickness of 108 nm and 0.5 vol% diphenyl ether (DPE) as the additive (Tables S1–S3). Compared with the reported D18:Y6 cells[4], D18-Cl:Y6 cells gave slightly higher Voc due to the deeper HOMO level of D18-Cl. On the other hand, the best D18-Cl:N3 solar cells afforded a higher PCE of 18.13%, with a Voc of 0.859 V, a Jsc of 27.85 mA cm–2, and a FF of 75.7%. These cells have a D/A ratio of 1 : 1.4 (w/w), an active layer thickness of 112 nm and 0.5 vol% DPE as the additive (Tables S4–S6). The best D18-Cl:N3 solar cells were also measured at the National Institute of Metrology (NIM) (Beijing), and a certified PCE of 17.6% (Voc, 0.843 V; Jsc, 27.13 mA cm–2; FF, 76.8%; effective area, 2.580 mm2) was recorded (Fig. S4). This efficiency is comparable to that of D18:Y6 solar cells[4]. It should be noted that different from D18:Y6 cells needing solvent-annealing treatment, no post-treatment is needed for D18-Cl:N3 cells. The external quantum efficiency (EQE) spectra for D18-Cl:Y6 and D18-Cl:N3 solar cells are shown in Fig. 1(d). The D18-Cl:N3 cells afford >80% EQE at 440–820 nm, with a maximum of 89% at 550 nm. The D18-Cl:Y6 cells show lower EQE, with a maximum EQE of 85% at 530 nm. The integrated current densities for D18-Cl:Y6 and D18-Cl:N3 solar cells are 25.92 and 26.86 mA cm–2, respectively. To the best of our knowledge, the 18.13% efficiency is the highest value achieved from chlorinated-donor-based solar cells so far (Fig. 1(e)).
To understand why D18-Cl:N3 solar cells gave higher Jsc and FF than D18-Cl:Y6 cells, we studied the charge generation, transport and recombination in both devices. As shown in Fig. S5, the exciton dissociation probabilities (Pdiss) for the D18-Cl:Y6 and D18-Cl:N3 cells are 97.4% and 98.4%, respectively, indicating more efficient charge generation in the latter. Hole and electron mobilities (μh and μe) were measured by using space charge limited current (SCLC) method (Figs. S6–S8 and Table S7)[47, 48]. The D18-Cl pure film shows a high μh of 1.00 × 10–3 cm2 V–1 s–1. For blend films, D18-Cl:N3 film gave a higher μh of 6.09 × 10–4 cm2 V–1 s–1, a higher μe of 3.83 × 10–4 cm2 V–1 s–1, and a smaller μh/μe of 1.59 than D18-Cl:Y6 film. This suggests the faster and more balanced charge transport in D18-Cl:N3 film. We studied the bimolecular recombination by plotting Jsc against light intensity (Plight) (Fig. S9)[49–53]. D18-Cl:N3 cells showed a α value of 0.980, which is closer to 1 as compared to that of D18-Cl:Y6 cells (0.975), suggesting less bimolecular recombination in D18-Cl:N3 cells. The more efficient charge generation, faster and more balanced charge transport, and the suppressed bimolecular recombination should account for the higher Jsc and FF of D18-Cl:N3 cells.
The morphology for D18-Cl:Y6 and D18-Cl:N3 blend films was studied by using atomic force microscope (AFM) (Fig. S10). D18-Cl:Y6 and D18-Cl:N3 films without DPE additive presented smooth surface and unique nanostructures. After adding 0.5 vol% DPE, both films became rougher. The root-mean-square roughnesses (Rrms) increased from 0.82 to 1.52 nm for D18-Cl:Y6 film, and from 0.79 to 1.22 nm for D18-Cl:N3 film, respectively. Since the addition of DPE enhanced Jsc and FF for both D18-Cl:Y6 and D18-Cl:N3 solar cells (Tables S3 and S6), such morphological changes might improve the phase separation and favor to build up more efficient charge-transporting networks at the nanoscale, thus facilitating charge transport and suppressing charge recombination.
In summary, we developed a highly efficient chlorinated copolymer donor D18-Cl. Compared with D18, D18-Cl presents deeper HOMO and LUMO energy levels. D18-Cl:N3 solar cells delivered a PCE of 18.13%, which is the highest efficiency for chlorinated-donor-based OSCs to date. This work demonstrates the great potential of cost-effective chlorinated polymer donors for OSCs.
Acknowledgements
We thank the National Key Research and Development Program of China (2017YFA0206600), the National Natural Science Foundation of China (51773045, 21772030, 51922032, 21961160720, 62074022), Fundamental Research Funds for the Central Universities (2020CDJQY-A055) and the Youth Association for Promoting Innovation (CAS) for financial support.
Appendix A. Supplementary materials
Supplementary materials to this article can be found online at