Bulk-heterojunction polymer solar cells (PSCs) as a clean and renewable energy resource have attracted great attention from both academia and industry[1-20]. Recently non-fullerene PSCs based on polymer donors (PDs) and small molecule acceptors (SMAs) have achieved remarkable success with the power conversion efficiencies (PCEs) over 18%[21-26]. Among various PSCs, all-polymer solar cells (all-PSCs) consist of PDs and polymer acceptors (PAs), showing unique merits including superior stability and mechanical robustness. However, the development of all-PSCs lag behind SMAs-based PSCs due to the scarcity of high-performance PAs[6].
The first all-PSCs can be traced back to 1995[27]. However, only ten years later, aromatic-imide-based PAs got achievements in all-PSCs. After the first report of perylene diimide (PDI) PA named PDI-DTT[28] by Zhan et al. in 2007 (Fig. 1, Table 1), PDI and naphthalene diimide (NDI) derivatives began to dominate PAs. Among them, a NDI-based copolymer PNDIOD-T2 (a.k.a. N2200) opened a new era for PAs research[29, 30]. With tailored PDs and device engineering[5, 31], steady progress has been achieved in all-PSCs based on N2200 and its derivatives. A PCE of 11.76% was realized in 2020[32]. However, a major drawback for PDI and NDI PAs is their low absorption coefficient in the NIR region due to the steric-hindrance-induced large torsion angles between NDI/PDI core and co-units, thus limiting the short-circuit current density (Jsc)[33, 34]. Moreover, their low LUMO energy levels led to relatively low open-circuit voltage (Voc). Other electron-deficient building blocks like diketopyrrolopyrrole (DPP), isoindigo (IID), bithiophene imide (BTI), thieno[3,4-c]pyrrole-4,6-dione (TPD) and B←N bridged bipyridine (BNBP) unit were also explored for PAs[35]. BTI derivative SPA2 and BNBP derivative PBN-12 were the representatives, affording 9.21% and 10.07% PCEs with >1 V Voc in all-PSCs, respectively[36, 37]. However, these acceptors suffer from the same defects as NDI and PDI PAs. Specifically, SPA2 and PBN-12 show weak light-harvesting capability with absorption onsets below 700 nm. In this regard, Guo et al. developed a strong electron-withdrawing building block 5,6-dicyano-2,1,3-benzothiadiazole (DCNBT)[38, 39]. PAs based on DCNBT show narrow bandgap (1.28 eV) with high absorption coefficient in the NIR region. A PCE of 12.1% was achieved in a recent work[38]. Higher efficiencies from DCNBT-based PAs are anticipated through further morphology and device optimization.

class="figure_img" id="Figure1"/>
Download
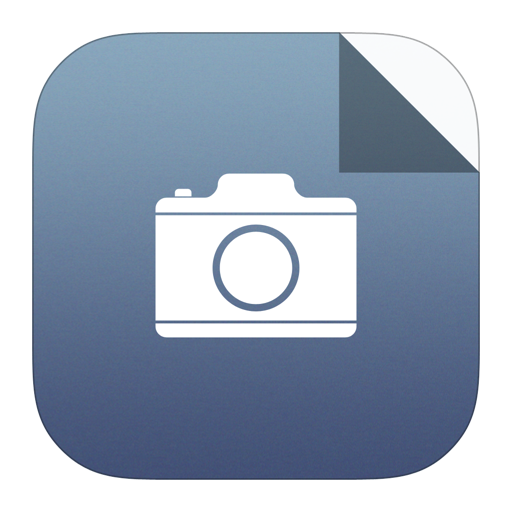
Larger image
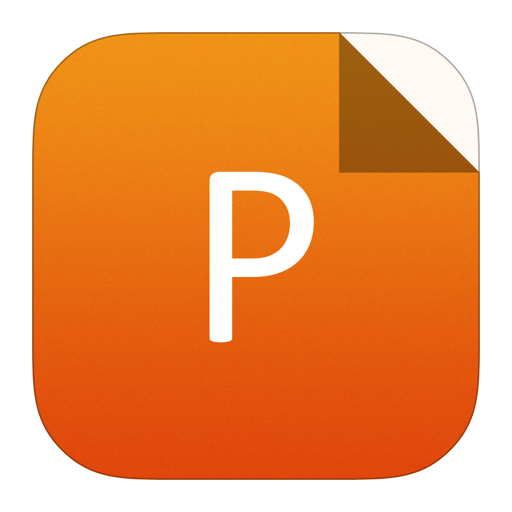
PowerPoint slide
Figure1.
High-performance polymer acceptors. (Note: the names in the parentheses indicate the polymer donors.)
Acceptor | Donor | Voc (V) | Jsc (mA/cm2) | FF (%) | PCEmax (%) | Ref. |
PDI-DTT | PTA | 0.63 | 4.2 | 39.0 | 1.5 | [28] |
N2200 | PTzBI-Si | 0.88 | 17.62 | 75.78 | 11.76 | [32] |
SPA2 | PTB7-Th | 1.02 | 15.16 | 59.4 | 9.21 | [36] |
PBN-12 | CD1 | 1.17 | 13.39 | 64.0 | 10.07 | [37] |
DCNBT-TPC | PTB7-Th:PBDB-T | 0.81 | 21.9 | 68.3 | 12.1 | [38] |
PZ1 | PBDB-T | 0.83 | 16.05 | 68.99 | 9.19 | [40] |
L14 | PM6 | 0.96 | 20.6 | 72.1 | 14.3 | [45] |
PY-IT | PM6 | 0.933 | 22.30 | 72.3 | 15.05 | [43] |
PZT-γ | PBDB-T | 0.896 | 24.7 | 71.3 | 15.8 | [44] |
PYT:BN-T | PM6 | 0.955 | 22.65 | 74.3 | 16.09 | [46] |
PY2F-T:PYT | PM6 | 0.90 | 25.2 | 76.0 | 17.2 | [47] |
Table1.
Performance data for the polymer acceptors.
Table options
-->

Download as CSV
Acceptor | Donor | Voc (V) | Jsc (mA/cm2) | FF (%) | PCEmax (%) | Ref. |
PDI-DTT | PTA | 0.63 | 4.2 | 39.0 | 1.5 | [28] |
N2200 | PTzBI-Si | 0.88 | 17.62 | 75.78 | 11.76 | [32] |
SPA2 | PTB7-Th | 1.02 | 15.16 | 59.4 | 9.21 | [36] |
PBN-12 | CD1 | 1.17 | 13.39 | 64.0 | 10.07 | [37] |
DCNBT-TPC | PTB7-Th:PBDB-T | 0.81 | 21.9 | 68.3 | 12.1 | [38] |
PZ1 | PBDB-T | 0.83 | 16.05 | 68.99 | 9.19 | [40] |
L14 | PM6 | 0.96 | 20.6 | 72.1 | 14.3 | [45] |
PY-IT | PM6 | 0.933 | 22.30 | 72.3 | 15.05 | [43] |
PZT-γ | PBDB-T | 0.896 | 24.7 | 71.3 | 15.8 | [44] |
PYT:BN-T | PM6 | 0.955 | 22.65 | 74.3 | 16.09 | [46] |
PY2F-T:PYT | PM6 | 0.90 | 25.2 | 76.0 | 17.2 | [47] |
In 2017, the strategy of “polymerizing SMAs” was proposed by Zhang et al.[40] SMAs were introduced as electron-deficient building blocks to construct new-generation PAs. These polymerized SMAs (PSMAs) not only inherit the merits like narrow bandgap, strong absorption and tunable energy levels from SMAs, but also present good film-forming capability and photostability of the polymers[41]. The first PSMA named PZ1 was synthesized by copolymerizing IDIC-C16 with thiophene unit, showing a high extinction coefficient (>105 cm?1) and yielding a PCE of 9.19% in all-PSCs[40]. Afterwards, a wide variety of SMAs and different conjugated linkage units were selected to tune the physicochemical and photovoltaic properties of PSMAs. A few high-performance PSMAs were designed and synthesized by several groups, enabling all-PSCs with PCEs over 14% (Fig. 1)[42]. Notably, the widely used end groups in SMAs are a mixture of two isomers with similar polarity, thus resulting in isomeric issues in PSMAs. This brings a negative effect to the batch-to-batch reproducibility of the PSMAs, leading to a large deviation in device performance. Luo et al.[43] reported a regioregular PSMA named PY-IT by using isomerically pure end groups, which enabled the PCE of all-PSCs exceeding 15% for the first time, significantly higher than its regiorandom counterparts. The higher performance resulted from enhanced absorption, more balanced charge transport and favorable morphology. Fu et al.[44] synthesized a new regioregular PSMA PZT-γ by replacing the benzothiadiazole moiety with benzotriazole. The PZT-γ-based all-PSCs exhibited a high PCE of 15.8%. To improve the electron mobility of PAs, Guo et al.[45] combined “polymerizing SMAs” and “acceptor–acceptor” (A–A) strategies to develop a new PSMA L14 by copolymerizing the distannylated BTI with a brominated SMA. The A–A type backbone renders L14 an enhanced electron mobility. L14 achieved an efficiency of 14.3%, which is a record value for all-PSCs based on A–A type PAs. The success of PSMA-based binary all-PSCs triggered the further exploration of efficient ternary all-PSCs. An impressive work is the incorporation of a B←N-based PA into PM6:PY-IT host blend by Liu et al.[46]. A PCE of 16.09% was achieved due to an optimal morphology and reduced nonradiative energy loss in ternary cells. Very recently, Min et al. reported a 17.2% efficiency from ternary all-PSCs with two well-compatible PSMAs[47].
Overall, remarkable progress has been made in all-PSCs in terms of materials diversity and device performance. Over 17% PCEs have been demonstrated. In addition, the energy losses in the devices can reach 0.5 eV or less, indicating that the theoretical limit is comparable to that of SMA-based cells. It should be noted that all-polymer blend films typically show poor nanoscale phase separation due to the long and intertwined polymer chains, thus leading to low FFs. Hence, more efforts in developing narrow bandgap PAs with high electron mobility and optimizing film morphology should be made.
Acknowledgements
We thank the Natural Science Foundation for Distinguished Young Scholars of Guangdong Province (2021B1515020027), the National Natural Science Foundation of China (21801124 and 21774055) and Shenzhen Science and Technology Innovation Commission (JCYJ20180504165709042). L. Ding thanks the National Key Research and Development Program of China (2017YFA0206600) and the National Natural Science Foundation of China (51773045, 21772030, 51922032, 21961160720) for financial support.