Organic solar cell (OSC) is a promising photovoltaic technology with great commercialization potential due to the advantages like solution-processing, roll-to-roll fabrication, lightweight, flexibility, and semitransparency[1-7]. Conjugated polymer donors are key materials for OSCs[8]. Their functions include harvesting photons, constructing a 3D interpenetrating network in the active layer for efficient exciton dissociation, and transporting holes to the anode[9-11]. Copolymers containing fused-ring acceptor units (i.e. electron-accepting building blocks) are the most efficient type of donors for OSCs. The copolymer donors, such as Li’s J71[12] and PTQ10[13], Hou’s PBDB-T[14], PM6[15] and PM7[16], Sun’s PDBT-T1[17] and PBT1-C[18], Yu’s PTB7[19], Chen’s PTB7-Th[20], You’s FTAZ[21], Janssen’s PDPP3T[22], Yan’s PffBT4T-C9C13[23], Huang’s NT812[24] and P2F-EHp[25], Ding’s D18[26] and D18-Cl[27], all contain fused-ring acceptor units. The merits for fused-ring acceptor units are prominent: 1) the strong electron-withdrawing capability of these units gifts polymers deep HOMO (the highest occupied molecular orbital) levels, leading to high open-circuit voltage (Voc); 2) the rigid and extended molecular planes of these units gift polymers enhanced π–π stacking and good hole mobility, leading to high short-circuit current density (Jsc) and fill factor (FF). In the past decade, our group has been developing fused-ring-acceptor-unit-based polymer donors (Fig. 1).

class="figure_img" id="Figure1"/>
Download
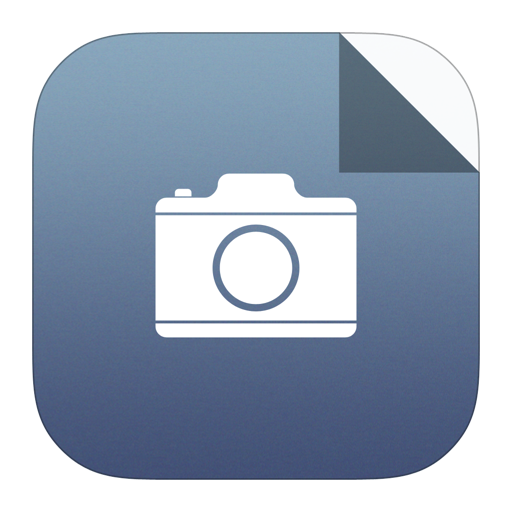
Larger image
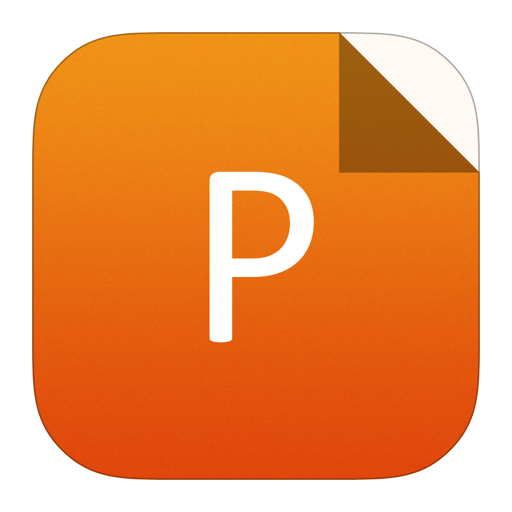
PowerPoint slide
Figure1.
(Color online) Polymer donors with fused-ring acceptor units developed in Ding’s lab.
Fused-ring ketones are frequently-used acceptor units for polymer donors due to their strong electron-withdrawing property. In 2012, we reported donor–acceptor (D–A) copolymers P1[28] and P2[29] based on thiophene-fused ketone units benzo[1,2-b:4,5-c′]-dithiophene-4,8-dione and cyclopenta[2,1-b:3,4-c′]dithiophene-4-one, respectively. 4.33% and 2.20% PCEs were obtained from P1:PC61BM and P2:PC71BM solar cells, respectively. Fused-ring lactams are another important building blocks. The lactam moieties endow the unit with electron affinity and offer the sites for introducing alkyl chains to guarantee good solubility. In 2013, we first reported pentacyclic aromatic bislactam unit TPTI[30]. By copolymerizing TPTI with a thiophene unit, we got a donor PThTPTI. PThTPTI:PC71BM solar cells gave a PCE of 7.80% with a high Voc of 0.87 V. PThTPTI was among the best polymer donors at that time. Following this work, we designed a hexacyclic bislactam unit TD2 and used it to construct a selenophene-containing copolymer donor PSeTD2 in 2015[31]. Inverted PSeTD2:PC71BM solar cells offered a PCE of 8.18%, which was a record for D–A copolymers with selenophene as the donor unit. Later on, we designed a bislactam unit BDTP[32]. The structure of BDTP can be viewed as dividing TD2 into two tricyclic dithieno[3,2-b:2′,3′-d]pyridin-5(4H)-one (DTP) units and connecting them with a single bond. The D–A copolymer PThBDTP based on BDTP exhibited deep HOMO levels, good hole mobility and excellent performance in solar cells when blending with PC71BM. PThBDTP:PC71BM solar cells gave a PCE of 9.13% with a Voc of 0.96 V. PThBDTP was one of the few D–A copolymers with PCEs of over 9% at that time. After this work, we considered the tricyclic lactam DTP could be a useful building block for copolymer donors. In 2017, we reported a low-bandgap copolymer PDTP4TFBT based on DTP[33]. PDTP4TFBT is compatible not only with fullerene acceptors but also with nonfullerene acceptors. Blending PDTP4TFBT with the fullerene acceptor PC71BM or the nonfullerene acceptor ITIC[34] yielded 8.75% and 7.58% PCEs, respectively. The ternary cells based on PDTP4TFBT:PC71BM:ITIC (1 : 1.2 : 0.3) delivered a PCE of 9.20%. In 2018, we designed an analogue of DTP, thieno[3,2-c]isoquinolin-5(4H)-one (TIQ), by replacing one thiophene of DTP with a benzene[35]. TIQ-based D–A copolymer PTIQ4TFBT has a high hole mobility of 1.33 × 10–3 cm2 V–1 s–1 and gave a PCE of 10.16% in polymer:fullerene solar cells with an active layer thickness of 267 nm. Moreover, the thick-film PTIQ4TFBT solar cells kept over 8% PCE with active layer thickness over 500 nm. Regarding the rapid advance of nonfullerene acceptors in recent years, developing efficient donors matching nonfullerene acceptors became an imperative mission. Thus, we started focusing on the development of nonfullerene-acceptor-compatible polymer donors. In 2019, we reported a wide-bandgap copolymer PBD2T based on DTP[36]. Solar cells based on PBD2T and a nonfullerene acceptor IT-M[37] afforded PCEs up to 10.34%. The good performance of PBD2T stimulated our exploration for other fused-ring-acceptor-unit-based polymer donors. In the same year, we first reported the fused-ring-lactone-based polymer donors[38]. Thanks to the strong electron-withdrawing capability and rigid molecular plane of the tricyclic lactone unit 5H-dithieno[3,2-b:2′,3′-d]pyran-5-one, the resulting polymer L1 presents a deep HOMO level and good mobility. Inverted solar cells based on L1 and a nonfullerene acceptor Y6[39] gave PCEs up to 14.36%. Later on, we designed the tricyclic thiolactone unit 5H-dithieno[3,2-b:2′,3′-d]thiopyran-5-one (DTTP), and used it to synthesize the copolymer D16[40]. From L1 to D16, the simple replacement of lactone with thiolactone improves polymer π–π stacking. D16:Y6 solar cells gave PCEs up to 16.72% (certified 16.0%). In 2020, we designed copolymer D18 by using a fused-ring thiadiazole unit dithieno[3′,2′:3,4;2′′,3′′:5,6]benzo[1,2-c][1,2,5]thiadiazole (DTBT)[26]. Compared with DTTP, DTBT has a larger molecular plane and gifts D18 a high hole mobility of 1.59 × 10–3 cm2 V–1 s–1. D18:Y6 solar cells demonstrated an outstanding PCE of 18.22% (certified 17.6%), which was the highest efficiency for OSCs at that time. Recently, we reported a chlorinated analogue of D18, the D18-Cl[27]. D18-Cl also showed high performance in solar cells. Blending D18-Cl with a nonfullerene acceptor N3[41] yielded a PCE of 18.13% (certified 17.6%), demonstrating the great potential of cost-effective chlorinated polymer donors in OSCs. We also tried other fused-ring acceptor units with larger molecular planes to make polymer donors. In 2020, we reported a donor P3 based on a fused-ring phenazine unit 9,10-difluorodithieno[3,2-a:2′,3′-c]phenazine (FDTPz)[42]. P3:Y6 solar cells gave PCEs up to 15.14%, which is the highest efficiency from phenazine-based donors to date. In the same year, we reported polymer donors P4 and P5 based on two novel pentacyclic bislactone units, BL1 and BL2[43]. Compared with previous lactone units, BL1 and BL2 have stronger electron-withdrawing capability and gift P4 and P5 deeper HOMO levels. P4:IT-4F and P5:IT-4F solar cells gave high Vocs of 0.96 and 0.94 V, respectively, and PCEs of 10.44% and 7.31%, respectively.
Among all the polymer donors invented in our lab, D18 exhibited high hole mobility and outstanding performance. The grazing-incidence wide-angle X-ray scattering (GIWAXS) for D18 neat film or blend film shows distinct (001) and (002) diffraction peaks[44]. Besides common π–π stacking and lamellar stacking ordering, D18 also shows unusually strong backbone direction ordering. This may explain its excellent hole mobility and photovoltaic performance. The high hole mobility of D18 suggests that it could be applied in thick-film solar cells. Recently, we made thick-film solar cells by adding small amount of PC61BM into the D18:Y6 blend[45]. D18:Y6:PC61BM (1 : 1.6 : 0.2) solar cells afforded a PCE of 17.89% (certified 17.4%) at the thickness of 110 nm, and offered 16.32% and 16.19% PCEs at the thickness of 300 and 350 nm, respectively. To the best of our knowledge, this is the first report that OSCs gave over 16% PCEs with active layer thickness over 300 nm. To further explore the potential of D18, we screened a series of nonfullerene acceptors and found that N3 is a good partner for D18. The solar cells with a structure of ITO/PEDOT:PSS/D18:N3/PDIN/Ag delivered a PCE of 18.56%, with a Voc of 0.862 V, a Jsc of 27.44 mA cm–2 and a FF of 78.5% (Fig. S1), setting a new world record of PCE for organic solar cells. These cells have a D/A ratio of 1 : 1.6 (w/w), an active layer thickness of 121 nm and 0.15 vol% 1-chloronaphthalene (CN) as the additive (Tables S1–S3). D18:N3 solar cells presented >80% EQE at 450–810 nm, showing a maximum of 89% at 540 nm (Fig. S2). The integrated current density for D18:N3 solar cells is 26.61 mA cm –2. The best D18:N3 solar cells were also measured at the National Institute of Metrology (NIM) (Beijing), and a certified PCE of 17.9% was recorded (Voc, 0.850 V; Jsc, 26.71 mA cm–2; FF, 78.9%; effective area, 2.580 mm2) (Fig. 2).

class="figure_img" id="Figure2"/>
Download
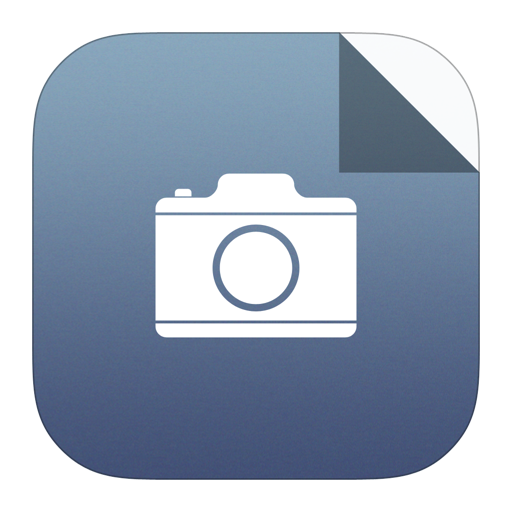
Larger image
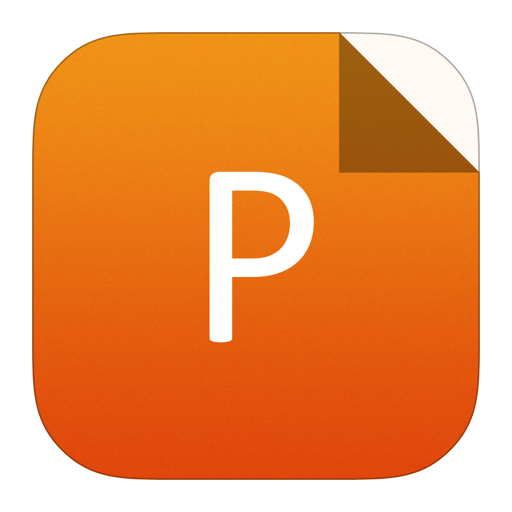
PowerPoint slide
Figure2.
NIM (Beijing) report for D18:N3 solar cells.
Since 2010, we have been devoting our efforts in inventing fused-ring-acceptor-unit-based copolymer donors. Some copolymer donors present high performance not only in fullerene-acceptor-based solar cells but also in nonfullerene-acceptor-based solar cells. Among them, D18 demonstrates the highest PCE (18.56%) in OSCs. We are expecting that more innovative building blocks will be synthesized, and we believe more high-performance donors and acceptors are coming. With the innovative contribution and synergetic efforts from OSC community, OSC products will eventually go to market.
Acknowledgements
We thank the National Key Research and Development Program of China (2017YFA0206600), the National Natural Science Foundation of China (51773045, 21772030, 51922032 and 21961160720) and the Youth Association for Promoting Innovation (CAS) for financial support.
Appendix A. Supplementary materials
Supplementary materials to this article can be found online at