1.
Introduction
N-type Si solar cells are of great interest for widespread photovoltaic applications. They exhibit many advantages compared to p-type Si substrates, such as higher minority carrier diffusion lengths, higher tolerance to metallic impurities and immunity from boron-oxygen related light-induced degradation[1–7]. In general, these superior properties allow n-type Si solar cells to attain higher photovoltaic conversion efficiencies. Nowadays, utilizing boron-doped (p+) emitters in n-type Si solar cells, by the incorporation of boron atoms into the Si wafer, has become a preferred method of forming the necessary p–n junction. There are several technologies for boron-doped (p+) emitter formation in n-type Si solar cells[8–11]. Firstly, this can be implemented by depositing (e.g. PECVD or APCVD) boron doped a-SiOx and subsequently exposing it to high temperature[12–14]. Secondly, boron can be incorporated by ion implantation followed by high-temperature annealing[15, 16]. Thirdly, a more promising technology is direct thermal diffusion of boron from a boron trichloride (BCl3)[17] or BBr3 source. Thermal gas-phase diffusion by BBr3 uses a conventional furnace with high throughput and low cost of ownership. However, the SiO2/B2O3 stack, also known as boron silicate glass (BSG), is often formed before the boron dopant is driven-in. When temperature is above 900 °C, the BBr3 diffusion takes place to allow the working of both the interstitial and vacancy diffusion mechanisms of boron[18]. In this process, an unintentional supersaturated boron-rich layer (BRL) on top of the p+ emitter is formed, due to the higher solubility of B in SiO2 than in Si during diffusion[19]. It is conjectured that the BSG and BRL act as high recombination sites due to inactive boron, segregated metal impurities and structural defects, resulting in lifetime degradation[20–23]. Therefore, these undesirable layers of both BSG and BRL must be removed after the diffusion step and replaced by a suitable passivation layer in order to obtain good surface passivation.
In this work, BRL removal by different treatment methods has been fully investigated in boron-doped p+-n Si solar cells. Our proposed chemical etch treatment (CET) demonstrates an effective way to remove the BRL by using a mixture of nitric acid (HNO3), glacial acetic acid (CH3COOH) and hydrofluoric acid (HF) (HF : HNO3 : CH3COOH = 1 : 100 : 100). After the CET, surface passivation is further needed for performance improvement of the boron-doped p+-n Si solar cells. By comparison with other treatments, it is found that the CET method for BRL removal has achieved an implied Voc of 680 mV in combination with Al2O3/SiNx stack passivation formed by ALD and PECVD. In addition, both sheet resistances and junction depth have been analyzed for possible dependence on the BRL removal of boron-doped p+-n Si solar cells.
2.
Experimental
Two-inch high-lifetime Czochralski (CZ) n-type Si wafers with a resistivity of 3 Ω·cm and thickness of 300 μm were used. Prior to boron diffusion, a conventional RCA clean was performed on all the Si wafers to get rid of the contamination on the substrate surface. The wafer was then rinsed in diluted HF to remove native oxide on the surface. Shortly after, the clean wafers were placed into a BBr3-based open-tube furnace and boron diffusion was immediately performed for boron doping in n-type Si substrate. The whole process of boron diffusion was divided into two sequences: the pre-deposition and the drive-in process. In the pre-deposition, a mixture gas composed of oxygen (O2), nitrogen (N2) and BBr3 vapor entered the furnace at temperature of 900 °C for a duration of 45 min. Boron is dissociated from the BBr3 molecule and forms a B2O3 layer on the Si wafer surface according to the chemical reaction of Eq. (1). At the hetero-interface, the B2O3 reacts with Si to generate SiO2, and simultaneously releases elementary boron which can then diffuse into the bulk Si wafer following Eq. (2).
$$ 4 { m{BBr}}_3 + 3{ m{ O}}_2 to 2{{ m{B}}_2}{{ m{O}}_3} + 6{ m{ Br}}_2,$$ ![]() | (1) |
$$ 2{{ m{B}}_2}{{ m{O}}_3} +3 { m{Si }} to 4{ m{ B}} + 3{ m{ SiO}}_2 .$$ ![]() | (2) |
The pre-deposition process was kept the same for all the wafers, which were divided into three groups labeled groups I, II and III. The drive-in process was then performed under different atmospheres at 950 °C for 25 min. The wafers of groups I and II were driven-in under the nitrogen ambient while those of group III were driven-in under an ambient of N2 and O2 mixture gas with O2 flow of 50, 80 and 110 sccm respectively. Furthermore, different treatments were used for group I, II and III wafers before surface passivation of the SiNx/Al2O3 stack. The group I samples received a CET method using a mixture of HNO3, CH3COOH and HF (HF : HNO3 : CH3COOH = 1 : 100 : 100) for a duration of 50, 90, 120 or 150 s. The group II samples were treated in boiling nitric acid (TBNA) for 40 min. The group III samples were treated in a 2 % HF dip to remove the BSG. After different surface treatments, all the wafers were passivated by Al2O3 (~15 nm) and SiNx (~70 nm) stack using ALD and PECVD. Finally, an annealing process at 450 °C in FGA ambient was carried out to activate the passivation. After sample preparation, all the wafers were measured to obtain the open-circuit voltage (Voc) parameter by the quasi-steady state photoconductivity technique in the SintonWCT-120 lifetime measurement instrument. In order to further compare the effect of different treatment methods on BRL removal, the cross-sectional microstructure of the boron-doped region before Al2O3/SiNx stack passivation was examined using high-resolution transmission electron microscopy (HRTEM) while the effect of surface passivation of Al2O3/SiNx stack on boron-doped p+–n Si solar cells with and without BRL was investigated.
3.
Results and discussion
Since both surface passivation and bulk lifetime are the key factors leading to high-efficiency cells, the BRL should be removed to attain high-efficiency n-type Si solar cells. It is found that sufficient O2 in the furnace during the thermal diffusion process could eliminate any potential residual BRL, while excessive O2 concentration results in boron depletion and a higher sheet resistance. Therefore, oxidation of heavily boron-diffused Si wafers after the drive-in process, can be used to remove or consume the BRL[24]. For samples from group III, the wafers were driven-in in an ambient of N2 and O2 mixture gas with different O2 flow of 50, 80, and 110 sccm. As shown in the results in Fig. 1, it is noted that the implied Voc decreased with increasing the O2 flow, which proves that BRL oxidation can be damaging to the high-quality surface passivation of boron-diffused surfaces. A detrimental effect on high-efficiency n-type Si solar cells is consistent with some early reports, which pointed out that segregated iron (Fe and Cr) impurities in the BRL can be re-injected from the p+ emitter into Si during the BRL oxidation[23, 25, 26]. Therefore, the O2 concentration during the diffusion process must be well-controlled and an alternate method for BRL removal is also needed in order to achieve high-efficiency n-type Si solar cells.

class="figure_img" id="Figure1"/>
Download
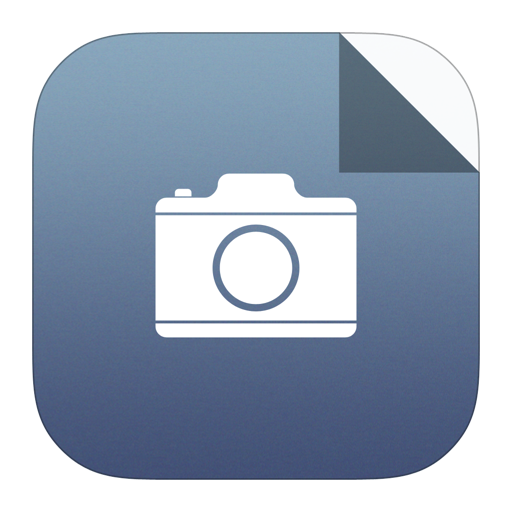
Larger image
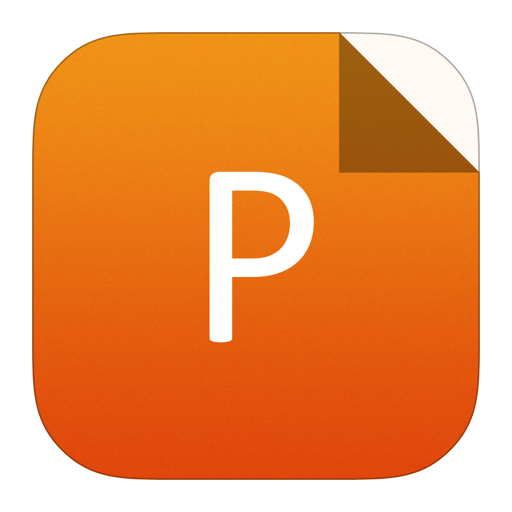
PowerPoint slide
Figure1.
(Color online) The implied Voc of group III samples driven-in under different O2 flows of 50, 80, and 110 sccm, respectively.
Furthermore, we investigated three methods of removing the BRL and compared the surface passivation quality of boron-diffused surfaces. The cross-sectional microstructure of the boron doped region before Al2O3/SiNx stack passivation is examined using HRTEM. Fig. 2 shows the HRTEM images of the boron-diffused samples with and without the BRL. A very thin dark layer (~10 nm) with periodic agglomeration, indicated by the blue arrow in Fig. 2(a), was observed at the Si/BSG interface in the sample with BRL. However, the p+ emitter without BRL showed a relatively clean interface with almost no dark thin layer or agglomeration in Figs. 2(b), 2(c) and 2(d). These agglomerations are often associated with impurities and structural defects in the crystal lattice that can give rise to recombination centers[27]. We can therefore draw the conclusion that all these methods are beneficial to eliminating potential residual BRL. Fig. 3 shows a positive synergistic effect of passivating the chemically etched p+ emitter surface with a Al2O3/SiNx stack layer. It is seen that Al2O3/SiNx stack passivation for the boron emitter, more so than only Al2O3 passivation, markedly increases the implied Voc from 630 to 680 mV by etching BRL.

class="figure_img" id="Figure2"/>
Download
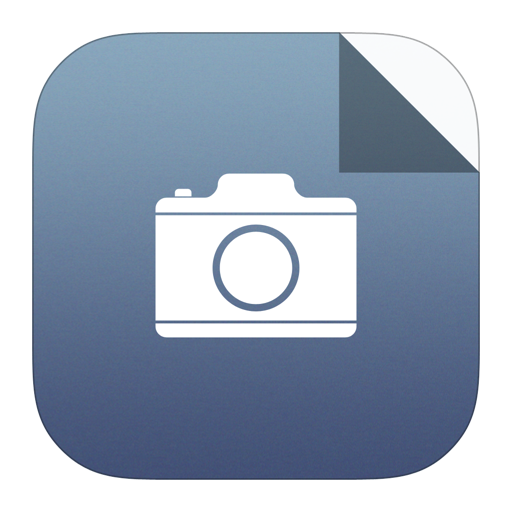
Larger image
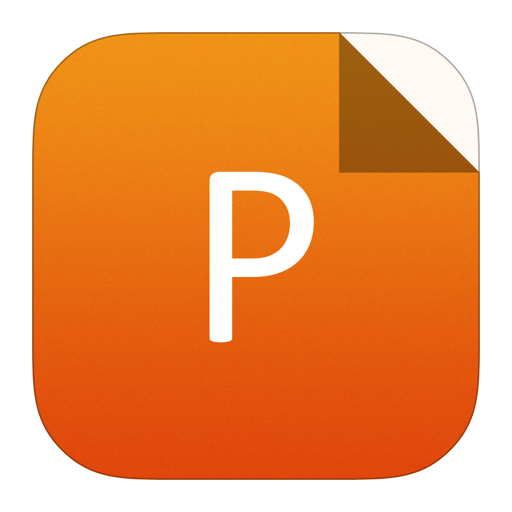
PowerPoint slide
Figure2.
(Color online) HRTEM image of boron doped region before passivation in different samples: (a) sample with BRL, (b) boiling HNO3 for 40 min to remove BRL, (c) chemical etch treatment to remove BRL, (d) 80 sccm O2 in the furnace during the thermal diffusion process to remove BRL.

class="figure_img" id="Figure3"/>
Download
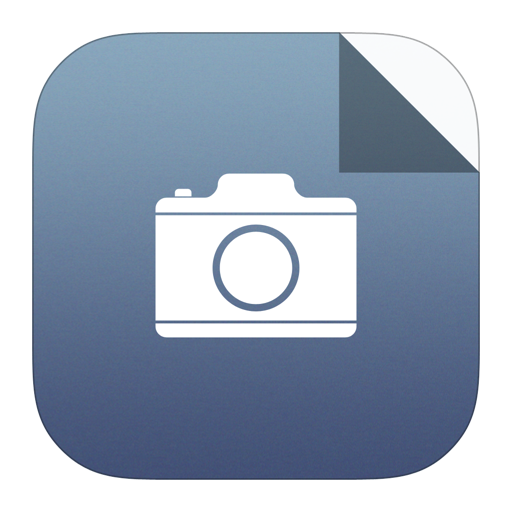
Larger image
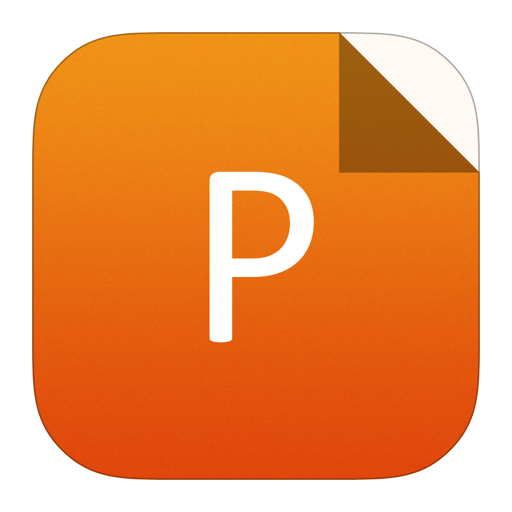
PowerPoint slide
Figure3.
(Color online) The effect of boron-diffused surfaces (a) with BRL and treated by three BRL removing methods of (b) boiling HNO3, (c) CET and (d) oxidation on the implied Voc of p+–n Si solar cells, respectively.
Considering the industrial applications of standard n-type screen-printed crystalline Si solar cells, substantial diffusion is quantitatively required to achieve the doping profile and to obtain sheet resistance, which is necessary for a low series resistance related to the p-type region and associated good electrical contact. Thus, the etched time for boron-doped p+ emitter needs to be well-controlled. As for group I samples, the CET time, using a mixture of HNO3, CH3COOH and HF, has been further investigated with times of 50, 90, 120, and 150 s. Fig. 4(a) shows that the CET time in order to remove a boron-rich layer has a significant impact on the p+ boron emitter. Correspondingly, their sheet resistance and junction depth for different CET times are also listed in Table 1. The increase of the CET time resulted in increasing p+ emitter sheet resistance from 19.65 Ω/sq at 0 s to 54.69 Ω/sq at 150 s as shown in Table 1. It has been found that high efficiency is maintained despite increases in junction depth ranging from a few nanometers to the hundreds of nanometers[28]. In Fig. 4(b), Al2O3/SiNx stack passivation on the BRL-etched boron emitter has markedly increased the implied Voc from ~630 to ~680 mV as the CET time is increased. It can be seen that a well-passivated surface has been successfully achieved for the p+ boron emitter with a higher implied Voc above the CET time of 50 s.
Etched time (s) | 0 | 50 | 90 | 120 | 150 |
Sheet resistance (Ω/sq) | 19.65 | 34.15 | 42.72 | 47.39 | 54.69 |
Junction depth (nm) | 700.0 | 565.6 | 495.5 | 474.6 | 450.2 |
Table1.
Sheet resistances and junction depth for different CET times.
Table options
-->

Download as CSV
Etched time (s) | 0 | 50 | 90 | 120 | 150 |
Sheet resistance (Ω/sq) | 19.65 | 34.15 | 42.72 | 47.39 | 54.69 |
Junction depth (nm) | 700.0 | 565.6 | 495.5 | 474.6 | 450.2 |

class="figure_img" id="Figure4"/>
Download
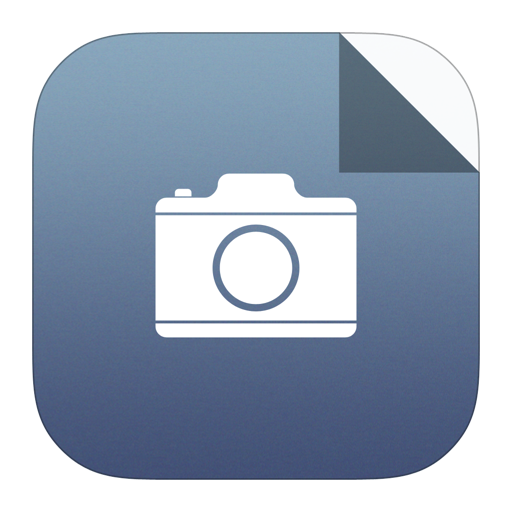
Larger image
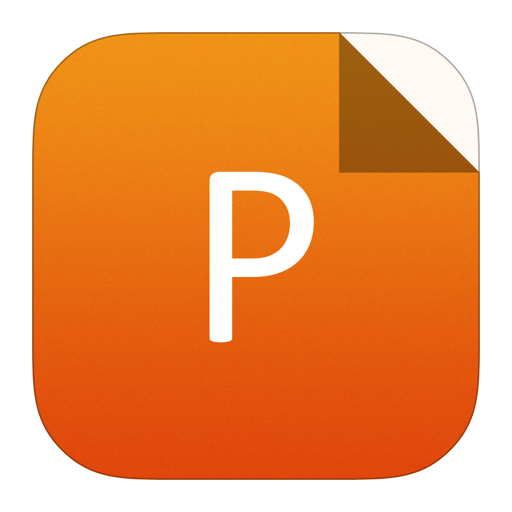
PowerPoint slide
Figure4.
(Color online) (a) Depth profile of boron concentration measured by ECV profiling under different etching time. (b) The performance comparison of Al2O3 passivation and Al2O3/SiNx stack passivation on the BRL-etched boron emitter.
4.
Conclusion
In summary, using CET a BRL has been removed from the boron-diffused p-emitters of n-type Si solar cells. Benefiting from the removal of the BRL, a reasonably high implied Voc has been achieved through surface passivation of Al2O3/SiNx stacks formed by ALD and PECVD. These results could open up an alternative way to realize low-cost c-Si solar cells.