1.
Introduction
There is considerable interest in investigating alternative materials to HgCdTe for the long wavelength spectral region. One of the promising materials is InAs1–xSbx, which has the lowest energy band gap (Eg = 0.1 eV for x = 0.65) among the III–V semiconductors compound[1]. This ternary alloy has prominent application to high speed electronic and optoelectronic devices in the wavelength range of 3–5 and 8–12μm[2–5]. However, it is awkward to obtain high-quality of InAs1–xSbx layers owing to the shortage of proper substrates. In spite of the fact that several III–V substrates such as GaAs, InAs, GaSb, InSb, and InP can be chosen for growing InAs1–xSbx, the huge lattice mismatch leads to a poor crystal quality. The growth of InAs1–xSbx on GaAs substrate is advantageous due to the large area, low cost, availability of high-quality GaAs substrates, and more developed GaAs processing technology. Unfortunately, the growth of high-quality InAs1–xSbx layers on GaAs substrate is difficult on account of the large lattice mismatch (7.2% ≤ ?a/a ≤ 14.6%)[6]. This later gives birth to a high dislocations density, which is detrimental to the electrical and structural properties. This shortcoming can be reduced by the incorporation of a thin buffer layer, such as InSb or AlSb[7], or step-graded InAs1–xSbx buffer layer[8], thick enough to comprise dislocations and so relieve the strain. The InAs1–xSbx epilayers can be grown by various techniques, such as molecular beam epitaxy (MBE)[6, 9–11], metal-organic chemical vapor deposition (MOCVD)[12–15] and liquid-phase epitaxy (LPE)[16, 17].
In this paper, we report on the growth of undoped and Be-doped InAs1–xSbx (0 ≤ x ≤ 0.71) on GaAs (001) substrate with 2° offcut towards <110>. In addition, the influence of the InAs buffer layer and Be doping on the electrical, structural properties of the epilayers has been investigated. Besides, we explore the dependence between the Sb/In flux ratio and the composition x. Also, we performed the differential Hall effect measurements in order to study the distribution of the electrically active defects along the growth direction of the grown layers. To the best of our knowledge, we are the first group to report this last result.
2.
Experiment
Undoped and Be-doped InAs1–xSbx (0 ≤ x ≤ 0.71) layers have been grown on GaAs (001) substrate with 2° offcut towards <110> in a RIBER COMPACT 21-DZ solid-source molecular beam epitaxy (MBE) system. Arsenic and antimony were supplied by valved cracker cells to produce As 4 and Sb2, respectively. A manipulator thermocouple (TC) and BandiT were used to monitor the substrate temperature. The growth temperature of GaAs layer was calibrated from the GaAs oxide desorption temperature. Firstly, a 300 nm-thick GaAs layer was grown at 655 °C on a manipulator thermocouple corresponding to 585 °C on the BandiT, in order to smooth the substrate surface. It is believed that the real temperature for the GaAs layer is that measured by the BandiT. Then, the substrate temperature was cooled down (400 °C TC, 335 °C BandiT) to start the growth of InAs or InAsSb epilayer. It is worth noting that the reported growth temperature measured by the BandiT corresponds to that measured just before the starting of InAs or InAsSb layer growth. During the layer growth, the temperature at the manipulator thermocouple was stabilized at 400 °C. The substrate temperature is believed to increase during the growth of the layer and to be less than 400 °C[18]. Three sets of samples were grown: A, B and C as can be seen in Table 1. In set A, a 400 nm-thick InAs layer was grown as a buffer layer before the deposition of 5 μm-thick InAsSb layers at 400 °C. Moreover, set B is the same as set A but without the InAs buffer layer. In set C, the properties of p-type InAsSb layers were investigated by doping the layers with beryllium (BE). The doping level at 80 K is 3.8 × 1017 cm?3 (p) and 1.8 × 1018 cm?3 (p+) for InAs0.32Sb0.68 and InAs0.39Sb0.71, respectively. The growth rate for the InAsSb layer was 0.80 μm/h. The growth process was monitored by in-situ reflection high-energy electron diffraction (RHEED).
Sets | Buffer layer | Doping | Composition x |
A | InAs | – | 0.13; 0.06; 0 |
B | – | – | 0.13; 0.27 |
C | – | Be | 0.68; 0.71 |
Table1.
InAs1–xSbx samples grown on GaAs (001) substrate.
Table options
-->

Download as CSV
Sets | Buffer layer | Doping | Composition x |
A | InAs | – | 0.13; 0.06; 0 |
B | – | – | 0.13; 0.27 |
C | – | Be | 0.68; 0.71 |
The crystallographic properties of the samples have been assessed by the high-resolution X-ray diffractometer of a PANalytical X’Pert. The Cu Kα1 radiation (λ ≈ 1.5406 ?) originating from a line focus was used. The X-ray beam was monochromatized by a four bounce, Ge (004) hybrid monochromator. The measurements were made in both ω and 2θ–ω directions. The electrical properties have been evaluated by Hall effect measurement using the Van der Pauw method between 80 K and room temperature with a step of 5 K. This measurement was performed by means of the ECOPIA Hall Measurements System. Nomarski optical microscopy and high resolution optical profilometry was utilized to evaluate the surface morphology and roughness of InAs1–xSbx layers.
3.
Results and discussion
In the case of growing InAs (x = 0), the RHEED exhibits a sharp (2 × 4) pattern[19]. When the Sb shutter is opened to grow InAs1–xSbx (x > 0), the RHEED pattern turns to spotty and faint due to the huge lattice mismatch. This indicates a three-dimensional growth mode. After the growth of a few monolayers, the RHEED pattern became brighter and the surface reconstruction switches to a streaky (1 × 3) pattern (as shown in Fig. 1), pointing out a continuous coverage and smoothening of the surface. Our observation is compatible with that of Tsukamoto et al.[11].

class="figure_img" id="Figure1"/>
Download
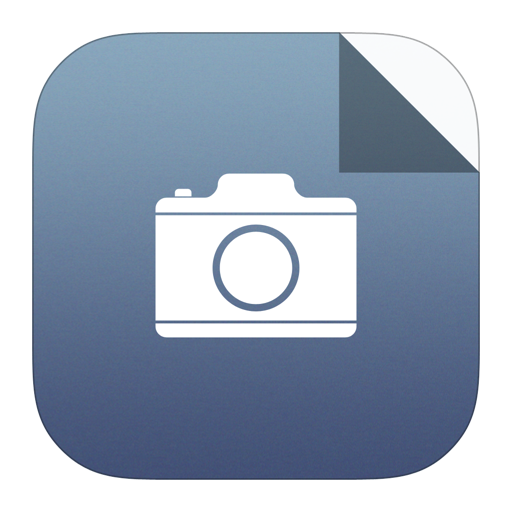
Larger image
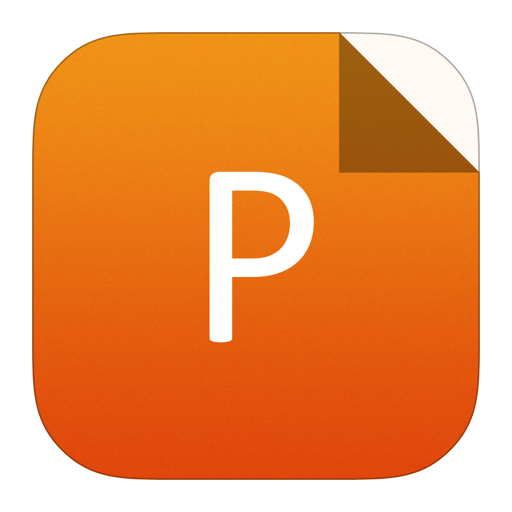
PowerPoint slide
Figure1.
(Color online) InAs1–xSbx layer RHEED pattern (a) (× 1) pattern and (b) (× 3) pattern.
The surface morphology characterized by Nomarski microscopy of InAs1–xSbx epilayers is shown in Fig. 2. A shiny mirror-like surface has been obtained for all the samples belonging to the three sets. However, the best root mean square (RMS) of surface morphology was that of set A, with a value of 17 nm. This outcome can be assigned to the InAs buffer layer, which reduces the large lattice mismatch between the GaAs substrate and InAsSb epilayers.

class="figure_img" id="Figure2"/>
Download
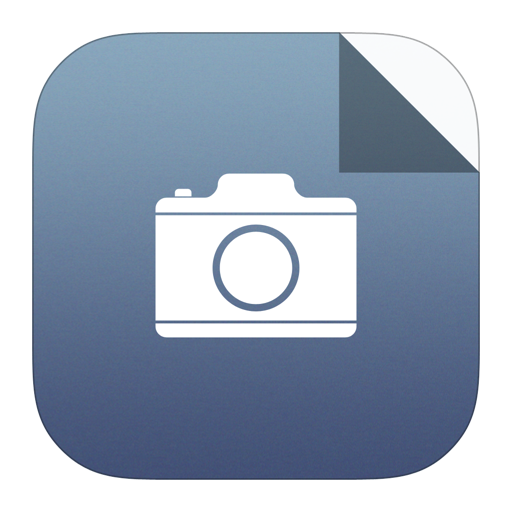
Larger image
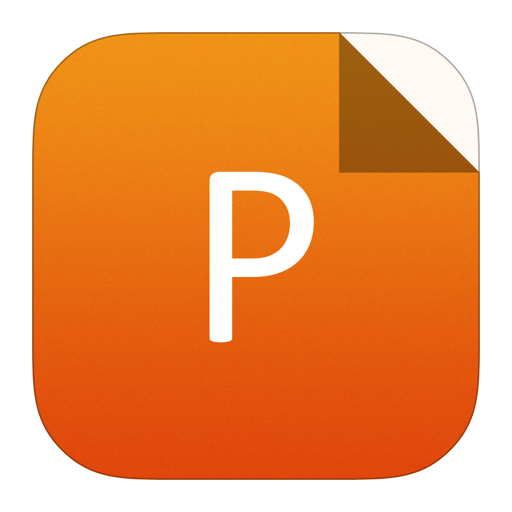
PowerPoint slide
Figure2.
The Nomarski optical microscopy pictures from (a) set A with x = 0.13, (b) set B with x = 0.13, and (c) set C with x = 0.71.
Fig. 3(a) exhibits the (004) diffraction curve in the 2θ–ω direction of InAs1–xSbx epilayers with different compositions x. Notoriously, every curve contains two peaks except that of set A. The peak at the position 2θ = 66.05° corresponds to GaAs substrate, while the other peak is that of InAs1–xSbx layers at different positions depending on the composition x. Mainly, the greater x is, the further the peak is from the GaAs peak, in other words, the more strained the layer is. In the case of set A, there is an extra peak at the position 2θ = 61.09°, that of the InAs buffer layer.
As follows from Fig. 3(b), set A exhibits the lowest FWHM, with a value of 571 arcsec (x = 0.13). On the other hand, an FWHM of 812 arcsec was obtained on set B (x = 0.13), which confirms the utility of the InAs layer in the improvement of crystal quality of InAsSb layers. Our result is much better than that reported by Nakamura et al.[8], who reported a value of 2664 arcsec for a 4 μm-thick InAs0.5Sb0.5 layer. Significant narrowing of the FWHM of InAs1–xSbx peaks with increasing Be doping level was reproducibly observed (as can be seen in Fig. 3). This is due probably to the vacancies and native defects in the InAsSb layers.

class="figure_img" id="Figure3"/>
Download
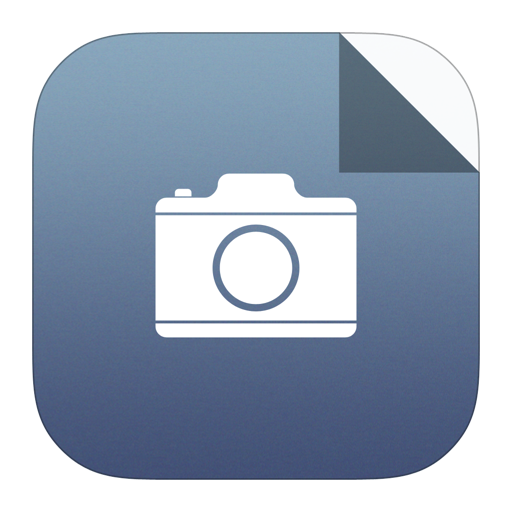
Larger image
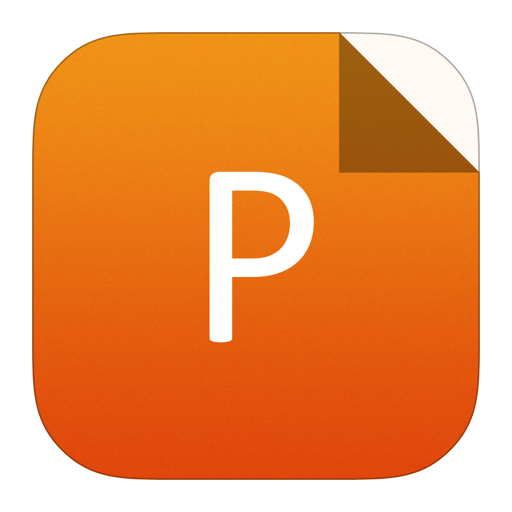
PowerPoint slide
Figure3.
(Color online) (a) (004) X-ray InAsSb layers (004) 2θ–ω scan and (b) the variation of the FWHM of the (004) InAsSb peaks measured in ω direction.
Fig. 4 presents the variation of the composition x as a function of Sb/In flux ratio. As can be seen in the figure, the fraction of Sb into the layer is linearly dependent on the Sb/In flux ratio, provided that the As flux is constant (1.06 × 10?6 Torr). The same dependence was reported by Yen et al.[9] and Marcadet et al.[20]. Essentially, the composition x could be readily controlled over the range 0 ≤ x ≤ 0.71. However, for low composition, the dependence is not linear. Surprisingly, when the Sb/In flux ratio was 0.05, the composition x was zero. Due to the low Sb flux compared to the As flux, Sb adatoms could not incorporate into the layer, which results in the formation of an InAs epilayer. In addition, when the growth rate is constant, which means that the In flux is constant, the composition x could be controlled by only the Sb flux. Sun et al.[21] reported that the Sb incorporation rate is linearly dependent on the Sb flux, under a constant As/In flux ratio. The control of the composition by As is not easy because the incorporation of As depends on both In flux and Sb flux at the same time.

class="figure_img" id="Figure4"/>
Download
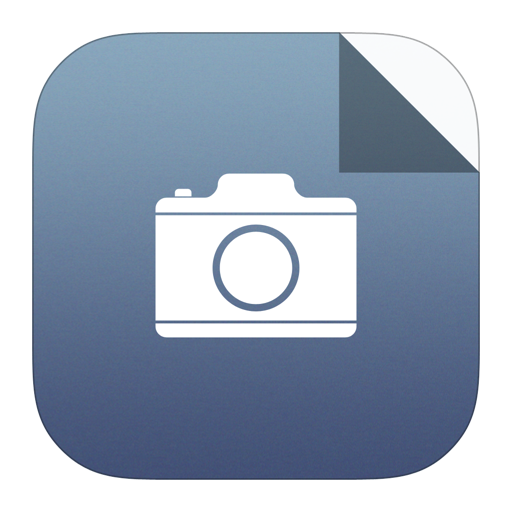
Larger image
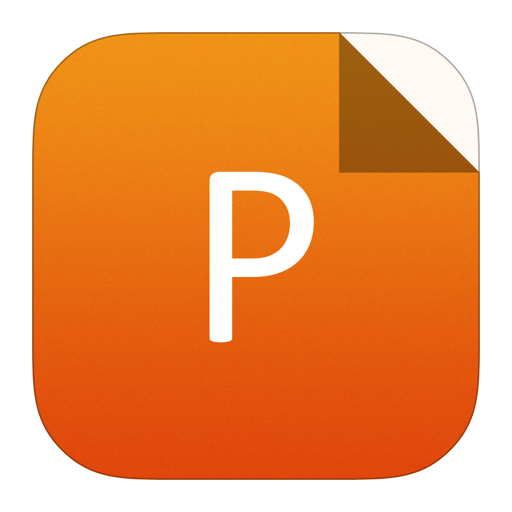
PowerPoint slide
Figure4.
(Color online) The composition x as a function of Sb/In flux ratio of InAs1–xSbx grown at 400 °C. The As flux is 1.06 × 10?5 Torr.
The Hall concentration and Hall mobility of InAs1–xSbx layers measured between 80 K and room temperature are shown in Fig. 5. It is worth noting here that the Hall concentration for set A and set B (undoped layers) is nearly constant over the temperature range, which is a typical behavior for n-type material and this reveals significant unintentional doping.
The unintentional n-type doping is due to a kind of donor defect in the material, which could be related to the dislocations. It should be noted that the Hall mobility is significantly higher in the layers grown using the InAs buffer layer, which emphasizes the advantage of the growth on the buffered substrates.
As can be noticed in Fig. 5(b), the Hall mobility for undoped InAsSb increases slightly as the temperature decreases. At high temperature, the optical phonon scattering dominates[9], which limits the mobility. On the other hand, at low temperature and low carrier concentration (n ≈ 1015 cm?3), the dominant scattering mechanism is alloy scattering[22], which is not noticed in our study, due to the relatively high carrier concentration. Meanwhile, the best Hall moblity was 20000 and 25000 cm2V?1s?1 at 300 and 80 K, respectively.
In contrast, the Hall concentration and the Hall mobility in the Be-doped layer highly depend on the temperature; the behavior is typical for narrow gap p-type semiconductors with low mobile holes as majority charge carriers and highly mobile minority electrons.
For the two-carrier conductivity, the Hall effect concentration and mobility are described by the well-known relations:
$${n_{ m{H}}} = frac{{{{left( {p{mu _{ m h}} + n{mu _{ m e}}} ight)}^2}}}{{pmu _{ m h}^2 - nmu _{ m e}^2}},$$ ![]() | (1) |
$${mu _{ m H}} = left| {frac{{pmu _{ m h}^2 - nmu _{ m e}^2}}{{p{mu _{ m h}} + n{mu _{ m e}}}}} ight|.$$ ![]() | (2) |
In p-type doped semiconductors, the charge neutrality relation can be written as follows:
$$n + N_{ m A}^ - = p.$$ ![]() | (3) |
where n is the concentration of electrons in the conduction band,
m A}^ - $

m i}^2$


class="figure_img" id="Figure6"/>
Download
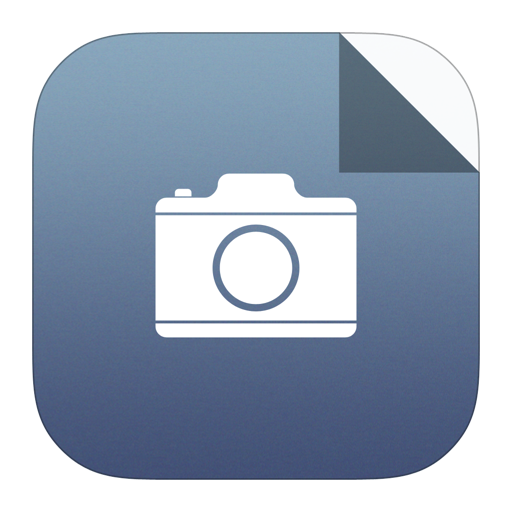
Larger image
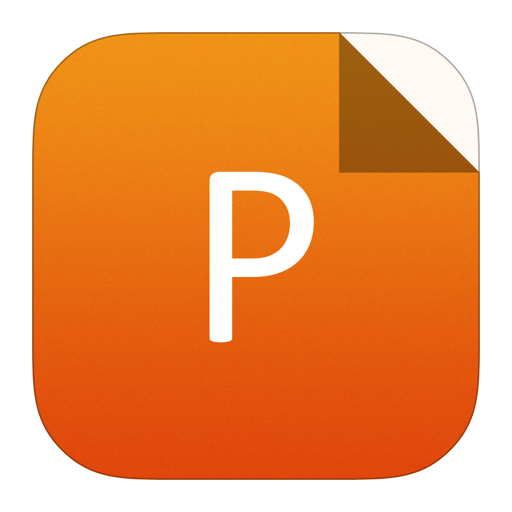
PowerPoint slide
Figure6.
(Color online) The theoretical Hall carriers, electrons, and holes concentration of Be-doped InAsSb layer with a doping level of 3.8 × 1017 cm?3.
As follows from Figs. 6 and 5(a), at high temperatures the Hall effect shows a negative sign of the Hall concentration, and a high mobility due to the significant concentration of the mobile minority electrons. This later decreases with decreasing temperature (as can be seen in Fig. 6), which results in a reversed sign of the Hall constant and the Hall concentration, and a change in the Hall mobility trend, when
m h}^2 = nmu _{
m e}^2$


class="figure_img" id="Figure5"/>
Download
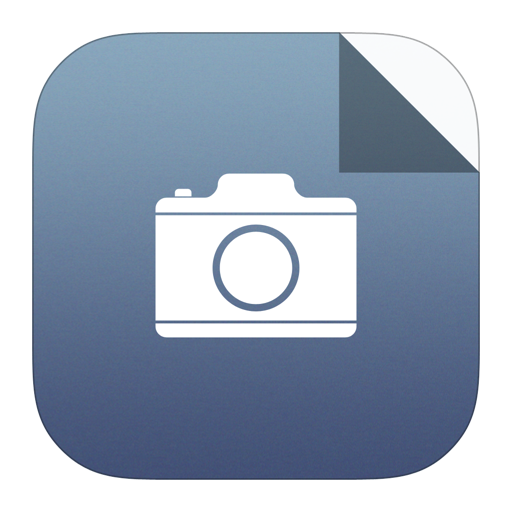
Larger image
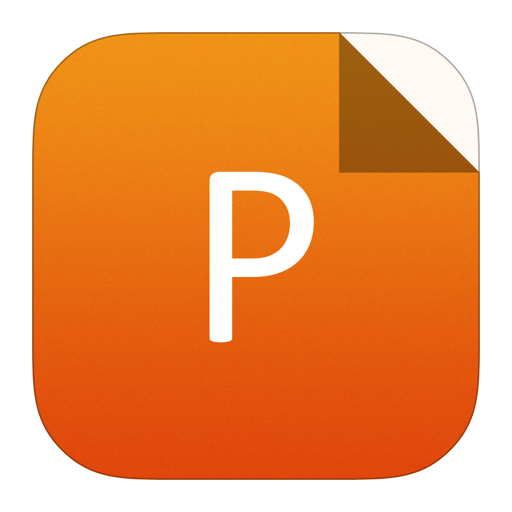
PowerPoint slide
Figure5.
(Color online) (a) Hall concentration and (b) Hall mobility of 5 μm-thick InAsSb epilayers measured at 80 K and room temperature.
In addition, we have investigated the distribution of the unintentional doping along the growth direction of the InAs0.94Sb0.06 layer by the differential Hall measurement, as shown in Fig. 7. Firstly, the Hall effect measurement is carried out for a 5.3 μm-thick InAs0.94Sb0.06 layer (whole layer). Secondly, having etched (wet etching) the 2.2 μm top portion of the layer, we perform Hall effect measurement for the remaining 3.1 μm-thick layer (bottom layer). Finally, with a simple calculation[23], we determine the Hall parameters for the 2.2 μm-thick etched layer (top layer). In principle, the Hall mobility (Hall concentration) decreases (increases) as the etched thickness increases (the film thickness decreases). The higher defect density and inhomogeneity in thinner layers lead to a lower Hall mobility and higher Hall concentration. Besides, the increase of the Hall mobility when the layer thickness increases, could be explained by the lowering of the effective mean free path of conductive carriers by scattering from the layer surface, when the film thickness is of the order of the bulk free path[24]. Notoriously, the top layer exhibits much better electrical parameters than the bottom layer. The Hall concentration (Hall mobility) for the top layer is 1.8 × 1016 cm?3 (39799 cm2V?1s?1) and 2 × 1016 cm?3 (24397 cm2V?1s?1) at 80 and at 300 K, respectively. This leads to the conclusion that the defects, which are probably related to misfit dislocations are located close to the interface.

class="figure_img" id="Figure7"/>
Download
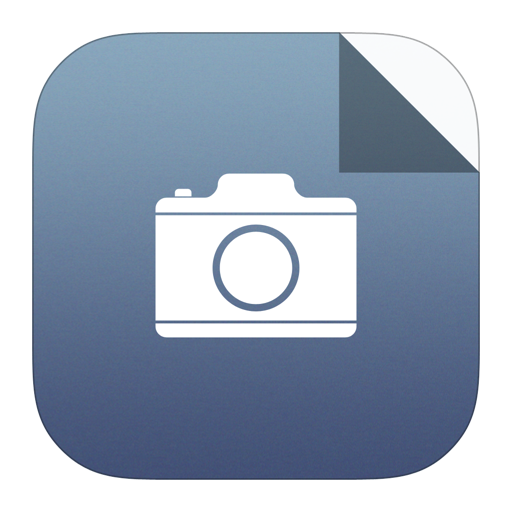
Larger image
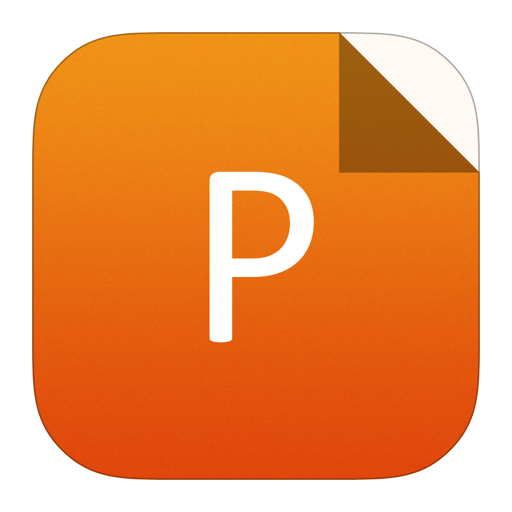
PowerPoint slide
Figure7.
(Color online) (a) Differential Hall concentration and (b) mobility of a 5.3 μm-thick InAs0.94Sb0.06. The etched thickness is 2.2 μm.
4.
Conclusion
In summary, we have grown undoped and Be-doped InAs1–xSbx epilayers on GaAs (001) substrates with 2° offcut towards <110> by molecular beam epitaxy. The use of the InAs buffer layer is found to be beneficial for the electrical and structural quality of InAsSb layers. This outcome is due to the reduction of the lattice mismatch between the InAsSb layer and GaAs substrate. In addition, it is important to note that the Sb fraction in InAsSb layer is linearly dependent of the Sb/In flux ratio. Therefore, a good control of Sb and In beam equivalent pressure (BEP) gives the opportunity to get the expected composition. The results acquired in this work enable the conclusion that the epitaxial layers have a good crystal quality. Furthermore, the InAsSb layer exhibits a Hall mobility (Hall concentration) as high (low) as 25000 cm 2V?1s?1 (5 × 1016 cm?3) at room temperature. For the p-type InAsSb layer, the Hall constant exhibits a positive sign at lower temperature, and negative sign for higher temperature, which is typical behavior for p-type narrow band gap material. Based on differential Hall measurement results, it can be concluded that the defects density decreases as the film thickness increases, and that the majority of these defects are located close to the interface.