1.
Introduction
The discovery of graphene opened a new era in material science since 2004[1]. Graphene consists of just a single layer of carbon atoms arranged in a hexagonal lattice, which is the first truly two-dimensional (2D) crystal. Peculiarities of the band structure of graphene explain its unusual electric properties[2, 3]. Many possible applications have been anticipated for graphene, such as field-effect transistors[4], photodetectors[5], transparent electrodes for displays[6] and so on, which has made graphene attractive for theoretical and experimental researches. The chemical stability of graphene makes it possible to be stacked in different combinations to produce a new class of functional materials, designed for specific device applications. Double-layer graphene consists of two monolayer graphene in Bernal stacking and has a hyperbolic energy momentum dispersion with a tunable bandgap[7]. Recent studies[8] have shown pronounced negative differential conductance (NDC) in double-layer graphene. The NDC has also been predicted in double-barrier resonant tunneling diodes[9], in diodes with graphene/boron nitride heterochannels[10], and in graphene nanoribbon superlattices including the resonant tunneling through minibands and the Wannier-Stark ladder regime[11]. NDC stimulates the prospects for applications in graphene-based frequency multipliers, fast switches, and, especially, terahertz frequency oscillators.
In this article by using a hydrodynamic model, we present detailed simulations of spatiotemporal characteristics of n+nn+ double-layer graphene diodes. It is found that n+nn+ graphene diodes exhibit undamped current self-oscillation related to field-domain dynamics. We have also calculated the dependence of self-oscillating behaviors on the applied DC voltage. The numerical results indicate that the graphene diodes is a promising candidate for THz generation at room temperature.
2.
Negative differential velocity of electrons in double-layer graphene and hydrodynamic model
The recent studies[8] have shown how to achieve gate-controlled resonant tunnelling and pronounced negative differential velocity (NDV) in graphene-based multilayer, which is shown in the inset of Fig. 1. They measured the current-voltage characteristics of their devices at room-temperature. The hBN barrier is 5 atomic layers thick; the active area for the flow of tunnel current is 0.6 mm2; Vg ranges from +50 to ?50 V in 5 V steps. The most notable feature of the data is the presence of a pronounced peak in the current, whose amplitude and voltage position are sensitive to Vg. Beyond the peak, there is an extended region of NDV.

class="figure_img" id="Figure1"/>
Download
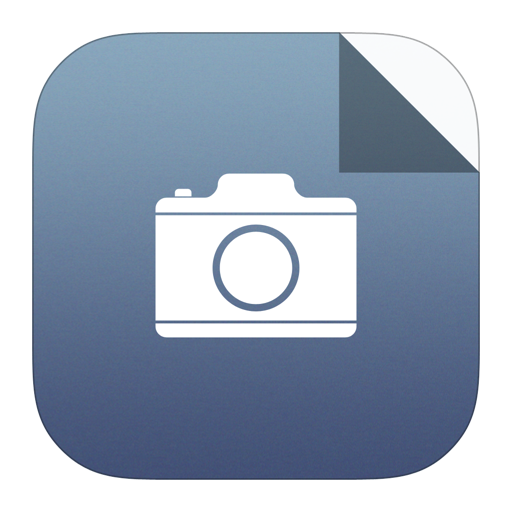
Larger image
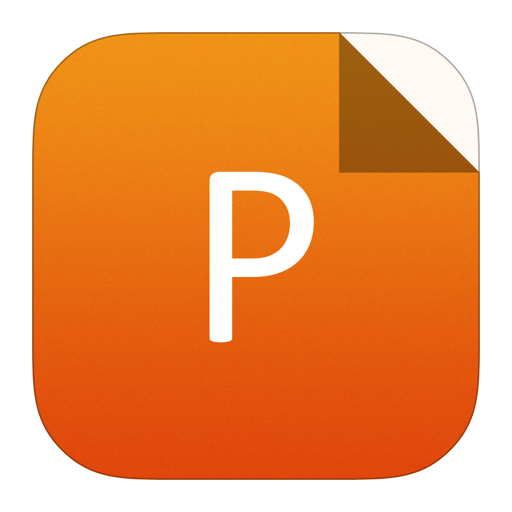
PowerPoint slide
Figure1.
(Color online) Analytical ?t for the velocity-field relation of double-layer graphene at lattice temperature T = 300 K.
We fit the experimental curve by the following velocity-electric field expression:
${V_{ m{d}}} = frac{{0.78E}}{{[1 + {{(E/11.5)}^2}] + 0.03Eexp (0.16E - 10)}}.$ ![]() | (1) |
It is shown that the analytical result (line) is in agreement with the experimental one in the whole region of electric fields.
By using the transient hydrodynamic equations, we can study electron transport in the n+nn+ double-layer graphene diode under a DC bias:
$frac{{partial n}}{{partial t}} = frac{1}{q}frac{{partial {j_{ m{n}}}}}{{partial x}},$ ![]() | (2) |
${j_{ m{n}}} = q{mu _{ m{n}}}left(frac{{{K_{ m{B}}}T}}{q}frac{{partial n}}{{partial x}} + frac{{{K_{ m{B}}}n}}{q}frac{{partial {T_{ m{e}}}}}{{partial x}} - nfrac{{partial phi }}{{partial x}} ight),$ ![]() | (3) |
$frac{{partial (nvarepsilon )}}{{partial t}} = - frac{{partial (nS)}}{{partial x}} - {j_{ m{n}}}frac{{partial phi }}{{partial x}} - nW,$ ![]() | (4) |
and the Poisson equation:
$frac{{{partial ^2}phi }}{{partial {x^2}}} = - frac{q}{epsilon }(n - {N_{ m{d}}}),$ ![]() | (5) |
in which n is the electron density, jn is the current density, t is the static dielectric constant, Φ is the electrostatic potential, μn = Vd/E is the electron mobility, Nd is the doping concentration, ε is the electron energy related to the electron temperature Te, S is the energy flow density, and W is the energy loss rate. For n(x, t) and Φ(x, t), the boundary conditions are as follows:
$n({x_{ m{L}}},t) = {N_{ m{d}}}({x_{ m L}}),; n({x_{ m{R}}},t) = {N_{ m{d}}}({x_{ m{R}}}),$ ![]() | (6) |
$phi ({x_{ m{L}}},t) = 0,; phi ({x_{ m{R}}},t) = {V_{{ m{dc}}}}.$ ![]() | (7) |
3.
Current oscillations in double-layer graphene diodes
For a semiconductor system with negative differential velocity (NDV), any doping inhomogeneity fluctuation may result in a current oscillation in semiconductor devices under biased conditions[12–14 ]. The interesting phenomenon of time-dependent currents show up due to the formation of high-field domains. Here we performed detailed simulations of time-dependent oscillations in the dc biased n+nn+ double-layer graphene diodes with the use of transient hydrodynamic equations.
The total current density consists of the conduction current density and the displacement current density:
$J(t) = {j_{ m{n}}}(x,t) + kappa {varepsilon _0}frac{{partial E(x,t)}}{{partial t}}.$ ![]() | (8) |
When the device is biased by proper Vdc and doped with proper concentration, there are time-dependent current oscillations in the device. We have carefully studied the dependence of the current densities on the device parameter and the dc bias. We find that the current in the diode with the length of the n base l = 0.4 μm has a time-periodic oscillating property. The formation of high-field domains in the device largely depends on the electron concentration and the DC bias.
In Fig. 2 we show the self-oscillation frequency of double-layer graphene diode with different applied biases. A schematic view of the n+nn+ double-layer graphene diode is shown in the inset of Fig. 2. Here the doping concentration is Nd = 5 × 1016 cm?3 and lattice temperature is T = 300 K. The self-oscillation frequency is in the THz region changing from 0.495 to 0.349 THz with the applied biases. It is clear that under different DC bias the diode exhibits current oscillations with different oscillation frequencies. Under DC bias there are two self-oscillation modes, the transit-time mode under sufficiently high bias and the quenched mode under insufficient bias. The self-oscillating frequency in the quenched mode is usually greater than that in the transit-time mode. Due to the transfer from quenched mode to transit-time mode, fs usually decreases with an increase of Vdc.

class="figure_img" id="Figure2"/>
Download
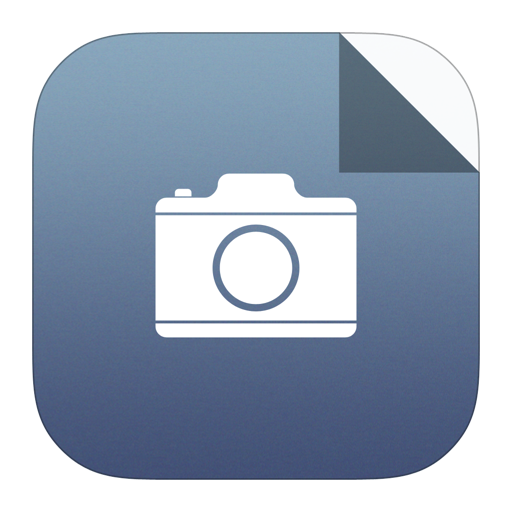
Larger image
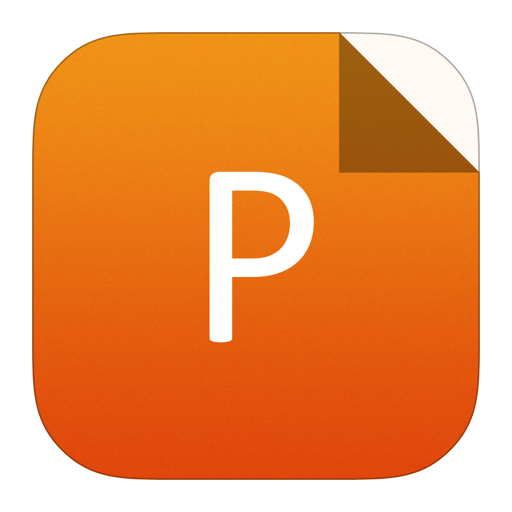
PowerPoint slide
Figure2.
(Color online) Self-oscillation frequencies of double-layer graphene diode at lattice temperature T = 300 K.
In Fig. 3 we show the time-dependent current densities J(t) (in the unit of 109 A/cm2) at different dc voltages Vdc = 0.825, 0.89 and 1.0 V. It is shown that there are self-oscillating currents in the double-layer graphene diode and their frequencies can reach up to 0.455 THz and 0.39 THz when Vdc = 0.89 and 1.0 V, separately. For the smaller Vdc = 0.825 V the diode exhibits a damped current oscillation, and finally reaches a constant current. The calculations indicate that the present double-layer graphene diode may be used in THz generation.

class="figure_img" id="Figure3"/>
Download
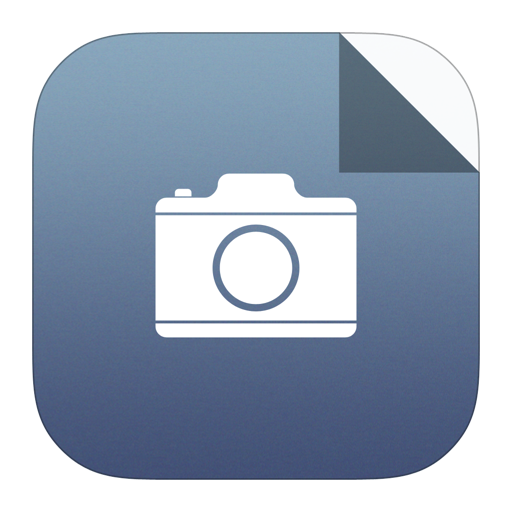
Larger image
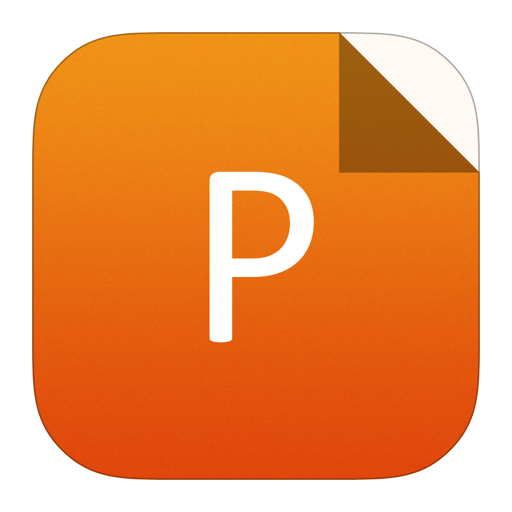
PowerPoint slide
Figure3.
(Color online) Temporal evolution of the current densities J(t) at different DC voltages.
This work is to investigate the THz self-oscillations and the dependence of the oscillation frequency on the biases in a double-layer graphene system with NDV. The present NDV relation is from the experiments, which may originate from the resonant-tunneling effect or anomalies in the joint tunneling density of states of graphene. The NDV may also be found in single-layer graphene arising from quantum tunneling through a barrier[15, 16]. By inserting this NDV relation into the hydrodynamic model, we have calculated the current self-oscillations in double-layer graphene n+nn+ diodes. The dominant scatterings are from the electron-impurity and electron-phonon interactions. For more careful consideration, we may include the electron-electron interaction. In fact, this kind of semi-classic hydrodynamic method has widely been used to study electron transport in different semiconductor systems.
4.
Conclusions
In summariy, we have calculated self-oscillating current densities in the n+nn+ double-layer graphene diodes with use of the transient hydrodynamic model. When the device is driven by the proper DC voltages, a high-field domain shows up in the device, forming time-dependent current self-oscillation in the THz region. By careful theoretical calculations we get the relation of current frequency in the THz region with the dc biases. The present studies may guide the design of room-temperature THz sources.