1.
Introduction
III-nitride semiconductor laser diodes (LDs) have attracted a great deal of attention and made remarkable progress in the last few years due to their great potential in laser lighting, communication systems, and laser display applications[1–3].
One of the emphasizing points for the widespread applications of these LDs as a promising light source, for example, used for the disk reading and writing, is to control the shape of beam spot, particularly to reduce the aspect ratio of the far-field pattern (FFP)[4–6]. A high aspect ratio deteriorates the coupling efficiency of the laser beam to the lens. However, the light emitted from GaN-based LDs is typically very elliptical. Normally, the FFP aspect ratio of GaN-based LDs is larger than 3[2, 7], which is nearly twice of that for conventional AlGaInP-based red LDs[8]. The reported θ⊥ for GaN-based LDs was usually larger than 20°[4, 5, 7, 9], while a smaller radiation angle of 16.5° was reported for conventional AlGaInP-based red LDs[8]. In order to reduce the aspect ratio of GaN-based LDs, it is necessary to decrease the radiation angle of the laser beam in the direction perpendicular to the junction plane (θ⊥) and increase the beam divergence angle parallel to the junction plane (θ//). In fact, Asano et al. have changed the thickness of waveguide layers in GaN-based LDs to reduce the θ⊥. However, it remains still as large as around 20° as reported in Refs. [4, 5].
For GaN-based LDs grown on bulk GaN substrates, the refractive index of the substrate is larger than that of AlGaN cladding layers (CLs). The waveguide mode may leak into the GaN substrate, which affects the FFP and threshold current. To solve this problem, the AlGaN cladding layers are required to be thicker[10–12]. These strained AlGaN layers introduce several significant drawbacks, including increased possibility of cracking due to tensile strain, a higher operation voltage, and a poor structural quality. Furthermore, a thick AlGaN layer growth by metal-organic chemical vapor deposition (MOCVD) could be problematic for reactor stability due to parasitic reactions of the Al-containing precursor. Previous studies of high-power GaN-based LDs had shown that reducing the Al composition in the AlGaN CLs could increase the level of catastrophic optical damage. It is noted that to overcome these problems, non-polar or semi-polar GaN-based Al-free cladding-layer LDs were reported in the literature[13–16]. However, till now the c-plane Al-free cladding-layer LDs have not been reported.
In this paper, we have numerically investigated the effects of Al-free CLs on θ⊥ of FFP and the deeply etched mesa on θ// of FFP for c-plane GaN-based blue LDs. After that, we also fabricated Al-free cladding-layer blue LDs with deeply etched mesa. The obtained aspect ratio of FFP is reduced to as low as 1.7, which is nearly the same as the value for conventional AlGaInP -based red LDs.
2.
Device structure and parameters
To examine the aspect ratio of FFP, the simulation of two selected laser structures, as shown in Figs. 1(a) and 1(b), is performed. The Al-free cladding-layer blue LD structure with deeply etched mesa (marked later as the new structure), as shown in Fig. 1(a), is composed of a 100-μm-thick n-type GaN substrate, a 1-μm-thick n-type GaN CL, a 120-nm-thick n-type In0.03Ga0.97N lower waveguide layer, the multi-quantum-well (MQW) active region, an 80-nm-thick unintentionally doped In0.03Ga0.97N upper waveguide layer, a 20-nm-thick heavily-doped p-type GaN electron blocking layer (EBL), a 500-nm-thick p-type GaN CL, and a 40-nm-thick heavily-doped p-type GaN contact layer. The MQW consists of three 2.5-nm-thick unintentionally doped In0.17Ga0.83N well layers and four 14-nm-thick n-type GaN barrier layers. For the purpose of comparison, a reference structure (marked later as reference structure), as shown in Fig. 1(b), is also calculated. The difference between it and the new structure is in the n-type CL, p-type CL and EBL. In the new structure, all n-type CL, p-type CL and p-type EBL are made of GaN, while in the reference structure, both n-type and p-type CLs are made of Al0.14Ga0.86N/GaN superlattices (SLs), and the EBL is made of p-type Al0.2Ga0.8N. Actually, the reference structure is a conventional structure and its performance has been reported many times in the literature[17, 18].

class="figure_img" id="Figure1"/>
Download
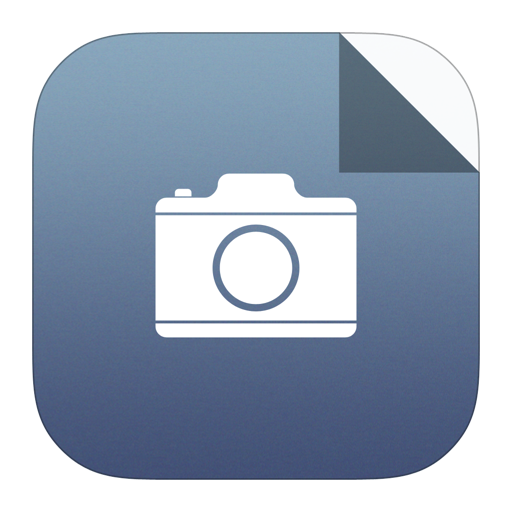
Larger image
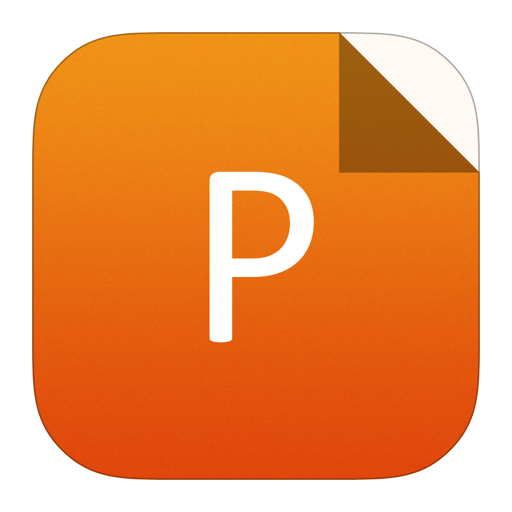
PowerPoint slide
Figure1.
(Color online) The schematic diagrams of (a) new structure and (b) reference structure.
The cavity lengths and the ridge widths for both the reference and new structures are 800 and 3.5 μm, respectively. The ridge depths are different and schematically shown in Fig. 1. For the new structure, the ridge depth is as deep as 640 nm, which is down to the MQW to enhance the confinement of optical field and current in the horizontal direction[19]. For the reference structure, the ridge depth is 540 nm, where both of p-type AlGaN/GaN SL CL and p-type GaN contact layer are etched away outside of the ridge. In simulation, the data of the refractive index are taken to be the same as those reported in previous studies[17]. Based on these refractive indexes, the 2-dimensional optical mode and FFP of two GaN-based LDs are calculated and analyzed by the commercial software Mode-Solution.
3.
Results and discussion
Fig. 2 shows the calculated optical field intensity distribution for both the reference and new structures in the direction perpendicular to the junction plane. For the reference structure, the maximum value of the optical field is in the MQW region. As the distance away from the MQW increases, the optical field intensity reduces gradually, and comes to nearly zero in both p-type and n-type CLs. The optical confinement in the active region is quite strong, and most of the optical field is distributed in the MQW and waveguide layer regions, which means that the θ⊥ of FFP for the reference structure is as large as 20.6°, as shown in Fig. 3(a). For the new structure with GaN CLs, the optical confinement in the active region is reduced a lot as compared with the reference structure. The maximum value of the optical field is still in the MQW, but it distributes in a much wider area, and spreads a little into the thick GaN substrate. According to the laser theory, the FFP of LDs is the diffraction pattern of the near-field pattern, and a larger optical field distribution area will induce smaller FFP. Therefore, the θ⊥ of FFP for the new structure is as small as 9.8°, which is less than half of the θ⊥ of FFP for the reference structure. Fig. 3(b) shows the calculated far-field intensity distribution of both the reference and new structures in the direction parallel to the junction plane (θ//). By increasing the etching depth of mesa, the optical confinement in the ridge of the new structure is enhanced, and the θ// is increased from 4.8° to 5.9° in comparison with the reference structure. Thus the aspect ratio of FFP for the new structure is 1.7, which is only nearly 39% of the reference structure and almost equal to that of the reported value for conventional AlGaInP-based red LDs.

class="figure_img" id="Figure2"/>
Download
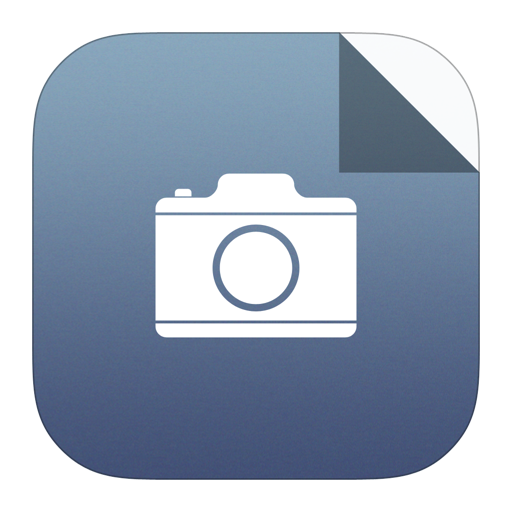
Larger image
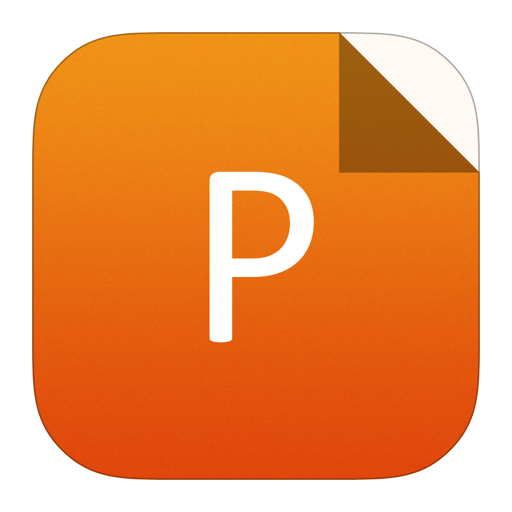
PowerPoint slide
Figure2.
(Color online) The calculated optical field distribution for both reference and new structures in the direction perpendicular to the junction plane.

class="figure_img" id="Figure3"/>
Download
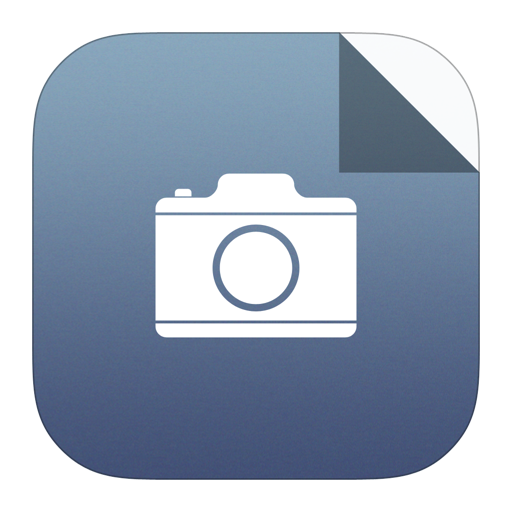
Larger image
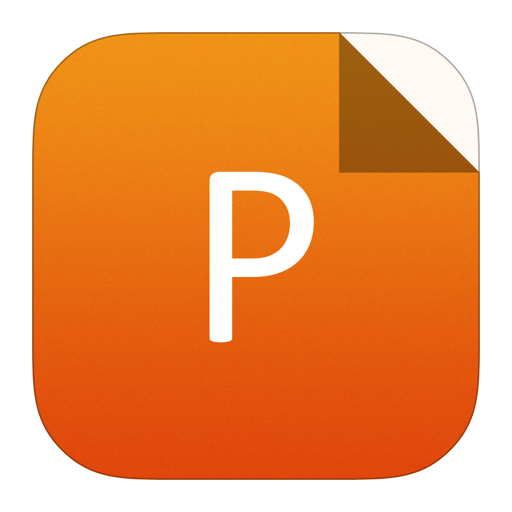
PowerPoint slide
Figure3.
(Color online) The calculated far-field intensity distributions of both reference and new structures in the directions (a) perpendicular to the junction plane (θ⊥) and (b) parallel to the junction plane (θ//).
To check the above-mentioned results, two blue LDs, i.e., LD A with the new structure and LD B with the reference structure, were fabricated. Both of them were grown on c-plane free-standing GaN substrate in a low-pressure MOCVD reactor. The ridge structure of LDs was formed by ion beam etching. SiO2 was used to isolate the ridge edges from the thick p-type electrode. Conventional Ni/Au and Ti/Al/Ti/Au metal schemes were used as p-electrode and n-electrode, respectively. The resonant cavity facets were made by cleavage and left uncoated. The electrical and luminescence characteristics of the devices were measured by on-bar probing. All measurements were taken at room temperature (25 °C).
Fig. 4(a) shows the measured far-field intensity distribution of both LD A and LD B in the direction perpendicular to the junction plane. The obtained θ⊥ values are almost the same as simulation results. For LD A with GaN CLs, the θ⊥ is reduced to be 10.1°, which is only 48% of that (20.3°) for LD B. The far-field intensity of LD B exhibits a narrow side lobe located at the angle of nearly ?18°, which is due to the waveguide mode leaking into the bulk GaN substrate. It is known that as the refractive index of bulk GaN substrate is larger than that of AlGaN CL, the waveguide mode could leak into the bulk GaN substrate when the n side optical confinement is not strong enough. It is why normally a thicker n-type AlGaN CL or an AlGaN CL with higher Al composition are often suggested to be adopted[11]. For LD A with the new structure, both CLs and substrate are of GaN material, so their refractive indexes are all the same. Therefore, no clear leakage mode exists.
Fig. 4(b) shows the measured far-field intensity distribution of both LD A and LD B in the direction parallel to the junction plane. For LD B with the reference structure, the θ// is 4.9°. For LD A with the deeper etched mesa, the θ// increases to 5.8°, making the aspect ratio of FFP for LD A with the new structure decrease to 1.7, which is much smaller than the value of 4.1 for LD B with the reference structure. Figs. 4(c) and 4(d) show the photographs of FFP for LD A and LD B, respectively, which also proves a smaller aspect ratio of FFP for LD A.

class="figure_img" id="Figure4"/>
Download
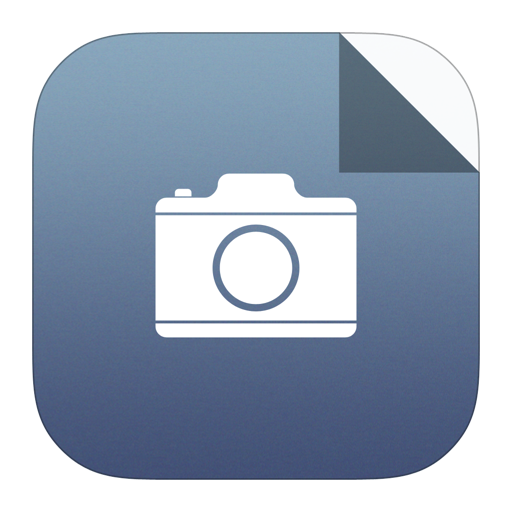
Larger image
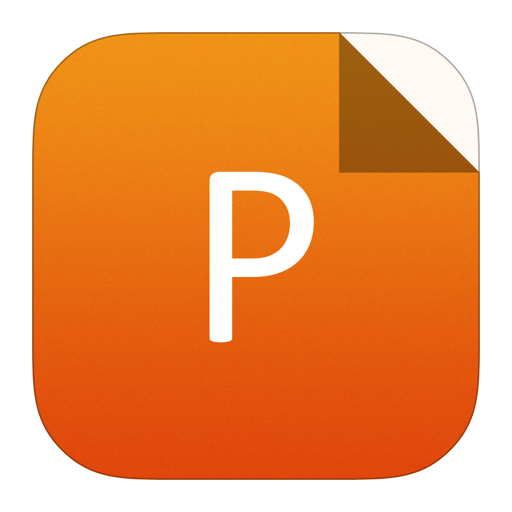
PowerPoint slide
Figure4.
(Color online) The measured far-field intensity distributions of both LD A and LD B in the direction (a) perpendicular to the junction plane (θ⊥) and (b) parallel to the junction plane (θ//), and the FFP photographs of (c) LD A and (d) LD B.
Fig. 5 shows the voltage-current curves of LD A and LD B. For LD A with the new structure, p-type GaN is used as the CL, and the operation voltage is 7.8 V at the injection current of 50 mA. On the other side, as the activation energy of the Mg acceptor increases with increasing Al composition of the p-AlGaN alloy[17], it has an influence on the conductivity of CLs. For LD B with p-type Al0.14Ga0.86N/GaN SL CL, the operation voltage is 9.8 V at the injection current of 50 mA, which is 2 V larger than that for LD A.

class="figure_img" id="Figure5"/>
Download
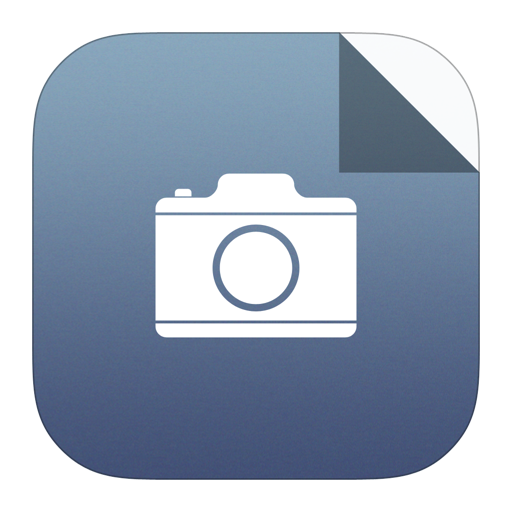
Larger image
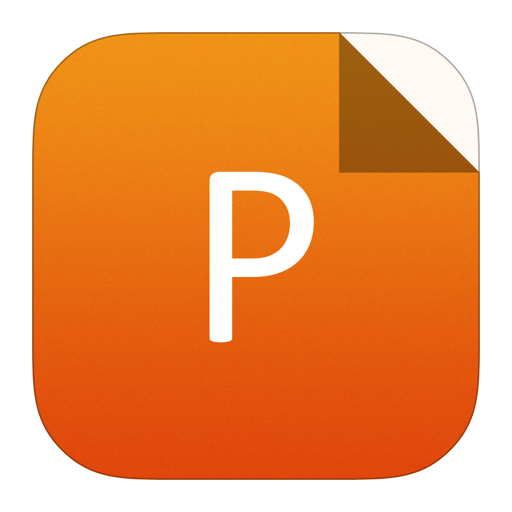
PowerPoint slide
Figure5.
(Color online) The voltage–current curves of LD A and LD B.
The measured pulsed power-current curves of LD A and LD B are shown in Fig. 6. The pulse width is 400 ns and the repetition rate is 10 kHz, which corresponds to a 4‰ duty cycle. For LD A with the new structure, the threshold current is 750 mA, which is much larger than that (~450 mA) of LD B with the reference structure. A possible reason for the difference could be explained as follows.

class="figure_img" id="Figure6"/>
Download
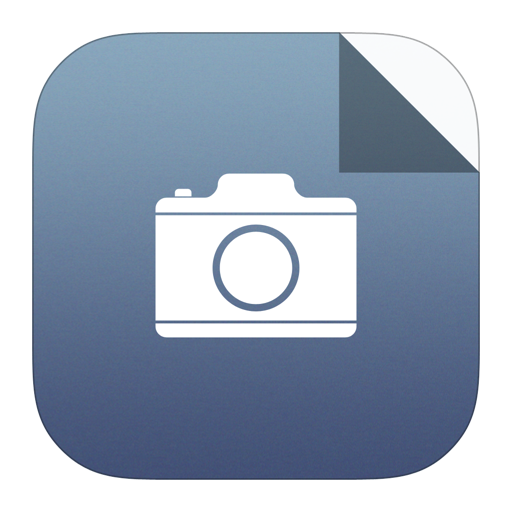
Larger image
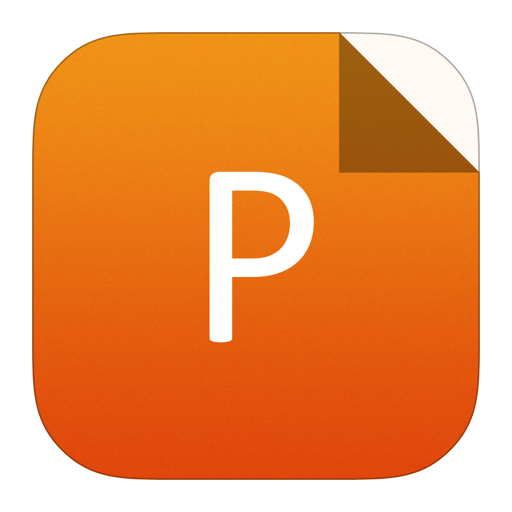
PowerPoint slide
Figure6.
(Color online) The power–current curves of LD A, LD B, and LD C.
(1) Optical confinement factor of the MQW region becomes smaller. Actually, compared with LD B, the optical confinement of the active region for LD A with the new structure is reduced, and the optical field is distributed in a much wider area, which decreases the optical confinement factor of the MQW. To make the LD lasing, the material gain of the LD should be increased, which would raise the threshold current.
(2) Absorption loss increases. Mg acceptor in the p-type region causes a strong absorption[20]. The optical loss of the LD mainly comes from the p-type region[21]. Due to there being less optical confinement in the active region of LD A, the optical field distributed in the p-type region increases. Therefore, the absorption loss for LD A is larger than that of LD B, which will increase the threshold current too.
(3) Etching damage. The etching process will cause a lot of etching damage, such as surface states, N vacancies, and so on[22, 23]. Such etching damage would become non-radiative recombination centers and influence the efficiency[19]. For LD A with deeply etched mesa, the current lateral spreading could be suppressed efficiently, and the optical confinement in the ridge is also enhanced. However, the etching damage is much closer to the MQW region where the peak optical field is located. Therefore, the non-radiative recombination due to etching damage will play a more important role, which would increase the threshold current.
To check the influence of etching depth, we have additionally fabricated another blue LD (named as LD C) with Al-free cladding layers by using the same wafer as LD A. The difference between LD A and LD C is in the etching depth. The etching depth for LD C is 540 nm, which is the same as LD B. As shown in Fig. 6, the threshold current of LD C is nearly 500 mA, which is a little larger than that of LD B (~450 mA), but much smaller than that of LD A (~750 mA). Therefore, it is shown that a smaller optical confinement factor and a larger absorption loss may play roles in the difference of threshold current for LD A and LD B, and the etching damage should be one of the main reasons responsible for that. To reduce the threshold current of LD A, the etching damage should be carefully removed or passivated. Therefore, a wet etching or passivation method is under way to remove or passivate the etching damage to significantly reduce the threshold current of LD A. Additionally, compared with LD B, the threshold current of LD C is only 11% larger, however, the operation voltage for LD A (or LD C) is 2 V less at the injection current of 50 mA (1.8 kA/cm2). Therefore, Al-free cladding-layer LDs could also play an important role in GaN-based high-power LDs.
4.
Summary
In conclusion, we have made c-plane GaN-based blue LDs with Al-free cladding layers, emphasized on achieving the beam spot with a low aspect ratio of FFP. The LD structures with and without Al-free CLs are compared with each other by simulation and experiment. By using Al-free CLs, the θ⊥ of FFP is reduced from 20.3° to 10.1°. With deeper etched mesa, the θ// of FFP is increased from 4.9° to 5.8°. Therefore, the aspect ratio of FFP is reduced to be 1.7, which is almost the same as the reported value for conventional AlGaInP-based red LDs. The LD operation voltage at the injection current of 50 mA is also reduced by 2 V when using Al-free CLs. In addition, we analyzed the reasons causing the threshold current difference between LDs with and without Al-free CLs; the etching damage is found to be one of the main reasons.