1.
Introduction
Magnetic semiconductors have attracted considerable attention due to their potential applications in spintronic devices, such as fast nonvolatile semiconductor memories and integrated magnetic–electronic–photonic devices[1, 2]. Recently, wide bandgap semiconductor Ga2O3 has received intensive research attentions due to its various potential applications in military and civilian facilities, such as missile tracking, fire detection, chemical/biological analysis, ozone holes monitoring and a series of fields[3, 4]. Many efforts have been devoted to incorporating other elements into Ga2O3 to obtain magnetic semiconductors[5–8].
Historically, as a transition-metal element, attempts have been made to dope Mn into Ga2O3 thin films for its multi-form of valence states and achieve RT ferromagnetism[9–15]. Minami et al. have firstly prepared Ga2O3:Mn thin film by using sol–gel method[9, 10]. Kim et al. have reported that their Ga2O3:Mn films by radio frequency planar magnetron sputtering began to crystallize into a monoclinic phase (β-Ga2O3) only after the annealing temperature exceeded 1073 K[11]. However, high quality Mn-doped β-Ga2O3 thin films for magnetic-semiconductor devices have not been reported. Hayashi et al. have obtained γ-(Ga0.93Mn0.07)2O3 thin film by using pulsed laser deposition technique at 773 K and pointed out that Mn atoms were located at tetrahedral coordinated Ga sites and the cation vacancies preferred the octahedral sites[12, 13]. However, among six crystal structure phases (α, β, γ, δ, κ and ε) of Ga2O3, β-Ga2O3 is considered to be the most stable thermally in the range from room temperature up to the melting point of about 1800 °C. Due to its high chemical and thermal stability, β-Ga2O3 is favorable for robust devices that can work in harsh environments[16–18]. We have obtained β-(Ga1–xMnx)2O3 with even high Mn content by controlling Mn concentrations with an alternately depositing technology at 1173 K and observed ferromagnetism even above room temperature[14, 15]. However, the process is complex and inapplicable in device production.
In this work, we use a standard solid-state reaction method and L-MBE technique to prepare fixed concentration (Ga0.96Mn0.04)2O3 thin film. The L-MBE technique was used to obtain high quality epitaxial single phase thin film and prevent other element contamination[19]. Structural, morphological, optical and magnetical properties with experiments and first principle calculations have been systematically analyzed. X-ray diffraction results indicate that the thin films have a high quality single monoclinic phase with a preferable orientation. RT ferromagnetism appears and the magnetic properties of β-(Ga0.96Mn0.04)2O3 thin film are enhanced compared with our previous works.
2.
Experimental
The (Ga0.96Mn0.04)2O3 target was prepared by a standard solid-state reaction method. High purity (5N) powders of Ga2O3 and Mn were thoroughly mixed, ground, and annealed at 1073 K for 8 h in air atmosphere twice, and then pressed into a Φ 25 × 4 mm target size followed a sintering process at 1273 K for 8 h in air atmosphere. (Ga0.96Mn0.04)2O3 and pure Ga2O3 thin films were then grown on sapphire substrates by L-MBE method at 1023 K and the oxygen pressure was 2 × 10?3 Pa. The laser ablation was carried out at a laser fluence of ~4 J/cm2 with a repetition rate of 2 Hz using a KrF excimer laser with a wavelength of 248 nm. The distance between target and substrate was 45 mm, and there followed an in situ annealing process at 1273 K in oxygen atmosphere for 30 minutes to improve the crystallinity property.
The crystal structure was characterized by a Bruker D8 Advance X-ray diffraction (XRD) using Cu Kα (λ = 1.5405 ?) radiation. The thickness and surface morphology were characterized by a Hitachi S-4800 field emission scanning electron microscope (FE-SEM) and an XE-100E atomic force microscopy (AFM), respectively. The elements composition in the Mn-doped thin film was measured by energy dispersive spectroscopy (EDS). Ultraviolet–visible (UV–vis) absorption spectrum was taken using a Hitachi U-3900 UV–visible spectrophotometer, and magnetic properties of the films were measured using a physical property measurement system (PPMS).
3.
Results and discussions
Fig. 1 shows the XRD patterns of (Ga0.96Mn0.04)2O3 and pure Ga2O3 thin films deposited on sapphire substrates. Except diffraction peaks of the substrate, three peaks are clearly observed around 18.8°, 38.2°, 58.9° which can be assigned as the
ight)$

ight)$

ight)$

ight)$


class="figure_img" id="Figure1"/>
Download
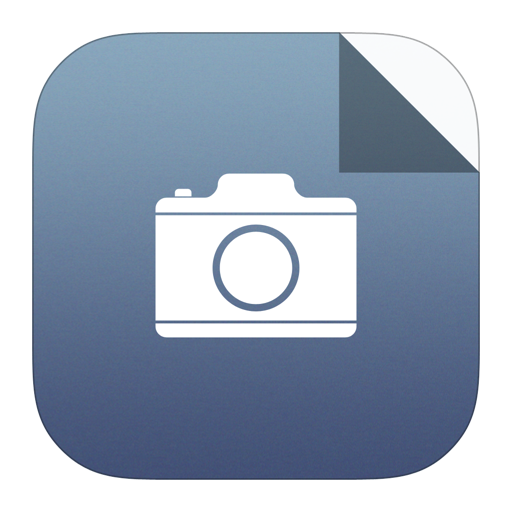
Larger image
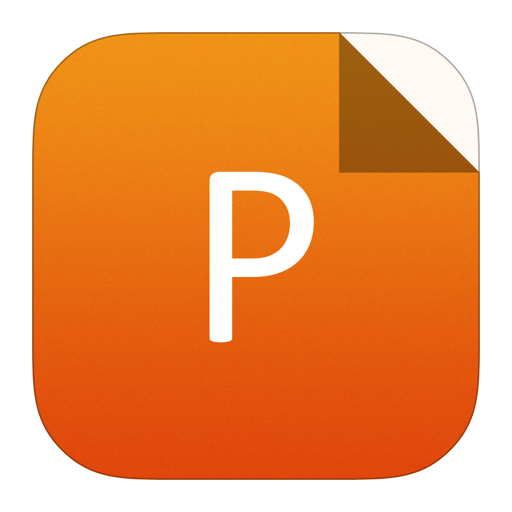
PowerPoint slide
Figure1.
(Color online) X-ray diffraction θ–2θ scan of (Ga0.96Mn0.04)2O3 and pure Ga2O3 thin films.
Fig. 2(a) shows the AFM surface morphology image of β-(Ga0.96Mn0.04)2O3 for a 5 × 5 μm2 scanning area. The mean surface roughness (Ra) is 0.583 nm. Two scanning lines along horizontal and vertical indicate that fluctuates of the crest and trough are in the range of ±1.6 nm. Here, the thin film has a thickness of about 200 nm, estimated by the cross-sectional SEM image in Fig. 2(b), implying a smooth surface which is suitable to fabricate magnetic-semiconductor devices[24–26].

class="figure_img" id="Figure2"/>
Download
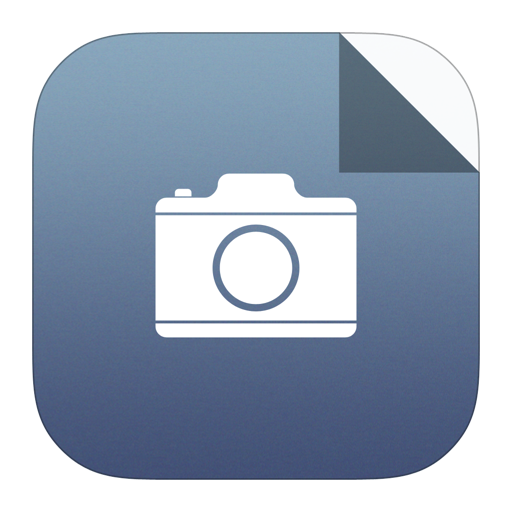
Larger image
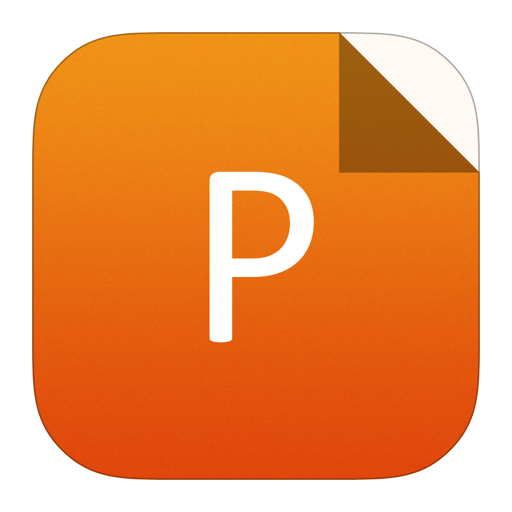
PowerPoint slide
Figure2.
(Color online) (a) Surface morphology and (b) cross-sectional images of the β-(Ga0.96Mn0.04)2O3 thin film.
Fig. 3 presents the EDS curve of β-(Ga0.96Mn0.04)2O3 thin films. From the spectra, elements of O, Al, Ga and Mn are observed, and no other elements are detected. The appearance of Al is ascribed to the sapphire substrate. It is obvious that Mn and Ga elements arise from the β-(Ga0.96Mn0.04)2O3 lattice. The Mn/(Ga+Mn) ratio is determined to 4.4%, which is close to the stoichiometric ratio of 4%. The result is approaching to the Mn concentration in our β-(Ga0.96Mn0.04)2O3 target, and it can also provide evidence that Mn ions have been well incorporated into Ga2O3 lattice[15].

class="figure_img" id="Figure3"/>
Download
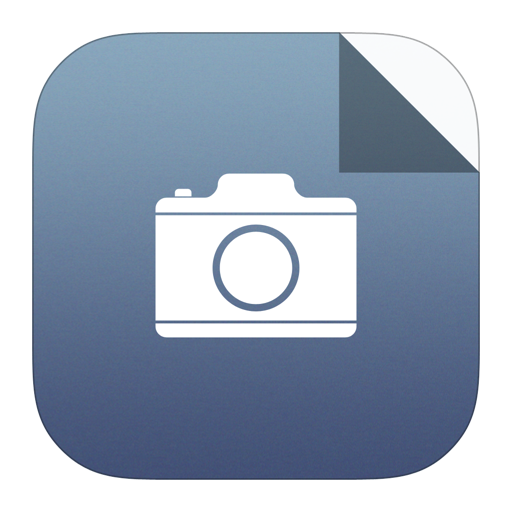
Larger image
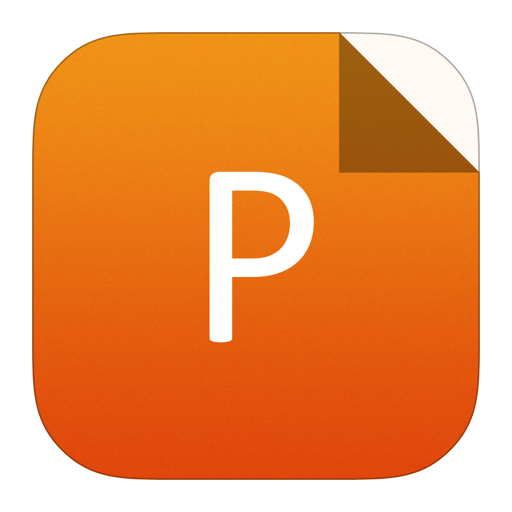
PowerPoint slide
Figure3.
(Color online) The EDS curve and analysis result (in the inset) of the β-(Ga0.96Mn0.04)2O3 thin film.
The optical absorption measurements are extremely important in evaluating the optical parameters of thin films such as absorption coefficient and energy bandgap. Fig. 4 shows the UV–vis absorbance spectrum of (Ga0.96Mn0.04)2O3 and pure Ga2O3 thin films. It is evident that the host curve exhibits a sharp absorption edge at 250 nm, whilst the spectrum of β-(Ga0.96Mn0.04)2O3 displays clear red-shift. It is known that β-Ga2O3 is a direct bandgap material and the bandgap can be modified by doping the Mn ions, since the incorporation of Mn ions on the substitutional sites of Ga may induce new unoccupied electron states in the gap below the conduction band edge[14]. The gap sizes can be derived by fitting the linear region of the (αhν)2 versus hν plot and taking the intercept on the hν-axis[27, 28], shown by the inset in Fig. 4. The bandgap values decrease from 4.99 eV for pure Ga2O3 to 4.47 eV for β-(Ga0.96Mn0.04)2O3, respectively.

class="figure_img" id="Figure4"/>
Download
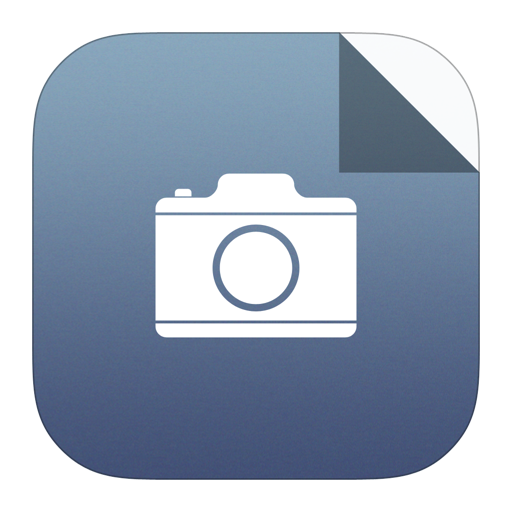
Larger image
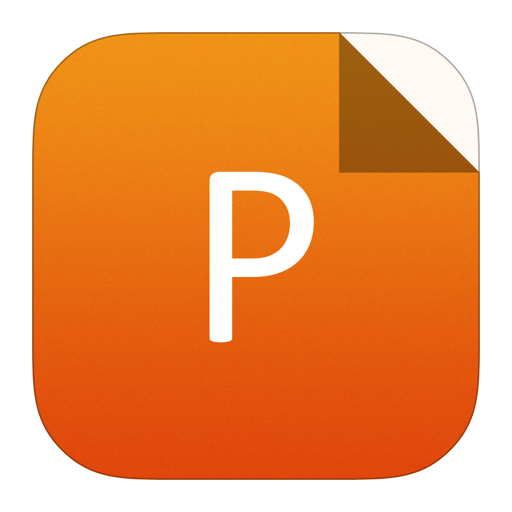
PowerPoint slide
Figure4.
(Color online) UV–vis absorbance spectrum of (Ga0.96Mn0.04)2O3 and pure Ga2O3 thin films with the plot of (αhν)2 versus hν for the samples in inset.
Fig. 5 shows the magnetization versus magnetic field (M–H) curves of (Ga0.96Mn0.04)2O3 and the pure Ga2O3 thin films at room temperature measured with the applied magnetic field parallel and perpendicular to the film. The diamagnetic contribution from the substrate has been subtracted from the data. For the pure Ga2O3 thin films, there appears little hysteresis loops which may due to the small amount of defects in films[29–31]. The β-(Ga0.96Mn0.04)2O3 thin film displays typical hysteresis loops indicative of ferromagnetism and exhibits obvious magnetic anisotropy. For example, the saturation magnetizations (Ms) for the case under parallel & perpendicular magnetic field at 5 kOe are 21.4 and 4.8 emu/cm 3, corresponding to magnetic moments as 0.76 & 0.17 μB/Mn, respectively. As seen in the enlarged image in the inset of Fig. 5 of M–H loops of β-(Ga0.96Mn0.04)2O3, the magnetic remanence (Mr) for the applied magnetic field parallel & perpendicular to the film are 1.69 & 0.52 emu/cm 3, respectively. The magnetic parameters of β-(Ga0.96Mn0.04)2O3 are listed in Table 1. In Hayashi’s results, γ-(Ga0.93Mn0.07)2O3 thin film has shown RT ferromagnetism with a magnetization of 8.6 emu/cm3 at 10 kOe under parallel magnetic field, and the magnetic moments is 0.42 μB/Mn, which may be attributed to different phase types[12]. As compared with our β-(Ga0.82Mn0.18)2O3 thin film prepared by an alternately depositing technology (Ms = 10.4 emu/cm3 and Mr = 1.6 emu/cm3 under parallel magnetic field), the concentration of Mn in this β-(Ga0.96Mn0.04)2O3 is lower but has a higher saturation magnetization and magnetic remanence, revealing apparent enhancement of ferromagnetism[14].

class="figure_img" id="Figure5"/>
Download
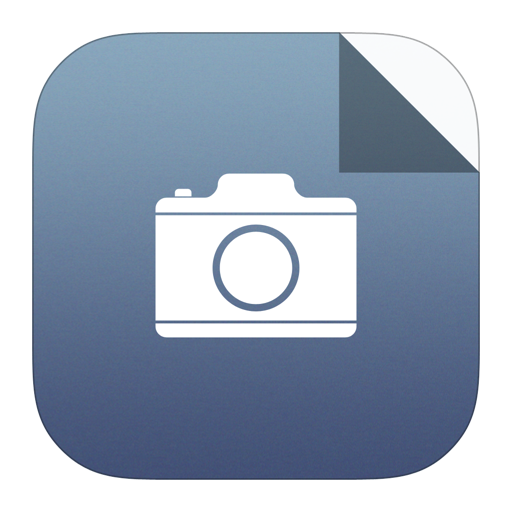
Larger image
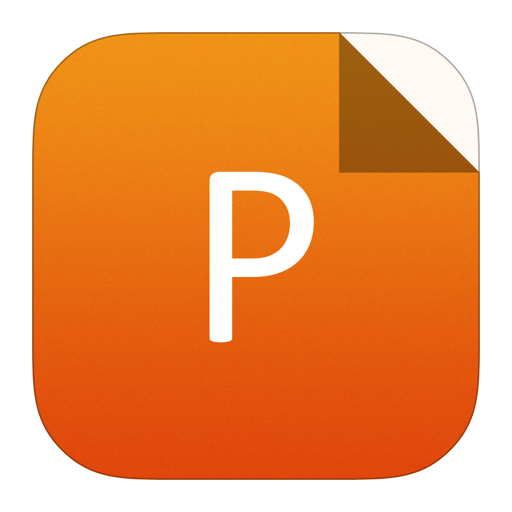
PowerPoint slide
Figure5.
(Color online) M–H curve of (Ga0.96Mn0.04)2O3 and pure Ga2O3 thin films and the corresponding enlarged image of β-(Ga0.96Mn0.04)2O3 (inset) with the applied magnetic field parallel and perpendicular to the film at room temperature.
Magnetic field direction | Magnetic moments (μB/Mn) | Mr (emu/cm3) | Coercivity (Oe) |
Parallel | 0.76 | 1.69 | 42.07 |
Perpendicular | 0.17 | 0.52 | 91.36 |
Table1.
The magnetic parameters of β-(Ga0.96Mn0.04)2O3 thin film.
Table options
-->

Download as CSV
Magnetic field direction | Magnetic moments (μB/Mn) | Mr (emu/cm3) | Coercivity (Oe) |
Parallel | 0.76 | 1.69 | 42.07 |
Perpendicular | 0.17 | 0.52 | 91.36 |
To prove the above conclusions and understand the impact of the Mn dopants on the structure and magnetic properties, a 1 × 3 × 1 super cell tripling the monoclinic unit along the b direction is modeled in Fig. 6(a). According to the total energy density functional theory calculation, one Mn ion substituting one Ga octahedral site is found in the most energetically stable, with a dopant concentration of 4.17%. The electronic band structure is given in Figs. 6(b) and 6(c), and the Fermi level is set at zero-point of energy. As shown in Fig. 6(b), the calculated width of the bandgap is 4.80 eV, approaching to theoretical value and our result in Fig. 4. From the total density of states (TDOS) pattern of the undoped Ga2O3, spin isotropy reveals that intrinsic Ga2O3 exhibits no magnetism. However, a small ferromagnetic behavior has been observed at room temperature in our pure Ga2O3 thin film, which could provide evidence that ferromagnetism in diluted magnetic oxide thin films are correlated not only with the concentration of transition element but also with nanostructures of films including crystallization, defects and oxygen vacancies[31]. Fig. 6(c) presents the 3d states of Mn and the TDOS of β-(Ga0.96Mn0.04)2O3 with a dopant concentration of 4.17%. The majority spin (up-spin) states are plotted along the positive y-direction, and the minority spin (down-spin) states are plotted along the negative y-direction. There is obvious spin anisotropy in TDOS pattern due to Mn doping, and the valued width of bandgap is about 4.39 eV (excluded an impurity state), which is close to our 4% Mn-doped Ga2O3 experiment result of about 4.47 eV in Fig. 4. The decrease of the bandgap is ascribed to new states appearing within the bandgap of pure Ga2O3 due to the Mn dopant replacing a Ga in an octahedral site[11, 32–34]. The results indicate that Mn doping can modify the bandgap and can induce spin anisotropy and vacancies in pure Ga2O3, which is important in Ga2O3-based magnetic-semiconductor devices.

class="figure_img" id="Figure6"/>
Download
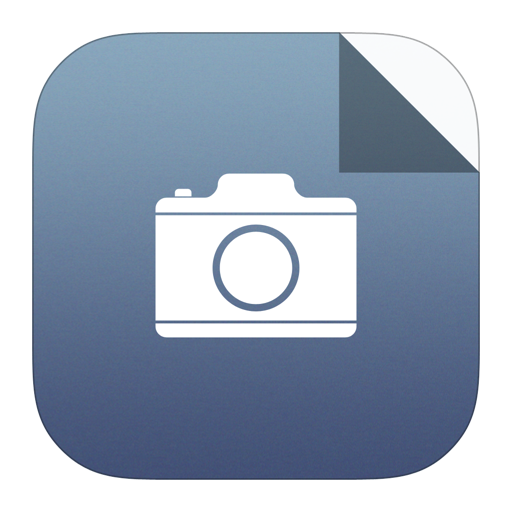
Larger image
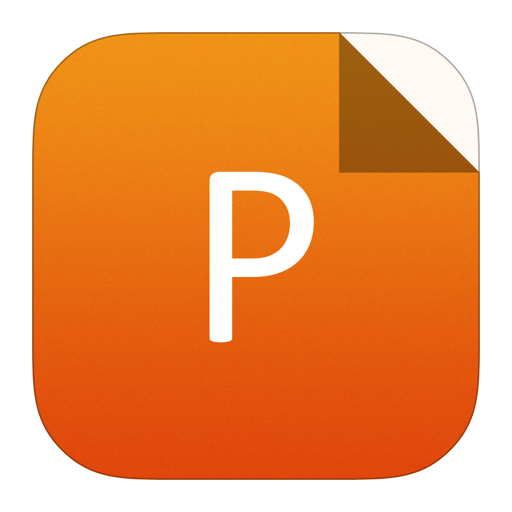
PowerPoint slide
Figure6.
(Color online) (a) Crystal structure diagram of (Ga0.96Mn0.04)2O3. (b) Band structure and TDOS plots of pure Ga2O3. (c) Mn replacing one octahedral sites in a 1 × 3 × 1 super cell with a dopant concentration of ~4%.
4.
Conclusion
In summary, pure Ga2O3 and (Ga0.96Mn0.04)2O3 thin films on sapphire substrates are deposited by L-MBE technique. XRD results revealed that the prepared thin films have monoclinic structure withpreferential orientation. The observed shift in XRD peak position confirmed the substitution of Mn into Ga2O3 lattice and revealed in high quality. Deposited thin films are smooth through the AFM images. A ferromagnetic behavior was observed at room temperature and explained by using the first principle method. The results are important both in the theoretical aspect to investigate the origination of ferromagnetism in dilute magnetic oxides and fabricating magnetic- semiconductor devices. Their distinct differences in magnetic properties provide evidence that ferromagnetism in magnetic semiconductors as Mn-doped Ga2O3 thin films are correlated not only with the concentration of transition element but also with crystallization of films, including defects and oxygen vacancies.