1.
Introduction
Ultraviolet (UV) photodetectors have attracted extensive attention due to their broad applications in biological and environmental fields, missile-launch detection and digital imaging, and optical communications[1–3]. The 1D nanostructure is capable of providing a big aspect ratio, direct transmitting channel for the charge transfer process[4–10]. By dint of this special geometrical and electrical efficiency, nanowires (NWs) are extensively utilized in UV photodetection. Considerable efforts have been devoted to fabricate NWs based UV photodetectors using semiconductors with a wide bandgap, such as ZnO, TiO2, SnO2, and ternary metal oxides[11–14]. 1D materials with heterostructures have properties not merely from each component, but also the occurrence of a reaction on the interface of each component while in comparison with pure semiconducting material[15–18]. Particularly, the 1D heterosturestures NWs based photodetector showed a much better photoresponse than that of the individual ones, as the photogenerated carriers can be spatially separated at the interface. For example, Tian et al. have made the new ZnS-ZnO branched nanostructures based photodetector that exhibits excellent performances compared with those of pure ZnS nanowires[19]. Chong and his co-workers designed a TiO2–ZnTiO3 heterojunction nanowire-based photodetector with increasing the device’s photosensitivity, spectra responsivity and external quantum efficiency[20]. Therefore, the construction of 1D heterostructures provided a significant pathway and new views to acquire optoelectronics devices with super performance.
In recent years, assembling the NWs array as great construction blocks can achieve the request of miniaturization and multiple functions for the photoelectric instruments[21]. Even though lots of strategies have been proposed, assembling perfectly arrayed NWs in an effective and inexpensive way remains a challenge[22]. The near-field electrospinning NWs array has been utilized in tissue engineering, solar batteries, sensing elements, transistors, and so on[23, 24]. The advantages of this new processing for manufacturing the 1D nano-structures are low cost, efficiency, and convenience to be assemblied into nanofilms[25]. ZnO and SnO2, as significant semiconducting material with wide band-gaps of 3.37 and 3.6 eV, have been researched in an intensive manner as the useful parts in the visible-blind UV photodetectors, on account of the high-performance of the photoelectronic property[11, 26]. The ZnO–SnO2 heterostructure NWs array may have the advantages for the UV photodetector, such as high stability, large Ion/Ioff ratio, and relatively fast response speed.
Hererin, we report the successful fabrication of UV photodetectors with 1D ZnO–SnO2 heterostructure array, obtained from the near-field electrospinning method. The as-fabricated photodetectors showed high Ion/Ioff ratio up to 103 with high response to ultraviolet incident light. All the parameters are determined by the numbers of the NWs. Besides, flexible photodetectors are also fabricated for the first time. Due to the robust flexibility and excellent stability, there is a strong possibility of them being used as the future flexible electronics.
2.
Experimental
2.1
Methods
2.1.1
Preparation of ZnO–SnO2 precursor solution
Firstly, Zn(NO3)2·6H2O (Aladdin, 99.9%) and SnCl4 (Aladdin, 99.9%) with a molar ratio of 1 : 1 are dissolved in a mixed solution that consisted of ethanol (99.7%) and dimethylformamide (DMF, 99.9%) with a volume ratio of 1 : 1. The mixed solution is processed under magnetic stirring until a uniform solution is obtained. Subsequently, polyvinylpyrrolidone (PVP, molecular weight of 1300000, Sigma-aldrich) with a concentration of 12 wt% in DMF solution is added in the above solution. Under powerful magnetic stirring for 4 h, a transparent and homogeneous solution is obtained. Then, 1 mL of the solution is sucked into the injection syringe to prepare for electrospinning.
2.1.2
Near-field electrospinning to prepare ZnO–SnO2 NWs
We choose two different substrates (SiO2 and Polyimide) as the collectors in order to study their various optoelectronic properties. The electrospinning parameters are different for different collectors. Here, the collectors should be grounded. A high voltage (+1.60 kV for SiO2 and +1.50 kV for polyimide) is applied to the stainless steel needle of the syringe and the top of the needle is controlled with a distance of 5 mm to the collector. When the Taylor Cone appears and it begins to spin, turn on the x-y moving stage to control the moving trajectory with a moving speed of 10 cm/s. In this way, aligned ZnO–SnO2 NWs arrays can be prepared on different substrates. The distance (> 5μm commonly) between two neighboring NWs can be adjusted by setting different y-axis values. To remove organic compositions and promote crystallization of grain, the NWs arrays on different substrates are calcined (at 550 °C for SiO2 and 400 °C for polyimide) for 2 h at air atmosphere. Then, the samples are cooled down to room temperature naturally and the ZnO–SnO2 NWs arrays are obtained.
2.1.3
Device fabrication and measurements
To investigate the optoelectronic properties of ZnO–SnO2 nanowires, the photodetectors are fabricated by a traditional photolithography process. 50 nm Au electrodes are evaporated thermally on both sides of as-prepared ZnO/SnO2 arrays. The photodetectors based on single wire and multi-wires on flexible and rigid substrates are fabricated by this method. A semiconductor parameter analyzer (Keithley 4200-SCS) with probe station is used to test the optoelectronic properties of ZnO–SnO2 devices. Ultraviolet light is emitted by a power adjustable light source system (CEL-HXF300) and an Ophir NOVA power meter is used to test the incident ultraviolet light intensity. All tests are finished at room temperature and in air atmosphere.
2.2
Characterizations
The size and morphology of the electrospun ZnO–SnO2 nanowires are observed by optical microscope (BX51, OLYMPUS) and scanning electron microscope (Zeiss, Supra55 (VP)). The composition of the products is analyzed by a powder X-ray diffractometer (XRD, Rigaku D/Max-2550, λ = 1.5418 ?). The flexible performance of the photodetector on polyimide substrate is tested by a one-dimensional moving stage, which can fix both ends of the devices.
3.
Results and discussions
The photodetectors based on ZnO–SnO2 hybrid NWs array were built by a modified near-field electro-spinning method. Due to the high voltage between the vessel outlet and the collector, two separate electrodes on the collector make the NWs assemble orderly (2a). Before electrospinning, the precursors were mixed with a polyvinyl pyrrolidone (PVP) solution, which was dissolved in dimethyl formamide (DMF). They were then annealed in air after the NWs array formed to remove excess PVP. The optical and SEM images of ZnO– SnO2 hybrid NWs are illustrated, respectively in Figs. 1(b)– 1(d). All NWs were assembled abreast forming a thin uniform layer. The density of the array could be adjusted by changing the electrospinning parameter, including the electrospinning time and the rate of precursor feeding. XRD was used to identify the compositions of the hybrid NWs (Fig. 2(e)). The XRD test result of the as-prepared 1D ZnO–SnO2 heterostructure, revealed peaks from only SnO2 (JCPDS No. 77-0447) and ZnO (JCPDS No. 36-1451) phases.

class="figure_img" id="Figure1"/>
Download
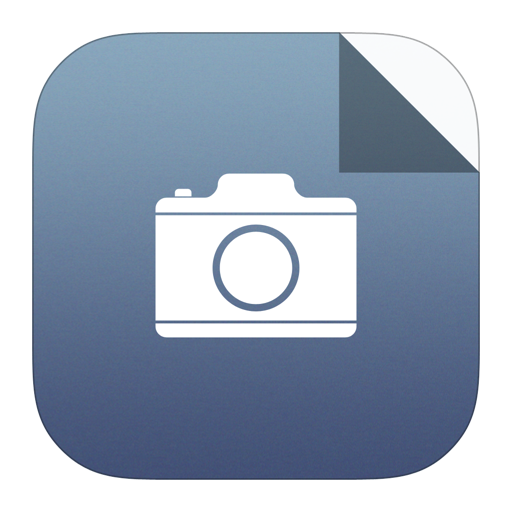
Larger image
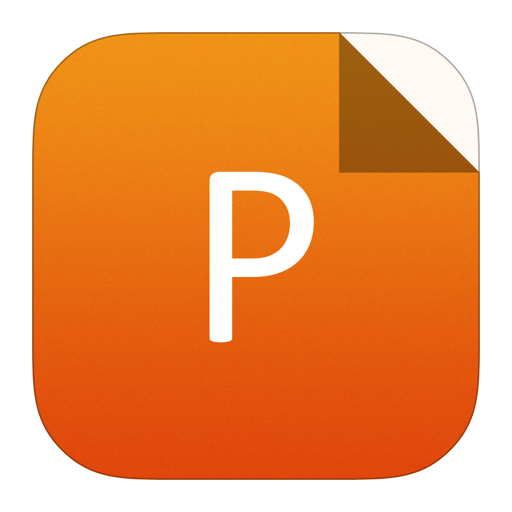
PowerPoint slide
Figure1.
(Color online) (a) Setup of the electrospinning machine. (b) Optical images of the as-synthesized ZnO–SnO2 heterostructure NWs array. (c, d) SEM image of ZnO–SnO2 heterostructure NWs array. (e) XRD pattern of the as-synthesized products.
As depicted in the Experimental Section, photodetectors were then built on individual 1D ZnO–SnO2 heterostructures according to the standard procedures. The schematic illustration in Fig. 2(a) is a single ZnO–SnO2 NW photodetector under illumination for measuring the photoresponsivity. Shown in Fig. 2(b) is the graph of current against voltage of the device in the dark and under light with different wavelengths. When the device was put under illumination, the photocurrent significantly increased. The dark current was approximately 200 fA, and the photocurrent could reach 300 pA when the device was exposed to light with a wavelength of 300 nm, exhibiting an ultrahigh Ion/Ioff ratio ~1.5 × 103. Furthermore, the device has also shown a great photoresponsivity under ultraviolet light with different wavelengths (280 to 350 nm). Fig. 2(c) illustrates the test result of the relationship between current and voltage of the photodetector in the dark and exposed to different intensities (1.3 to 3.4 mW/cm2) of 300 nm light irradiation. We can see that at the same voltage, the light current increases sharply with the increasing light intensities, because the absorbed photon flux adjusts the efficiency of photogenerated charge carrier. The relationship between photocurrent and light intensity is shown by a power law (Fig. 2(e)), I = APθ, which can be interpreted as follows: the photocurrent I, a constant A, the light intensity P and the empirical value θ. As shown in Fig. 2(d), the photocurrent is strongly correlated with light intensity. The value of θ is 0.96 (I~P0.96) with a voltage within 2 V, which demonstrates that the ZnO–SnO2 NWs possess an amazing photocurrent capability and linearity. Another important parameter of the photodetector is the time-dependent photoresponsivity. Fig. 2(e) illustrates that measurements were taken in four cycles at a voltage of 2 V with the testing condition switching between total darkness and illumination of 3.4 mW/cm2 UV light. It was also found that the photocurrent is not affected by switching on/off the illumination during the four cycles, showing that the photodetector has great stability and reproducibility. Fig. 2(f) illustrates one cycle from Fig. 2(e). The rise and decay time was defined as the time interval for the peak value of the photocurrent to change between 10% and 90%. Its values were about 162.5 and 75.5 s, respectively.

class="figure_img" id="Figure2"/>
Download
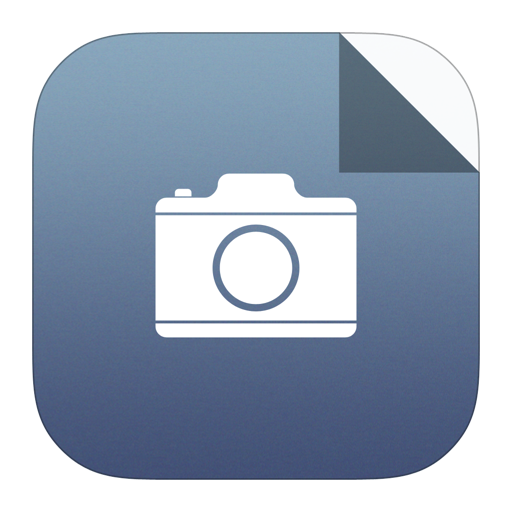
Larger image
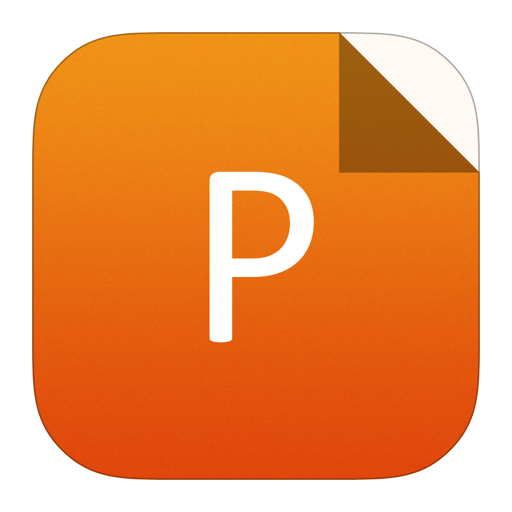
PowerPoint slide
Figure2.
(Color online) (a) Schematic illustration of as-fabricated photodetector. (b) I–V curves of the photodetector under various wavelength lights and in the dark. (c) I–V test result of the device under different power intensities of 300 nm light illumination and in the dark. (d) The dependence of photocurrent on light intensity. (e) Photoresponse characteristics of the device. (f) Enlarged view of (e).
Following are some explanations of the high photoresponsivity of the ZnO–SnO2 heterostructures. Firstly, a thicker charge depletion layer was formed because more oxygen molecules were absorbed by the 1D heterostructures with large surface area. This results in lower dark current. Secondly, the existence of the ZnO–SnO2 interface, which results in a junction barrier, further decreased the dark current. Moreover, the energy band alignment of the 1D ZnO–SnO2 heterostructure is also responsible for the performance improvement. Under light illumination, electron-hole pairs were generated and electrons were transferred to SnO2, forming a charge transfer state, which led to a spatial distribution of the charge carriers. The carrier density increases sharply and the barrier height decreases due to the separation of the photogenerated electron-hole pair. As a result, there was a decrease in the recombination rate of the carriers.
A hybrid NWs based photodetector can further enhance the photo sensing performance by increasing the number of the NWs of the array. Fig. 3(a) shows the I–V curves of different numbers of NWs. As illustrated, the photocurrents of the photodetector increased as the NWs increased, from 308 pA to 9.3 nA, 22.8, nA and 38.1 nA. The inset of Fig. 3(a) is a schematic diagram of the nanowire array photodetectors. The fitted curve shown in Fig. 3(b) reveals the good linearity of the photocurrent with the number of NWs changing. This result shows that we can provide different performances of the photodetector according to the needs of different application scenarios. Fig. 3(c) shows the single response and recovery curve of the 5, 10, and 20 NWs based photodetectors, respectively. As can be seen, the response and recovery time did not change with increasing the NWs. However, the photoresponse of the 20 NWs device had an obvious improvement. Fig. 3(d) illustrates the measurement of the photoresponsivity of the 20 NWs based photodetector by repeatedly turning on/off the 300 nm light. During the four given cycles, no significant change in photocurrent was observed, which indicates that the device has great stability and reproducibility.

class="figure_img" id="Figure3"/>
Download
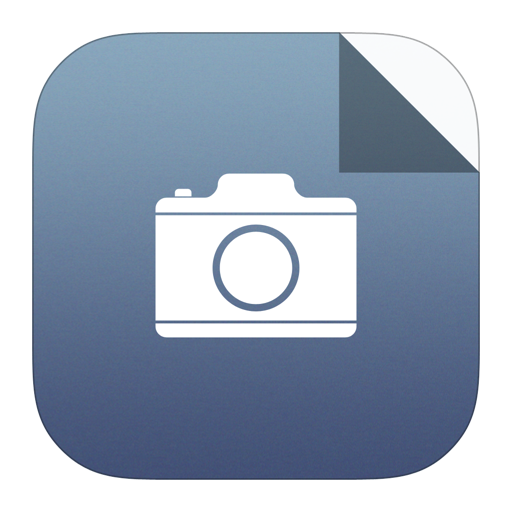
Larger image
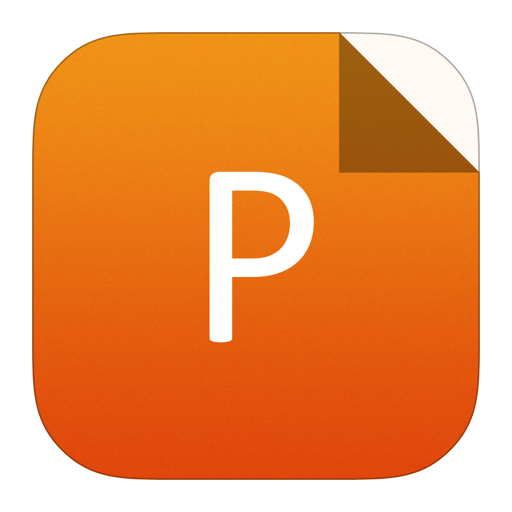
PowerPoint slide
Figure3.
(Color online) (a) I–V curves of the photodetector based on different numbers of NWs. (b) The dependence of photocurrent on the number of NWs. (d) Single photoresponse curve of 5, 10, 20 NWs. (d) Photoresponse characteristics of 20 NWs device.
Recently, portable and flexible electronics have greatly developed, resulting in that flexible sensors and detectors are urgently needed. To study the potential applications of ZnO–SnO2 NW in flexible electronics, ZnO–SnO2 NW based photodetectors were built on flexible PI substrate, as shown in Fig. 4(a), which is the schematic illustration of the ZnO–SnO2 NW array flexible photodetector. As shown in Fig. 4(b), the relationship between photocurrent and voltage of the flexible photodetector is investigated under the 300 and 350 nm light illumination and in dark conditions. It is observed that the flexible device was also highly sensitive to UV light with an excellent photoresponsivity. Meanwhile, the photocurrent significantly increases under 300 nm incident light at the identical voltage. As shown in Fig. 4(c), the recovery and response properties of the flexible device were also investigated. No significant change in photocurrent was observed, indicating that through the cyclic test the as-fabricated flexible photodetectors have high reproducibility. Moreover, as illustrated in Fig. 4(d), the values of the rise and decay time were 0.63 and 14.38 s, respectively. These behaviors are consistent with those of rigid SiO2/Si substrates. In addition, the reason the photoresponse of the device is a little decreased is the poorer contact between NWs and the flexible substrate. In the flexible device, the polymer PI was used as the flexible substrate. The contact between NW and PI substrate is poorer than that of NW and SiO2/Si substrate. This will degrade the device performance and result in a relatively low light current for photodetectors based on a flexible substrate compared to photodetectors based on SiO2/Si substrate.

class="figure_img" id="Figure4"/>
Download
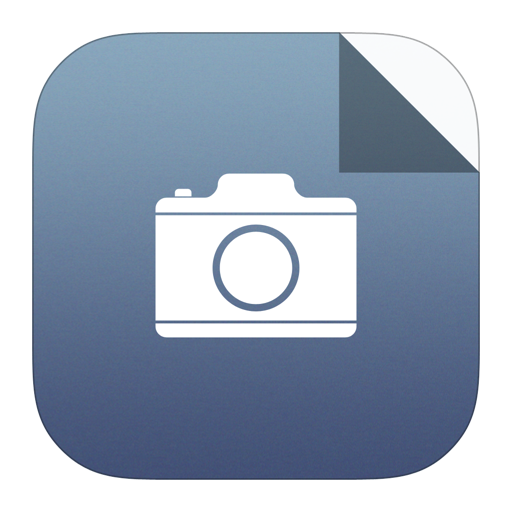
Larger image
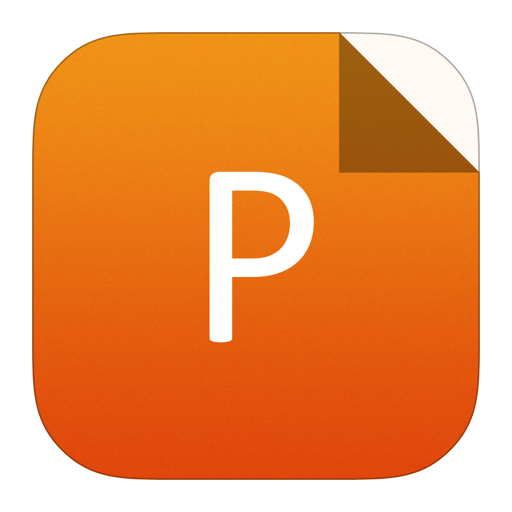
PowerPoint slide
Figure4.
(Color online) (a) Schematic illustration of as-fabricated flexible photodetector. (b) I–V curves of the flexible device under 300 and 350 nm light and in the dark. (c) Photoresponse characteristics of the device. (d) Enlarged view of (c).
The electrical stability is a key factor for the measurement of a flexible electrical device. Therefore, as shown in Fig. 5(a), we measured the photoresponse of the device under various curvatures. The different bending curvatures of the detector can be achieved by setting the two stages at different horizontal distances, which resulted in three different bending testing states that are marked as convex, concave and flat (insets in Fig. 5(a)), respectively. The I–V curves were measured at each state. No significant change in the curves was observed, indicating that the conductance of the device is not affected by bending stress. The observation concludes that the flexible device has great mechanical flexibility and electrical stability. Furthermore, measurements of the device were taken at a voltage of 2 V at different bending times for 50, 200, 500, and 1000 cycles, by switching between 300 nm UV light and total darkness. The flexible photodetector shows high photoresponsivity to UV light. Moreover, no significant change in the conductance of the flexible device was observed, showing that the flexible ZnO–SnO2 device has an outstanding folding tolerance. The measurements above have shown that 1D ZnO–SnO2 heterostructure based flexible photodetectors have an excellent flexibility, which means that they have the potential to be applied in future flexible and portable devices.

class="figure_img" id="Figure5"/>
Download
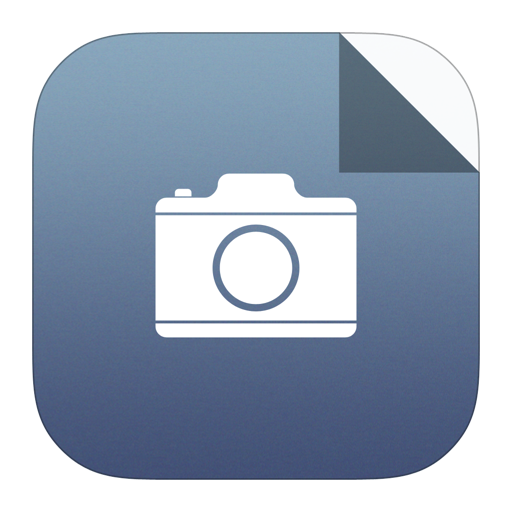
Larger image
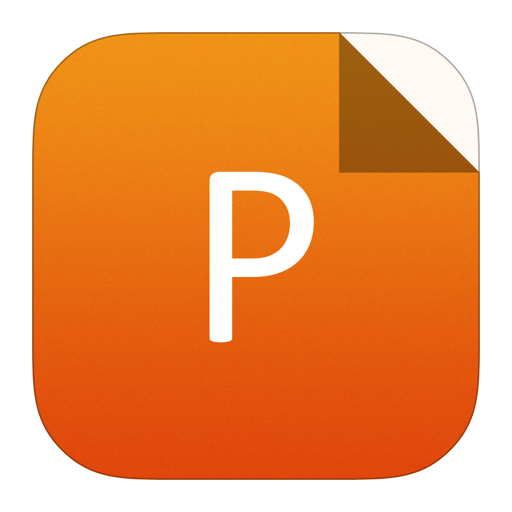
PowerPoint slide
Figure5.
(Color online) (a) The I–V characteristics of the flexible photodetector under different bending conditions. (b) Photoresponse characteristics of the flexible photodetector under different bending cycles.
4.
Conclusions
In summary, we fabricated high performance UV photodetectors using 1D ZnO–SnO2 heterostructures NW array as the sensing materials. As-fabricated devices exhibited a great photoresponse on the UV light (28–50 nm), which showed a good sensitivity to UV light. Flexible photodetectors were also fabricated on PI substate. Studies found that both of the two devices showed excellent properties such as highIon/Ioff and good reversibility, which may be due to the spatial separation of the photogenerated carriers at the interface within the NWs. In addition, the flexible devices exhibited good stable mechanical ability, even after a 1000-cycle bending test, the current still maintains the original value. Our results indicate that the presently constructed ZnO–SnO2 photodetectors in the UV spectrum with ultrahigh sensitivity, fast response speed and good flexibility have a great potential as photoelectronic switches, optoelectronic circuits and so on.