1.
Introduction
Flexible electronics have experienced dramatic development during the past decade and have shown the promising applications in health management and Internet of Things (ToT) applications which people, data, sensors and command are integrated together to enhance the living quality[1, 2]. Recently, positioning interfaces based on touch sensing devices, which are simulated by direct contact, are play more and more important roles in modern electronics[3]. However, compared with touch sensor, the touchless sensor provides more convenience, especially when users have no spare hand to control the sensor by directly touching it.
Several reported works have shown the promise of constructing a touchless control interface based on humidity sensitive materials such as H3Sb3P2O14[4], polyelectrolyte coating (poly (diallyldimethylammonium) (PDDA)/poly (styrenesulfonate) (PSS))[5], VS2[6], etc.[7, 8]. However, the fabrication method of these sensing materials is very complex and compared with capacitive sensor, the response speed of those reported touchless sensors is relatively slow (2–30 s). Therefore, seeking novel sensor with fast response time via simple fabrication method is still in demand. Graphene oxide (GO) is an oxidized derivative of graphene with many oxygen-containing functional groups (e.g. hydroxyl, epoxy and carboxyl group), which is a type of water-soluble insulating material that shows the potential to prepare touchless sensors[7–9]. Different from other reported flexible dielectric materials such as poly styrene[10], polydimethylsiloxane[11] and other polymer materials, GO can be easily obtained in large scale and the novel structural features of GO endow it with diverse unique chemical and physical properties for various applications. For example, the existing numerous functional groups allow GO to be functionalized with other materials to form GO-based composite material[12, 13] and work as precursor to modify other dielectric materials for enhancing their dielectric properties[11]. In addition, the π–π interaction between unfunctionalized areas of GO sheets and H-bonds between oxygen-containing functional groups can link the sheets together and assemble to macroscopic scale flexible films for further utilization[14, 15]. Several researchers have investigated the GO membrane as a functional membrane for selectively sieving gases or ions[16, 17]. As an insulating material, GO can also serve as a dielectric layer to fabricate capacitive sensor. Borini et al.[8] designed a capacitive humidity sensor based on GO with fast response time (~30 ms response and recovery time). Owing to the hydrophilic property of GO, the dielectric coefficient of GO increased after adsorption of water molecules and leaded to the increase of the capacitance of the sensor. Furthermore, as a water-soluble dielectric material, it is possible to prepare an all-print capacitive matrix sensor using GO dispersion as the ink and combine it with electronic printing technology in the future.
Recently, a great deal of effort has been made to assemble the water-soluble oxidized graphene sheets to form membrane-shaped film via various methods such as filtration[18–20], evaporation induced assembly[14], Langmuir-Blodgett assembly and spraying[20]. Dikin et al.[18] revealed a method to obtain GO film by filtering colloidal dispersion of GO sheets onto a membrane and then peeling it off after drying. The Young modulus of the obtained paper-like GO film was very high (32 GPa) and the tensile strain of GO paper was comparable to flexible graphite foils, but the thickness of GO paper was larger than 1 μm. Eda et al.[19] adopted a similar method to fabricate GO film by filtering the GO dispersion onto a substrate membrane and then dissolving the substrate membrane in acetone. The thinnest GO film was around 1–2 nm but the residual dissolved membrane may contaminate the GO film. Chen et al.[14] proposed a simple self-assembly approach to prepare GO films at a liquid/air interface by heating the GO dispersion. However, the prepared GO film was also thick (~0.5 to 20 μm). Cote et al.[20] employed the LB assembly method to fabricate GO film under alkali condition. Contributing to the edge-to-edge electrostatic repulsion formed between GO sheets under alkali condition, the GO sheets tended to assemble face-to-face together and thus the size of the formed GO film was rather small when compared with the above approaches. Although GO can be widely used in different areas, a facial method to controllably fabricate GO film in large scale is still in demand.
In this paper, we proposed a facile method to prepare ultrathin free-standing GO film (~12 nm) by filtrating GO dispersion (1.5 ml, 20 mg/L) on the PTFE/Ni(OH)2 substrate and then using diluted HCl solution (2 mol/L) to remove the Ni(OH)2 nanosheets and to exfoliate GO film from the substrate. The thickness of the GO film is controllable by changing the volume of the GO dispersion, and the obtained GO film can be used as a dielectric layer to construct highly sensitive flexible touchless sensor.
2.
Experimental
2.1
Materials and method
All of the chemicals were of analytical grade and were used directly without further purification. Nickel wires (Ni, > 99.5%), sodium chloride (NaCl, > 99.5%), ammonium chloride (NH 4Cl, > 99.5%), sodium hydroxide (NaOH, > 99.5%), potassium permanganate (KMnO 4, > 99.5%), graphite powder sodium nitrite (NaNO 2) and concentrated sulfuric acid (H2SO4, ~98%) were purchased from the Sinopharm Chemical Reagent Co.Ltd., China. Nickel wires were pre-treated in 1.0 M HCl solution for 30 s, ultrasonically washed in absolute ethanol for 15 min, and then dried naturally.
Ni(OH)2 nanosheets were fabricated by referring to our previous work[21],and the Ni(OH)2 nanosheets were then dispersed in deionized water (0.01 mg/mL) for further use. Graphene oxide nanosheets were synthesized via a modified Hummers method[12]. In a typical synthesis experiment, 18 mL concentrated H2SO4 was added into the mixture of 0.2 g graphite and 0.4 g NaNO2 under stirring. Then 0.8 g KMnO4 was gradually added into the three-necked flask which contains the mixture and an ice bath was used to control the temperature of the mixture to below 20 °C. The suspension was continuously stirred for 4 h at 35 °C. Then 100 mL H2O was added into the suspension and then reacted for 30 min at 95 °C before adding 3 mL H2O2 (30%). The obtained suspension was washed with diluted HCl (10%) and deionized water several times, respectively, until the residual water is neutral. Then the prepared graphene oxide (GO) was dispersed in deionized water and sonicated via ultrasonic probe.
Free-standing GO film was prepared via filtrating Ni(OH)2 nanosheets and GO dispersion onto the hydrophilic polytetrafluoroethylene (PTFE) membrane in sequence, followed by gradually immersing the obtained PTFE/Ni (OH)2/GO film into diluted HCl solution (2 mol/L) to remove the Ni(OH)2 nanosheets substrate. The free-standing GO film was then transferred onto a flexible PET substrate with patterned gold electrodes, and then subsequently deposited patterned gold electrodes on the top of GO film to construct the flexible capacitive sensor.
2.2
Characterization and measurements
The prepared GO film was characterized by X-Ray diffraction (XRD), atomic force microscopy (AFM) and scan electron microscopy (SEM). The XRD analysis was performed using a BRUKER D8 Discover X-Ray diffractometer with Cu-Ka Radiation (λ = 1.5405 ?). The capacitance–time curves (C–T curves) were measured with semiconductor parameters test instruments (Agilent B1500A).
3.
Results and discussion
The obtained GO sheets are characterized with X-ray diffraction, Raman spectroscopy and energy dispersive X-ray spectroscopy (EDX) as shown in Fig. S1. The (001) peak of GO appear at 11.25° (Fig. S1(a)) indicating the d-space between interlayer is 0.79 nm attributing to the existing oxygen-containing functional groups[7, 22]. The Raman spectrum of GO has two major peaks (Fig. S1(b)). The peak at 1331 cm?1 is the D mode from a breathing mode of k-point phonons of A1g symmetry which is attributed to the defects introduced during graphite oxidation, and the peak at 1597 cm?1 arises from the first order scattering of E2g phonon of sp2 C atoms[23, 24]. EDX shows the C : O atom ratio is 0.88 (Fig. S1(d)) indicating the high oxidation level of GO.
The typical fabrication process of free-standing GO film is shown in Fig. 1. First, Ni(OH)2 nanosheets are fabricated based on the electrochemical method[27]. Before filtering GO dispersion, 10 mL 0.01 mg/mL Ni(OH)2 dispersion is filtered to serve as the sacrifice layer. By gradually inserting PTFE/ Ni(OH)2/GO membrane into diluted HCl solution (2 mol/L), the Ni(OH)2 layer will be dissolved and then the GO film will automatically peel off from the PTFE membrane. As the Ni(OH)2 nanosheets can be thoroughly etched by HCl, the surface of the obtained GO film is very clean. This method has a good reproducibility owing to the facile approach to synthesize Ni(OH)2 nanosheets and prepare GO dispersion.

class="figure_img" id="Figure1"/>
Download
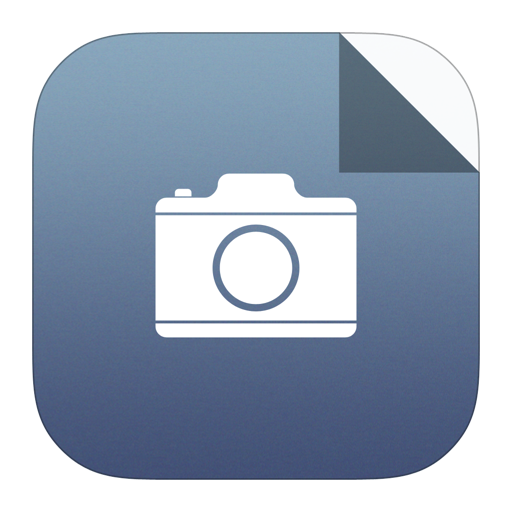
Larger image
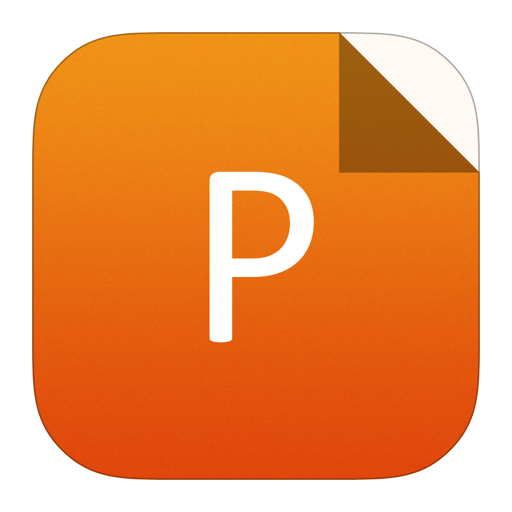
PowerPoint slide
Figure1.
(Color online) Schematic of the fabrication process of the free-standing GO film.
SEM images at different stages (bare PTFE membrane, PTFE/Ni(OH)2 membrane, PTFE/Ni(OH)2/GO membrane and GO film) are characterized in order to investigate the formation process of GO film. Fig. 2(a) is the photograph of PTFE/ Ni(OH)2 membrane. Compared with bare PTFE membrane, the color of the membrane changes from white to light green after filtering Ni(OH)2 nanosheets (10 mL, 0.01 mg/mL). According to our previous work, the thickness of the obtained Ni(OH)2 nanosheets is ~6 nm[24]. For the bare PTFE membrane, there are many micropores (~0.45 μm) distributed on the surface of the membrane as shown in Fig. 2(b). After filtering Ni(OH)2 nanosheets, the nanosheets stack together and cover on the PTFE membrane which can reduce the size of the pores and make the PTFE membrane become smoother (Fig. 2(c)). The amount of Ni(OH)2 nanosheets can significantly influence the quality of the GO film. The GO film cannot successfully exfoliate from the membrane if there are not enough Ni(OH)2 nanosheets to completely cover the pores of the PTFE membrane. By studying the morphology of the PTFE/Ni(OH)2 membrane with different volume of Ni(OH)2 suspension, the optimized volume of the Ni(OH)2 dispersion is 10 mL (0.01 mg/mL) (Fig. S2(d)), as the amount of Ni(OH)2 nanosheets are not enough to uniformly cover the surface of PTFE when the volume of Ni(OH)2 is less than 10 mL (0.01 mg/mL) (Figs. S2(a)–S2(c)). Fig. 2(d) is a picture of PTFE/Ni(OH)2/GO which is prepared by filtering 10 mL GO suspension (20 mg/L) onto the surface of PTFE/Ni(OH)2. As shown in Fig. 2(d), the color of the membrane changes to light brown from light green. Fig. 2(e) is the SEM image of the PTFE/Ni(OH)2/GO membrane, some wrinkles are formed at the edges of sub-micro level pores of the bare PTFE membrane and these wrinkles can be observed on the surface of the obtained GO film in Fig. 2(f). Due to the high transparency of GO film, Ni(OH)2 nanosheets which are covered by GO film can be recognized in Fig. 2(e).

class="figure_img" id="Figure2"/>
Download
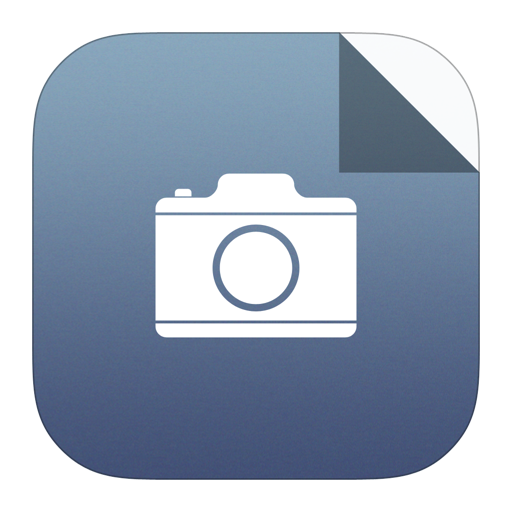
Larger image
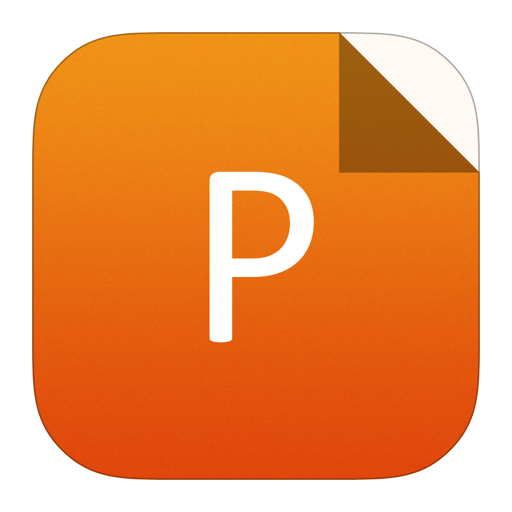
PowerPoint slide
Figure2.
(Color online) A photograph of the PTFE/Ni(OH)2 membrane which is filtered with 10 mL 0.01 mg/mL Ni(OH)2 nanosheets solution. (b), (c) SEM images of bare PTFE membrane and PTFE/Ni(OH)2 membrane, respectively. (d) A photograph of the PTFE/Ni(OH)2/GO membrane by filtering 10 mL 20 mg/L GO dispersion onto the PTFE/Ni(OH)2 membrane. (e), (f) SEM images of PTFE/Ni(OH)2/GO membrane and the obtained GO film, respectively.
The thickness of GO film is tuned by adjusting the volume of GO dispersion. As shown in Figs. 3(a)–3(d), the color of the GO film become darker with the increase of GO dispersion volume, indicating the thickness of the GO film also increases. Due to the strong π–π interaction and H-bonds between GO sheets[25, 26], GO film has a good stability without disintegrating in the diluted HCl solution. Moreover, the acid condition can restrict the hydrolyzing effect and suppress the electrostatic repulsive force, which is caused by the ionized carboxylic and phenol hydroxyl groups, so it can further strengthen the bonding force between the GO sheets[20]. Fig. 3(e) demonstrates the ultraviolet-visible light transmittance spectroscopy of the as-prepared GO film with different thicknesses. The results show that the transmittance declines significantly with the increase of the GO film thickness. The transmittance of 12 nm GO film can reach around 99% from the wavelength of 350 to 1100 nm. For all these GO films, the transmittance can reach above 80% between 500 and 1100 nm.

class="figure_img" id="Figure3"/>
Download
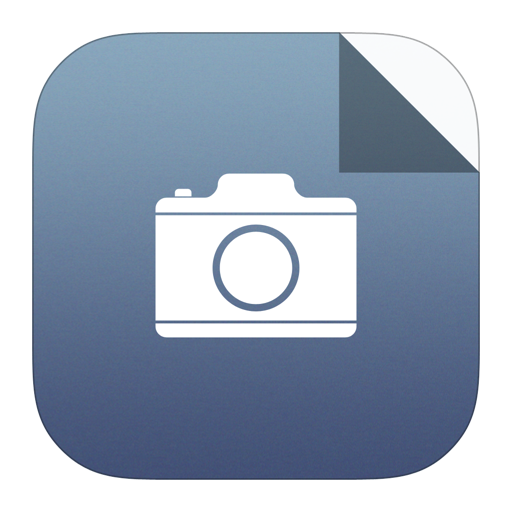
Larger image
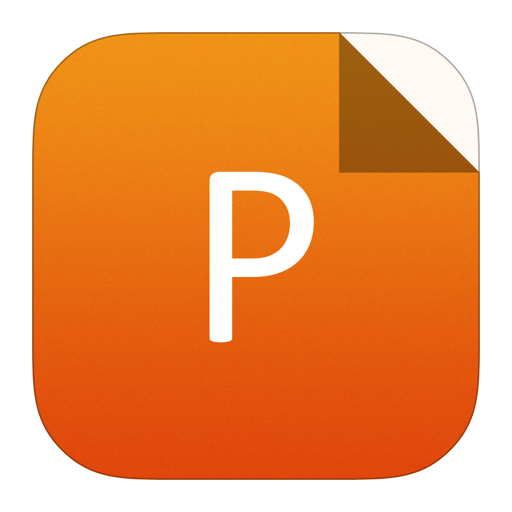
PowerPoint slide
Figure3.
(Color online) (a)–(d) The photographs of the prepared GO films with different thickness by tuning the volume of GO dispersion (20 mg/L) which are transferred onto PET substrates: (a) 1.5 mL, (b) 2 mL, (c) 3 mL, and (d) 10 mL. (e) Transmittance of the GO films which are obtained with different GO dispersion volume (300 to 1200 nm).
The thickness of the prepared GO film is measured by AFM, which is shown in Fig. 4. From the height profile curves of the GO films, when filtering 1.5 mL 20 mg/mL GO dispersion, the thickness of the obtained GO film is around 12 nm. By comparison with the other reported methods listed in Table 1, the thickness of GO film in this work is very small. When the volume of GO dispersion increases to 5 mL, the thickness of GO film reaches to ~50 nm (Fig. S3) and reaches to ~100 nm when filtering 10 mL GO dispersion (Fig. 4(b)). Therefore, the thickness of GO film has a linear relationship to the amount of GO dispersion and is very controllable.

class="figure_img" id="Figure4"/>
Download
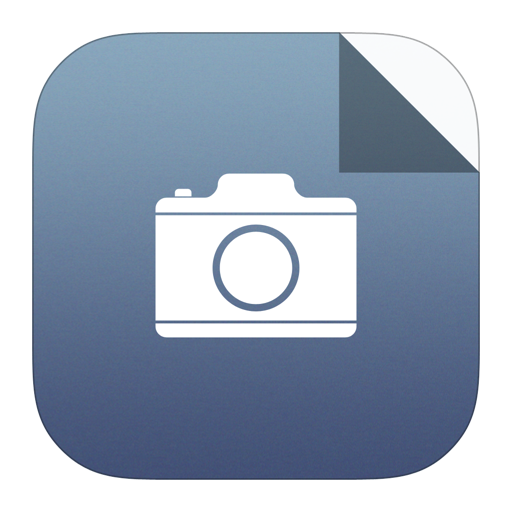
Larger image
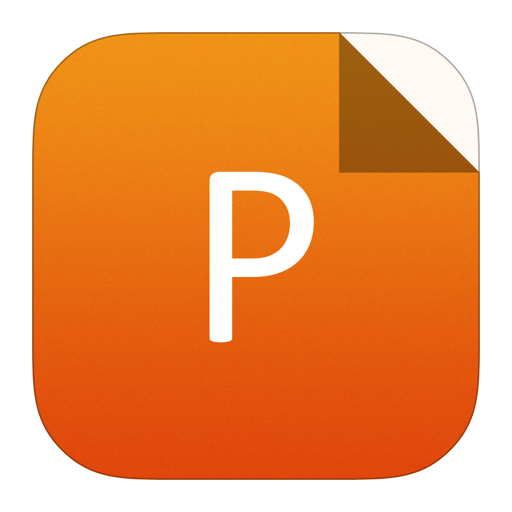
PowerPoint slide
Figure4.
(Color online) The AFM images and height profile of prepared GO films with different volume of GO dispersion (20 mg/mL): (a)1.5 mL, (b) 10 mL.
Method | Thickness | References |
Filter | 2 nm | [19] |
Spin-coating | 3–10 nm | [16] |
Spray- or spin-coating | 0.1 μm | [17] |
Vapor induced assemble | 0.5 μm | [14] |
Filter | 1 μm | [18] |
Chemical ‘stitching’ | 1 μm | [27] |
Vacuum drying | 5 μm | [28] |
Filter | ~12 nm | Our work |
Table1.
The least thickness of GO films obtained via different fabrication method.
Table options
-->

Download as CSV
Method | Thickness | References |
Filter | 2 nm | [19] |
Spin-coating | 3–10 nm | [16] |
Spray- or spin-coating | 0.1 μm | [17] |
Vapor induced assemble | 0.5 μm | [14] |
Filter | 1 μm | [18] |
Chemical ‘stitching’ | 1 μm | [27] |
Vacuum drying | 5 μm | [28] |
Filter | ~12 nm | Our work |
As a flexible insulating material, GO film has been used as the dielectric layer and sensing material in capacitive sensors[29]. In this work, a touchless capacitive sensor based on GO dielectric film is prepared. Fig. 5(a) displays the schematic graph of the flexible sensor in which one pair of Au electrodes (0.25 cm2) works as the panel electrodes on both sides of GO film and a PET film servers as the flexible substrate of the sensor. Figs. 5(c)–5(e) are the C–T responses of the sensor to different approaching objects and the test frequency is 1 MHz. When an insulator gradually approaches from 3 cm height above the sensor, the sensor does not show any response (Fig. 5(c)). However, when approached objectives switch to conductors such as metals and hands, the capacitance of the sensors decreases rapidly (Figs. 5(d)–5(e)), which is due to the approaching conductor, and can affect the distribution of the electronic field around the sensor[30]. A series of pictures and a scatter plot which are showing the process of capacitance changing when the hand approaching the sensor are provided in Fig. S4 and Fig. S5. The changing value of the sensor becoming more obvious when the distance between hand and sensor are more and more smaller and this phenomenon can also be recognized in Refs. [3, 31].

class="figure_img" id="Figure5"/>
Download
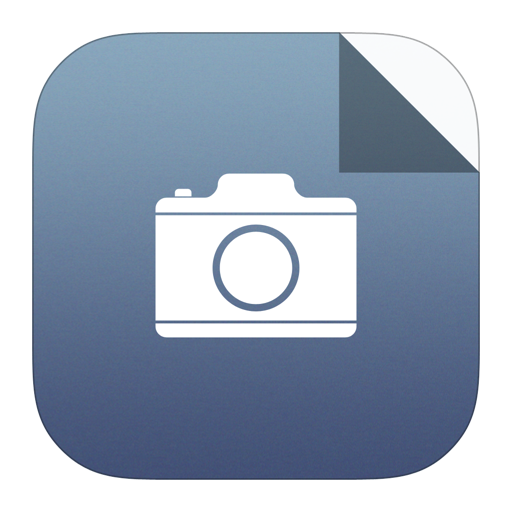
Larger image
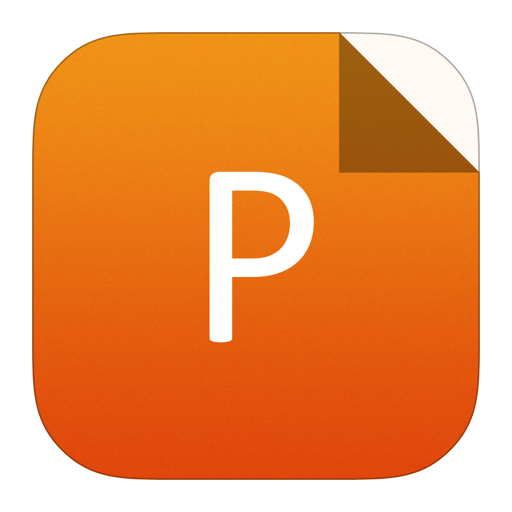
PowerPoint slide
Figure5.
(Color online) (a) The schematic graph and (b) photograph of the capacitive sensor based on GO film. Gold electrodes work as the panel electrodes on both sides of GO film; capacitance time (C–T) response to different objects when they are close to the sensor: (c) insulator, (d) conductor, (e) hand.
Fig. 6 is the schematic graph of the electrical field distribution of the sensor before and after the conductor approaches the top electrode. Before the conductor approaches to the sensor, the main electrical field is formed between the panel electrodes (top and down electrode) and a small amount of electrical field formed at the fringe of the electrodes (fringe-field) as shown in Fig. 6(a). The ideal of the capacitance of a panel capacitor is defined as:
$$C = frac{Q}{U}.$$ ![]() | (1) |
So the capacitance between the panel electrode is determined by the number of the charges (Q) on the electrode and the voltage which is applied on the electrodes (U). However, when a conductor approaches to the top electrode of the sensor, the electrical field of the sensor will be affected and form another electrical field between the conductor and the top electrode. Garbini et al.[32] reported a method to measure the surface profile and the hole geometry using fringe-field capacitive and claimed that the capacitance generated by the fringe-field electrode would only be tuned by the vertical distance (h) to the flat surface profile and when the distance between the electrode and the surface becomes small, the capacitance increases rapidly. Fig. 6(b) is the schematic graph of the sensor when the conductor vertically approaches to the sensor according to the theory given by Garbini et al.[32]. In this case, the capacitance between the top electrode and the conductor is also tuned by the distance between the top electrode and the conductor, and the relationship between the capacitance (C1) and h is given by the following formula[32].

class="figure_img" id="Figure6"/>
Download
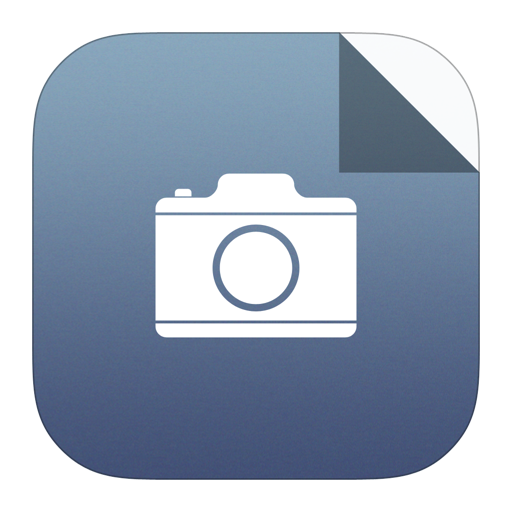
Larger image
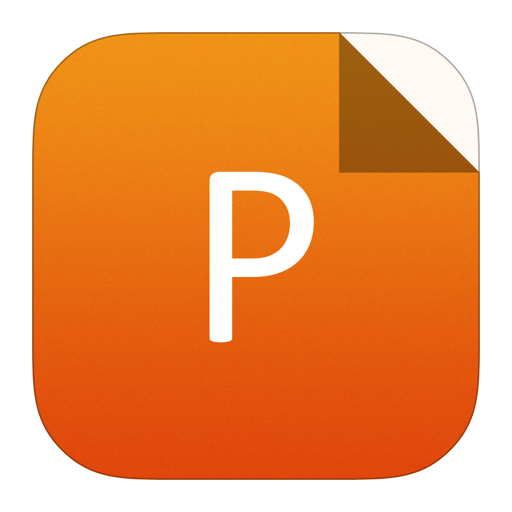
PowerPoint slide
Figure6.
(Color online) The schematic graph of the electrical field distribution of the capacitive sensor (a), and the electrical field distribution of the top electrode when the conductor is close to the top electrode (b).
$$C = frac{{4varepsilon W}}{pi }ln frac{{2H}}{h}.$$ ![]() | (2) |
Here h is the distance between the top electrode and the conductor, while H and W are the height and the width of the conductor.
When the conductor approaches to the top electrode, the capacitance between them (C1) increases rapidly. That means more charges of the top electrode are depleted to produce C1, and therefore the number of charges (Q) between the panel electrodes decreases, which leads to the rapidly decreasing of the capacitance of the panel capacitive touchless sensor (C0). By contrast, when the conductor departs from the top electrode of sensor, C1 will decrease according to Eq. (2), and therefore the less charges are depleted producing C1. In the result, the number of charges on the panel capacitive touchless sensor increases relatively, causing the increasing of the capacitance value of the sensor (C0).
4.
Conclusion
An ultrathin GO film was successfully prepared by a novel and simple filtration method. The thickness of the ultrathin GO film is controllable by changing the amount of GO dispersion. The thinnest film reached is thin as 12 nm, and its corresponding transparency is around 99%. Owing to the excellent physical properties of GO film (insulativity and flexibility), GO film was used as the dielectric material for preparing flexible capacitive sensor which can distinguish the approaching of the conductor or insulator. The sensing mechanism is due to the influence of the fringe-field capacitance formed between the top electrode and the approached object.
Acknowledgment (Optional)
Lin Liu and Yingyi Wang contributed equally to this work. The authors acknowledge the funding support from the National Natural Science Foundation of China (No. 61574163) and the Foundation Research Project of Jiangsu Province (No. BK20160392 and No. BK20170008). The NANO-X Workstation scientifically supported this research. This infrastructure would not be possible without the significant contributions of the Chinese Academy of Sciences, Jiangsu Province, Suzhou City, Suzhou Industrial Park.