1.
Introduction
Electrically conductive circuits as the fundamental components to power the electronic devices have great influence on the device performance. The most extensively used electric circuits that dominate the current electronic markets are commonly based on printed circuit boards (PCBs). The conventional PCBs are usually fabricated via a tedious process commonly involving photolithography and/or chemical etching, which is complicated, cost-intensive and pollutes the environment. Moreover, they are mainly fabricated on comparatively rigid substrates without arbitrary deformability and compliance. Recently, flexible electronics have attracted extensive attention from both the industry and academia because of their fascinating and unique properties, such as light weight, portability and especially the mechanical compliance, namely the capability to be bent, twisted, stretched and compressed without degradation of performance. Typical examples include implantable biomedical devices[1, 2], health-monitoring sensors[1, 3, 4], artificial intelligence[5], epidermal electronics[6] and flexible displays[7]. The development of these flexible electronics requires the conductive interconnects to possess the ability to be highly deformed while remaining the superior electrical conductivity. Apparently, the tedious fabricating methods and the brittle nature of conventional PCBs drastically constrain their further applications in low-cost and large-area fabrication of flexible electronics.
As a novel manufacturing technology, inkjet printing exhibits multifarious advantages compared with conventional fabrication methods[8–12]. It is a typical additive manufacturing, saving raw materials and reducing cost. It is also compatible with the roll-to-roll technique and easy to realize large-area production. Additionally, inkjet printing is generally controlled by computers, which facilitates patterning through an automatic process. The obvious advantages of inkjet printing render it rather attractive for manufacturing large-area flexible circuits. Silver inks based on silver nanoparticles manifest great promise in printed flexible circuits because of their high electrical conductivity and small sizes[9, 13]. However, current printed silver lines usually show relatively coarse resolution due to the inhomogeneous morphology and the large feature sizes, both of which are highly dependent on the wettability of substrates, the attribute of inks, as well as the way of droplet generation. For instance, polyethylene terephthalate (PET) is a typical substrate for flexible electronics. However, the droplets printed on PET are apt to spread, resulting in a larger base diameter. The relative coarse resolution of the printed patterns greatly restricts the application of inkjet printing in designing exquisite circuits. Much recent effort has been paid to enhance the resolution, including optimizing the formulation of inks[14], modifying the wettability of substrates[15–17], and developing novel printing apparatus[18]. Nevertheless, they are relatively complicated, lacking in versatility, which are still not feasible for cost-effective scalable fabrication. A facile methodology to enhance the printing quality of inkjet printing is highly demanded.
In this manuscript, a simple methodology was developed to enhance the patterning resolution of inkjet printing, involving process optimization and substrate modification and treatment. The width of the inkjet-printed silver lines was successfully reduced to 1/3 of the original value using this methodology. Moreover, large-area flexible circuits with delicate patterns and good morphology were thus fabricated. The resultant flexible circuits exhibited excellent electrical conductivity as low as 4.5 Ω/□ and strong tolerance to mechanical bending, showing great potential in replacing the conventional PCBs. The simple methodology is suitable for treating substrates with various wettability, which suggests a general strategy to enhance the patterning resolution of inkjet printing for manufacturing high-performance large-area flexible electronics.
2.
Methods to enhance the resolution
2.1
Optimization of the process parameters
The silver inks were purchased from the Advanced Nano Products (ANP) Co., Ltd and the ink properties are summarized in Table 1. A Dimatix Materials Printer (DMP) 3000 series supplied by FUJIFILM was used, and the nozzle diameter was around 20 μm in this work. The driving wave form used to print silver inks is illustrated in Fig. 1(a). PET was selected as the substrate due to its various merits, including low cost, high transparency and superior flexibility. However, its comparatively high wettability can greatly affect the spreading of the inkjet-printed silver lines, leading to the real line width being much larger than the pre-set value (the pre-set line width was 10 μm). Within a printing cycle, a droplet was ejected from the nozzle, impacting on the substrate and eventually spreading or retracting on the surface. In this process, the wettability of the substrate, the property of inks and the way of droplet generation play important roles in the printing quality of the printed dots or lines. In this regard, two main methods are used to constrain the spreading of the printed silver lines: one is to optimize the process parameters, such as the substrate temperature and the drop spacing; the other is to modify the substrate.
Solid content (%) | Viscosity (cPs) | Surface tension (mN/m) | Solvent | Particle size (nm) |
30–35 | 10–17 | 35–38 | Triethylene glycol monoethyl ether | 50–80 |
Table1.
Specification of silver inks.
Table options
-->

Download as CSV
Solid content (%) | Viscosity (cPs) | Surface tension (mN/m) | Solvent | Particle size (nm) |
30–35 | 10–17 | 35–38 | Triethylene glycol monoethyl ether | 50–80 |

class="figure_img" id="Figure1"/>
Download
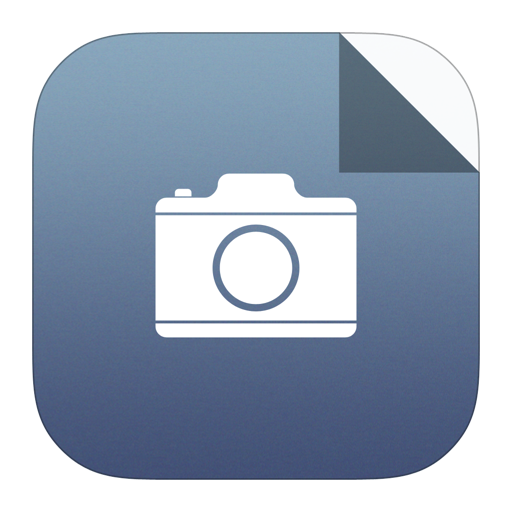
Larger image
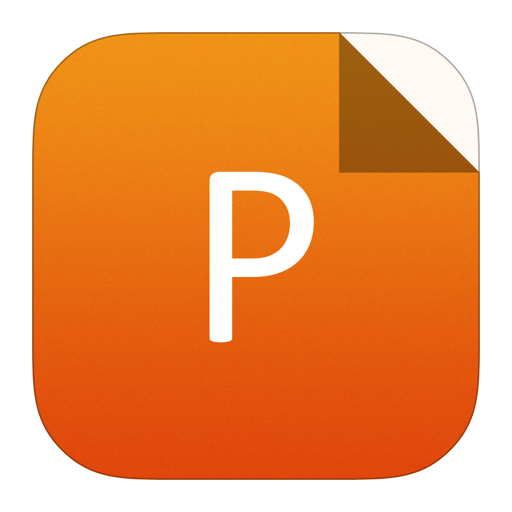
PowerPoint slide
Figure1.
(Color online) (a) The waveforms used to print silver lines. (b) The optical image of the inkjet-printed silver lines on PDMS/PET substrate without plasma treatment, splitting into a series of scatters.
Fig. 2 shows the optical microscopic images (taken directly from the camera of the inkjet printer) of a series of silver lines printed on pristine PET substrates while modulating the drop spacing from 20 to 50 μm and the substrate temperature from 35 to 55 °C. As illustrated in Fig. 2(a), the silver lines spread to ~150 μm despite the pre-set value being 10 μm with the substrate temperature of 35 °C and the drop spacing of 20 μm. Intriguingly, the line width reduced significantly from ~150 to ~95 μm (Fig. 2(i)) when fixing the drop spacing at 20 μm and increasing the substrate temperature from 35 to 55 °C. At a specific drop spacing, the line width gradually decreased with the increment of the substrate temperature. Under this circumstance, increasing the temperature greatly accelerated solvent volatilization, and the printed lines rapidly solidified, which therefore restricted the spreading of the lines. However, the allowed temperature on the platen is up to 60 °C, which is limited by the printer itself. The highest temperature used in this work was 55 °C. When we set the temperature to 55 °C and changed the drop spacing from 20 to 50 μm, the line width decreased from ~95 to ~70 μm (Fig. 2(l)).

class="figure_img" id="Figure2"/>
Download
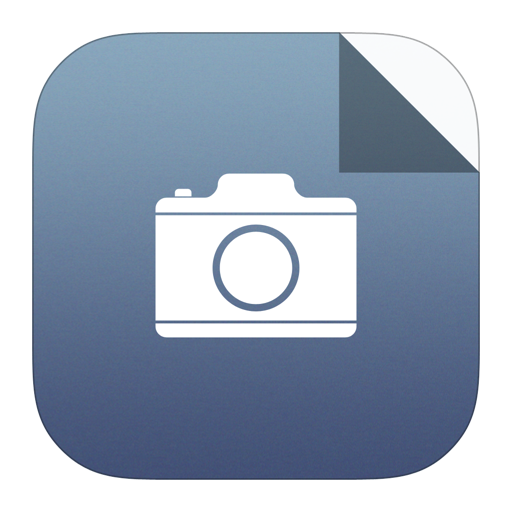
Larger image
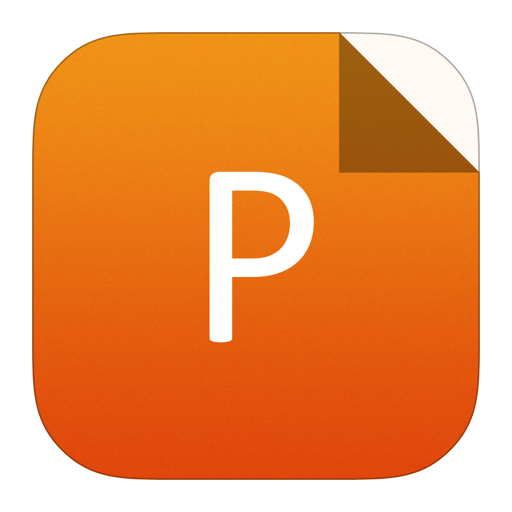
PowerPoint slide
Figure2.
(Color online) Optical microscopic images of the printed Ag lines on PET substrate, showing the line width changing with the drop spacing and the substrate temperature.
2.2
Substrate modification and treatment
To further reduce the line width, an ultra-thin hydrophobic polydimethylsiloxane (PDMS) layer was spin-coated onto PET substrate (PDMS/PET). The PDMS layer was spin-coated at 8000 rpm for 15 s followed by curing at 120 °C for 2 min. However, the inkjet-printed silver lines split into a series of scatters because the printed silver droplets tend to recede on the highly hydrophobic PDMS surface, as illustrated in Fig. 1(b). The PDMS/PET substrate was thus treated with oxygen plasma to enhance the wettability. The Plasm-Preen System supplied by Mycro Technologies was used to improve the wettability of the PDMS/PET substrates. To systematically investigate the influence of substrate wettability on the line width, the PDMS/PET substrate was treated by oxygen plasma for 3, 5 and 10 s, respectively, while keeping the power constant at 700 W. Fig. 3 shows the line width of the printed silver lines changing with the drop spacing and the time of plasma treatment while fixing the substrate temperature at 55 °C. It was observed that the wettability of the PDMS/PET substrate was improved via oxygen plasma treatment for just a few seconds. Compared with those scatters on the PDMS/PET substrate without plasma treatment, the silver lines printed on the treated PDMS/PET substrate were well-connected and uniformly distributed. It could also be found that decreasing the time of plasma treatment was favorable for acquiring narrower line width. As shown in Fig. 3(l), the line width was further reduced from ~70 to ~48 μm when the PET substrate was modified with an ultra-thin PDMS layer followed by plasma treatment for 3 s.

class="figure_img" id="Figure3"/>
Download
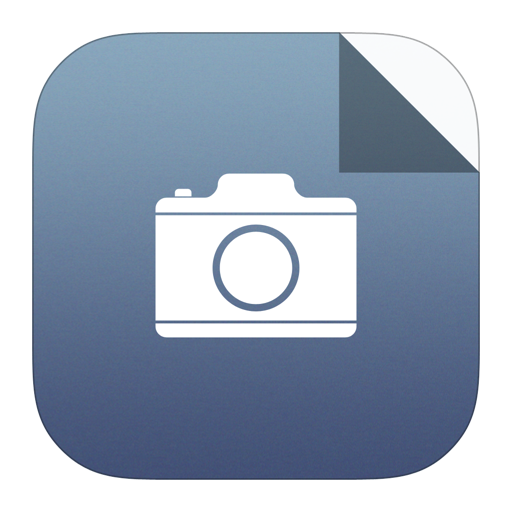
Larger image
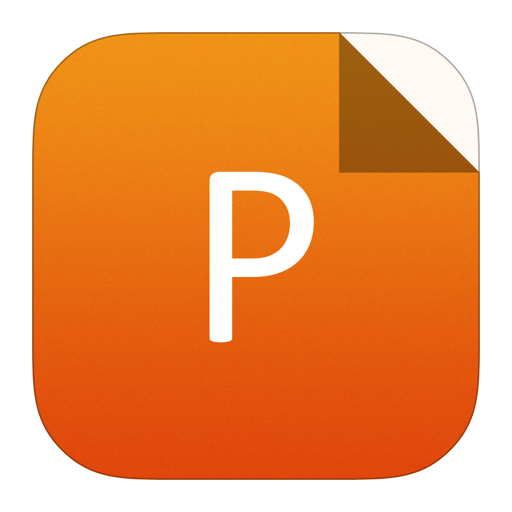
PowerPoint slide
Figure3.
(Color online) Optical microscopic images of the printed Ag lines on PDMS/PET substrate, showing the line width changing with the drop spacing and the time of the plasma treatment.
Fig. 4(a) illustrates the variation of the line width with the drop spacing and the substrate temperature. Fig. 4(b) shows the line width under different drop spacing and treatment times while keeping the temperature constant at 55 °C. Apparently, besides the substrate temperature and the drop spacing, the wettability of the substrate also has a great influence on the width and the morphology of the lines. This is because the formation of the line primarily depends on the interaction between the two adjacent drops. At suitable drop spacing, the two adjacent drops spread (rely on the wettability) to overlap and coalesce, and a series of overlapping drops will finally form lines. Increasing the drop spacing facilitates reducing the line width, which can be adequately explained by Eq. (1) shown as follows:

class="figure_img" id="Figure4"/>
Download
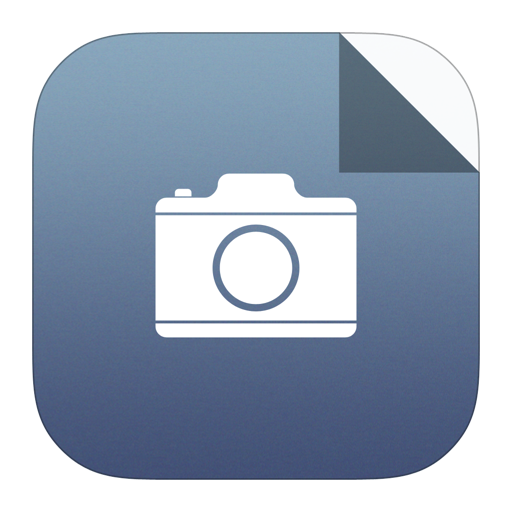
Larger image
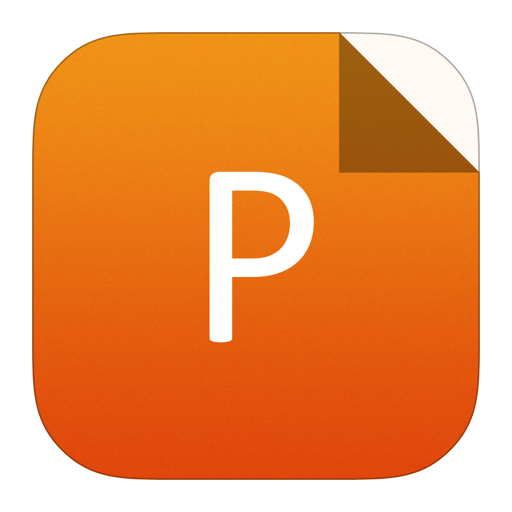
PowerPoint slide
Figure4.
(Color online) The line width changing with various parameters. (a) Line width as a function of substrate temperature and drop spacing. (b) Line width as a function of plasma treatment time and drop spacing.
$$w = sqrt {frac{{2pi {d^3}}}{{3pleft( {frac{theta }{{{{(sin theta )}^2}}}-frac{{cos theta }}{{sin theta }}} ight)}}}, $$ ![]() | (1) |
where w is the line width, d is the droplet diameter, p is the drop spacing and θ is the static advancing contact angle.
When the drop spacing was too small, especially for the substrates with relatively high wettability, such as PDMS/PET substrate with plasma treatment for 10 s, bulging instability and large width were commonly observed in the silver lines, as illustrated in Figs. 3(a) and 3(b). When the drop spacing became larger or the wettability of the substrate got weaker (plasma treatment for only 3 s), stable silver lines with smooth parallel sides and narrower width were obtained, as shown in Figs. 3(c)–3(l). If the drop spacing got too much larger (> 50μm), especially for the substrate treated for 3 s, the overlap would be difficult to occur between the adjacent drops and discrete droplets were observed. Obviously, minimum line width appears at the critical drop spacing that determines the threshold for drop overlap. In this work, the optimal conditions for obtaining silver lines with narrow width and good morphology were achieved with the drop spacing of 50 μm and the substrate temperature of 55 °C while the PDMS/PET substrate was treated with oxygen plasma for 3 s.
2.3
Microscopic morphology of the optimized silver lines
Scanning electron microscopy (SEM) was used to study the microscopic structures of the resultant silver lines. As illustrated in Fig. 5, the morphology of the optimized silver lines was investigated under various magnifications. The silver lines were smooth and well-connected, demonstrating nearly parallel sides, as shown in Figs. 5(a) and 5(b). Figs. 5(c) and 5(d) were the corresponding amplified images, from which compacted and uniformly distributed granular silver nanoparticles with diameters of 50–80 nm were clearly observed. The good morphology was resultant of the optimized process, demonstrating that the methodology developed in this work is feasible for printing high-quality flexible circuits.

class="figure_img" id="Figure5"/>
Download
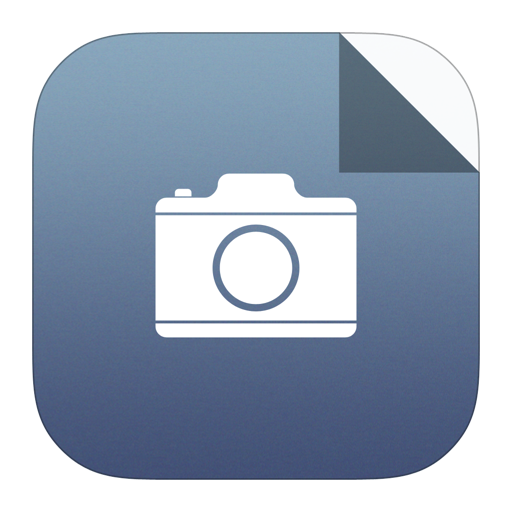
Larger image
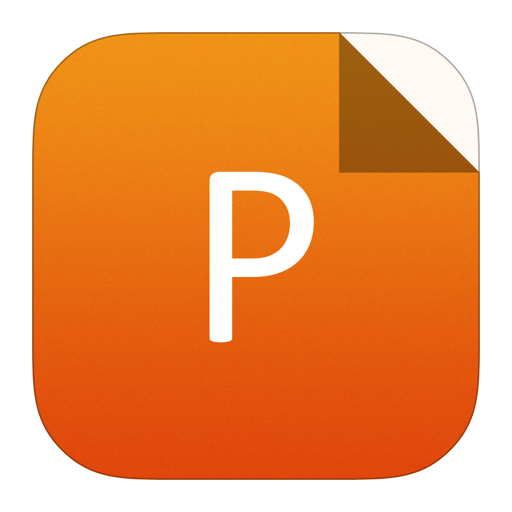
PowerPoint slide
Figure5.
(Color online) SEM images of the optimized silver lines with different magnification.
3.
Inkjet-printed large-area flexible circuits
Large-area flexible circuits with different patterns and high resolution were successfully inkjet-printed on PET substrates (without PDMS modification) using the optimized process, namely keeping the drop spacing and the substrate temperature at 50 μm and 55 °C, respectively. As illustrated in Fig. 6(a), flexible conductors composed of sophisticated silver grids were inkjet-printed. The silver grids were well-connected without obvious fracture and their line width was as low as ~70 μm (inset of Fig. 6(a)), manifesting high resolution. The well-connected and delicate silver grids showed excellent electromechanical stability. It was still capable of powering the light-emitting diodes (LEDs) under bending conditions. Apart from flexible silver grids, highly integrated flexible circuits were also fabricated. The flexible circuits not only exhibited large area (about 19 × 16 cm2) but also showed dense distribution as well as high resolution, as depicted in Fig. 6(b). Additionally, excellent deformability and mechanical flexibility was shown. As illustrated in Fig. 6(c), it could be highly bent with ease. However, without optimizing the drop spacing and the temperature, coalescence of two adjacent lines was generally observed in the printed circuits because of spreading of the silver lines. For instance, as shown in Fig. 6(d), obvious spreading and coalescence of two adjacent lines was observed in the printed circuits when the drop spacing was set at 30 μm and substrate temperature was at room temperature. Normally, the printed circuits were obtained with bad morphology and very poor resolution in most cases without using the optimized process. The simple methodology was also suitable for treating highly hydrophobic PDMS substrate, which is a typical ultra-elastic substrate for being bent, twisted, crumpled and stretched. Highly elastic circuits with exquisite patterns and superb mechanical deformability were also successfully inkjet-printed on the PDMS substrates. The PDMS substrates were pre-treated with plasma for 3 s while fixing the substrate temperature at 55 °C and drop spacing at 50 μm before printing. The PDMS-based circuits showed uniform and smooth morphology, as can be seen in the inset of Fig. 7(a). Figs. 7(a)–7(e) are the photographs of the PDMS-based circuits under various conditions when bent, twisted, rolled, crumpled and stretched respectively, demonstrating their high deformability and excellent mechanical compliance. As shown in Fig. 7(f), the circuits under largely folded conditions could still power the LEDs, verifying their excellent flexibility as well. The superb electromechanical stability of the PDMS-based circuits demonstrates their great potentials for applications in the emerging wearable electronics or bio-inspired devices compatible with the human body.

class="figure_img" id="Figure6"/>
Download
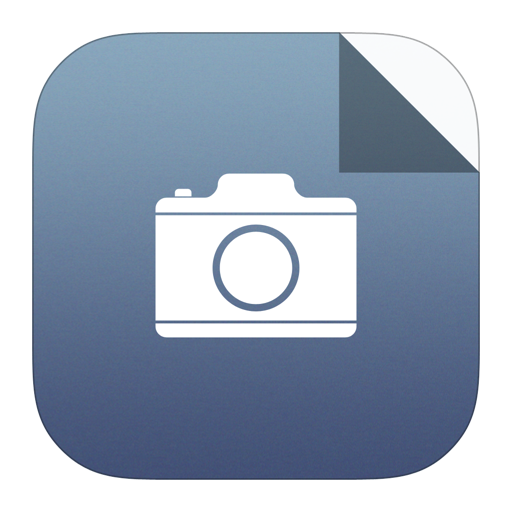
Larger image
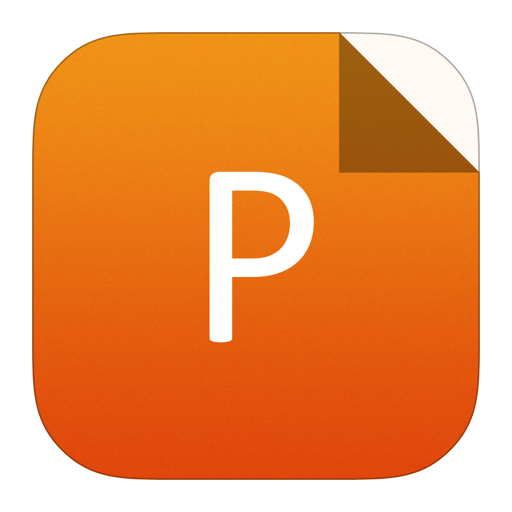
PowerPoint slide
Figure6.
(Color online) (a) Photograph of the inkjet-printed flexible silver grids (using the optimized process) and the optical microscopic image of its microstructure (inset). (b) and (c) Photographs of the large-area flexible inkjet-printed circuits (using the optimized process) before and after bending. (d) Photograph of the inkjet printed circuits without using the optimized process.

class="figure_img" id="Figure7"/>
Download
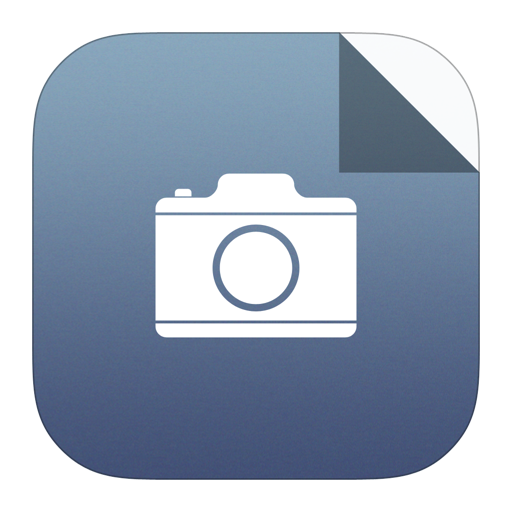
Larger image
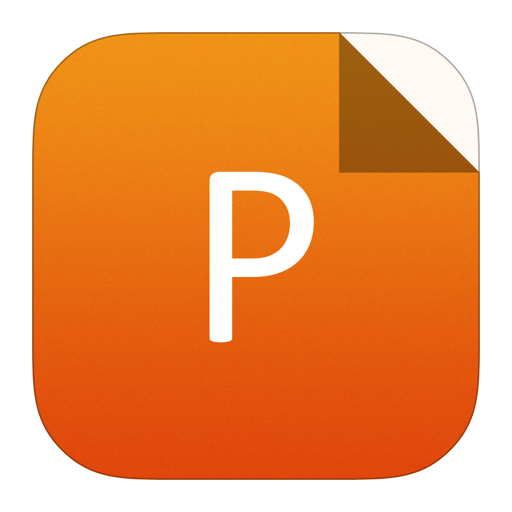
PowerPoint slide
Figure7.
(Color online) PDMS-based circuits under different deformed conditions: (a) bent; (b) twisted; (c) rolled; (d) crumpled; and (e) stretched. (f) The circuits powering the LEDs under folded condition.
4.
Experimental results
To characterize the electrical conductivity of the circuits, two parallel silver lines were inkjet-printed on PET substrates while keeping the drop spacing of 50 μm and the substrate temperature at 55 °C, respectively. The printed silver lines were then annealed at 120 °C for half an hour on a hotplate. The electrical conductivity was measured by four-point probes with the results around 4.5 Ω/□. The superior electrical conductivity mainly resulted from the close compaction of the silver nanoparticles, as confirmed in Fig. 5(d). The electrical conductivity can be further enhanced via increasing the annealing temperature or annealing time. Besides superior electrical conductivity, the printed silver lines also exhibited excellent flexibility. Their sheet resistance only showed a slight increment after being bent to 180° for 1000 cycles, as illustrated in Fig. 8(a). Fig. 8(b) schematically illustrates the flexible silver conductors under bending states. The silver lines could still power the LEDs integrated on them under high bending angle, also demonstrating the superior electromechanical stability (Fig. 8(c)). To investigate the flexibility of the printed silver lines on PET substrates in depth, their morphology was characterized before and after they were bent for 1000 cycles using the optical microscopy self-contained in the printer. Fig. 9 shows the optical microscopic images of the morphology before and after bending. Intriguingly, no obvious change was observed in the morphology. The silver lines still showed a well-connected and uniformly distributed morphology without fracture or buckles, leading to superior electromechanical stability.

class="figure_img" id="Figure8"/>
Download
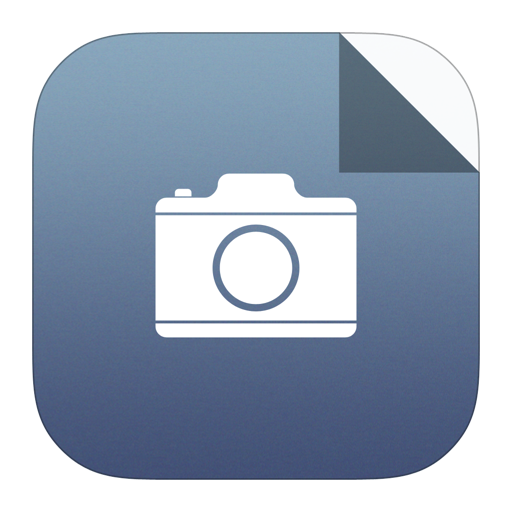
Larger image
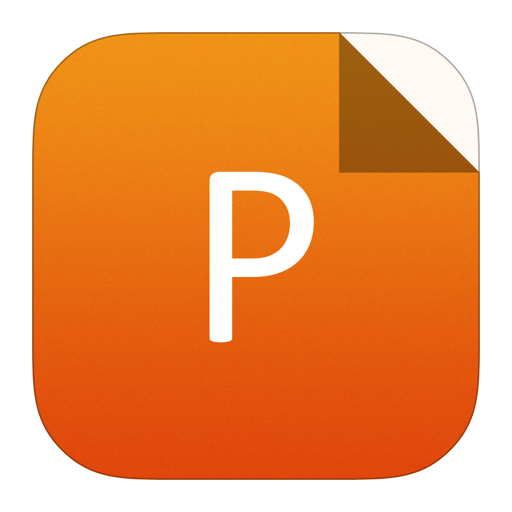
PowerPoint slide
Figure8.
(Color online) (a) Electrical conductivity changing with bending cycles. (b) Schematic illustration of bending test. (c) The silver lines powering the LEDs under bending condition.

class="figure_img" id="Figure9"/>
Download
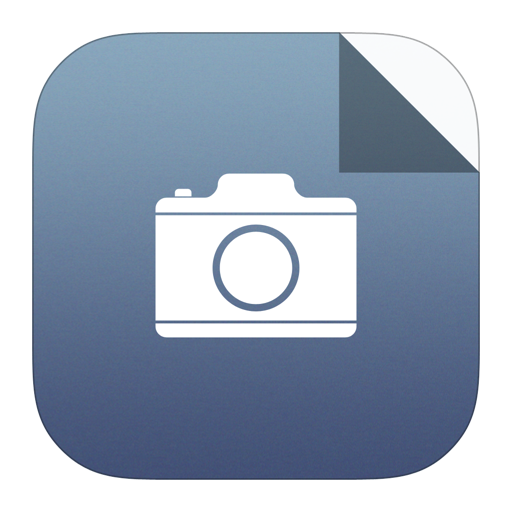
Larger image
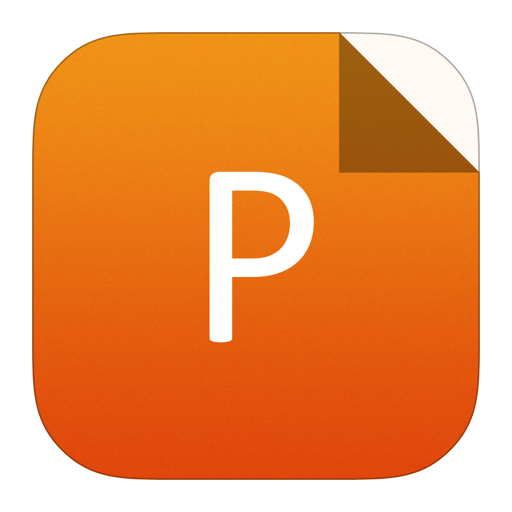
PowerPoint slide
Figure9.
(Color online) The morphology of the printed silver lines (a) before and (b) after bending.
5.
Conclusion
A simple methodology was developed to enhance the resolution of inkjet printing, involving process optimization as well as substrate modification and treatment. The width of the inkjet-printed silver lines was successfully reduced to 1/3 of the original value. By taking advantage of this methodology, the resolution of the printed patterns could be significantly enhanced. Large-area flexible circuits with delicate patterns and good morphology were thus successfully inkjet-printed based on the optimized process. Encouragingly, the resultant flexible circuits also showed excellent electrical conductivity as low as 4.5 Ω/□ and strong tolerance to mechanical bending. The simple methodology developed in this work is not only suitable for treating PET substrates but also other substrates with various wettabilities, which shows great potential as a general strategy to enhance the patterning resolution of inkjet printing, paving the way to the cost-effective large-area mass manufacturing of high-performance flexible electronics.