1.
Introduction
Flexible and stretchable electronics are attracting much more attention due to their excellent conformability to non-planar surfaces and the potential applications in wearable electronics, flexible display and bioengineering[1–4]. Conventional electronic devices based on stiff substrates are unable to fulfill these applications. Stretchable electronics are designed to maintain good performance, reliability, and integration with no mechanical or electrical degradation under various strain conditions: twisting, bending, folding, and even stretching.
A basic building block of stretchable devices is the flexible and stretchable electrode. In the past few years, various strategies applied to the fabrication of stretchable electronics have been used, which include the design of mechanically stretchable structures of metals and the use of networks of one-dimensional nanomaterials. Several groups have reported using metal materials combined with buckled or serpentine structures to achieve stretchability[5–7]. These structures were fabricated using conventional semiconductor processing techniques, such as photolithography, sputtering, evaporation and etching, which are complex and expensive. Others used conductive network structures, such as carbon nanotubes, graphene, silver nanowires or a mixture of them to form stretchable electronics[8–12]. Compared with metal nanostructures, carbon nanomaterials demonstrate some limited applications and performance due to their poor electrical conductivity and instability during the straining process. Among the metal nanostructures, silver nanowires (Ag NWs) have been recognized as a promising candidate for stretchable electronics because they have the highest electrical conductivity among metallic nanowires[13–19], and their one-dimensional wire structure with a superior aspect ratio is better to form electrical percolation networks than particles or flakes, and can maintain good electrical conductivity under deformation, especially when stretched.
Due to the outstanding performance of Ag NWs, a number of interesting applications in stretchable electronics have been demonstrated in recent years, including stretchable organic light emitting diodes (OLED)[20], flexible transparent electrodes[21], stretchable photodetectors[22], ultrasensitive strain sensors[23] and so on. So far, there are usually two methods to form stretchable electrodes by using the combination of Ag NWs and stretchable materials such as polydimethylsiloxane (PDMS) or platinum catalyzed silicone elastomer (EcoFlex). The first method is that Ag NWs networks were deposited or transferred on the top of the pre-strained substrate and released to form stretchable electrodes with buckled Ag NWs films[24]. However, the length of Ag NWs needs to be more than 100 μm in this method, and what is more, the Ag NWs film may generate cracks and be detached from the pre-strained substrate after stretching and releasing repeatedly. In the second method[13], Ag NW networks were buried underneath the elastic polymer surface, forming a conductive and stretchable electrode. Due to the embedded structure, the Ag NWs film was bonded strongly to the elastic substrate. In this method, pattering Ag NWs film is an essential step for fabrication of a stretchable electrode. For patterning techniques, a variety of methods have been developed, such as photolithography with wet chemical etching[25]; laser ablation[26]; stamp transferring[27]; spray-coating with shadow mask[28] and so on. For these top-down and subtractive methods, the post-etching process after film deposition and furthermore solvent contamination restrict their potentials. As we all known, printing is an effective and additive patterning method for many nanomaterials. Therefore, in this work, we used the screen-printing method to pattern Ag NWs film[29], and then casting and curing PDMS to prepare the Ag NWs/PDMS composite electrode.
In this article, we report the use of a printing strategy to prepare flexible and stretchable electrodes of Ag NWs/PDMS composite and analyze the relationship between the surface micro-structure and electrical property during the straining process. We also demonstrate the printed stretchable circuit integrated with commercial ICs (LED arrays, micro control unit, capacitance and resistance) for wearable application.
2.
Experimental section
2.1
Preparation of Ag NWs screen-printing ink
Ag NWs with diameter of 90 nm and length of 30–40μm were used in the present work. In order to avoid the coffee-ring effect during the printing and drying process, a high boiling point solvent should be added to form a mixed solvent. Here, we used terpineol to regulate the solvent volatility, whose boiling point is 220 °C. Careful optimization of the mass ratio of Ag NWs : ethanol : terpineol to 7 : 1 : 2 results in the best printing performance and highest conductivity.
2.2
Fabrication process of stretchable circuit
Fig. 1 illustrates the fabrication process of patterned Ag NWs/PDMS stretchable electrode. Firstly, the prepared Ag NWs paste was screen printed on glass substrate to pattern conductive circuits, and then the printed Ag NWs patterns were dried in a vacuum oven to remove solvent and to form a uniform and conductive film of Ag NWs network. Next, freshly mixed PDMS liquid was casted on top of the printed Ag NWs patterns and to peel off the substrate after curing at 80 °C for 2 h. In this way, the randomly oriented Ag NWs were buried in PDMS to form a conductive and stretchable circuit.

class="figure_img" id="Figure1"/>
Download
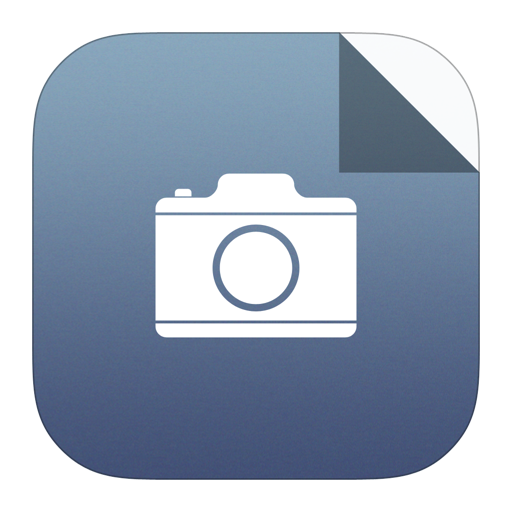
Larger image
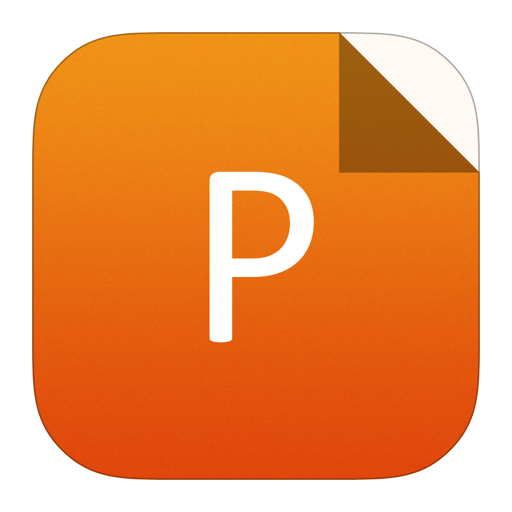
PowerPoint slide
Figure1.
(Color online) Schematic illustration of the fabrication process of patterned stretchable circuit
3.
Results and discussion
Figs. 2(a) and 2(b) are photographs of the printed stretchable Ag NWs/PDMS composite conductors with complex patterns, which have excellent flexibility and stretchability as shown in Fig. 2(c). Optical microscopic images shown in Figs. 2(d)–2(f) further present printed stretchable conductors with various line widths of 300, 500, 800, and 1000 μm, respectively. All the printed lines contain sharp edges, and have uniform and well-defined line widths. The magnified image in Fig. 2(f) shows that the edge of the printed stretchable electrode is fairly smooth, which is essential to the stability of certain electronic devices. In this paper, the effective printed pattern resolution can be achieved as high as 300 μm, which satisfies most stretchable electronic applications. The liquid PDMS penetrates into the interconnected pores of the three-dimensional (3D) Ag NW network, owing to its low viscosity and low surface energy. When the PDMS is cured, it becomes highly cross-linked, all Ag NWs are randomly buried into the PDMS surface without significant voids as shown in Fig. 2(g). Figs. 2(h) and 2(i) demonstrate the cross-sectional SEM images of the stretchable electrode. It can be seen that the first layer is a composite of Ag NWs and PDMS with thickness of about 7 μm, which forms the conductive and stretchable layer, and the second layer is pure cured PDMS, which acts as the stretchable substrate. The magnified SEM further shows the Ag NWs are bonded to the cured PDMS with good adhesion ability.

class="figure_img" id="Figure2"/>
Download
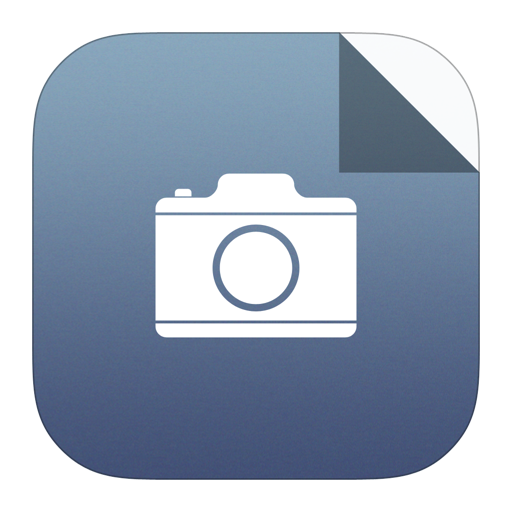
Larger image
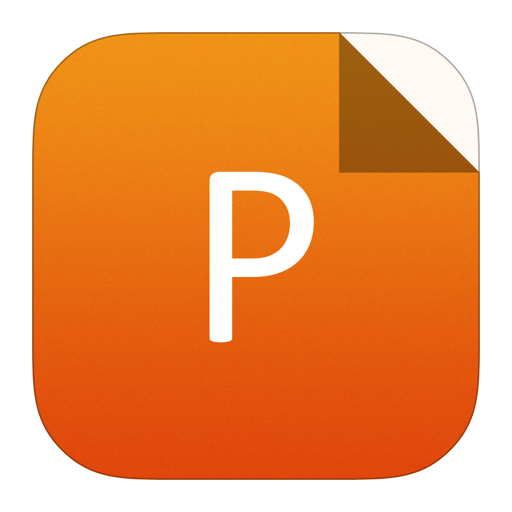
PowerPoint slide
Figure2.
(Color online) (a, b) Photographs of stretchable composite electrodes with different patterns, (c) under tensile state. (d–f) Photograph and optical microscope images of printed stretchable conductor with different line widths. (g) Surface morphology of stretchable electrode. (h) Cross section of Ag NWs/PDMS electrode. (i) Magnified SEM image of the area marked with red dotted line in (h).
The resistance of the printed Ag NWs electrode (L = 3 cm, W = 5 mm) before and after casting PDMS was examined, before casting PDMS the resistance is 0.98 Ω, after curing and peeling off from the glass substrate the resistance is 1.24 Ω, about an increase of 26.5%. Pouring PDMS onto the printed Ag NWs film might lead to filling of liquid PDMS at the Ag NW junctions, which increase the effective resistance of the Ag NW network. However, the Ag NWs/ PDMS composite electrode still has high conductivity of 6912 S/cm before stretching. Next, the stretchable electrode was tested for tensile strain with synchronous resistance data acquisition. Fig. 3(a) shows the resistance of printed stretchable electrode as the function of applied strain. The resistance increased almost linearly from 1.24 to 3.96 Ω when the first tensile strain increased to 100%. Upon releasing the strain, the resistance recovered slowly, and finally decreased to 2.95 Ω when the electrode was fully released. Interestingly when the conductor was stretched back to 100% strain, the resistance remained nearly constant up to about 50%. However, beyond 50% strain, the resistance increased again with strain. More stretching/releasing cycles in the strain range of 0–50% were performed. The resistance showed almost no change in each of the following stretching cycles. A stable resistance was achieved by the four stretching/releasing cycles within the strain range of 0–50% as shown inFig. 3(b).
In order to understand the mechanism of the stable resistance behavior under tensile strain, the surface morphology of Ag NWs/PDMS conductor after the initial 100% stretching/releasing cycle was examined by SEM (Fig. 3(c)). A periodic wrinkle pattern with 12 μm width and 11.6 μm height (Fig. 3(d)) appeared on the surface of the Ag NWs/PDMS layer, in contrast to the flat surface before stretching (Fig. 2(g)). Note that no cracks and debonding were observed on the Ag NWs/PDMS conductive layer and between the conductive layer and PDMS substrate. The buckled wrinkle structure of the Ag NWs/PDMS layer is responsible for the observed stable resistance in the large strain range of 0–50%.
To probe the formation mechanism of the buckling structure during stretching and releasing cycle, the surface morphology of the Ag NWs/PDMS layer was in situ observed during a stretching/releasing cycle via scanning electron microscopy. SEM images corresponding to different applied strain are shown in Fig. 3(e). It can be seen that the surface of the Ag NWs/PDMS layer before stretching is smooth without noticeable folding. When the conductor was stretched, the surface morphology did not show obvious change, and no cracks were observed until the strain of the conductor was up to 100%. When the strain was released, surface buckling structures started to appear in the stretching direction at about 50% strain. More buckles appeared with further release of the strain.

class="figure_img" id="Figure3"/>
Download
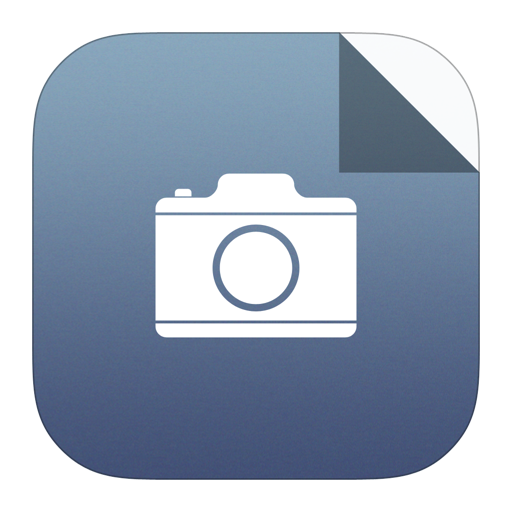
Larger image
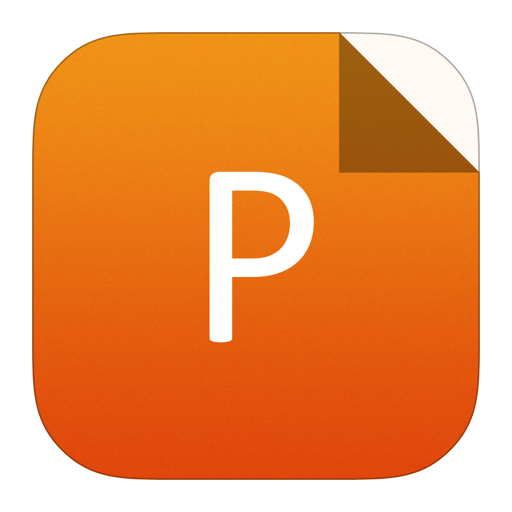
PowerPoint slide
Figure3.
(Color online) (a) Resistance of printed Ag NWs/PDMS composite conductor as a function of the initial 100% tensile strain, inset picture shows the stretchable conductor (L = 3 cm, W = 5 mm) for the tensile test. (b) Resistance of Ag NWs/PDMS stretchable electrode for multiple-cycle tests at 0–50% strain after the initial 100% stretching recovery. (c) SEM image of the stretchable conductor after the initial 100% stretching and releasing cycle. (d) Three-dimensional image of the buckling structure. (e)In situ SEM observation of the surface morphology of a stretchable conductor during the initial 100% stretching and releasing cycle.
The above phenomena can be explained as follows: when the Ag NWs/PDMS layer is firstly stretched, Ag NWs might slide between each other to meet deformation; when the strain is released, the Ag NWs slide back by a certain degree but cannot slide back to their initial state because of the friction force between the NWs and the PDMS; however, the bottom pure PDMS substrate can return back to the initial state, which results in the buckling of the top Ag NWs/ PDMS layer. As a result of the buckling, the resistance of the Ag NWs/PDMS layer does not change during releasing from 50% to 0% strain. The second stretching (up to 50% strain) is essentially flattering of the buckled Ag NWs/PDMS layer, which results in a stable resistance as expected.
Fig. 4 displays the resistance of printed Ag NWs/PDMS composite conductor during 1200 cycles of tensile stretching and releasing between 0 and 50% strain after the initial 100% stretching and releasing cycle. It is obvious that the curve of resistance contains two different stages as functions of stretching and releasing cycles. After the first 100% stretching and releasing, Ag NWs cannot slide back to their initial state because of the friction force between the nanowires and the PDMS matrix. However, during the following repeated stretching/releasing cycles between 0 and 50% strain, some Ag NWs can slide back a bit to overcome the friction force, which causes a slight decrease in resistance (less than 0.5 Ω) both at 0% and 50% strains. After about 30 stretching/releasing cycles, the resistance values increase slightly at 0% as well as 50% strain. After 1200 cycles of deformation, the resistance of Ag NWs/PDMS composite conductor at 0% and 50% strains increase from initial 2.9 to 4.15 Ω and 2.95 to 4.57 Ω. At the 1200 th cycle, the resistance value increased from 4.15 to 4.57 Ω between 0 to 50% strains, only increased by 0.42 Ω, which means the printed stretchable conductor with buckled structures have very stable conductivity after a long time stretching fatigue test.

class="figure_img" id="Figure4"/>
Download
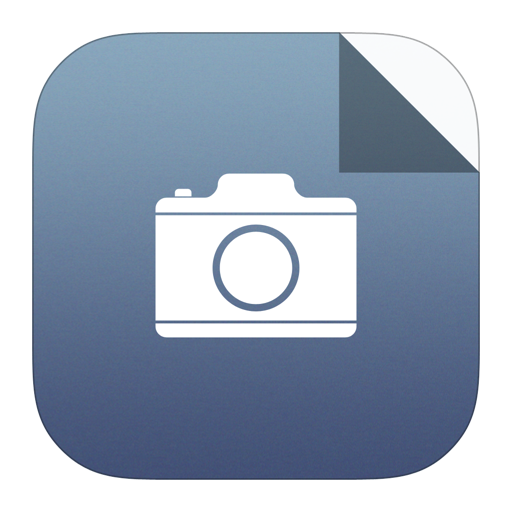
Larger image
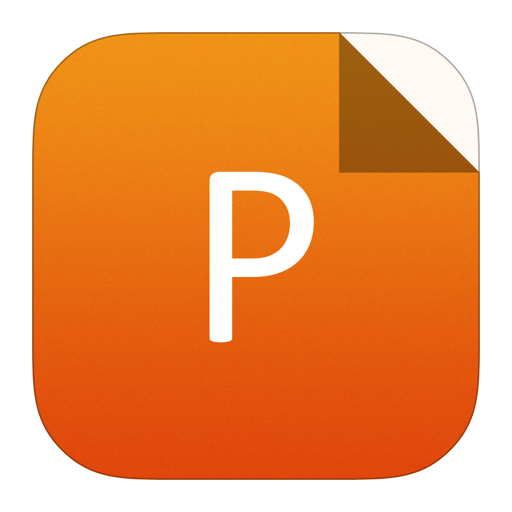
PowerPoint slide
Figure4.
(Color online) Resistance of printed Ag NWs/PDMS composite conductor during 1200 cycles of tensile stretching and releasing between 0 and 50% strain.
In order to realize the application of a printed stretchable circuit, there is a critical issue about the reliable bonding between the soft stretchable electrode and the rigid electronic components such as resistors, capacitors and integrated circuit (IC) chips. In the present work, a solution was developed using a PDMS of a different modulus[30] to encapsulate rigid electronic components, as illustrated in Fig. 5(a). Here, the integrated design is akin to the stretchable devices reported by Rogers et al.[5], using a hybrid rigid island-stretchable interconnect approach. The stiff PDMS with a relatively high concentration (16.7%) of curing agent covers the area of rigid components, and the soft PDMS with less (6.3%) curing agent covers the other area of stretchable interconnect wiring. After encapsulating with PDMS, the stretchable LED circuit (Fig. 5(b)) can work normally under tensile strain; as shown in Fig. 5(c), the LED remains lit as the circuit goes under a 100% tensile strain state. Using this encapsulation method, some wearable applications of the printed stretchable circuit integrated with commercial ICs were demonstrated, such as a stretchable lighting chain wrapping around fingers (Fig. 5(d)), and washable lighting fabric with high mechanical strength (Fig. 5(e)). Fig. 5(f) shows a simple functional circuit with an MCU chip, capacitors, resistors and an LED, which can control the LED to flash at the designed frequency. The circuit shows good flexibility and works normally even under twisting and bending conductions.

class="figure_img" id="Figure5"/>
Download
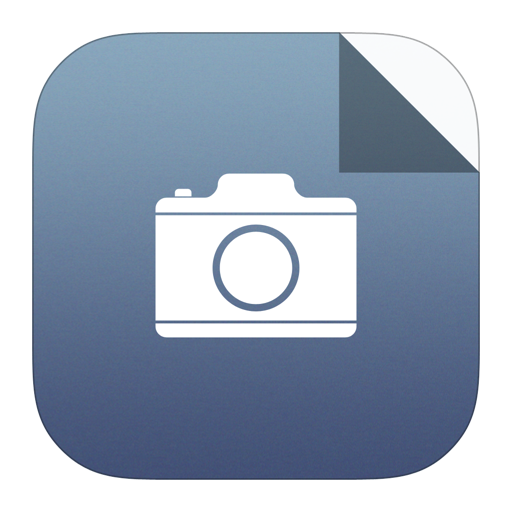
Larger image
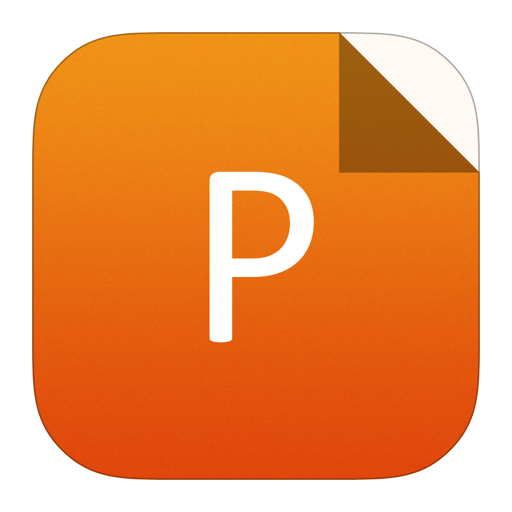
PowerPoint slide
Figure5.
(Color online) (a) Schematic diagram of the stretchable LED circuit encapsulated with PDMS. (b) Photograph of the encapsulated stretchable single LED circuit. (c) Pictures of lighting the LED circuit under tensile strain state. (d) Stretchable lighting hand chain. (e) Washable lighting smart fabric. (f) Simple functional circuit integrated with microcontroller unit (MCU), capacitance, resistance and LED.
4.
Conclusion
Flexible and stretchable electrodes have been fabricated by the printing method based on Ag NWs/PDMS composite. Due to the buckling of the Ag NWs /PDMS layer after the initial stretching/releasing cycle, a stable conductivity was achieved in a large range of tensile strain (0–50%). Furthermore, some wearable applications were demonstrated with the printed stretchable circuit integrating rigid electronic components. It has been proved that printing enables the manufacture of fully functional stretchable circuits and is much easier to implement than other approaches to making stretchable electronics.