全文HTML
--> --> -->激光尾场电子加速器产生的高品质电子束可以进一步产生高质量的辐射源. 2001年, Catravas等[11]提出了全光汤姆逊散射光源的设想, 使用激光尾场加速产生高能电子, 再用激光与尾场电子相作用产生X射线脉冲. 全光汤姆逊散射光源有希望获得脉宽短至10 fs, 源尺寸小于5 μm、发射角mrad量级、单色性很好的高品质台面硬X射线和伽马射线源. 目前, 实验建立的全光汤姆逊散射光源主要有两种类型: 一种采用两束激光对打[12-16], 对激光和电子的时空同步要求极高, 但能够用于喷嘴、气室、毛细管等多种尾场电子加速器上; 另一种使用等离子体镜反射主激光, 使其与尾场电子相作用[17-19], 激光与电子的时空同步难度低, 但需要主激光被良好地自聚焦且能量不能损耗过大, 只能用于较短的喷气靶尾场电子加速器.
稳定的高品质单能全光汤姆逊散射光源的核心需求是稳定的高品质单能尾场电子, 这也是激光尾场电子加速技术本身发展的需求. 为了获得稳定的高品质单能尾场电子, 国内外的研究者开展了一系列针对尾场电子注入机制的研究. 早期研究中, 波破导致的电子注入被称为自注入[20-26], 其发生时间、位置以及注入的电量严重依赖于激光在等离子体内部的非线性传输及自聚焦过程, 因此具有很强的随机性, 对初始参数也非常敏感, 每发打靶电子的注入位置都会改变, 使得最终获得的尾场电子发与发之间的重复性很差. 为了进一步提高尾场电子的稳定和重复性, 电子注入技术得到了深入的研究, 如离化注入[7,27-30]、碰撞注入[31-33]、密度梯度注入[34-37]、冲击波前沿注入[38-43]等. 离化注入[27]是在低Z气体里混入少量高Z气体, 例如在He气里混入少量N2气, 激光功率密度较高的部分可以将N原子内层的电子电离并直接注入进尾场加速相从而得到加速. 激光在混合气体中传输时, 离化注入通常发生在整个等离子体通道中, 由此产生的尾场电子电量较大, 但能谱为连续谱. 碰撞注入[31-33]是使用第二束激光与主激光碰撞形成驻波, 在驻波波峰所在区域激光强度大大提高, 使得电子只在驻波波峰附近注入, 该方法也可以获得稳定的单能尾场电子, 但需要实现两束激光高精度时空同步, 实现的技术难度很高. 密度梯度注入[34-37]通过使用具有连续变化的密度梯度的气体靶, 变化的等离子体密度可以控制尾场加速结构的尺度, 从而实现电子的注入和持续加速. 密度梯度注入也是一个持续的过程, 导致电子能谱通常也为连续谱. 冲击波前沿注入(shock wave front injection)[38-43]可以看作一种发生在极陡峭的密度梯度处的电子注入. 其原理是在超音速喷嘴上附加刀片, 超音速气流接触刀片时, 在刀边边缘被减速, 产生一个尺度极小但密度变化非常陡峭的高密度区域. 激光在这个区域的密度下降沿处形成急速膨胀的尾场结构, 尾场相速度降低, 使原本不能被注入进尾场加速区的电子包含进加速区, 从而在一个尺度极小且位置受控的区域内实现电子的注入. 在冲击波前沿注入的电子的初始状态以及后续的加速距离都一致, 因此可以大大提高尾场电子的单能性和稳定性.
因为尾场电子的注入和加速需要不同的等离子体密度条件, 一个很自然的想法就是将电子的注入和加速分开, 分别使用不同的气体密度来满足两个过程不同的需求. 小尺度的高密度注入级可以产生能量较低的单能电子作为种子束. 大尺度的低密度加速级无法注入电子但是可以通过较长距离的加速将种子束加速至高能量, 这就是所谓的级联加速[44,45]. 级联电子加速技术既可以实现对电子注入和加速过程的控制, 同时也可以为之后开展多路级联研究奠定基础, 有希望通过多次尾场加速将电子加速至TeV量级[46,47]. 在多路级联电子加速技术中, 小能量高重频激光器产生的尾场电子可以作为种子源, 在后续的级联加速过程中得到进一步的加速. 种子源的单能性和稳定性对后续的电子加速过程以及最终获得的电子束参数有重要影响, 因此有必要探索小能量高重频激光条件下降低尾场电子绝对能散并提高电子束参数稳定性的途径.
项目组在之前的研究中已经在激光聚变研究中心的SILEX-I装置上成功获得了58 MeV的单能尾场电子[48], 但尾场电子的重复性仍然不够好. 基于新建的45 TW重频激光器, 通过对现有的电子注入技术以及电子加速技术的调研, 项目组确定了技术路线: 在现有的超音速喷嘴尾场电子加速装置上引入冲击前沿注入技术、离化注入技术以及级联电子加速技术, 来产生全光汤姆逊散射光源所需的可以稳定重复运行的单能电子束. 为了获得更加稳定且重复性好的尾场电子, Thaury等[49]和Golovin等[50]的研究表明, 结合多种控制注入技术以及级联加速技术的混合加速技术可以有效克服单个技术的极限, 获得质量和稳定性更好的单能尾场电子. 目前混合加速相关的研究成果较少, 还有大量的物理细节需要探索. 因此我们开展了这方面的研究, 通过对喷嘴的设计, 使用单个喷嘴实现了注入和加速的分离, 然后在注入级引入冲击前沿结合离化注入的方式, 获得了稳定产生的尾场电子.
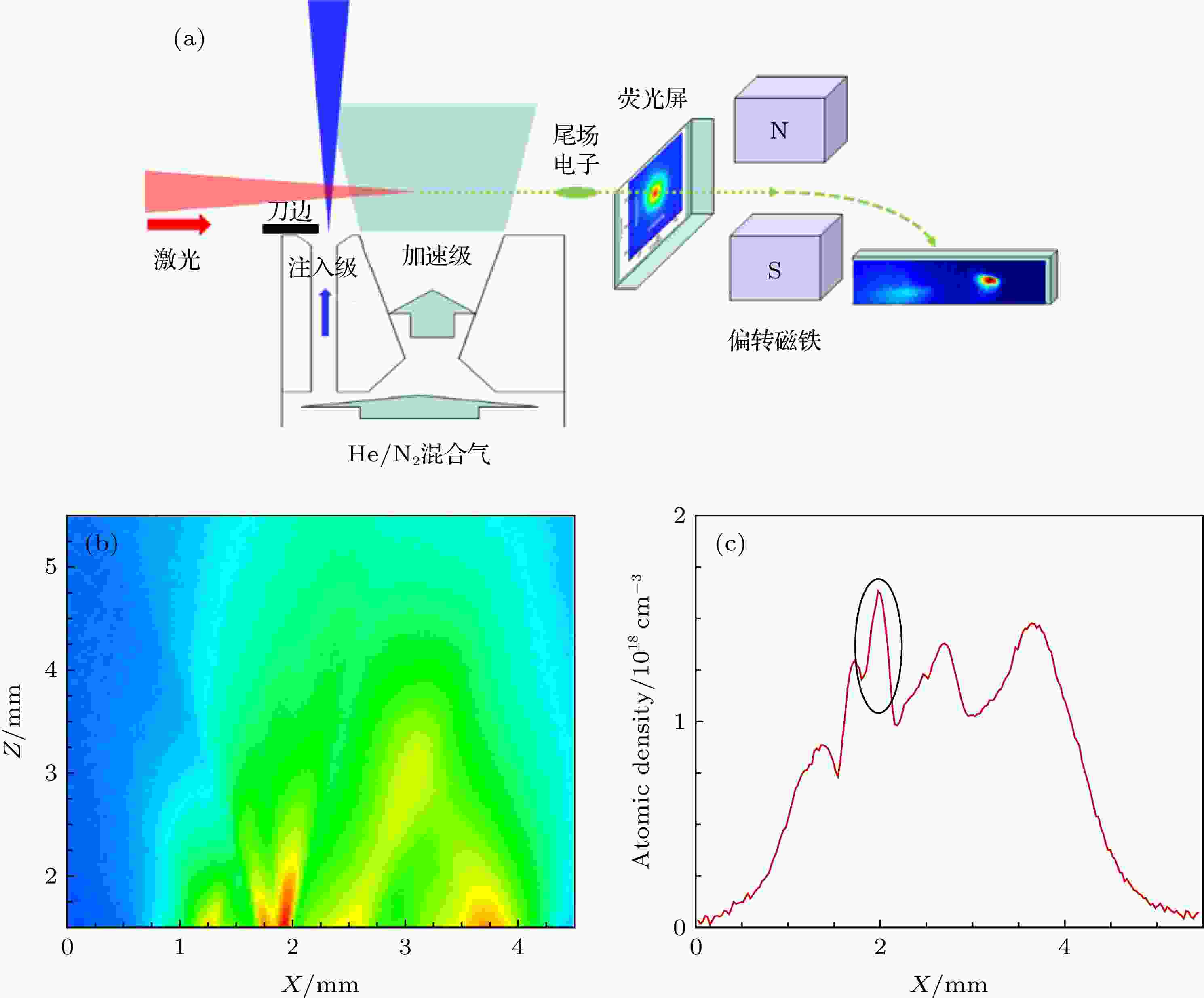
Figure1. (a) The gas jet designment and the experimental layout; (b) the side view of the gas density distribution; (c) the one dimensional electron density at a height of 2 mm from the gas jet.
实验中为了保护激光器, 打靶功率限制为30 TW, 到靶能量为700 mJ, 脉宽25 fs, 激光波长为800 nm, 激光聚焦OAP的焦距为420 mm, 聚焦焦斑为10 μm(半高全宽, 40%能量集中度), 激光的归一化强度a0 = 2.9. 激光打靶高度距离喷嘴上沿2 mm, 激光聚焦位置为加速级中心. 为了测量喷嘴附近的气体密度分布, 使用探针激光对气体进行阴影照相, 同时在0—180°范围内旋转喷嘴, 每隔3°测量一次气体密度分布, 最后使用三维CT重建算法计算得到喷嘴附近气体密度的三维分布. 喷气气压为650 kPa且喷嘴附加刀边时, 其附近的气体密度分布如图1(b)所示, 喷嘴轴线上距离喷嘴2 mm处气体密度的一维分布如图1(c). 可以看到, 刀边的引入在喷嘴的两个出口之间2 mm位置处产生了一个高密度气柱, 其后沿存在一个极陡的气体密度下降沿.
通过比较不同的实验条件下电子束斑随喷气气压的变化, 可以研究冲击波前沿注入、离化注入、级联加速等技术的有效性. 实验中涉及四种实验条件: I)使用刀边堵住注入级, 形成只有单加速级的喷嘴, 工作气体使用纯He气; II)喷嘴包含注入级和加速级, 不附加刀边, 工作气体为He(97.5%)/N2(2.5%)混合气; III)喷嘴包含注入级、加速级和刀边, 工作气体为纯He气; IV) 喷嘴包含注入级、加速级和刀边, 工作气体为He(97.5%)/N2(2.5%)混合气.
图2(a)—图2(e)为条件I)下所获得的电子束斑, 可以观察到, 对于纯He气、单加速级情况, 喷气气压低于2200 kPa时完全没有电子产生. 只有当喷气气压提升至2200 kPa时, 才能产生尾场电子, 但束斑位置非常不稳定. 在更高的气压下(2600 kPa)会出现多次注入导致的多个电子束斑. 进一步的测量显示, 电子能谱的稳定性很差, 且全为连续谱, 说明电子主要来自于自注入机制, 并且在激光与靶相互作用的全过程中都会发生.
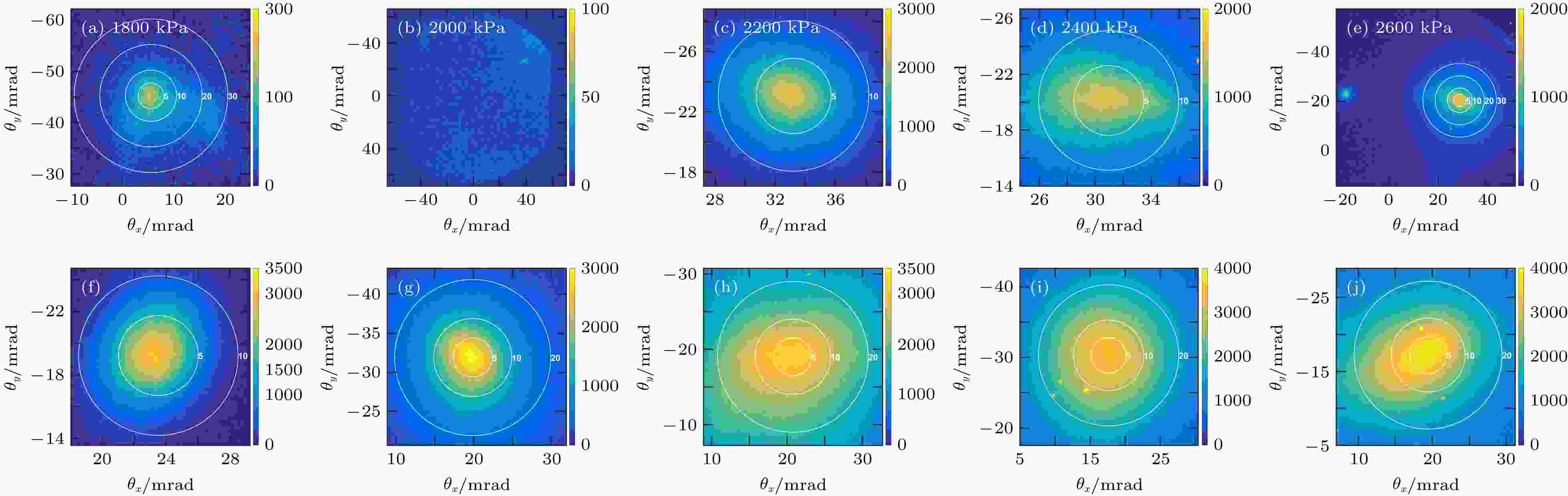
Figure2. Electron angular distribution for ((a)?(e)) single stage gas jet and ((f)?(j)) dual-stage gas jet.
去掉刀边, 同时将工作气体更换为混合气体, 条件II)下所获得的电子束斑如图2(f)—图2(j)所示. 与条件I)下获得的结果相比, 注入级以及混合气体的使用使得产生尾场电子的下限气压明显降低至1800 kPa, 且电子束斑的位置变稳定. 进一步对电子能谱的测量显示, 电子能谱仍然为连续谱, 但稳定性变好, 说明混合气体的引入使得电子注入的物理机制变为离化注入, 提高了电子注入的稳定性. 但在不附加刀边的情况下, 注入级与加速级的等离子体密度差不够高, 不能稳定地将电子注入位置限制于注入级, 在激光与靶相互作用的全过程中都会发生离化注入.
通过在注入级附加刀边, 可以在注入级中产生冲击波前沿, 比较工作气体分别为纯He气以及He/N2混合气时的电子束斑, 如图3(a)—图3(e)以及图3(f)—图3(j), 分别对应实验条件III)和IV). 图3(a)—图3(e)显示, 工作气体为纯He气时, 在1800 kPa才开始有电子注入. 提高喷气气压时, 角分布光斑的位置波动极大, 并且高于2400 kPa时会有多光斑出现. 进一步的电子能谱测量结果也显示, 在下限气压(1800 kPa)时, 仍然无法产生稳定的单能电子. 通过比较图2(f)—图2(j)以及图3(a)—图3(e)的结果可以发现, 对于离化注入(混合气)、冲击波前沿注入(附加刀边)、级联加速(双喷嘴)这三种条件, 实验中只采用其中两种时, 发生电子注入所需的气压皆高于1800 kPa, 并且皆不能将电子注入稳定地约束在注入级内. 而同时应用上述三种条件, 即实验条件IV), 不同喷气气压下的电子束斑如图3(f)—图3(j)所示. 可以看到, 在喷气气压500 kPa时就开始有电子注入, 且电子的出射方向基本稳定. 对电子能谱进行测量, 发现有部分发次出现单能电子. 由此可见, 混合气体中的离化注入可以显著降低电子注入发生的气体密度, 结合冲击波前沿注入, 可以有效地将离化注入约束到冲击波前沿处. 这些注入的电子可以在加速级中得到进一步加速, 有希望获得稳定的单能电子.
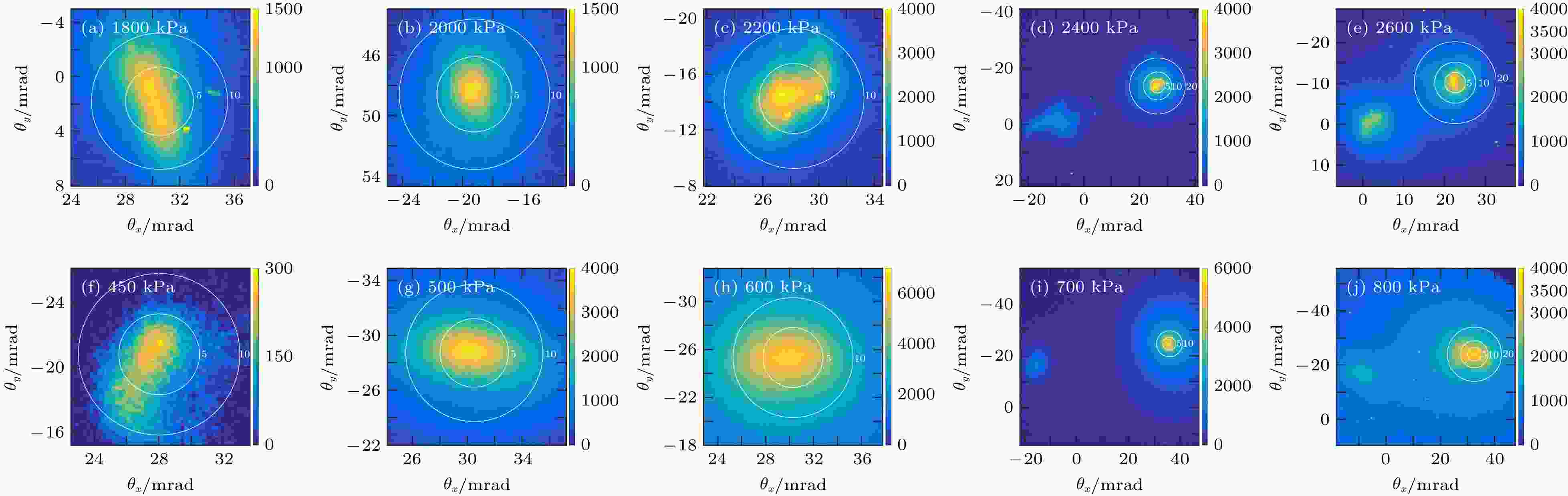
Figure3. The electron angular distribution for (a)?(e) pure He and (f)?(j) the mixed gas of He with 2.5% N2.
基于图3(f)—图3(j)的结果, 在条件IV)时, 在喷气气压600 kPa附近精细地调节喷气气压, 最后发现喷气气压为650 kPa时电子束斑大小和指向的稳定性最佳, 因此在该密度下连打6发, 测试其多发打靶条件下的稳定性, 打靶结果见表1.
发次号 | θx/mrad | θy/mrad | σx/mrad | σy/mrad |
558 | –25.5 | 28.9 | 8.5 | 5.6 |
559 | –22.1 | 27.3 | 5.0 | 5.6 |
560 | –23.5 | 23.3 | 6.2 | 4.8 |
561 | –19.0 | 23.3 | 6.5 | 5.1 |
562 | –18.1 | 24.2 | 3.8 | 3.6 |
563 | –19.7 | 19.4 | 6.4 | 4.4 |
表1喷气气压650 kPa时连续打靶6发得到的电子束斑参数, θx,, θy为出射方向, σx, σy为角分布光斑的半高全宽直径
Table1.The emitting direction θx, θy and the FWHM angular spread σx, σy of the electron angular distribution for continuous 6 shots under jet pressure of 650 kPa.
从表1可以看到, 连续打靶六发, 电子出射角度的平均值为(–21.3 ± 2.6, 24.4 ± 3.06) mrad, 角分布光斑的半高全宽(FWHM)为(6.07 ± 1.4, 4.85 ± 0.7) mrad. 光斑最小的发次为562发, 其放大的角分布如图4, 角分布光斑在x和y方向的半高全宽分别为3.8和3.6 mrad. 对电子束斑的测量结果显示, 基于级联加速、冲击前沿注入以及离化注入技术, 尾场电子出射方向的稳定性得到了提升, 发散角也得到了控制.
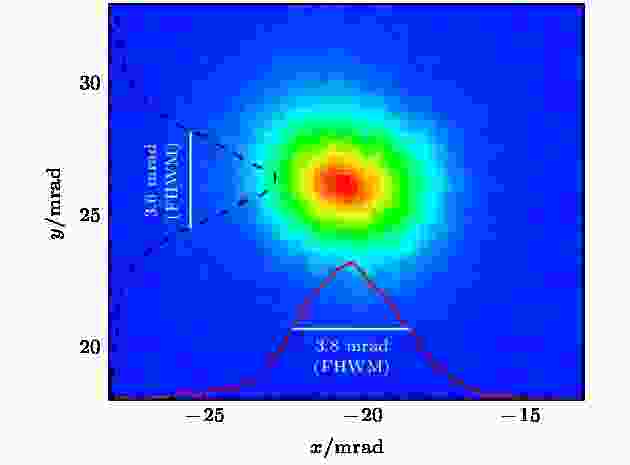
Figure4. The spot size for shot 0562 when the jet pressure is 650 kPa.
接下来使用磁场强度为8000 Gs (1 Gs = 10–4 T)的磁谱仪对电子能谱进行测量, 连续打靶5发, 测量得到的电子能谱结果如图5, 其具体参数如表2所列, 其中电子束电量由经标定后的荧光成像系统给出. 由能谱测量结果可以看到, 连续打靶时, 可以基本保持电子的单能性, 且电子能谱基本没有低能尾部, 呈现干净的单能峰结构. 多发打靶时电子中心能量会有抖动, 抖动范围为58.2—71.0 MeV, 平均能量为(63.24 ± 6.12) MeV. 电量的抖动为2.0—9.4 pC, 平均值为(5.99 ± 3.10) pC, 电子绝对能散(FWHM)的抖动为6.9—17.0 MeV, 平均值为(13.0 ± 3.9) MeV.
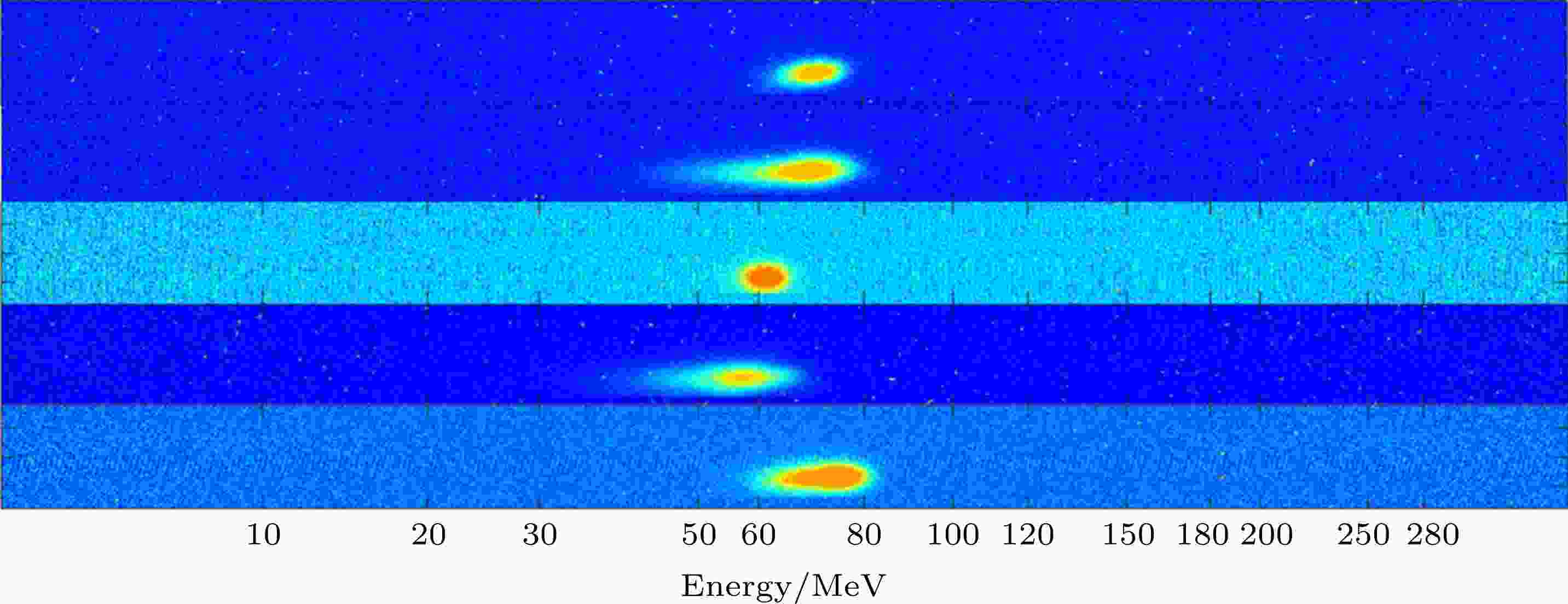
Figure5. Electron energy spectra for continuous 5 shots under jet pressure of 650 kPa.
发次号 | 中心能量/MeV | 电量/pC | 能散FWHM/MeV |
570 | 66.7 | 6.5 | 10 |
571 | 66.1 | 9.4 | 17 |
572 | 58.2 | 2.0 | 6.9 |
573 | 54.2 | 9.2 | 14.3 |
574 | 71 | 2.86 | 16.6 |
表2喷气气压650 kPa时连续打靶5发得到的电子能谱参数
Table2.The central energy, charge and energy spread of the electrons for continuous 5 shots when the jet pressure is 650 kPa.
通过研究离化注入、冲击波前沿注入、级联加速这三种技术对电子束斑的影响, 发现可以将三种技术结合, 降低电子注入的喷气气压下限, 将电子的注入约束于注入级中的冲击波前沿处, 从而获得了可以稳定产生的单能尾场电子. 尾场电子出射角度的平均值为(–21.3 ± 2.6, 24.4 ± 3.06) mrad, 角分布光斑的半高全宽(FWHM)为(6.07 ± 1.4, 4.85 ± 0.7) mrad. 尾场电子中心能量的平均值为(63.24 ± 6.12) MeV, 能散为(13.0 ± 3.9) MeV. 在下一步的工作中, 通过减小激光入射方向和输出能量的抖动, 有希望进一步提升尾场电子的稳定性. 目前获得的尾场电子中心能量约60 MeV, 这主要由于喷气气压较低, 限制了尾场加速梯度以及最终获得的尾场电子能量. 如何使用更高密度的喷嘴产生更高能量的尾场电子, 同时保持尾场电子的单能性和稳定性, 是我们下一步的研究内容.
使用粒子模拟(PIC)程序对图1(c)所示气体密度分布下的尾场加速过程进行了研究. 取高斯脉冲激光强度a0 = 1.5、脉宽25 fs、焦斑5 μm、波长800 nm. 为了与喷气气压650 kPa下混合气体被完全电离时的电子密度相对应, 取峰值处电子密度1.9 × 1018/cm3. 模拟得到的冲击波前沿附近等离子体中电子的纵向相空间分布(x-γVx)如图6所示, 在冲击波前沿所在2.05—2.15 mm处, 电子可以有效地被注入并被进一步加速. 进一步的模拟研究显示, 降低电子密度至1.6 × 1018/cm3时(对应喷气气压547 kPa), 全过程不再有电子被注入及加速. 这与实验结果图3(f)—图3(j)基本符合, 当喷气气压稍微降低以后不再有尾场电子产生.
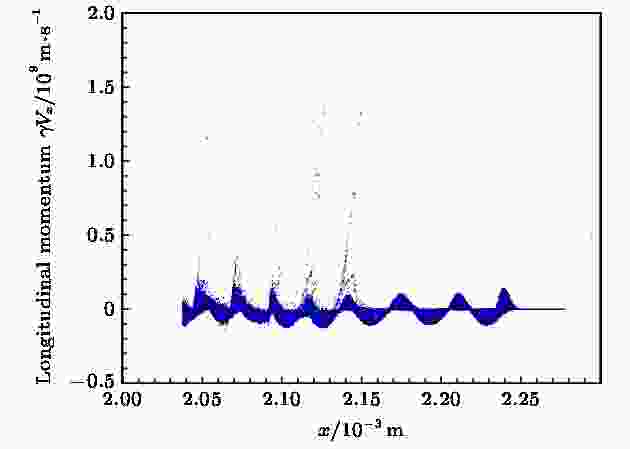
Figure6. The Distribution of electrons in longitudinal phase space (x-γVx).
在当前的研究中, 产生的尾场电子的中心能量较低(约60 MeV), 且多发打靶时中心能量有一定的抖动. 在下一步的研究工作中, 计划通过使用更高密度的喷嘴, 并通过对喷嘴气体密度分布进行设计使尾场电子的注入位置不变, 有希望产生更高中心能量的单能尾场电子. 同时通过对激光器的改造, 将激光器输出能量的波动控制在5%以下, 有希望进一步提高尾场电子中心能量的稳定性. 稳定获得单能尾场电子以后, 计划开展全光汤姆逊散射光源的研究, 并诊断光源的光子能量、发射角、源尺寸等参数.