1.
Introduction
Since it was originally discovered in the 1970s[1], surface-enhanced Raman spectroscopy (SERS), a technique with an enormous enhancement factor of 108 or even higher in Raman signals after adsorbing molecules on certain surfaces, has been widely studied[2], and increasingly used to develop highly sensitive molecular analytical tools[3]. It is now widely accepted that Raman enhancement phenomena are attributed to two mechanisms: the electromagnetic mechanisms (EMs), and chemical mechanisms (CMs). With regard to EMs, Raman enhancement mainly originates from the local field enhancement around the surfaces of rough or nanostructured noble metals (e.g., gold and silver), induced by the surface plasmon effect[4]. CMs usually occur due to the charge transfer effect between molecules and substrates[5]. In comparison with EMs, enhancement factors in CMs are typically moderate and material-dependent[6]. Due to a significant enhancement in Raman signals, SERS chips have enabled significant developments in chemical and biological sensing applications for trace gas monitoring[7], single-molecule analysis[8], disease diagnosis[9-11], and pesticide residue detection[12, 13].
Two-dimensional (2D) materials provide us with emerging opportunities in SERS, since the discovery of graphene in 2004[14]. By virtue of advantages such as outstanding fluorescence quenching capability, excellent biomolecular compatibility, tunable Fermi levels, and potentially low-cost material preparation, 2D materials could potentially be considered as ideal metal-free SERS materials. However, such materials enhance Raman signals via CMs, and usually suffer from limited Raman enhancement factors (EFs) (typically below 103), as compared with metallic materials[15]. In recent studies, researchers have found that this problem may be solved by utilizing 2D heterostructure materials[16] or by doping 2D materials via chemical methods[17]. Consequently, 2D-material SERS chips have attracted significant research attention in the past few years. Having first explored graphene-enhanced Raman spectroscopy (GERS), researchers have devoted much attention to studies of SERS based on other 2D materials and their hybrids, such as transition metal dichalcogenides (TMDs), black phosphorus (BP), hexagonal boron nitride (h-BN), and 2D titanium carbide or nitride (MXenes). By integrating these materials on silicon-based chips, SERS detection devices could be made widely accessible, meeting the requirements of diverse analytic scenarios.
In this review, we comprehensively summarize novel SERS techniques based on metal-free 2D materials beyond graphene, including TMDs, BP, h-BN, and MXenes. Firstly, we briefly introduce 2D materials’ physicochemical properties, before categorizing the cutting-edge progress of SERS studies based on these substrates. Due to this paper’s length limit, SERS studies of metal-2D-material hybrid substrates are not included here. Finally, we summarize the review, and discuss prospects in this area. We hope this review will serve as a useful reference for researchers in the fields of 2D materials, spectroscopy, and their applications in chemical and biological sensing.
2.
Mechanisms of SERS
It is generally believed that the Raman enhancement in SERS originates primarily from two mechanisms: EMs and CMs. With respect to EMs, the Raman enhancement usually occurs around surface locations in noble metal nanoparticles, known as “hot spots”[18]. When the incident light is in resonance with the nanoparticle’s localized surface plasmon resonance (LSPR) frequency, the incident light excites electrons on the metal nanoparticle’s surface, leading to a polarization of charge and oscillating dipoles, as shown in Fig. 1(a). Since the frequencies of Raman scattered Stokes (or anti-Stokes) fields are typically close to that of the incident light, the resonance results in an intensity enhancement of both incident light and Raman scattering light. Consequently, overall enhanced Raman signal intensity (
m{SERS}} $


class="figure_img" id="Figure1"/>
Download
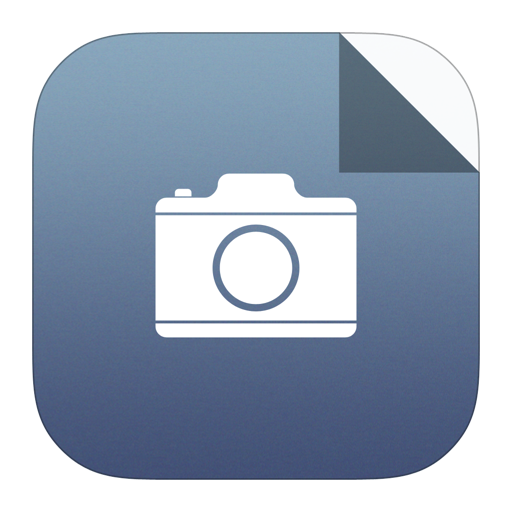
Larger image
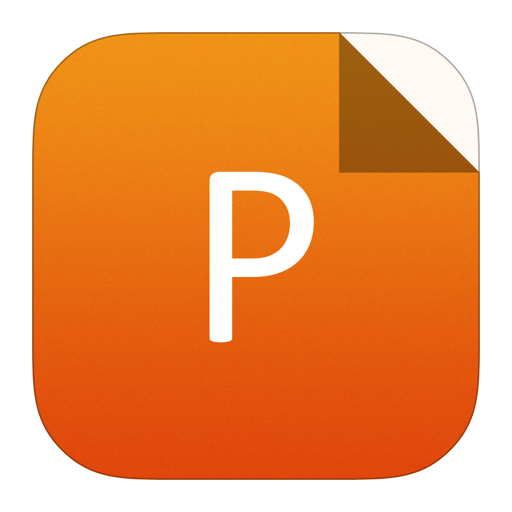
PowerPoint slide
Figure1.
(Color online) Schematics of the mechanisms of SERS. (a) With respect to EMs, when the incident laser is in resonance with the nanoparticle LSPR frequency, the incident laser excites electrons on the metal surface, leading to a polarization of charge and oscillating dipoles. As the frequencies of Raman scattering light are close to that of the incident laser, the resonance also increases the intensity of the Raman scattering light. (b) For CMs, electrons are transferred from the Fermi level of the substrate to the LUMO of the molecule, thereby forming a charge transfer intermediate. The energy transition (
m{CT}} $
m{mol}} $
$$ {I}_{ m{SERS}}={left|{E}_{ m{in}} ight|}^{2}{left|{E}_{ m{s}} ight|}^{2}approx {left|{E}_{ m{in}} ight|}^{4}, $$ ![]() | (1) |
where
m{in}} $

m{s}} $

On the other hand, CMs are often attributed to the charge transfer effect, which is not yet clearly understood[24-26]. Compared with EMs, CMs originate from short-range effects arising from the strong electronic coupling interaction between substrates and molecules, as shown in Fig. 1(b). Taking graphene-based SERS as an example[25], this interaction transfers electrons from the Fermi level of the substrate to the lowest unoccupied molecular orbital (LOMO) in a molecule, thereby forming a charge transfer intermediate, whose Raman scattering cross-section is much higher than that of the unabsorbed molecule, which is similar to the resonant Raman scattering[26]. Moreover, the charge-transfer resonance between 2D semiconductor materials and molecules, associated with the photon-induced charge transfer from semiconductor band edges to the affinity levels of molecules, is considered to contribute to changes in molecular polarizability in relation to SERS[27]. In practical metallic SERS applications, both EMs and CMs usually co-occur on metal surfaces, while chemical enhancement factors are usually moderate (typically below 103) compared with those of EMs[28], but do not suffer from the disadvantage of photothermal heat generation. It is worthwhile to note that some studies show that chemical enhancement factors could be significantly improved using dielectrics[29], semiconductors[30], or 2D materials[31], offering us an unprecedented opportunity to develop and revolutionize plasmon-free SESR substrates.
3.
SERS based on 2D materials beyond graphene
In this section, we summarize SERS advances based on diverse 2D materials beyond graphene, as shown in Fig. 2. The section is divided into five parts, covering the development of SERS chips based on TMDs, h-BNs, BP, MXenes, and their heterostructures, respectively. In each part, we first briefly introduce the physiochemical properties of 2D materials for use in developing SERS, then discuss state-of-the-art experimental results.

class="figure_img" id="Figure2"/>
Download
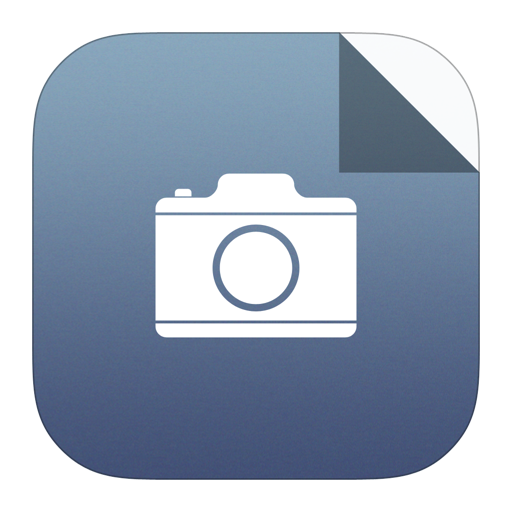
Larger image
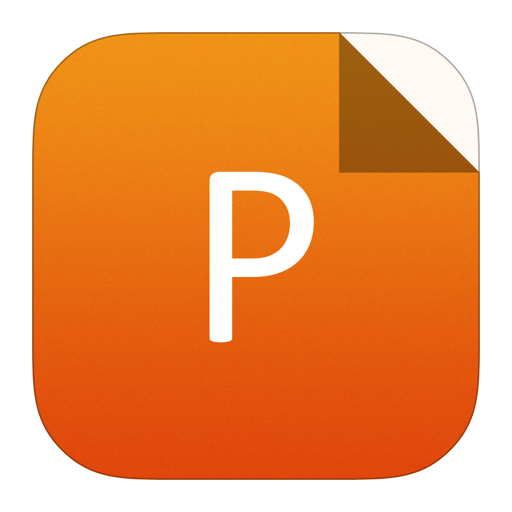
PowerPoint slide
Figure2.
(Color online) Comparison of various 2D materials beyond graphene for SERS applications, including TMDs, BP, h-BN, MXenes, and their heterostructures.
3.1
SERS based on TMDs
TMDs, consisting of covalently bonded X–M–X atoms (M = transition metals of groups IV–VI; X = chalcogen), where M is a transition metal atom (e.g., Mo or W), and X is a chalcogenide atom (e.g., S, Te or Se), have a series of superior properties, ideal for potential use in SERS chips[32-37]. Firstly, as the density of states (DOSs) near the Femi level plays an important role in changing the electron transition probability, TMDs show superior enhancement capability as compared to that of graphene[38]. Secondly, in their thermodynamically 2H phase, MoS2, MoSe2, WS2, and WeSe2 are semiconductors[39], facilitating the photoinduced charge transfer (PICT) effect between substrates and absorbed molecules. It is helpful to increase molecular Raman scattering cross-sections. Finally, TMDs usually have three-layered atomic structures, thereby offering abundant surface sites for chemisorption, with high oscillator strengths in the exciton bands, together with excitonic resonance[19], with the potential to boost Raman signals.
Given these excellent properties, TMDs have been extensively studied for use in SERS chips[40-49]. In 2016, Muehlethaler et al.[50] first observed an EF of




class="figure_img" id="Figure3"/>
Download
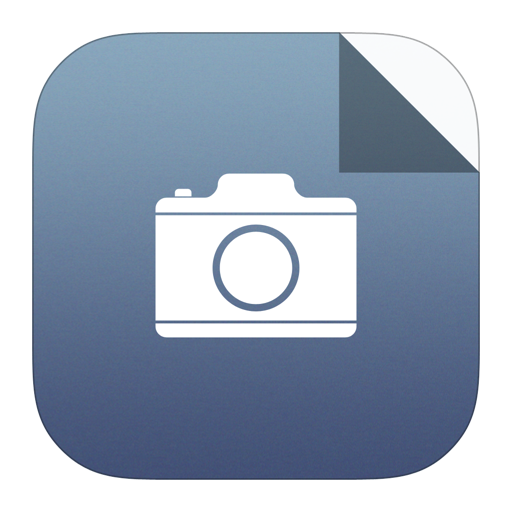
Larger image
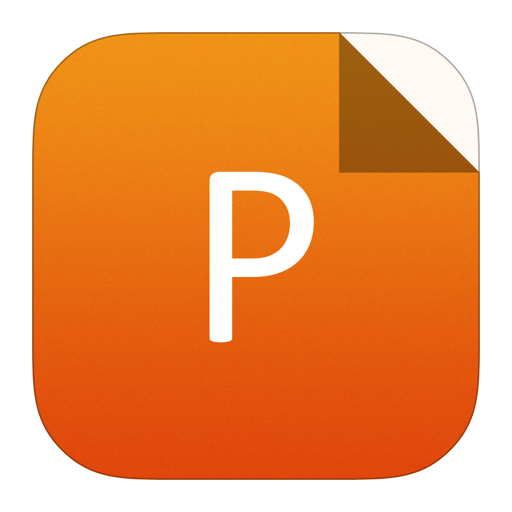
PowerPoint slide
Figure3.
(Color online) SERS studies based on TMDs. (a) Schematic of measurement and enhanced Raman spectra of 4-mercaptopyridine on monolayer MoS2. (b) Energy levels of the oxygen-incorporation MoS2-R6G system. Here,
m{ex}} $
m{mol}} $
m{PICT}} $
m{M}} $
3.2
SERS based on h-BN
h-BN, sp2-hybridized 2D insulator is a structural analog of graphene, with sublattices being occupied by equal numbers of boron and nitrogen atoms, arranged alternately in a honeycomb configuration[59]. Due to their atomic thin surfaces, which are free of dangling bonds, and exhibit negligible defects, as well as only a small lattice constant mismatch (~17%)[60] with graphene, h-BN, also known as “white graphene”, has been studied as an ideal platform for use in photodetectors[61], energy harvest devices[62], electronic packaging[63], and biomedicines[64]. Compared with nonpolar and conductive 2D materials, e.g., graphene, h-BN is highly polar, as well as insulating, due to its large bandgap of 5.9 eV[65]. Moreover, it has a polar surface with high resistance to oxidation[66, 67].
Based on these unique properties, h-BN may be a good alternative to graphene for the purpose of Raman enhancement. It has been proposed that the Raman enhancement mechanism for h-BN may stem from different factors. In 2014, Ling et al.[68] comprehensively compared and studied different SERS chips consisting of graphene, h-BN, and MoS2, respectively, as shown in Fig. 4(a). The enhancement factors of graphene-based and h-BN-based SERS chips are strong, as shown in Fig. 4(b). In contrast to the strong charge transfer between graphene and CuPc, the dipole-dipole interaction between h-BN and CuPc can cause local symmetry-related perturbation to increase LUMO-HOMO transitions, inducing an increase in the probability of electron transition[68]. The high enhancement factors of CuPc on the h-BN SERS chips inspired researchers to explore their possible application in the field of biological sensing. For example, Liu et al. [69] created a novel theragnostic platform, in which CuPc, hairpin quadruplex (HG) DNA, and h-BN were integrated for the purpose of real-time imaging and in-situ monitoring of miR-21. In this way, CuPc was used as a diagnostic sounder for miR-21, while HG-DNA was used to further enhance detection sensitivity.

class="figure_img" id="Figure4"/>
Download
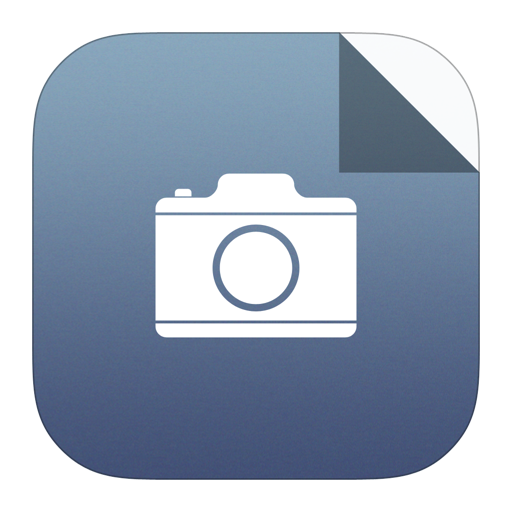
Larger image
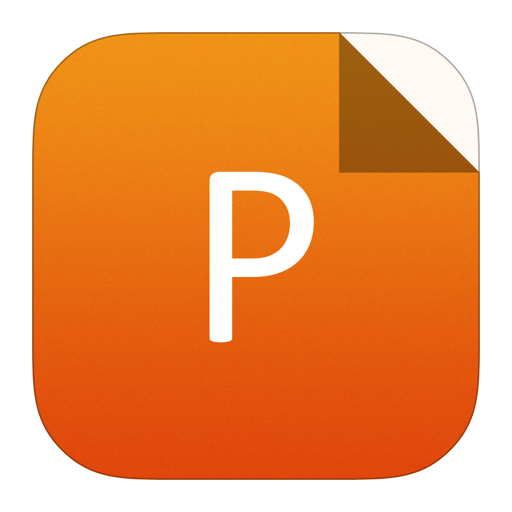
PowerPoint slide
Figure4.
(Color online) SERS studies based on h-BN, BP, and MXenes. (a) Preparation of SERS chips based on graphene, h-BN, and MoS2. The layered 2D materials are shown in gray, while probe molecules are shown in red. (b) Raman spectra of CuPc molecules on SiO2/Si (black line), MoS2 (green line), h-BN (red line), and graphene (blue line) substrates. (c) Raman spectra of RhB molecules (~10–8 M) on a BP substrate, showing different Raman peaks, which could be attributed to different vibrational transitions in the RhB molecules. (d) Schematic of Ti2NTx, etched and delaminated from Ti2AlN, and employed as a SERS substrate. (a) and (b) are reprinted with permission from Ref. [68]. ? 2019 Wiley‐VCH Verlag GmbH & Co. KGaA, Weinheim. (c) is reprinted with permission from Ref. [80]. Copyright ? 2019 the Royal Society of Chemistry. (d) is reprinted with permission from Ref. [92]. Copyright ? 2017 American Chemical Society.
3.3
SERS based on BP
Of all the 2D materials, BP demonstrates a series of unique characteristics for use in Raman enhancement. Firstly, BP, as a 2D layered material with anisotropy, can provide detailed information about the charge-transfer process as compared to the use of isotropic materials[70]. Secondly, the bandgap of BP is dependent on its thickness, which is tunable from about 0.3 eV (bulk) to 2.0 eV (monolayer), making it suitable for developing SERS substrates that can be specifically tailored[71]. Finally, as the first-layer effect plays an important role in CMs[72], BP possesses a much higher surface-to-volume ratio than that found in other 2D materials, e.g., graphene and TMDs, due to its puckered lattice configuration[73, 74], maximizing the adsorbing effect of molecules on a chip.
To explore the intrinsic SERS performance of BP[75, 76], a process flow of controlled nano-structuring has been established. Several approaches have been studied in relation to the nano-patterning and thinning of BP flakes, such as plasma oxidation[77], thermal sublimation[78], and laser oxidation processes[79]. For example, Kundu et al. [80] used a low-power laser, at a wavelength of 532 nm, to irradiate few-layer BP flakes. They demonstrated that few-layer BP flakes with a thickness of ~200 nm can be uniformly thinned down to 2–3 layers, with a thickness of ~ 4 nm, by controlling both laser power and exposure time. This promising result may prove useful in the development of metal-free SERS chips with an EF of ~106, and an LOD of ~10–8 M, for RhB detection, as shown in Fig. 4(c). Moreover, due to its in-plane low symmetry and anisotropic charge carrier mobility[81-83], BP is an ideal material with which to investigate how the electronic properties of a substrate can affect its chemical enhancement. Based on a few-layer BP flake on a chip, Lin et al.[84] utilized CuPc molecules as a Raman probe, and observed a unique angle-dependent SERS enhancement, which was attributed to the excellent mobility of charge carriers along its armchair direction, regardless of the molecular orientation of the CuPc.
3.4
SERS based on MXenes
MXenes share a general formula of Mn+1XnTx (n = 1–3), where M is an early transition metal, X is a carbon or nitrogen, and T is the surface termination (O, OH, F, or Cl)[85]. They exhibit unique optoelectronic properties, such as high metallic conductivity and hydrophilicity[86], which are suitable for the development of SERS chips[87-89].
For their proof-of-the-concept, Ye et al.[90] reported the large-scale synthesis of highly crystalline monolayer Ti3C2 nanosheets via an effective chemical exfoliation method. These ultrathin Ti3C2 nanosheets can be assembled into flexible SERS chips with an LOD of 10–11 M, and an EF of up to



3.5
SERS based on 2D-material heterostructures
Recently, 2D van der Waals (vdW) heterostructures, which are assembled by stacking different 2D crystals on top of one another, have been shown to provide promising platforms for developing SERS chips, since they can take advantage of the merits of various SERS materials. For instance, GERS depends on the ground-state charge transfer at the interface, while the enhancement of the Raman scattering is subject to the DOS of graphene[93, 94]. On the other hand, TMDs have a more complex band structure, and abundant electronic states, yet the intensity of the Raman scattering is weak due to a combination of ground-state charge transfer and dipole-dipole coupling[68]. 2D vdW heterostructures of graphene and TMDs could combine the ground-state charge transfer of graphene with the abundant electronic states of TMDs to enhance Raman signals. In addition, layered 2D-material heterostructures facilitate interlayer electronic tunneling to artificially build electronic band structures[95]. As CMs rely heavily on the electronic structures of materials, the electronic band structures of heterostructures with an artificially designed sequence could be anticipated to significantly enhance EFs[16]. Moreover, the weak vdW interface could lead to an enhanced electric dipole moment and dipole-dipole interaction, facilitate effective charge transfer across the vdW interfaces[72, 73], and therefore contribute to an overall improvement in SERS.
Owing to the merits of 2D heterostructures, many efforts have been made to develop 2D heterostructures for studying SERS[96, 97]. In 2017, Tan et al.[16] demonstrated a heterostructure based on graphene (G) and WSe2 (W) monolayers as an efficient platform for SERS. The interlayer distance of the heterostructure was decreased from ~4 to 0.4 nm to achieve an optimized combination of graphene and WSe2. By changing the number and sequence of the stacked layers, i.e., G/W, G/W/G/W, and W/G/G/W, it was demonstrated that the influence of the stacking method on SERS performance primarily depends on the 2D material on the top, as shown in Fig. 5(a), indicating that Raman enhancement due to heterostructures is a surface effect. Heterostructures with more than two layers have a little impact on the enhancement of charge transfer, resulting in a similar performance, in terms of enhancement, with bilayer heterostructures, as shown in Fig. 5(b). Moreover, heterostructures could integrate the merits of two or more types of material exhibiting EM-comparable sensitives when utilized in SERS chips[98, 99]. For example, Qiu et al.[100] developed a highly effective SERS chip, based on graphene-microflowers (GMFs)/2H-MoS2, with a LOD of only



m{ex}} $

m{PICT}} $


class="figure_img" id="Figure5"/>
Download
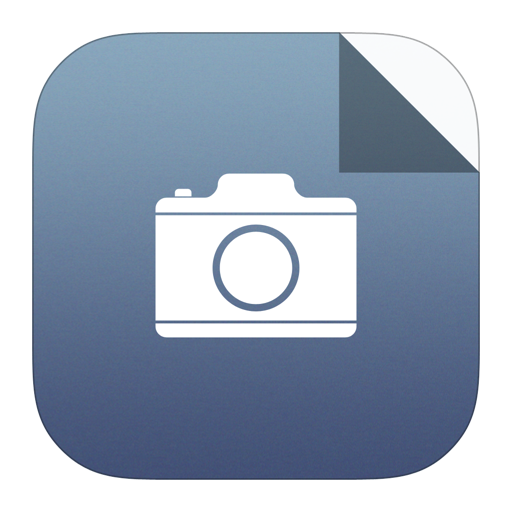
Larger image
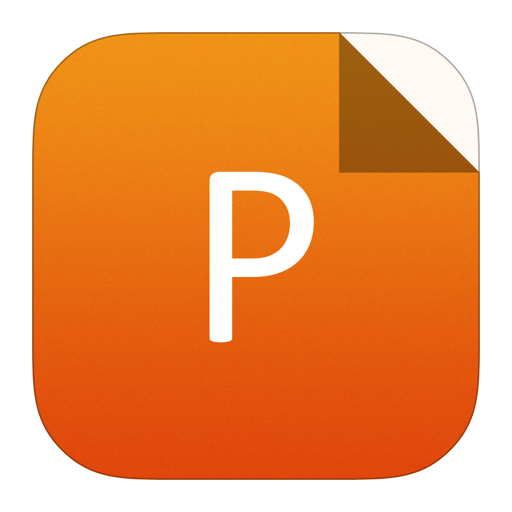
PowerPoint slide
Figure5.
(Color online) SERS studies based on 2D heterostructures. (a) Schematic of Raman measurement of CuPc molecular coating on G/W/G/W chips. (b) Raman spectra of CuPc molecular coating on G/W/G/W and G/W chips, respectively. (c) Schematic of Raman enhancement mechanism of graphene/ReOxSy-MT chips. (d) Energy level diagrams and charge transfer in the R6G-W18O49/MoS2 complex. (a) and (b) are reprinted with permission from Ref. [16]. Copyright ? 2017 American Chemical Society. (c) is reprinted with permission from Ref. [98]. Copyright ? 2020 American Chemical Society. (d) is reprinted with permission from Ref. [104]. Copyright ? 2019 American Chemical Society.
4.
Conclusion and perspective
In this paper, we have reviewed the recent advances in SERS chips based on 2D materials for chemical and biological sensing. Since many excellent review papers of GERS have been published in the past few years[17, 93, 105-112], here, we focused primarily on state-of-the-art SERS experimental results based on other 2D materials, i.e., TMDs, h-BN, BP, MXenes, and their heterostructures, as shown in Table 1. Over 50 papers published in this area have been systematically summarized and categorized. Specifically, we have discussed the structures, physicochemical properties, SERS enhancement capability, Raman enhancement mechanisms, and sensing applications of these substrates. We hope that this snapshot of the status of 2D-material-based SERS chips could arouse more interest in this emerging area.
Materiala) | Detection moleculeb) | LOD (M) | EF | Wavelength (nm) | Ref. |
MoS2 | R6G | 10–7 | – | 532 | [48] |
MoS2 | 4-MPy | – | 105 | 488 | [50] |
MoS2 | Py-EtOH | – | 109 | 555 | [47] |
MoS2 | R6G | 10–7 | – | 532 | [53] |
MoS2 | R6G | – | 63 | 532 | [52] |
MoS2 | R6G | 10–8 | – | 532 | [55] |
WS2 | R6G | 10–8 | – | 532 | |
WS2 | R6G | 10–7 | – | 532 | [45] |
WS2 | R6G | 10–7 | – | 532 | [42] |
ReS2 | R6G | 10–9 | – | 532 | [46] |
RhB | 10–8 | – | 532 | ||
CV | 10–8 | – | 532 | ||
MB | 10–9 | – | 532 | ||
NbS2 | MeB | 10–14 | – | 633 | [36] |
1T’-MoSe2 | R6G | 10–8 | – | 532 | [57] |
SnSe2 | R6G | 10–12 | 106 | 532 | [34] |
SnS2 | CuPc | 10–10 | 50 | 633 | [33] |
WSe2 | CuPc | 10–10 | 50 | 633 | |
WSe2 | CuPc | – | 120 | 532 | [43] |
WTe2 | R6G | 10–15 | 1010 | 532 | [58] |
MoTe2 | R6G | 10–14 | 109 | – | |
h-BNNS | CuPc-miR-21 | 10–15 | – | 655 | [69] |
BP | CuPc | – | <10 | 633 | [84] |
BP | RhB | 10–8 | 106 | 532 | [80] |
BP | CV | 10–5 | – | 532 | [76] |
Ti3C2Tx | R6G | 10–7 | 106 | 488 | [91] |
MB | 10–7 | 106 | 633 | ||
CV | 10–7 | 105 | 633 | ||
AB | 10–6 | 103 | 514 | ||
r-Ti3C2Tx | CV | 10–6 | 106 | 532 | [87] |
Ti3C2Tx | MB | 10–6 | 105 | – | |
Ti3C2Tx | R6G | 10–6 | 107 | 532 | |
Ti3C2Tx | CV | 10–8 | – | 532 | [88] |
R6G | 10–8 | 104 | 532 | ||
MB | 10–7 | – | 633 | ||
Ti3C2 | R6G | 10–11 | 108 | 532 | [90] |
Ti2N | R6G | – | 1012 | 532 | [92] |
Graphene/WSe2 | CuPc | – | 77 | 633 | [16] |
MoS2 QD/rGO | R6G | 10–9 | 107 | 532 | [99] |
MB | 10–8 | – | 532 | ||
MoS2/ graphene | R6G | 10–12 | – | 532 | [98] |
R6G | 10–11 | – | 532 | ||
W18O49/ MoS2 | R6G | 10–10 | 107 | 532 | [104] |
GMFs/ W-MoS2 | MB | 10–10 | – | – | [100] |
Adenosine | 10–9 | – | – | ||
a)Materials: quantum dot MoS2 hybrid with reduced graphene oxide (MoS2 QD/rGO), wrinkled semiconducting 2H-phase MoS2 platform decorated with graphene-microflowers (GMFs/MoS2). b)Molecules: rhodamine (RhB), rhodamine 6G (R6G), crystal violet (CV), methylene blue (MB), acid blue (AB), copper phthalocyanine (CuPc), pyridine and ethanol (Py-EtOH), and 4-Mercaptopyridine (4-MPy). |
Table1.
Comparison of SERS results based on different substrates.
Table options
-->

Download as CSV
Materiala) | Detection moleculeb) | LOD (M) | EF | Wavelength (nm) | Ref. |
MoS2 | R6G | 10–7 | – | 532 | [48] |
MoS2 | 4-MPy | – | 105 | 488 | [50] |
MoS2 | Py-EtOH | – | 109 | 555 | [47] |
MoS2 | R6G | 10–7 | – | 532 | [53] |
MoS2 | R6G | – | 63 | 532 | [52] |
MoS2 | R6G | 10–8 | – | 532 | [55] |
WS2 | R6G | 10–8 | – | 532 | |
WS2 | R6G | 10–7 | – | 532 | [45] |
WS2 | R6G | 10–7 | – | 532 | [42] |
ReS2 | R6G | 10–9 | – | 532 | [46] |
RhB | 10–8 | – | 532 | ||
CV | 10–8 | – | 532 | ||
MB | 10–9 | – | 532 | ||
NbS2 | MeB | 10–14 | – | 633 | [36] |
1T’-MoSe2 | R6G | 10–8 | – | 532 | [57] |
SnSe2 | R6G | 10–12 | 106 | 532 | [34] |
SnS2 | CuPc | 10–10 | 50 | 633 | [33] |
WSe2 | CuPc | 10–10 | 50 | 633 | |
WSe2 | CuPc | – | 120 | 532 | [43] |
WTe2 | R6G | 10–15 | 1010 | 532 | [58] |
MoTe2 | R6G | 10–14 | 109 | – | |
h-BNNS | CuPc-miR-21 | 10–15 | – | 655 | [69] |
BP | CuPc | – | <10 | 633 | [84] |
BP | RhB | 10–8 | 106 | 532 | [80] |
BP | CV | 10–5 | – | 532 | [76] |
Ti3C2Tx | R6G | 10–7 | 106 | 488 | [91] |
MB | 10–7 | 106 | 633 | ||
CV | 10–7 | 105 | 633 | ||
AB | 10–6 | 103 | 514 | ||
r-Ti3C2Tx | CV | 10–6 | 106 | 532 | [87] |
Ti3C2Tx | MB | 10–6 | 105 | – | |
Ti3C2Tx | R6G | 10–6 | 107 | 532 | |
Ti3C2Tx | CV | 10–8 | – | 532 | [88] |
R6G | 10–8 | 104 | 532 | ||
MB | 10–7 | – | 633 | ||
Ti3C2 | R6G | 10–11 | 108 | 532 | [90] |
Ti2N | R6G | – | 1012 | 532 | [92] |
Graphene/WSe2 | CuPc | – | 77 | 633 | [16] |
MoS2 QD/rGO | R6G | 10–9 | 107 | 532 | [99] |
MB | 10–8 | – | 532 | ||
MoS2/ graphene | R6G | 10–12 | – | 532 | [98] |
R6G | 10–11 | – | 532 | ||
W18O49/ MoS2 | R6G | 10–10 | 107 | 532 | [104] |
GMFs/ W-MoS2 | MB | 10–10 | – | – | [100] |
Adenosine | 10–9 | – | – | ||
a)Materials: quantum dot MoS2 hybrid with reduced graphene oxide (MoS2 QD/rGO), wrinkled semiconducting 2H-phase MoS2 platform decorated with graphene-microflowers (GMFs/MoS2). b)Molecules: rhodamine (RhB), rhodamine 6G (R6G), crystal violet (CV), methylene blue (MB), acid blue (AB), copper phthalocyanine (CuPc), pyridine and ethanol (Py-EtOH), and 4-Mercaptopyridine (4-MPy). |
Compared with SERS based on metallic materials, 2D-material-based SERS chips are still in development. Firstly, in terms of mechanisms, Raman enhancement of 2D-material substrates mainly originates from CMs, specifically charge transfer resonance and dipole?dipole interaction, which varies based on different substrate materials and analytes. With the discovery of emerging low-dimensional materials, such as MnPS3–xSex[113], and transition metal oxides[114], a wealth of physical and chemical processes in SERS remain to be explored. Compared with the EMs, these are not yet well-understood. Secondly, due to the atomic-layer thickness of 2D materials, their physicochemical properties could be tunable by virtue of applying an external electric-field, tailoring morphologies, or constructing heterostructures consisting of multiple materials (e.g., low-dimensional materials, dielectrics, or metallic materials), representing a significant opportunity to engineer Raman enhancement capability. This unique feature may greatly expand the application scope of the sample analysis based on CMs, since chemical enhancements are usually system-dependent. As a result, this is a promising field, meriting in-depth study. Thirdly, it would be interesting to integrate 2D materials with microfluidic channels[115, 116] or photonic structures[117, 118] to improve light-matter interactions, as well as to explore lab-on-a-chip applications. Finally, the study of biosensing applications based on 2D-material-based SERS chips is in its infancy. Compared with metallic materials, 2D materials offer potential low-cost production, easy synthesis, excellent biocompatibility, and outstanding reproducibility for SERS chips, with great potential for use in a variety of chemical and biological sensing applications, including analytical chemistry, environmental science, pharmaceutical science, food science, forensic science, and pathology. Consequently, we believe tremendous opportunities exist with respect to 2D-material-based SERS chips, which are expected to become the next-generation SERS techniques.
Acknowledgements
This work was supported by the National Natural Science Foundation of China (61805175), Japan Society for the Promotion of Science (JP18K13798), China Postdoctoral Science Foundation (2020M670641).