1.
Introduction
GaN-based LEDs have made significant progress respecting internal quantum efficiency, forward voltage, and chip design to provide high performance over a broad UV-to-green spectral range[1–3]. However, to completely enter high-power applications such as projection, automotive forward lighting, and solid-state lighting, further improvements in light extraction are required. In LEDs, a significant amount of light emitted from the active layer is trapped inside the semiconductor structure because of the total internal reflection at top and bottom surface[4–8], which leads to the very low light extracting efficiency. The light scattering effect is commonly applied to enhance the light extraction, such as patterned sapphire substrate[9–11]. In addition, metallic nanotextured reflectors are also widely used to improve the light scattering effect and provide a greater chance for the trapped light to be extracted[12–18]. However, most previous works neglect the metal loss caused by the SP intrinsic absorption in the metallic nanotextured reflectors, which often occur in SP-based devices[19].
Generally, when the light interacts with the nanotextured reflector, part of the light will couple with the SP mode of the metal nanostructure at the reflector surface, and then transfer the energy to the SP mode. The energy transferred to the SP mode will quickly be dissipated through two channels. One is light re-radiation with the same photon energy of the incident light but with a changed radiation direction, which leads to the strong light scattering effect and an enhanced light extraction; the other channel is the metal intrinsic absorption as heating loss which cause the serious performance degradation of the optoelectronic devices[19]. Here, the influence of the metal intrinsic absorption of the metallic nanotextured reflectors on the LEDs optoelectronic properties were investigated. Two different Ag and Al nanotextured reflectors were applied to the green GaN-based LEDs. It has been found that metal loss strongly impacts the light output of the LEDs, and the LEDs with Ag nanotextured reflectors are much brighter than with Al. Our findings will aid the design of high performance metal nanotextured reflectors and optoelectronics devices.
2.
Experimental
The green LED epilayer was grown on a 2-inch (0001) sapphire substrate using metal-organic chemical vapor deposition (MOCVD). The structure consists of a 2 μm undoped GaN layer, 2 μm n-GaN layer with a carrier concentration of 3 × 1018 cm?3, ten-period In0.23Ga0.77N (2 nm)/GaN (10 nm) MQWs and 200 nm Mg-doped p-GaN layer with a hole concentration of 5 × 1017 cm?3. Afterwards, the LEDs with nanotextured reflectors were processed as follows: firstly, the surface region of the p-GaN layer was partially etched by an inductively coupled plasma-etching process (ICP) using Cl2/BCl3/Ar gases to expose the n-GaN layer, then a 280 nm indium tin oxide (ITO) layer was deposited as a transparent current-spreading layer on the p-GaN layer followed by annealing in ambient N2at 550 °C for 30 min. To form the p-type and n-type electrode, Cr/Pt/Au (30/100/1500 nm) metal layers were deposted using electron beam (EB) evaporation. This device structure corresponds to conventional plane LEDs. After the electrode evaporation, a 300 nm thick anodic aluminum oxide (AAO) membrane was transferred to the LED chip wafer. The details of the transfer method have been reported elsewhere[20]. Then the ITO layer was etched into nanoporous structure using the AAO membrane as an ICP mask. The Ar gas was used with the chamber pressure kept at 10 mTorr. The ICP power and RF power were set at 200 and 100 W, respectively. After the ICP process, the residues of the AAO template was removed by dilute NaOH solution. Finally, 500 nm Ag and Al were evaporated onto the nanoporous ITO layer of the nanotextured LEDs respectively to form LEDs with nanotextured reflectors. The device structures of the LEDs mentioned above were shown in Fig. 1. The light emission of these LEDs was detected from the substrate side with the flip-chip package.

class="figure_img" id="Figure1"/>
Download
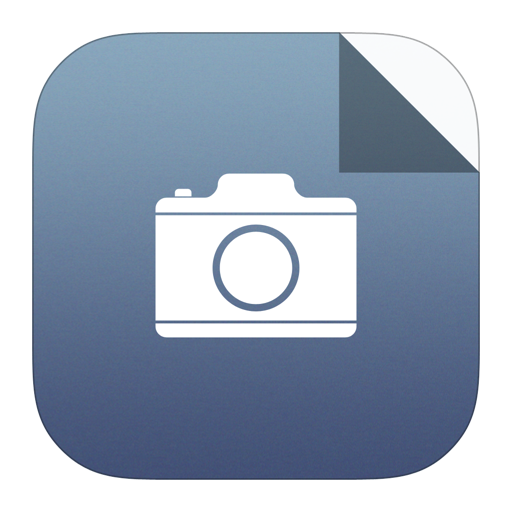
Larger image
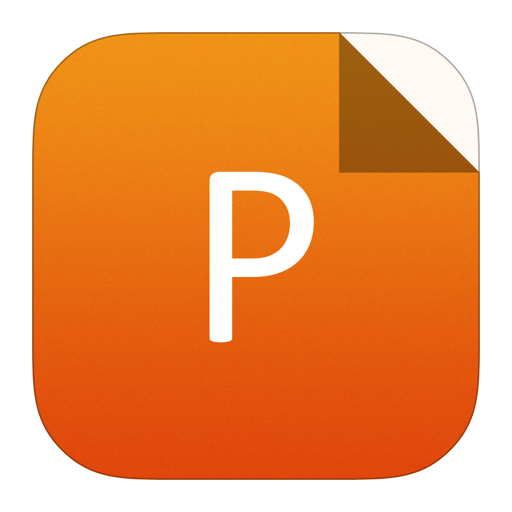
PowerPoint slide
Figure1.
(Color online) The device structures of the (a) conventional plane LEDs and (b) LEDs with nanotextured reflectors.
3.
Results and discussion
Fig. 2(a) shows the optical image of an AAO template on the conventional LED wafer. The pore diameter and pore interval of the AAO template are mainly distributed at 330 ± 30 and 430 ± 30 nm, respectively, as shown in Fig. 2(b). Using this AAO template as an ICP etching mask, the nanoporous structures are well transferred to the ITO layer, as shown in Fig. 2(c). The pore interval of the nanoporous ITO was also 430 ± 30 nm, with the diameter of 260 ± 30 nm which is smaller than that of the AAO template. The smaller pore diameter of ITO-GaN surface was caused by the re-deposition of ICP etching residues on the pore wall of the AAO template[21]. Fig. 2(d) shows the SEM cross-section image of nanotextured metallic reflector deposited onto the nanoporous ITO layer. The heights of the protrusions on the lower surface are about 280 nm, which just corresponds to the thickness of the ITO layer.

class="figure_img" id="Figure2"/>
Download
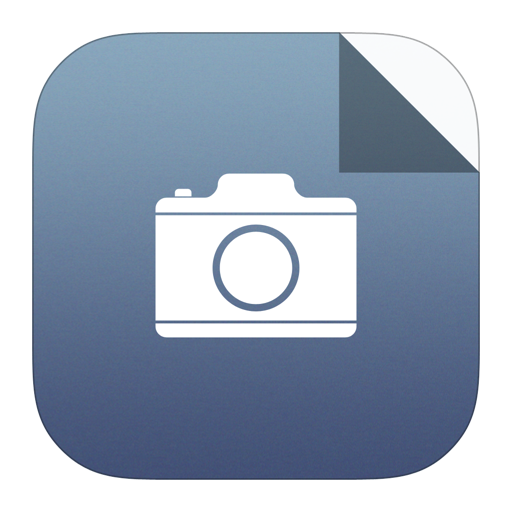
Larger image
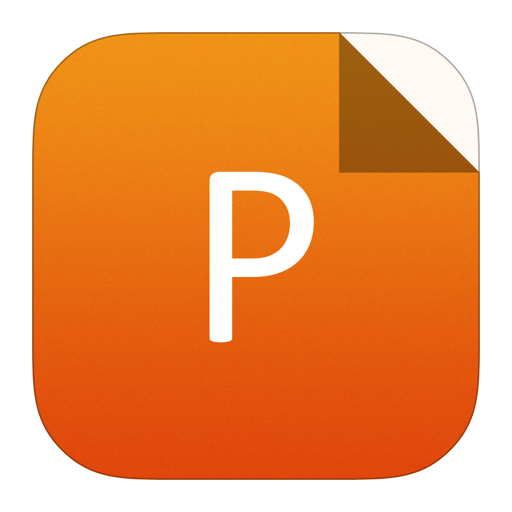
PowerPoint slide
Figure2.
(Color online) (a) Optical image and (b) scanning electron microscopy (SEM) image of an AAO template on the conventional plane LED wafer. The white region corresponds to the AAO template. (c) SEM images of nanotextured ITO layer and (d) nanotextured metallic reflector.
Fig. 3(a) shows the light output power as a function of the injection current for these various LEDs. By applying a Ag nanotextured reflector to conventional plane LEDs, the light output power were greatly enhanced by 78%. However, for the case of the Al reflector, the application of an Al nanotextured reflector caused only 6% light output power enhancement. This light output power difference between the LEDs with Ag and Al nanotextured reflectors may be related to SP absorption. Fig. 3(b) shows the I–V characteristics of LEDs with and without metal reflectors. The LEDs with different metal reflectors have similar electrical properties. On the other hand, the LEDs with metal reflectors have a relatively smaller operation voltage than without metal reflectors, which is due to the 500 nm Al or Ag metal film providing a much greater conductive capacity than that of the 280 nm ITO film, leading to better current spreading of the p-type contact. The reverse current at ?5 V was lower than 10?7 A/cm2 for all the LEDs, as shown in the inset of Fig. 3(b), indicating that the metallic nanotextured reflectors did not induce additional current leakage path, and the light output power difference between LEDs with different metal reflectors were not caused by a change in electrical property.

class="figure_img" id="Figure3"/>
Download
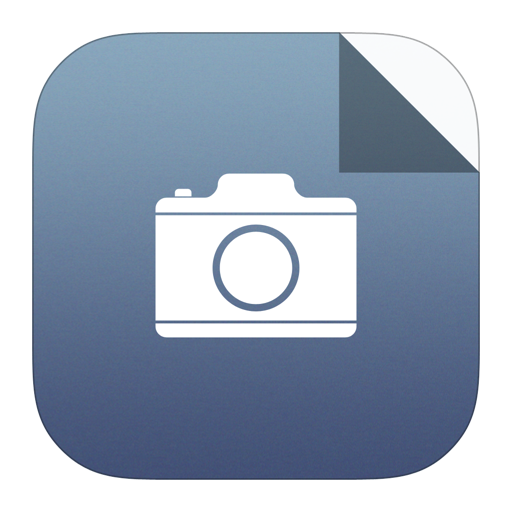
Larger image
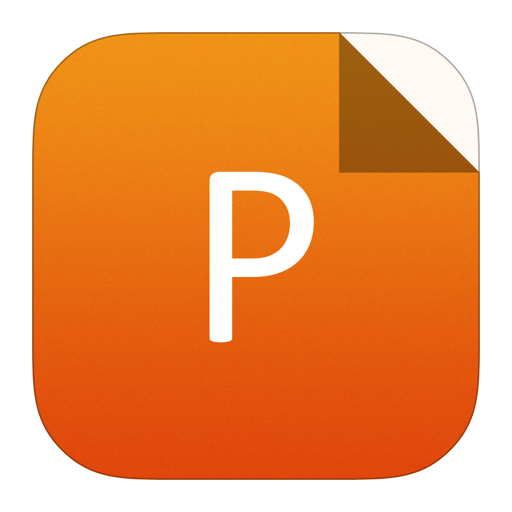
PowerPoint slide
Figure3.
(Color online) (a) Light output power as a function of the injection current for conventional plane LEDs and LEDs with metal nanotextured reflectors. (b) I–V characteristics of LEDs with and without metal reflectors. The inset shows the local amplification of the I–V curves with the voltage below the turn-on voltage.
In order to clarify the light output power difference between the LEDs with Ag and Al nanotextured reflectors, the reflectivity and optical images of Ag and Al nanotextured reflectors were measured from the polished substrate side, as shown in Fig. 4(a). The Ag reflector has the higher reflectivity in the visible region and a brighter optical image compared with the Al reflector. This indicated that Ag nanotextured reflectors have less light absorption loss and stronger light scattering ability. To further analyze the interaction between the light and the metallic nanotextured reflectors, absorption spectrums of the metallic nanotextured reflectors were simulated using three-dimensional (3D) finite-difference time-domain (Lumerical FDTD Solutions), as shown in Fig. 4(b). The simulated models have a similar surface morphology and dielectric environment as the experimental metallic nanotextured reflectors. The numerical simulation showed that the Ag nanotextured reflector has a lower absorption loss than the Al nanotextured reflector in the visible region, which is consistent with the measured reflectivity. It need to be noted that the simulated absorption spectrum slightly deviates from the experimental one in the wavelength regimes of 400–430 nm, which may be due to the different morphology of the experimental and the simulated nanorods. Meanwhile, the SP field distribution near the surface of the Ag nanotextured reflectors is much stronger than that near the Al nanotextured reflector, as shown in Fig. 4(c). This should be related to the metal loss caused by the surface plasmon absorption when the light expose to the metallic reflectors with nanotexture structure. By the interaction between light and metal nanotextured reflectors, part of the light will couple with the surface plasmon mode of the metal nanostructure at the reflector surface, and transfer the energy to the SP mode, as shown in Fig. 4(d). The energy transferred to the SP mode will soon be dissipated through two channels. One is the light re-radiation with the same photon energy of the incident light but the relative random radiation direction[22], which leads to the strong light scattering effect and the light extraction improvement[8]; the other one is the metal intrinsic absorption as heating loss which leads to the serious light extraction decrease. For different metals, Ag has a much lower SP intrinsic absorption than that of Al due to the lower interband transitions loss and intraband transitions loss in the visible region[23], which leads to a larger light scattering efficiency and the greater light output power enhancement of LEDs with Ag nanotextured reflectors. Our finding indicates that SP intrinsic absorption of metallic nanotextured reflectors play an important role in optoelectronics devices, and Ag is a relative suitable materials for nanotextured reflectors in the visible region.

class="figure_img" id="Figure4"/>
Download
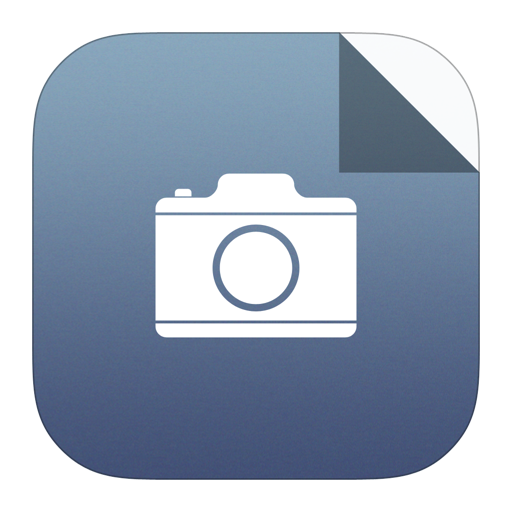
Larger image
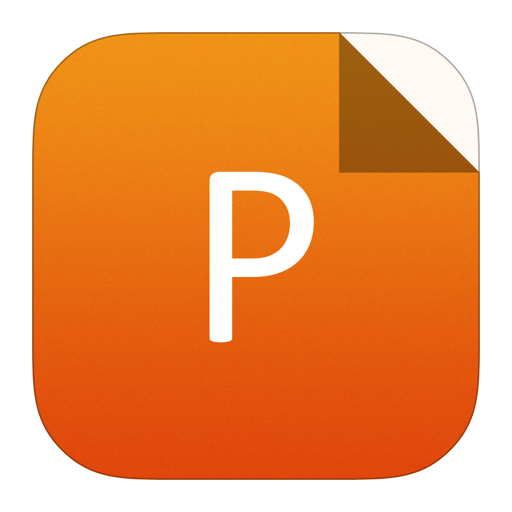
PowerPoint slide
Figure4.
(Color online) (a) The reflectivity spectrum and optical images of Ag and Al nanotextured reflectors. (b) Absorption spectrum of Ag and Al nanotextured reflectors simulated using three-dimensional finite-difference time-domain. (c) SP field distribution (|E|2) near the surface of the Ag and Al nanotextured reflectors. (d) Schematic of interaction between light and metallic reflector.
4.
Conclusion
The influence of the SP intrinsic absorption loss for the metallic reflectors with nanotexture structure on the LEDs optoelectronic properties were investigated. By applying a Ag nanotextured reflector, the light output power of the LEDs was enhanced by 78% due to the improved light extraction. But for an Al nanotextured reflector, only 6% enhancement of the light output power was achieved. By analyzing the simulated absorption spectrum and SP field distribution of the Ag and Al nanotextured reflectors, we found that metal loss strongly impact the light output of the LEDs and Ag is a much more suitable material in the visible region for nanotextured reflectors than Al. Our finding will aid the design of the high-performance metal nanotextured reflectors and optoelectronics devices.
?
Acknowledgements
This work was supported by the National Key Research and Development Program of China (No. 2017YFB0402900) and the National Natural Science Foundation of China (No. 61504132, 61505197).